INTRODUCTION
Mild cognitive impairment (MCI), which is characterized by cognitive decline with preserved independence of function in daily life, is considered to be the intermediate stage between normal aging and mild dementia (Petersen, Reference Petersen2004). Amnestic MCI (aMCI), which is typically characterized by a deficit in memory ability, is strongly associated with progression to Alzheimer’s disease (AD) and has been most commonly studied in that context (Petersen & Morris, Reference Petersen and Morris2005). In a 4-year longitudinal study, the conversion rate to AD was approximately 12% per year among aMCI subjects but only 1–2% among age-matched healthy subjects (Petersen et al., Reference Petersen, Smith, Waring, Ivnik, Tangalos and Kokmen1999).
Memory, which is defined as information processing that comprises encoding, storage and retrieval, can be enhanced by learning strategies (Baddeley, Reference Baddeley2001). Two common encoding strategies for verbal learning are semantic clustering and serial clustering. Semantic clustering is an effective learning strategy in which subjects actively reorganize items based on a shared semantic feature and then recall the words according to their superordinate category; in contrast, serial clustering is an automatic and more passive strategy in which individuals recall words based on their original order of presentation (Delis, Kramer, Kaplan, & Ober, Reference Delis, Kramer, Kaplan and Ober2000). The semantic learning strategy, which is based on internal cues from the word list, has a stronger influence on memory than the serial learning strategy (Hutchens et al., Reference Hutchens, Kinsella, Ong, Pike, Parsons, Storey and Clare2012). Semantic clustering is positively correlated with the recall scores of cognitively healthy individuals on verbal memory tests (Sunderaraman, Blumen, DeMatteo, Apa, & Cosentino, Reference Sunderaraman, Blumen, DeMatteo, Apa and Cosentino2013), and memory impairment is attributable to reduced use of the semantic strategy in several mental and neurological disorders, such as bipolar disorder (Nitzburg et al., Reference Nitzburg, Cuesta-Diaz, Ospina, Russo, Shanahan, Perez-Rodriguez and Burdick2017), schizophrenia (Gsottschneider et al., Reference Gsottschneider, Keller, Pitschel-Walz, Frobose, Bauml and Jahn2011), traumatic brain injury (Geary, Kraus, Rubin, Pliskin, & Little, Reference Geary, Kraus, Rubin, Pliskin and Little2011), and Parkinson’s disease (Bronnick, Alves, Aarsland, Tysnes, & Larsen, Reference Bronnick, Alves, Aarsland, Tysnes and Larsen2011). Moreover, improving the semantic strategy by providing participants with an external organizational cue before learning was previously shown to increase recall scores in both healthy individuals and patients with schizophrenia or traumatic brain injury (Chan et al., Reference Chan, Kwok, Chiu, Lam, Pang and Chow2000; Strangman et al., Reference Strangman, O’Neil-Pirozzi, Goldstein, Kelkar, Katz, Burke and Glenn2008). It has been reported that patients with AD do not use the semantic learning strategy efficiently during memory processing (Carlesimo et al., Reference Carlesimo, Mauri, Graceffa, Fadda, Loasses, Lorusso and Caltagirone1998; Delis & Massman, Reference Delis and Massman1991; Perri, Carlesimo, Serra, & Caltagirone, Reference Perri, Carlesimo, Serra and Caltagirone2005). Furthermore, reductions in semantic clustering during both learning and recall trials, as measured by a verbal learning test, have also been observed in MCI subjects (Ribeiro, Guerreiro, & De Mendonca, Reference Ribeiro, Guerreiro and De Mendonca2007).
Executive function, which is generally conceptualized as controlling or guiding behavior in a top-down fashion, including decision-making, planning, self-monitoring, and behavior initiation, organization and inhibition (Anderson & Tranel, Reference Anderson and Tranel2002), has also been considered to be impaired in subjects with MCI (Ribeiro et al., Reference Ribeiro, Guerreiro and De Mendonca2007) and is associated with the brain atrophy observed in the prefrontal and temporal cortices in such patients (Zheng et al., Reference Zheng, Sun, Dong, Liu, Xu, Chen and Wang2014). It has been reported that semantic processing might be related to or mediated by the executive system based on increased brain activity in left prefrontal regions, as measured by positron emission tomography (PET), during spontaneous and directed application of a verbal learning strategy task in cognitively healthy individuals (Savage et al., Reference Savage, Deckersbach, Heckers, Wagner, Schacter, Alpert and Rauch2001). It has been further observed in aMCI subjects that reduced executive processing contributes to poor semantic clustering and difficulties in detecting the semantic structure of a list (McLaughlin et al., Reference McLaughlin, Wright, Larocca, Nguyen, Teng, Apostolova and Woo2014). In contrast, Malek-Ahmadi et al. reported that executive function correlated with verbal recall but not semantic clustering indices, as measured by the Hopkins Verbal Learning Test-Revised (HVLT-R), in aMCI subjects (Malek-Ahmadi, Raj, & Small, Reference Malek-Ahmadi, Raj and Small2011). Therefore, although a relationship between executive function and memory has been identified (Daly et al., Reference Daly, Zaitchik, Copeland, Schmahmann, Gunther and Albert2000), the contributions of executive function to learning strategies are still controversial.
