Introduction
Chondrichthyan species are rare in modern freshwater ecosystems (Compagno et al., Reference Compagno, Dando and Fowler2005). However, ancient aquatic systems abounded in sharks and rays, especially those with linkages to marine habitats (e.g., Kirkland et al., Reference Kirkland, Eaton, Brinkman, Titus and Loewen2013). Throughout the latter half of the Cretaceous an intercontinental seaway flooded part of the North American continent, providing ideal conditions for the invasion of freshwater ecosystems by sharks and rays as well as the subsequent preservation of those ecosystems in the fossil record.
The latest Cretaceous Hell Creek Formation is a set of siltstone-/sandstone-dominated rocks named for a section exposed in the Hell Creek valley of Montana (Hartman et al., Reference Brinkman, Newbrey, Neuman, Wilson, Clemens, Horner and Hartman2014). In South Dakota, where fossils from the “SUE” locality are located, the age of the Hell Creek Formation lies between 67.4 Ma and 65.5 Ma, according to LeCain et al. (Reference LeCain, Clyde, Wilson, Riedel, Wilson, Clemens, Horner and Hartman2014). These sediments were deposited at a time when the intercontinental seaway was retreating from North America due in part to uplift of the Laramide orogeny (Lawton, Reference Lawton2008). This created a wide coastal plain where abundant aquatic communities containing crocodylians (Carpenter and Lindsey, Reference Carpenter and Lindsey1980), choristoderans (Matsumoto and Evans, Reference Matsumoto and Evans2010), turtles (Holroyd and Hutchison, Reference Holroyd, Hutchison, Hartman, Johnson and Nichols2002), amphibians (Demar, Reference DeMar2013), osteichthyan fish (Brinkman et al., Reference Brinkman, Newbrey, Neuman, Wilson, Clemens, Horner and Hartman2014), and chondrichthyans (Cook et al., Reference Cook, Newbrey, Brinkman, Kirkland, Wilson, Clemens, Horner and Hartman2014) flourished. Estes et al. (Reference Estes, Berberian and Meszoely1969) were the first to examine the Hell Creek Formation freshwater fauna comprehensively. Subsequent researchers have used similarly rich microvertebrate localities to identify new species from the aquatic ecosystems (e.g., Bryant, Reference Bryant1989; Demar, Reference DeMar2013; Cook et al., Reference Cook, Newbrey, Brinkman, Kirkland, Wilson, Clemens, Horner and Hartman2014) and to elucidate preservational biases among fossil sites (e.g., Wilson, Reference Wilson2008; Peterson et al., Reference Peterson, Scherer and Huffman2011).
Here we present the chondrichthyan fauna from the Field Museum of Natural History “SUE” locality (Fig. 1), a highly productive microsite locality that also contained the nearly complete Tyrannosaurus rex (Osborn, Reference Osborn1905) specimen “SUE” (FMNH PF 2081) and a diverse microvertebrate fauna that included small theropod dinosaurs (Gates et al., Reference Gates, Zanno and Makovicky2013), actinopterygian and sarcopterygian fish, lissamphibians, and reptiles (Lyson and Joyce, Reference Lyson and Joyce2009; Gates et al., Reference Gates, Makovicky and Rieppel2010b), all collected either during the excavation of the dinosaur specimen or subsequently during screen washing of the associated matrix in this study. The taxa identified in our fauna contain several observed in other localities as well as two new species. The results of this study illuminate the potential for new fossil taxon discoveries in the Hell Creek Formation and promote the utility of using microsite data from macrofossil assemblages.

Figure 1. Location of “SUE” locality near Faith, South Dakota, USA. Circle indicates the location of the “SUE” locality in the dark gray Hell Creek Formation. Medium gray shows outcroppings of the Fox Hills Sandstone and lightest gray shows Quaternary fill. Geologic map obtained from https://mrdata.usgs.gov/geology/state/map-us.html#home.
Materials and methods
Geologic setting
The “locality is situated in the lower portion of the Hell Creek Formation, ~4.8 m above the contact with the Fox Hills Formation (Larson, Reference Larson, Larson and Carpenter2008). It contains distinct layers of siltstone, fine-grained sandstone, and mudstone, with interbedded plant fossils. This association was possibly produced on a point bar deposit within a meandering river because no geologic evidence exists of a channel (e.g., rip-up clasts, coarser-grained sediment from a thalweg, or fall bocks from a cut-bank).