Memory impairment in individuals with aMCI is thought to be the result of early neuropathological changes in related brain structures, such as medial temporal lobe atrophy (MTA) and white matter hyperintensities (WMH), which have been observed on magnetic resonance imaging (MRI) (Fox et al., Reference Fox, Warrington, Freeborough, Hartikainen, Kennedy, Stevens and Rossor1996). MTA, especially in the hippocampus, is considered a diagnostic biomarker of AD and is thought to be related to episodic memory impairment in patients with AD or MCI (Das, Mancuso, Olson, Arnold, & Wolk, Reference Das, Mancuso, Olson, Arnold and Wolk2016; England, Gillis, & Hampstead, Reference England, Gillis and Hampstead2014; La et al., Reference La, Korf, Wm, Brashear, Fox, Barkhof and Scheltens2007; Pantel, Schönknecht, Essig, & Schröder, Reference Pantel, Schönknecht, Essig and Schröder2004; Sanchez-Benavides et al., Reference Sanchez-Benavides, Gomez-Anson, Molinuevo, Blesa, Monte, Buschke and Pena-Casanova2010; Shim et al., Reference Shim, Youn, Na, Kim, Cheong, Moon and Seo2011; Stelmokas et al., Reference Stelmokas, Yassay, Giordani, Dodge, Dinov, Bhaumik and Hampstead2017). Moreover, a brain network that includes the medial temporal lobe and contributes to the development of learning strategies has been observed in neuroimaging studies. The majority of activity is concentrated in the left hemisphere, although both sides of the brain are activated during semantic processing (Baker, Sanders, Maccotta, & Buckner, Reference Baker, Sanders, Maccotta and Buckner2001). The left-lateralized network is comprised of seven regions, including the posterior inferior parietal lobe, middle temporal gyrus, fusiform and parahippocampal gyri, dorsomedial prefrontal cortex, inferior frontal gyrus, ventromedial prefrontal cortex, and posterior cingulate gyrus, which have been shown to be activated during semantic processing by a quantitative meta-analysis (Binder, Desai, Graves, & Conant, Reference Binder, Desai, Graves and Conant2009). The significant roles of the frontal and temporal areas, especially the medial temporal lobe, in the semantic clustering process have been reported in several studies (Becker & Lim, Reference Becker and Lim2003; Manning, Sperling, Sharan, Rosenberg, & Kahana, Reference Manning, Sperling, Sharan, Rosenberg and Kahana2012). Additionally, WMH, which are generally viewed as evidence of small vessel disease, are commonly observed on MRI across the cognitive impairment spectrum (Burns et al., Reference Burns, Church, Johnson, Xiong, Marcus, Fotenos and Buckner2005), including in patients with AD and MCI (Mendonça, Ribeiro, Guerreiro, Palma, & Garcia, Reference Mendonça, Ribeiro, Guerreiro, Palma and Garcia2005). Disruption of white matter tracts connecting cortical regions, especially frontal-subcortical pathways, is a possible mechanism for memory impairment (Geschwind, Reference Geschwind1965). Although it has been observed that MTA severity and WMH volume are negatively correlated with episodic memory in subjects with MCI (Fujishima et al., Reference Fujishima, Maikusa, Nakamura, Nakatsuka, Matsuda and Meguro2014), whether learning strategy changes are involved in these effects remains unknown.
Few studies have focused on the brain network involved in serial clustering. An early study indicated that patients with left hemispheric lesions tend to have increased serial clustering during word learning compared to that of patients with right hemispheric lesions (Hildebrandt, Brand, & Sachsenheimer, Reference Hildebrandt, Brand and Sachsenheimer1998). However, serial clustering has not been observed to correlate with the volume of any area in terms of gray matter volume in an MRI study (Kirchhoff, Gordon, & Head, Reference Kirchhoff, Gordon and Head2014).
In our previous study, a decline in semantic clustering, as measured by the HVLT-R, was observed in subjects with aMCI compared to subjects with vascular cognitive impairment-no dementia (VCIND) and healthy controls (HCs) (Sun et al., Reference Sun, Luo, Ren, Wei, Xing, Cheng and Zhang2016). In the present study, the MTA visual rating scale and the Fazekas rating scale, which are well validated and easier to perform in clinical practice than volumetric assessment, were used to evaluate MTA and WMH, respectively, in subjects with aMCI. Correlations of learning strategies with executive function and brain structural changes were further analyzed in this cohort.
METHODS
Participants
A total of 96 subjects with aMCI, with ages ranging from 55 to 85 years, were recruited from April 2015 to July 2016 at the memory clinic of Tianjin Medical University General Hospital. The study was approved by the Ethics Committee of Tianjin Medical University General Hospital. Written informed consent was obtained from all participants. The evaluation procedure consisted of a detailed medical history, physical and neurologic examinations, psychiatric and cognitive evaluations, laboratory tests, and brain MRI.
The subjects with aMCI (including single- and multiple-domain aMCI) were included according to the following criteria of Petersen and colleagues (Reference Petersen, Doody, Kurz, Mohs, Morris, Rabins and Winblad2001): (1) subjective memory complaints, usually corroborated by an informant; (2) objective memory impairment of 1.5 or more standard deviations (SDs) below the age- and education-corrected norm for delayed recall on the Logical Memory subtest of the Wechsler Memory Scale-Chinese Revised (Gong, Reference Gong1989; Wechsler, Reference Wechsler1987), which has been validated and widely used in China; (3) preserved general cognitive function, as indicated by a Mini-Mental State Examination (MMSE) (Folstein, Folstein, & Mchugh, Reference Folstein, Folstein and Mchugh1975) score ≥24; (4) intact activities of daily living (ADL), as indicated by a 20-item scale score ≤22 (He et al., Reference He, Qu, Xiong, Chi, Zhang and Zhang1990), which was modified from Lawton and Brody’s scale (Lawton & Brody, Reference Lawton and Brody1969) and included both instrumental and basic ADL; and (5) absence of dementia, according to the Diagnostic and Statistical Manual of Mental Disorders, Fourth Edition (DSM-IV) (Bell, Reference Bell1994), criteria for dementia and a Clinical Dementia Rating (CDR) (Berg, Reference Berg1988) ≤0.5.