Matrix preparation
Approximately two tons of matrix ranging in grain size from sandy siltstone to mudstone were obtained from fossil jackets containing the bones of Tyrannosaurus rex, FMNH PF 2081. No additional sediment was sampled from the site. Precise locality information can be obtained from the Field Museum of Natural History. The site likely represents a point bar deposit within a meandering river channel, as evinced by alternating layers of mud-rich sediment containing leaf fossils and sandy siltstone.
Sediment was washed in nested screens of sieve openings 4.6 mm (mesh #4), 0.841 mm (mesh #20), and 0.595 mm (mesh #30) utilizing an overhead sprinkler system separated from the matrix by a 0.841 mm (mesh #20) screen in order to decrease damage to the fossils. The fossil concentrate was manually picked under a stereo-microscope. All orectolobiform teeth were found within the 0.595 mm size fraction, whereas the carcharhiniform tooth was found in the 0.841 mm mesh concentrate. The teeth were photographed on a Keyence VHX-5000 microscope. Scanning electron microscope imaging of FMNH PF 15982 was performed on a Carl Zeiss NTS EVO60 XVP scanning electron microscope in both high and variable pressure vacuum modes, using VPSE detectors without extraneous coating. All fossils are accessioned at the Field Museum of Natural History.
Phylogenetic analyses
In order to better determine the phylogenetic affinities of Galagadon n. gen. and other possible Cretaceous orectolobids, we identified a dozen traits related to dental anatomy (Table 1), following the pioneering work of Adnet and Cappetta (Reference Adnet and Cappetta2001) and Shimada (Reference Shimada2005) demonstrating that dental traits can provide phylogenetically useful information. We combined these twelve traits with a larger morphological analysis for orectolobiformes by Goto (Reference Goto2001) that includes 136 morphological traits arrayed across the body (Step II in his paper). Of those original traits, however, only two are related to teeth (characters 36 and 37), and of these, only character 37 involves the morphology of the teeth (apron breadth). The modified dataset consists of 148 morphological characters (ordered as in the original publication) and 28 ingroup taxa (Table 2), including several fossil forms.
Table 1. Phylogenetic characters created in this study. This list is amended to Goto (Reference Goto2001) and found in the complete NEXUS file included in the Supplementary Information. Numbering of characters follows that of the character matrix.

Table 2. List of the 28 ingroup taxa included in the phylogenetic analysis.

Coding for the ingroup extant species was performed using Herman et al. (Reference Herman, Hovestadt-Euler and Hovestadt1992) and Compagno et al. (Reference Compagno, Dando and Fowler2005). Goto (Reference Goto2001) used a composite outgroup, which was coded originally as an amalgamation of Lamniformes and Carcharhiniformes. Our coding of tooth characters was based on the lamniform Otodus obliquus (Agassiz, Reference Agassiz1843) and the carcharhiniform Carcharhinus leucas (Müller and Henle, Reference Müller and Henle1839), which were combined into a composite scoring for the outgroup taxon following Goto's (Reference Goto2001) protocol, resulting in numerous polymorphic characters.
Parsimony analysis
We conducted several parsimony-based phylogenetic analyses (one of which was backed by molecular data), including: 1) a Maximum Parsimony (MP) analysis on the full data with characters ordered following Goto (Reference Goto2001); 2) an MP analysis in which the three Cretaceous fossil taxa Cretorectolobus olsoni, Cederstroemia triangulata, and Galagadon nordquistae n. gen. n. sp. are constrained as a monophyletic cluster endemic to the Western Interior Seaway (WIS); and 3) an MP analysis in which extant orectolobid species were arranged according to a backbone constraint following analysis of molecular data by Corrigan and Beheregaray (Reference Corrigan and Beheregaray2009), but positions of fossils were left unconstrained. Importantly, this last analysis follows the ‘molecular scaffold’ approach advocated by Springer et al. (Reference Springer, Teeling, Madsen, Stanhope and de Jong2001) and later authors that creates a framework of extant taxa on which morphology-based fossil species move around without affecting molecular relationships. All analyses were conducted in the software PAUP ver. 4.05 build 157 (Swofford, Reference Swofford2003) using the Branch and Bound search algorithm with default settings.
Bayesian analysis
We also conducted phylogenetic analyses under the Bayesian Inference (hereafter BI) optimality criterion executed in MrBayes ver. 3.2.6 (Huelsenbeck and Ronquist, Reference Huelsenbeck and Ronquist2001; Ronquist and Huelsenbeck, Reference Ronquist and Huelsenbeck2003) using the Mk likelihood model of morphological evolution (Lewis, Reference Lewis2001). Six different partitions of the data set were tested with the partitions differing by the rates of character state parameter, either equal or variable rates with the latter sampled from a gamma-shaped distribution. The partitions were divided into anatomical sets characterized by either non-dental or dental-based characters within the data set. Two models utilized only one partition encompassing the entire data set (equal rates and variable rates model), whereas four additional models varied in the rates for the non-dental and dental partitions, respectively (equal/equal, equal/variable, variable/equal, variable/variable models). Otherwise, the default parameter settings in MrBayes 3.2.6 were utilized. Each model ran for 10 million generations with sampling occurring every 1,000 generations during the Markov-chain Monte Carlo (MCMC) with one hot and one cold chain exploring tree-space. Each model reached stationarity with low standard deviation of split frequencies early in their respective runs. The first 25% of the sampled trees were discarded to remove the initial climbing-phase of the analysis (i.e., “burn-in”). Models were then compared by their harmonic mean likelihoods via the Bayes Factor (Kass and Raftery, Reference Kass and Raftery1995).
Biogeographic analysis
Select results of our phylogenetic analyses were subjected to biogeographic analysis using the BioGeoBEARS package in R (Matzke, Reference Matzke2013), which allows multiple models and great flexibility in user-applied geographic constraints and time slicing protocols. Six biogeographic areas were defined for these analyses: Western Pacific, Indian Ocean, Eastern Pacific, and Atlantic for living taxa, and Tethys/Paratethys (combined as one area for the analyses) and the North American Western Interior Seaway (WIS) for fossil taxa. Distributions for the living species were based on FAO Marine Fishing Areas, as reported by the IUCN Red List (http://www.iucnredlist.org/) and on locality data reported in Fossilworks.org (Uhen et al., Reference Uhen, Alroy and Carrano2017) for fossils. The best fitting MCC BI tree and a randomly chosen MPT from the scaffold MP analysis were employed for biogeographic evaluation because the analysis requires a fully resolved bifurcated tree. Two different branch scaling methods were examined for each tree: the first applied an arbitrarily chosen minimum branch length (here 2 MYA) to every zero length branch (Laurin, Reference Laurin2004), the second used the method of dividing a root time increment over those branches that have no length due to them being the oldest member of a lineage (Brusatte et al., Reference Brusatte, Benton, Ruta and Lloyd2008). Tree scaling was conducted using the STRAP routine (Bell and Lloyd, Reference Bell and Lloyd2015) in the R environment. Living taxa were given ages either according to molecular estimates produced by Corrigan and Beheregaray (Reference Corrigan and Beheregaray2009), or the default value of 2MYA, whereas fossils were calibrated according to their data provided in Fossilworks.org (Uhen et al., Reference Uhen, Alroy and Carrano2017). The Minimum Branch Scaling method provided calibrated trees that were more congruent with the molecular divergence age estimates recovered by Corrigan and Beheregaray (Reference Corrigan and Beheregaray2009), whereas the second method pushed many orectolobid divergences between extant species into the Paleogene or even Cretaceous. We, therefore, will present results of our biogeographic analyses on the best fitting BI tree, equal/variable rates model, and MP scaffold tree scaled using the first method.