Ninety age- and gender-matched HCs were recruited at the physical examination center of the same hospital. The inclusion criteria for HCs included the following: (1) age range from 55 to 85 years; (2) absence of subjective memory complaints; (3) performance within the normal range on neuropsychological measures (within 1.5 SDs of the age- and education-corrected norm for delayed recall on the Logical Memory subtest of the Wechsler Memory Scale-Chinese Revised); (4) an MMSE score ≥26; and (5) a global score of zero on the CDR.
All participants had at least an elementary education and no medical treatments that might affect cognitive function, such as cholinesterase inhibitors or memantine. The exclusion criteria for both groups were as follows: (1) significant visual and/or auditory impairment; (2) presence of significant medical, neurological, or psychiatric illness (e.g., severe depression based on a Beck Depression Inventory (BDI) (Zheng & Lin, Reference Zheng and Lin1991) score ≥16) likely to impact cognitive ability; and (3) history of alcohol or substance abuse.
Among all the participants, 36 aMCI subjects and 26 HCs from our previous study (Sun et al., Reference Sun, Luo, Ren, Wei, Xing, Cheng and Zhang2016) were also recruited, and the MRI and neuropsychological data acquired at that time complied with the present protocol.
Neuropsychological Assessment
All participants were administered a neuropsychological battery in the proper order, including the HVLT-R (Shi et al., Reference Shi, Kang, Yao, Ma, Li, Liang and Yu2015), Trail Making Test-A (TMT-A) and TMT-B (Rossini & Karl, Reference Rossini and Karl1994), the Wisconsin Card Sorting Test (WCST) (Mok et al., Reference Mok, Wong, Yim, Fu, Lam, Hui and Wong2004), the Paced Auditory Serial Addition Test (PASAT) (Gronwall, Reference Gronwall2011), and the Symbol Digit Modalities Test (SDMT) (Smith, Reference Smith1982), by a trained investigator who was blinded to the participants’ clinical conditions. Memory and learning abilities were evaluated according to the total learning, delayed recall and recognition scores on the HVLT-R. The word list in this Chinese version of the HVLT-R has been slightly modified by a team of experts according to cultural and linguistic factors, and the number of syllables and frequency of the words used were also taken into account. For example, in accordance with the familiarity of most Chinese people, the words “coin”, “Er Hu” (a traditional Chinese stringed instrument), and “He Shang” (Buddhist Monk) were used instead of “penny”, “violin” and “priest”, respectively. Indices based on TMT-A and TMT-B (ΔTMT, defined as the TMT-B score minus the TMT-A score; TMT B/A, the ratio of the TMT-B score to the TMT-A score) and the numbers of total errors (TE), perseverative errors (PE), and categories achieved (CA) on the WCST were used to test executive function. Higher ΔTMT, TMT B/A, WCST-TE, and WCST-PE scores and a lower WCST-CA score indicate worse performance in executive function. The number of correct responses on the PASAT and SDMT was used to assess the information processing speed. The procedures for all the neuropsychological assessments were conducted as previously described (Sun et al., Reference Sun, Luo, Ren, Wei, Xing, Cheng and Zhang2016).
Semantic and Serial Clustering in the HVLT-R
The HVLT-R consists of 3 learning trials that include a list of 12 words divided into three semantic categories (jewels, animals, and dwellings). Once the 12 words had been read, the participants were asked to recall as many words as they could remember. After a 20-min interval, the participants were asked to complete the delayed recall task. After the delayed recall task, a yes–no recognition task was administered, including 12 target and 12 nontarget words (6 semantically related, 6 semantically unrelated). A semantic cluster was defined as any time a participant recalled a correct word immediately following another correct word from the same category (i.e., sapphire and emerald belong to jewels) (Gaines, Shapiro, Alt, & Benedict, Reference Gaines, Shapiro, Alt and Benedict2006). A serial cluster referred to the consecutive recall of two spatially related words (bidirectional) contained in a word list (Meijs et al., Reference Meijs, Hurks, Kalff, Slaats-Willemse, Rozendaal and Jolles2009). As more words are recalled, it is thought that the number of semantic and serial clusters during recall could increase simply by chance. Therefore, the semantic clustering ratio and serial clustering ratio, which were calculated by dividing the number of semantic clusters and serial clusters, respectively, by the total number of words correctly recalled across the three learning trials, were used to evaluate the learning strategies of all participants. The possible score range for the semantic clustering ratio was 0–0.75, and the possible score range for the serial clustering ratio was 0–0.92. A higher score indicated greater use of the respective learning strategy.
MRI Acquisition
Within 1 week after the neuropsychological assessment, MRI assessments were performed on a 3.0 T clinical scanner (Discovery MR750, General Electric, Milwaukee, WI, USA) with an 8-channel phase-array coil for all aMCI subjects. Tight but comfortable foam padding was used to minimize head movement, and earplugs were used to reduce any scanner noise. The MRI acquisition protocol for this study included sagittal 3D T1-weighted images with a brain volume sequence (echo time/repetition time = 3.2 ms/8.2 ms; flip angle = 12°; field of view (FOV) = 256 × 256 mm; matrix = 256 × 256; section thickness = 1 mm; no gap; and number of slices = 192), T2-weighted axial images (echo time/repetition time = 92.928 ms/6816 ms; flip angle = 142°; FOV = 240 × 240 mm; matrix = 512 × 512; section thickness = 6 mm; section gap = 1.5 mm; and 18 axial sections), and fluid-attenuated inversion recovery (FLAIR) images (echo time/repetition time = 149.58 ms/8400 ms; flip angle = 111°; FOV = 240 × 240 mm; matrix = 256 × 192; section thickness = 6 mm; section gap = 1.5 mm; and 18 axial sections).