Each input tree was analyzed using three different base models: BAYAREALIKE (likelihood interpretation of BayArea; Landis et al., Reference Landis, Matzke, Moore and Huelsenbeck2013; Matzke, Reference Matzke2013), DEC (Ree and Smith, Reference Ree and Smith2008), and DIVALIKE (likelihood interpretation of DIVA; Ronquist, Reference Ronquist1997) and three alternative models that include a parameter governing founder-event speciation, j, for each of the base models (Matzke, Reference Matzke2013). Seven dispersal constraint matrices were constructed to reflect different continental configurations and oceanic connections over the last 255 Ma of Earth history. These matrices and the time slices for which each applies are provided in the Supplemental Data. Results from different model applications were compared using both the log-likelihood and AIC score of each respective model. Analyses of both trees recovered BAYAREALIKE + j as the best-fitting model, as measured by model log likelihood and AIC scores regardless of optimality criterion and branch scaling method. Alternatively, if the recently employed + j parameter is excluded, then the DEC model is the best fitting model for the MP topology and BAYAREALIKE model for the BI topology.
Repositories and institutional abbreviations
FMNH: Field Museum of Natural History, Chicago, IL, USA; ROM: Royal Ontario Museum, Toronto, ON, CAN; UCMP: University of California Museum of Paleontology, Berkeley, CA, USA.
Systematic paleontology
Class Chondrichthyes Huxley, Reference Huxley1880
Subclass Elasmobranchii Bonaparte, Reference Bonaparte1838
Superfamily Hybodontoidea Zangerl, Reference Zangerl and Schultze1981
Family Lonchidiidae Herman, Reference Herman1977
Genus Lonchidion Estes, Reference Estes1964
Type species
Lonchidion selachos, UCMP 53897, isolated tooth.
Lonchidion selachos Estes, Reference Estes1964
Figure 2.1–2.4
Type specimen
UCMP 52897, isolated tooth.
Occurrence
Field Museum “SUE” locality; Hell Creek Formation; Faith, South Dakota, USA; Upper Maastrichtian.
Description
The two Lonchidion specimens recovered from the “SUE” locality (Fig. 2.1–2.4) are identical to those described in other studies (Estes, Reference Estes1964; Estes et al., Reference Estes, Berberian and Meszoely1969; Bryant, Reference Bryant1989; Cook et al., Reference Cook, Newbrey, Brinkman, Kirkland, Wilson, Clemens, Horner and Hartman2014). Their occlusal surfaces are marked by a single moderately sharp ridge that runs the entire mesiodistal length of the central cusp. Other than the lingual protuberance, the central cusps are unornamented.
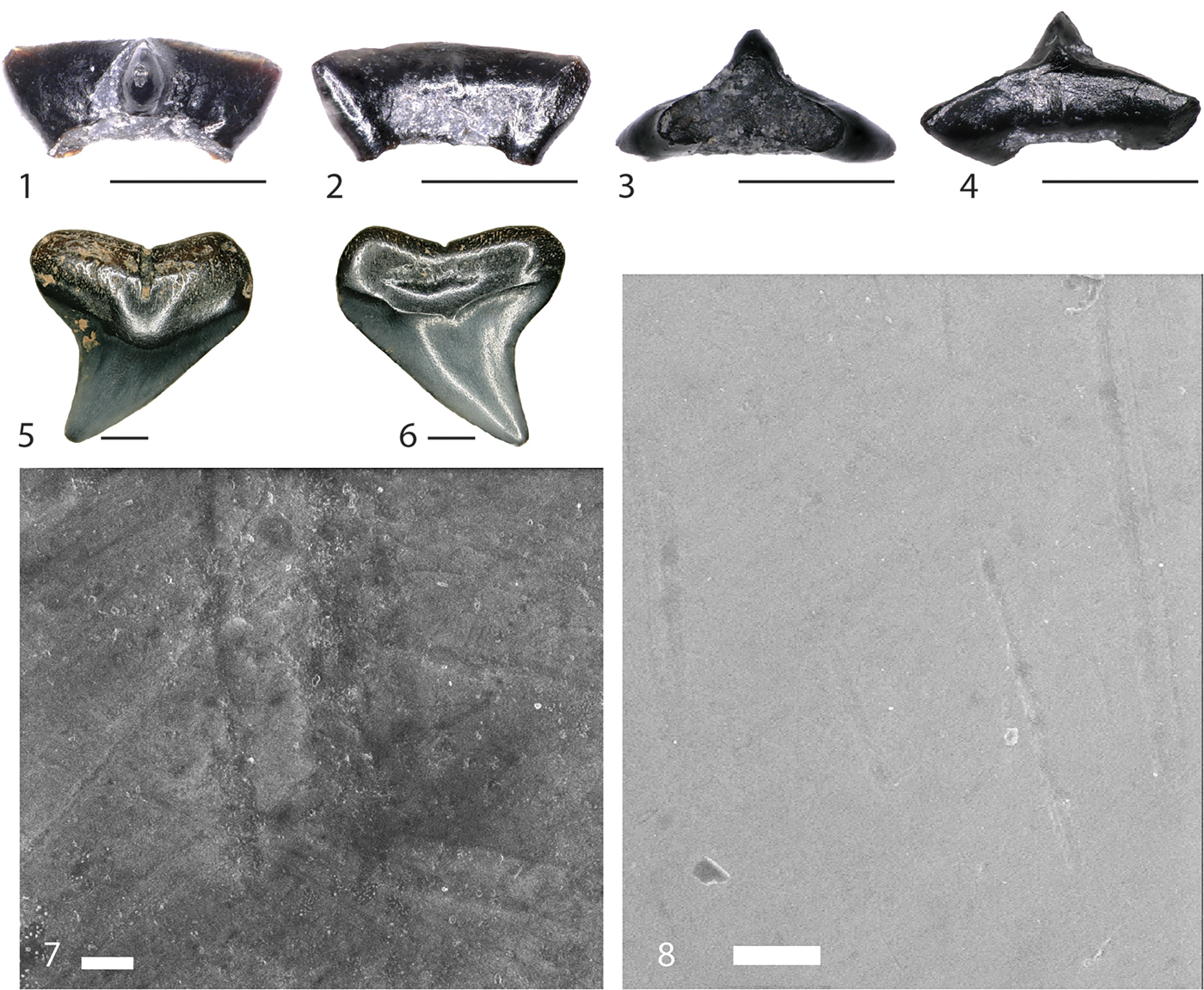
Figure 2. Lonchidion selachos (FMNH PF 15756) and Carcharhinidae indet. (FMNH PF 15982) teeth from the FMNH “SUE” locality. FMNH PF 15756 in (1) lingual, (2) labial, (3) basal, and (4) occlusal views. FMNH PF 15982 in (5) lingual and (6) labial views. Scanning electron microscopic image of Carcharhinidae indet. FMNH PF 15982 (7) and (8). Scale bar (1–4) equals 1 mm. Scale bar (5, 6) equals 2 mm. Scale bar in (7) equals 10 μm. Scale bar in (8) equals 100 μm.
Materials
FMNH PF 15756.
Remarks
When compared to other larger specimens of this species (e.g., Estes, Reference Estes1964; Estes et al., Reference Estes, Berberian and Meszoely1969; Bryant, Reference Bryant1989; Cook et al., Reference Cook, Newbrey, Brinkman, Kirkland, Wilson, Clemens, Horner and Hartman2014), the teeth recovered in this study are identical to other specimens. Therefore, it appears that the teeth of Lonchidion selachos do not undergo ontogenetic shape change.
Class Chondrichthyes Huxley, Reference Huxley1880
Subclass Elasmobranchii Bonaparte, Reference Bonaparte1838
Superorder Galeomorphii Compagno, Reference Compagno1973
Order Carcharhiniformes Compagno, Reference Compagno1973
Family Carcharhinidae Jordan and Evermann, Reference Jordan and Evermann1896
Genus gen. indet. sp. indet.
Figure 2.5–2.8
Occurrence
Field Museum “SUE” locality; Hell Creek Formation; Faith, South Dakota, USA; Upper Maastrichtian.
Description
Anatomical terminology follows Shimada (Reference Shimada2002). A single rounded tooth attributable to the Carcharhinidae (Fig. 2.5, 2.6; FMNH PF 15982) was recovered during this study. General morphology of this specimen suggests a member of the genus Carcharhinus, although this is uncertain. This tooth clearly suffered taphonomic alteration, but many important characteristics are still visible. The tooth is labio-lingually compressed with a central cusp that is broadly triangular with no obvious evidence of a serrated cutting edge, although the pre-burial rounding of the specimen may have eroded a small serrated cutting edge. There are no lateral cusplets or heels. Diagnostic traits of the crown are few, but the root possesses many traits that aid in assignment to the Carcharhinidae. A pronounced nutrient groove runs labio-lingually in the midline of the root, terminating just after reaching the lingual face. There appears to be a weak lingual protuberance, although not like the prominent protuberance easily identified on triakid or hemigaleid sharks. Root lobes are expanded with the basal margin flat and slightly widened, giving the tooth and overall plateaued appearance. Finally, the labial root face possesses a shallow ovoid impression presumably to house the weak lingual protuberance of the preceding tooth within the tooth family.