Visual Rating of MTA and WMH
To enhance reliability, MTA and WMH were evaluated by both a neuroradiologist (Y.Z.) and a neurologist (Y.W.) who were blinded to the clinical data. Both raters received professional training for consistency. Although each case was rated by each rater individually, a consensus was ultimately reached for the MTA and WMH scores. T1-weighted images were viewed in the coronal plane, and MTA was evaluated separately for the right and the left sides according to a five-point scale (0 = absent, 1 = minimal, 2 = mild, 3 = moderate, and 4 = severe) that was developed and validated by Scheltens et al. (Scheltens et al., Reference Scheltens, Leys, Barkhof, Huglo, Weinstein, Vermersch and Valk1992). This scale considers both the shrinkage of the hippocampal formation (the hippocampus and the parahippocampal gyrus) and the enlargement of the surrounding cerebrospinal fluid spaces (the width of the choroid fissure and the width of the temporal horn). According to the mean MTA scores of both sides, aMCI subjects were dichotomized into a no atrophy subgroup (mean MTA score <1.5) and an atrophy subgroup (mean MTA score ≥1.5) (La et al., Reference La, Korf, Wm, Brashear, Fox, Barkhof and Scheltens2007).
On the T2-weighted axial images and FLAIR images, the severity of WMH was evaluated according to the modified criteria of Fazekas et al. (Reference Fazekas, Chawluk, Alavi, Hurtig and Zimmerman1987). The Fazekas scale provides evaluations from two different areas, including periventricular hyperintensity (PVH) and deep white matter hyperintensity (DWMH), which are rated on a 0–3 point scale of increasing severity. Participants were classified as having no lesion or mild, moderate, or severe lesions (0, 1, 2, or 3 points, respectively) in each location. The sum of the PVH and DWMH scores provides a total WMH score. According to the severity of WMH, aMCI subjects were divided into a low-grade WMH subgroup (total WMH score ≤1) and a high-grade WMH subgroup (total WMH score >1) (Sorianoraya et al., Reference Sorianoraya, Miralbell, Lópezcancio, Bargalló, Arenillas, Barrios and Dávalos2012).
Statistical Analysis
Statistical analyses were performed using SPSS 21.0 (SPSS Inc., USA). Values of P < .05 were regarded as significant. Raw scores from the neuropsychological tests were used for analyses in this study. Demographic data from the two groups were analyzed using χ2 tests for categorical data or independent two-sample t-tests for continuous variables. To examine differences in memory, executive function, and learning strategies between the aMCI and HC groups, the data were analyzed with a general linear model (GLM). Partial correlation analyses controlling for age and education were performed to analyze the relationships between memory ability and learning strategies and executive function in aMCI subjects. We did not analyze the correlation in the whole sample because there were no deficits in memory or executive function in the HCs. Finally, we compared the scores for verbal memory, learning strategies and executive function between HCs and aMCI subjects with different degrees of MTA and WMH using a GLM. The Bonferroni correction was applied to adjust for multiple comparisons when applicable. The partial eta squared (ŋp2) value was used to show the effect sizes of the parametric statistical tests, according to Cohen’s (Reference Cohen1988) criteria (Cohen, Reference Cohen1988): ~.01–.06 indicated a small effect size, ~.06–.14 indicated a medium effect size, and ≥.14 indicated a large effect size. The receiver operating characteristic (ROC) curves were calculated by plotting sensitivity against 1-specificity for each score in the clustering ratios for discriminating between the aMCI subjects and the HCs and between the aMCI subjects with and without MTA. The optimal cutoff value was calculated based on the maximum sum of sensitivity and specificity using the ROC curve. Sensitivity refers to the test’s ability to correctly detect positive subjects, i.e., aMCI subjects and aMCI subjects with MTA, who do have the conditions. Specificity relates to the test’s ability to correctly reject negative subjects, i.e., HCs and aMCI subjects without MTA, who do not have the conditions. The positive predictive value (PPV) and negative predictive value (NPV) are defined as the proportions of positive and negative results in clustering ratios that are true positive and true negative results, respectively.
RESULTS
Demographics
The demographic characteristics of aMCI subjects and HCs are shown in Table 1. No significant differences were found between the aMCI and HC groups in terms of age, gender, years of education, handedness, ADL, or BDI scores. Compared to the HCs, aMCI subjects had lower MMSE scores (P < .001).
Table 1. Demographic variables in the aMCI group and the HC group
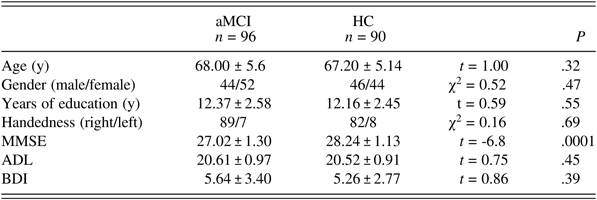
Values represent the mean ± SD unless otherwise indicated. aMCI: amnestic mild cognitive impairment; HC: healthy control; MMSE: Mini-Mental State Examination; ADL: Activities of Daily Living; BDI: Beck Depression Inventory.
Neuropsychological Assessment
Table 2 displays the neuropsychological test performance of the two groups. Generally, aMCI subjects showed poorer performance than the HCs on the HVLT-R (total learning (F (1,184) = 120.06, P < .001, ŋp2 = .43), delayed recall (F (1,184) = 57.30, P < .001, ŋp2 = .24), recognition (F (1,184) = 69.75, P < .001, ŋp2 = .29)), ΔTMT (F (1,184) = 82.83, P < .001, ŋp2 = .31), TMT B/A (F (1,184) = 42.53, P < .001, ŋp2 = .19), the PASAT (F (1,184) = 47.06, P < .001, ŋp2 = .20), and the SDMT (F (1,184) = 115.24, P < .001, ŋp2 = .39). In terms of learning strategies, aMCI subjects demonstrated a lower semantic clustering ratio (F (1,184) = 49.33, P < .001, ŋp2 = .21) than the HCs. There were no differences in the serial clustering ratio or the WCST indices between the two groups (P > .05).