Materials
FMNH PF 15982, isolated tooth.
Remarks
Scanning electron microscopy of FMNH PF 15982 reveals no pitting on the crown enameloid as would be expected of a tooth that has undergone prolonged digestion. However, microabrasions are abundant across the enameloid surface (Fig. 2.7, 2.8) and large pits are present across the root, of which the latter maybe natural. Possibilities for the relocation from marine to terrestrial sediments include: (1) reworking into the terrestrial “SUE” Locality sequence from another stratigraphic interval (possibly the underlying marine Fox Hills Formation); or (2) loss of the tooth by a carcharhinid shark traveling in freshwater during deposition of the Hell Creek Formation.
Carcharhinid species have been recorded from sediments prior to the Paleocene (Cappetta, Reference Cappetta and Schultze2012); however, Underwood and Ward (Reference Underwood and Ward2008) described teeth they considered carcharhinid from Santonian rocks of Britain, which Guinot et al. (Reference Guinot, Underwood, Cappetta and Ward2013) challenged, instead placing the teeth in the Triakidae as opposed to the Carcharhinidae. Later, Guinot et al. (Reference Guinot, Cappetta and Adnet2014) named Archaeogaleus lengadocensis, a new carcharhinid taxon from the Valanginian of France, making that the earliest fossil occurrence of the species. Molecular phylogenies suggest that carcharhinid sharks evolved in the Cretaceous, or possibly the Jurassic, according to the calibrations used in Sorenson et al. (Reference Sorenson, Santini and Alfaro2014). Therefore, the discovery of teeth from this family in uppermost Cretaceous rocks should not come as a surprise. If the tooth should be found to represent the genus Carcharhinus, it means that FMNH PF 15982 pushes the oldest known occurrence of this genus into the upper Maastrichtian. At least two published instances document the discovery of marine shark teeth in Maastrichtian fluvial environments of western North America (Wroblewski, Reference Wroblewski2004; Wynd et al., Reference Wynd, Demar and Wilson2018).
Class Chondrichthyes Huxley, Reference Huxley1880
Subclass Elasmobranchii Bonaparte, Reference Bonaparte1838
Order Orectolobiformes Applegate, Reference Applegate1972
Family incertae sedis
Galagadon new genus
Type species
Galagadon nordquistae n. gen. n. sp. by monotypy.
Diagnosis
Same as for type species by monotypy.
Etymology
Galaga, named for the shape of the teeth, which when seen in different views resemble the spaceships in the arcade game “Galaga” developed and copyrighted by the company Namco; and don- Greek for “tooth.”
Remarks
Designation of this new genus is based on distinct traits seen across the entire series of teeth of the current hypodigm, which is consistent with variation found in other Orectolobiformes genera. All teeth come from a single locality, which is lower in section than other sites (e.g., Bug Creek Anthills). Other localities at this stratigraphic level may reveal more specimens of this genus.
Galagadon nordquistae new species
Figures 3–7
Holotype
FMNH PF 15769, an isolated tooth, possibly from an anterolateral position.
Diagnosis
Orectolobiform shark possessing the following autapomorphies: central cusp with distinctly swollen lingual face forming a clearly demarcated constriction, or neck, between the cusp and the root; labial surface of central cusp ornamented with a raised ridge or closely arrayed plications, which in most cases are distributed in such a pattern as to follow the slope of the heels; convex heels, well developed both mesial and distal to the central cusp with a distinct convex angle mid-distance along slope seen in anterior teeth and some lateral teeth. This taxon is further differentiated by the following suite of shared characters: anterior teeth possess distinct, high central cusp; heels on lateral teeth slightly serrated; heels of anterior teeth and some lateral teeth do not slope gradually toward root lobes, but are instead squared-off or rounded at their terminus; one or two rounded diminutive cusplets may be present although this trait varies among individual teeth; apron is generally broadly rounded and shows a bifid habit on some but not all specimens; root lobes enlarged on lingual side of tooth compared to more constricted structure on labial side, bestowing an exaggerated heart-shape in basal view; a central foramen pierces between the root lobes with the foramen divided by thin struts in some teeth.