Table 2. Neuropsychological tests in the aMCI group and the HC group

Values represent the mean ± SD. aMCI: amnestic mild cognitive impairment; HC: healthy control; ΔTMT: Trail Making Test-B (TMT-B) minus TMT-A; TMT B/A: TMT-B/TMT-A ratio; WCST: Wisconsin Card Sorting Test; TE: total errors; PE: perseverative errors; CA: category achieved; PASAT: Paced Auditory Serial Addition Test; SDMT: Symbol Digit Modalities Test; HVLT-R: Hopkins Verbal Learning Test-Revised. ***P < .001.
Since no difference in the serial clustering ratio was observed between aMCI subjects and HCs, only the semantic clustering ratio was determined for the ROC curve (Figure 1). The area under the curve (AUC) was 0.79 (95% CI 0.72 to 0.85). With a cutoff value of 0.37, the sensitivity, specificity, PPV, and NPV of the semantic clustering ratio for distinguishing the aMCI subjects from the HCs were 83.3%, 62.2%, 70.2%, and 77.8%, respectively.

Fig. 1. ROC curve of the semantic clustering ratio for discriminating between aMCI subjects and HCs. The AUC is 0.79 (95% CI 0.72–0.85), with a sensitivity, specificity, positive predictive value, and negative predictive value of 83.3% (95% CI 74.0–89.9), 62.2% (95% CI 64.2–99.6), 70.2% (95% CI 60.8–78.2), and 77.8% (95% CI 66.2–86.4), respectively, with a cutoff of 0.37.
Correlation between Memory and Executive Function Measures
Table 3 displays the correlation coefficients between HVLT-R variables and measures of executive function in the aMCI group, controlling for age and education, since it was demonstrated that age and education were correlated with total learning, delayed recall and the semantic clustering ratio (P < .05) in the preanalysis. The total learning score and the delayed recall score negatively correlated with ΔTMT (r = −.47, df = 92, P < .001; r = −.46, df = 92, P < .001), TMT B/A (r = −.30, df = 92, P < .01; r = −.53, df = 92, P < .001), WCST-TE (r = −.36, df = 92, P < .001; r = −.21, df = 92, P < .05), WCST-PE (r = −.40, df = 92, P < .001; r = −.31, df = 92, P < .01), and the serial clustering ratio (r = −.50, df = 92, P < .001; r = −.27, df = 92, P < .05) and positively correlated with WCST-CA (r = .28, df = 92, P < .01; r = .38, df = 92, P < .001) and the semantic clustering ratio (r = .45, df = 92, P < .001; r = .32, df = 92,P < .01). The semantic clustering ratio was negatively correlated with the serial clustering ratio (r = −.33, df = 92, P < .01). The semantic clustering ratio and the serial clustering ratio were not correlated with any executive function measure of the TMT or the WCST (P > .05).
Table 3. Correlation between HVLT-R factors and executive function measures in aMCI subjects

Values represent the r values. Partial correlation analyses were conducted, controlling for age and education. HVLT-R: Hopkins Verbal Learning Test-Revised; aMCI: amnestic mild cognitive impairment; ΔTMT: Trail Making Test-B (TMT-B) minus TMT-A; TMT B/A: TMT-B/TMT-A ratio; WCST: Wisconsin Card Sorting Test; TE: total errors; PE: perseverative errors; CA: category achieved; N/A: not applicable. *P < .05; **P < .01; ***P < .001.
Association between Learning Strategies and Brain Structure Changes Measured with MRI
According to the severity of MTA, aMCI subjects were divided into two subgroups (no atrophy group, n = 76; atrophy group, n = 20). The total learning score (F (1,183) = 76.78, P < .001, ŋp2 = .46), delayed recall score (F (1,183) = 58.57, P < .001, ŋp2 = .39), and semantic clustering ratio (F (1,183) = 42.58, P < .001, ŋp2 = .32) of the HVLT-R were highest in HCs and lowest in aMCI subjects with MTA among the three groups (aMCI without MTA vs. HC, P < .05; aMCI with MTA vs. HC, P < .05; aMCI without MTA vs. aMCI with MTA, P < .05). Although ΔTMT and TMT B/A were higher in aMCI subjects with or without MTA than in HCs (P < .05), these values did not differ between aMCI subjects with MTA and those without MTA (P > .05). There were no differences in performance on the WCST or the serial clustering ratio between aMCI subjects with or without MTA and HCs (P > .05) (Table 4).
Table 4. Learning and memory and executive function in HCs and aMCI subjects with and without MTA

The data were analyzed using GLM. Values represent the mean ± SD. HC: healthy control; MTA: medial temporal lobe atrophy; ΔTMT: Trail Making Test-B (TMT-B) minus TMT-A; TMT B/A: TMT-B/TMT-A ratio; WCST: Wisconsin Card Sorting Test; TE: total errors; PE: perseverative errors; CA: category achieved; HVLT-R: Hopkins Verbal Learning Test-Revised. ***P < .001. After the Bonferroni correction: aaMCI without MTA vs. HC, P < .05; baMCI with MTA vs. HC, P < .05; caMCI without MTA vs. aMCI with MTA, P < .05.
Only the semantic clustering ratio was determined for the ROC curve (Figure 2) because no difference in the serial clustering ratio was observed between aMCI subjects with and without MTA. The AUC was 0.83 (95% CI 0.72–0.94). With a cutoff of 0.23, 65.0% of the aMCI subjects with MTA showed a significant decrease in the semantic clustering ratio, whereas only 7.9% of the aMCI subjects without MTA showed a significant decrease.