Occurrence
Field Museum “SUE” locality; Hell Creek Formation; Faith, South Dakota, USA; Upper Maastrichtian.
Description
The tooth series was reconstructed based on a combination of tooth central cusp size and distal recurvature of the central cusp. Teeth with less distal recurvature are considered anterior teeth, slight distal recurvature typifies lateral teeth, whereas shorter central cusps represent posterior teeth.
The root of each Galagadon nordquistae n. gen. n. sp. tooth is heart-shaped and more similar to Eometlaouia spp. (Noubhani and Cappetta, Reference Noubhani and Cappetta2002), Restesia americana (Estes, Reference Estes1964) (Cook et al., Reference Cook, Newbrey, Brinkman, Kirkland, Wilson, Clemens, Horner and Hartman2014), and Chiloscyllium spp. (Cook et al., Reference Cook, Newbrey, Brinkman, Kirkland, Wilson, Clemens, Horner and Hartman2014) than to other species, yet remains distinct (Figs. 3, 4, 5.7, 5.8, 6.13–6.16). The lingual extensions of the root lobes are narrow, beginning sub-parallel in anterior teeth and widening into a broad “V”-shape in more posterior teeth (e.g., Figs. 3, 6.13–6.16; note that we define posterior teeth by their relatively shorter crowns compared to other teeth in the hypodigm). Similar to the fashion in which the lingual root lobes vary anteriorly to posteriorly, the labial root lobes expand into the upper curved portion of the heart-shape with different degrees of exaggeration. In this case, anterior teeth (Figs. 3, 4.13–4.16) tend to possess an abrupt contact between the mesial and distal halves of the root, which produces a mouse-eared look to the entire root lobe; however, the lateral files show a much more gradual progradation from narrow to expanded root lobes (Figs. 5.3, 5.4, 6.13–6.16). As such, the sharpness of the contact between the lingual and labial regions of the root appears less abrupt than in more anterior teeth. Even the roots of Restesia, a taxon also present in the Hell Creek Formation (Cook et al., Reference Cook, Newbrey, Brinkman, Kirkland, Wilson, Clemens, Horner and Hartman2014), are much more subdued than Galagadon n. gen., with smaller labial root expansions. A nutrient furrow (canal) is variably open throughout all specimens. Between the root lobes, a large central foramen is present and is sometimes divided in two by a narrow strut of dentine (e.g., Figs. 3.13, 3.14, 4.15, 5.13). A tooth designated as Chiloscyllium sp. (UCMP 191578) from Bug Creek shows a similar foramen, but specimens of Restesia americana have a more similar foramen morphology with needle-like struts to varying degrees (Cook et al., Reference Cook, Newbrey, Brinkman, Kirkland, Wilson, Clemens, Horner and Hartman2014). The central foramen is observable on only ~30% of the recovered teeth, due to infilling by sediment, so the prevalence of the bisected foramen condition across the tooth row is unclear. A condition of possessing one large nutrient foramen and two smaller foramina between the root lobes is seen on Brachaelurus waddi (Cappetta, Reference Cappetta1980), whereas Ornatoscyllium freemani has two subequal foramina oriented mesio-distally (Underwood and Ward, Reference Underwood and Ward2004). In virtually all teeth of this new species, the root is mesio-distally wider than the central cusp.

Figure 3. Anterior teeth of Galagadon nordquistae n. gen. n. sp. from the FMNH “SUE” locality. FMNH 15759 (1, 5, 9, 13, 17); FMNH 15761 (2, 6, 10, 14, 18); FMNH 15765 (3, 7, 11, 15, 19); FMNH PF 15766 (4, 8, 12, 16, 20). Specimens in lingual view (1–4), labial view (5–8), lateral view (9–12), basal view (13–16), and occlusal view (17–20). Scale bars equal 1 mm.