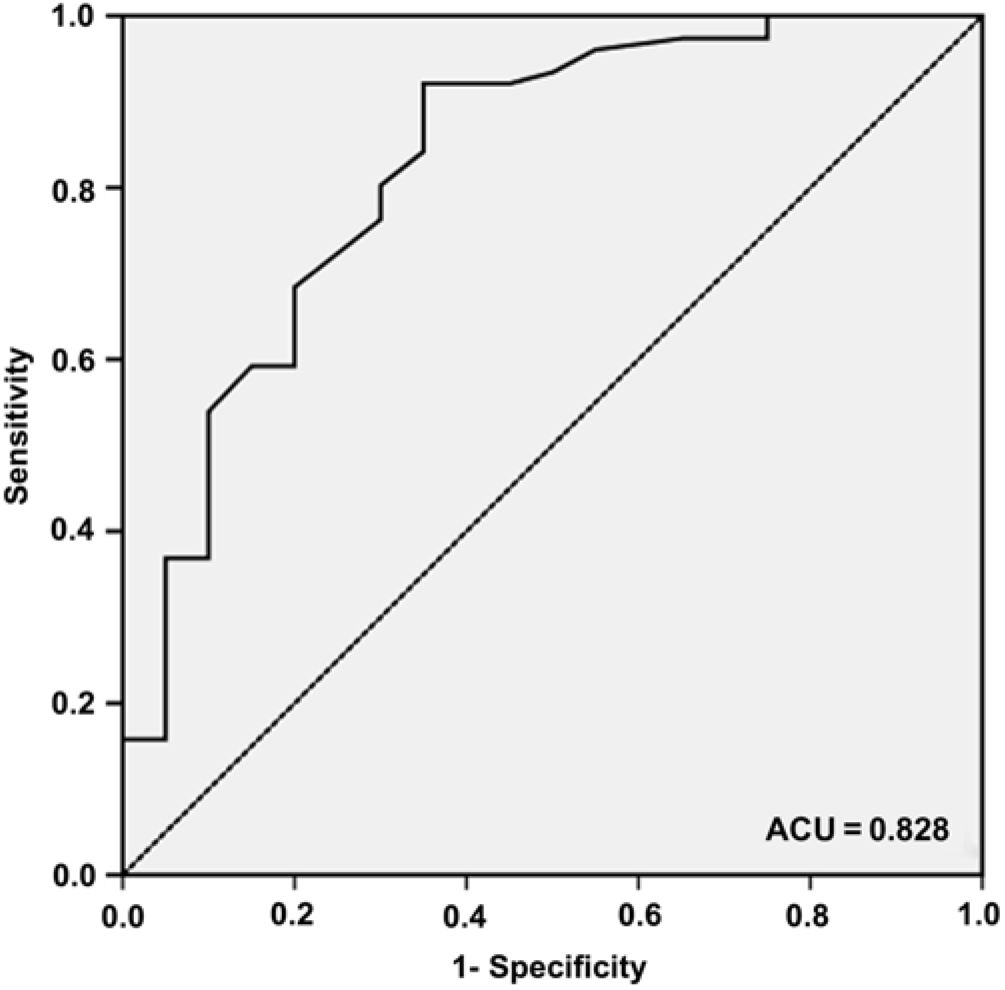
Fig. 2. ROC curve of the semantic clustering ratio for discriminating between aMCI subjects with MTA and those without MTA. The AUC is 0.83 (95% CI 0.72–0.94), with a sensitivity, specificity, positive predictive value, and negative predictive value of 65.0% (95% CI 40.9–83.7), 92.1% (95% CI 83.0–96.7), 68.4% (95% CI 43.5–86.4), and 90.9% (95% CI 81.6–96.0), respectively, with a cutoff of 0.23.
With respect to WMH, the HC group had better performance in total learning (F (1,183) = 56.20, P < .001, ŋp2 = .38), delayed recall (F (1,183) = 67.40, P < .001, ŋp2 = .41) and the semantic clustering ratio (F (1,183) = 27.17 P < .001, ŋp2 = .23) on the HVLT-R, as well as ΔTMT (F (1,183) = 41.95, P < .001, ŋp2 = .31) and TMT B/A (F (1,183) = 23.55, P < .001, ŋp2 = .21), than either the low-grade group (total WMH score ≤1; n = 66; P < .05) or the high-grade group (total WMH score >1; n = 30; P < .05). The high-grade group showed worse performance than the low-grade group in total learning, delayed recall, and ΔTMT (P < .05), but not TMT B/A or the semantic clustering ratio (P > .05). In addition, aMCI subjects with high-grade WMH had worse performance on the WCST-TE and the WCST-PE than HCs (P < .05). There was no difference in the serial clustering ratio or the WCST-CA among the three groups (P > .05) (Table 5).
Table 5. Learning and memory and executive function in HCs and aMCI subjects with low-grade and high-grade WMH
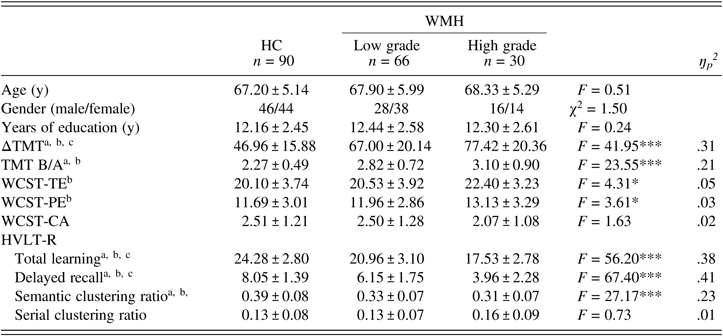
The data were analyzed using GLM. Values represent the mean ± SD. HC: healthy control; WMH: white matter hyperintensities; ΔTMT: Trail Making Test-B (TMT-B) minus TMT-A; TMT B/A: TMT-B/TMT-A ratio; WCST: Wisconsin Card Sorting Test; TE: total errors; PE: perseverative errors; CA: category achieved; HVLT-R: Hopkins Verbal Learning Test-Revised. *P < 0.05; ***P < 0.001. After the Bonferroni correction: aaMCI with low-grade WMH vs. HC, P < 0.05; baMCI with high-grade WMH vs. HC, P < 0.05; caMCI with low-grade WMH vs. aMCI with high-grade WMH, P < 0.05.
DISCUSSION
Although statistical parameters have been frequently used in MRI studies, this method completely relies on developed software and is a labor-intensive technique in clinical practice. The MTA visual rating scale and the Fazekas rating scale have been shown to be quick and reproducible methods for assessing the degree of atrophy and subcortical ischemia in the clinical setting. To our knowledge, this study is the first to explore the contributions of executive function and brain structural changes to learning strategies in subjects with aMCI. Our findings showed that aMCI subjects had poorer performance than HCs in verbal memory and the semantic learning strategy. Furthermore, inefficient use of the semantic learning strategy was associated with MTA but not with WMH or executive dysfunction in aMCI subjects. These findings indicate that semantic learning strategy decline is more attributable to AD pathology than to subcortical ischemia in subjects with aMCI.
In addition to acquisition (immediate recall) and retrieval (delayed recall and recognition) deficits, we found that compared to HCs, aMCI subjects showed significantly diminished use of the semantic strategy during learning but a nonsignificant increase in the passive learning strategy of serial clustering. These results are consistent with previous findings that compared to age-matched healthy adults (Price et al., Reference Price, Kinsella, Ong, Mullaly, Phillips, Pangnadasa-Fox and Storey2010) or VCIND subjects (Sun et al., Reference Sun, Luo, Ren, Wei, Xing, Cheng and Zhang2016); aMCI subjects show decreased use of semantic clustering strategies. Semantic clustering is considered an efficient learning strategy that helps individuals “chunk” large amounts of information into smaller units, which allows for more efficient encoding, storage, and retrieval of information (Baddeley, Reference Baddeley2001; Delis et al., Reference Delis, Kramer, Kaplan and Ober2000). However, serial clustering is a passive, low-level approach to recall, which does not involve any new transformation or organization of the presented information (Glosser, Gallo, Clark, & Grossman, Reference Glosser, Gallo, Clark and Grossman2002). Taking advantage of the semantic structure of the material to learn, if this structure exists, is an automatic behavior in healthy subjects. It has been demonstrated that poor memory performance can be related to the diminished use of the semantic learning strategy in MCI subjects (Ribeiro et al., Reference Ribeiro, Guerreiro and De Mendonca2007). This hypothesis is further supported by our present findings that learning and recall positively correlated with the semantic clustering ratio and negatively correlated with the serial clustering ratio in subjects with aMCI. In addition, the semantic clustering ratio showed a relatively high sensitivity (83.3%) and a moderate specificity (62.2%) for discriminating between subjects with aMCI and HCs, with a cutoff value of 0.37. These findings indicate that the semantic clustering ratio may be a useful measure for screening patients with aMCI in clinical neuropsychological practice, but possible candidates should be further evaluated to exclude false-positives, as seen in the HCs.
Executive dysfunction, which was associated with verbal memory impairment, was also observed in aMCI patients according to the TMT scale in this study, although there was no difference in WCST scores between aMCI patients and HCs. The inconsistent findings on the WCST between the present study and our previous study (Sun et al., Reference Sun, Luo, Ren, Wei, Xing, Cheng and Zhang2016) may be ascribed to the heterogeneity of cognitive impairment and pathology in patients with aMCI (Dong et al., Reference Dong, Toledo, Honnorat, Doshi, Varol, Sotiras and Davatzikos2017). Although executive function plays a significant role in the encoding process and general function of memory, the relationship between semantic clustering and executive function remains controversial (Malek-Ahmadi et al., Reference Malek-Ahmadi, Raj and Small2011; McLaughlin et al., Reference McLaughlin, Wright, Larocca, Nguyen, Teng, Apostolova and Woo2014). A magnetic resonance spectroscopy study suggested that metabolic changes in the posterior cingulate gyrus had the strongest relationship with executive functioning in subjects with aMCI (Griffith et al., Reference Griffith, Hollander, Okonkwo, Evanochko, Harrell, Zamrini and Marson2007). However, the semantic clustering process is thought to be mediated by a system consisting of the prefrontal cortex and the medial temporal lobe (Becker & Lim, Reference Becker and Lim2003). In this study, there was no correlation between the semantic clustering ratio and executive function performance in aMCI subjects. Our present findings support the idea that the semantic learning strategy may share a separate brain network, which is independent of the system used for executive function.
It has been demonstrated that MTA, particularly hippocampal atrophy, is one of the earliest changes seen in the brains of patients with AD (Braak & Braak, Reference Braak and Braak1991) or even MCI (La et al., Reference La, Korf, Wm, Brashear, Fox, Barkhof and Scheltens2007). Memory impairment is related to structural and functional changes in medial temporal regions in individuals with aMCI (Apostolova et al., Reference Apostolova, Morra, Green, Hwang, Avedissian and Woo2010; Jack Jr et al., Reference Jack, Petersen, Xu, O’Brien, Smith, Ivnik and Kokmen1999). Consistent with a previous study (Overdorp, Kessels, Claassen, & Oosterman, Reference Overdorp, Kessels, Claassen and Oosterman2014), we found that aMCI subjects with clear MTA showed poorer performance than aMCI subjects with no MTA on learning and memory tasks. In addition, aMCI patients without MTA showed better performance in the use of the semantic strategy during word learning than patients with MTA and worse performance than HCs in this study. Moreover, the semantic clustering ratio could predict MTA severity in aMCI subjects, with a sensitivity of 65% and a specificity of 92.1%. These findings indicate that the deficit in the semantic strategy is associated with the severity of damage in the medial temporal lobe in patients with aMCI or AD spectrum. Our present findings are supported by the results of several functional neuroimaging studies, in which semantic encoding processes were found to be subserved by networks involving bilateral temporal lobe structures during learning (Baker et al., Reference Baker, Sanders, Maccotta and Buckner2001; Jackson & Schacter, Reference Jackson and Schacter2004). For instance, the semantic clustering process has been reported to be mediated by a system consisting of the medial temporal lobe and the prefrontal cortex (Becker & Lim, Reference Becker and Lim2003). Manning et al. demonstrated that spontaneously reactivated patterns of neural activity in the frontal and temporal cortices, as well as the hippocampus, predict the method of cluster recall according to the semantic similarity of the words being learned (Manning et al., Reference Manning, Sperling, Sharan, Rosenberg and Kahana2012). Furthermore, neural activity has been shown to overlap between the episodic and semantic memory systems during a word list-learning task (Weidemann et al., Reference Weidemann, Kragel, Lega, Worrell, Sperling, Sharan and Kahana2019). Within the medial temporal lobe, the perirhinal cortex, which provides item information and familiarity, works together with the entorhinal cortex in a complementary manner for both episodic and semantic memory (Naya, Reference Naya2016). Therefore, the structural and functional integrity of the medial temporal lobe might be very important in the process of semantic encoding during verbal learning.
WMH reflects small vessel disease (Decarli, Reference Decarli2004) and is attributed to vascular risk factors. It has been reported that WMH is observed in patients with AD, indicating concurrent vascular and AD pathology. WMH has been associated with poor memory performance in both MCI subjects and AD patients (Appel et al., Reference Appel, Potter, Bhatia, Shen, Zhao, Greig and Duara2009; La et al., Reference La, Korf, Wm, Brashear, Fox, Barkhof and Scheltens2007). However, we did not find any distinction in learning strategies among aMCI subjects with different grades of Fazekas scores, although WMH was associated with total learning and delayed recall in this study. No studies have directly investigated the relationship between WMH and learning strategies in subjects with aMCI. In our participants, WMH were mainly distributed in frontal-subcortical pathways, which strongly relate to retrieval but not to the encoding process during learning and memory (Luchsinger et al., Reference Luchsinger, Brickman, Reitz, Cho, Schupf, Manly and Decarli2009). Accordingly, the semantic encoding strategy seems to be unaffected by WMH in aMCI individuals. Additionally, our observation that aMCI patients with high-grade WMH had more severe impairments in executive function based on the ΔTMT index than those with low-grade WMH is supported by previous findings that executive function strongly correlated with the integrity of the subcortical white matter, especially in the frontal lobe (Delanowood et al., Reference Delanowood, Bondi, Sacco, Abeles, Jak, Libon and Bozoki2009).
Limitations
There are certain limitations of this study. First, although the Chinese version of the HVLT-R has been widely used and standardized in China, it still lacks validation in the elderly population (i.e., individuals over 60 years of age). Second, the relatively small sample size and the unbalanced sample sizes of the subgroups, e.g., the presence of fewer subjects in the subgroups with high MTA scores and high-grade WMH, was a recognized limitation that may have reduced the reliability of the statistical analysis and resulted in a lack of power for generalizing the findings to all individuals. Third, as the HCs did not receive MRIs, the correlation between learning and memory and underlying structural brain changes could not be determined under normal conditions. In addition, the MTA and WMH measurements were dichotomized in this study because aMCI subjects rarely have high severity grades of MTA and WMH. Therefore, whether increasing clustering strategy deficits are associated with increasing severity of MTA or WMH was not examined. Moreover, we used the visual rating scales to assess structural brain changes in aMCI participants. Although visual rating scales are easy to perform in clinical practice, the reliability of the MTA (interrater: 0.72–0.84, intrarater: 0.83–0.94, weighted Cohen’s κ) and Fazekas scales (interrater: 0.70–0.72, intrarater: 0.79–0.82) remains modest (Gao et al., Reference Gao, Swartz, Scheltens, Leibovitch, Kiss, Honjo and Black2011; Harper, Barkhof, Fox, & Schott, Reference Harper, Barkhof, Fox and Schott2015). The use of volumetric measurements of temporal lobe structures and WMH is warranted in our future studies. Furthermore, the Fazekas score is a relatively global measure for white matter hyperintensity, whereas the MTA scale is used to evaluate atrophy for only the medial temporal lobe. Reductions in global volume and brain atrophy in other areas, especially those involved in the learning strategy-related network mentioned above, were not analyzed in this study. Finally, the etiology and pathogenesis of aMCI are relatively heterogeneous. Biological markers, such as tests for amyloid β and tau in cerebrospinal fluid or with PET imaging, should be used to include patients with identified neuropathology.
CONCLUSION
MTA, but not WMH or executive dysfunction, contributes to the impaired use of the semantic strategy during the learning process in subjects with aMCI. Our results indicate that in clinical practice, the decline of the semantic learning strategy may reflect hippocampal neurodegeneration to some extent in patients with AD and aMCI.
ACKNOWLEDGMENTS
This work was supported in part by the National Natural Science Foundation of China (Grant no. 81870831); the Social Development Project of Tianjin Binhai New Area (Grant no. BHXQKJXM-SF-2018-02); and the National Key Clinical Specialty Construction Project of China.
CONFLICTS OF INTEREST
The authors have nothing to disclose.