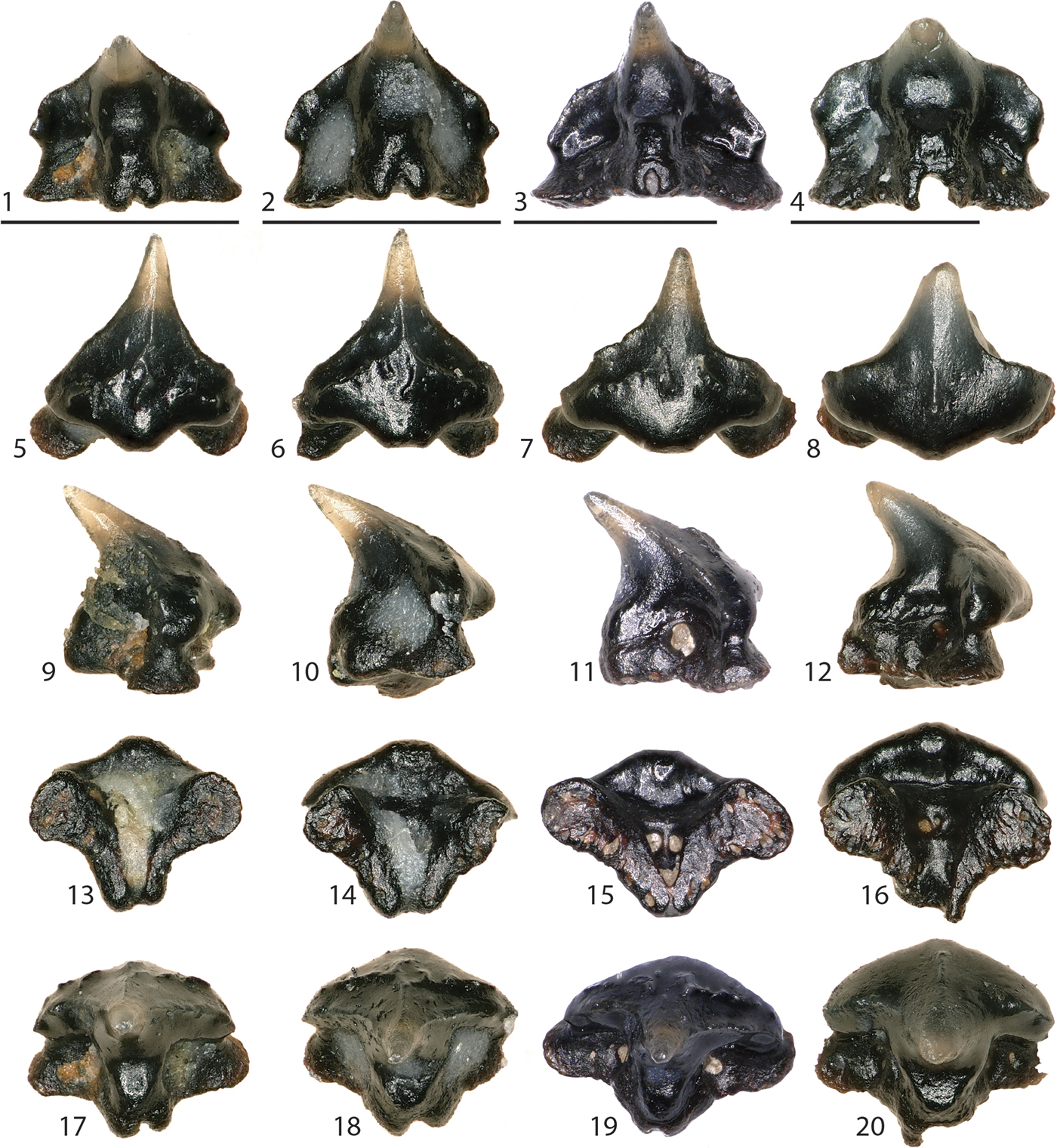
Figure 4. Anterior and possible anterolateral teeth of Galagadon nordquistae n. gen. n. sp. from the FMNH “SUE” locality. FMNH PF 15772 (1, 5, 9, 13, 17); FMNH PF 15773 (2, 6, 10, 14, 18); FMNH PF 15769 (3, 7, 11, 15, 19); FMNH PF 15770 (4, 8, 12, 16, 20). Specimens in lingual view (1–4), labial view (5–8), lateral view (9–12), basal view (13–16), and occlusal view (17–20). Scale bars equal 1 mm.
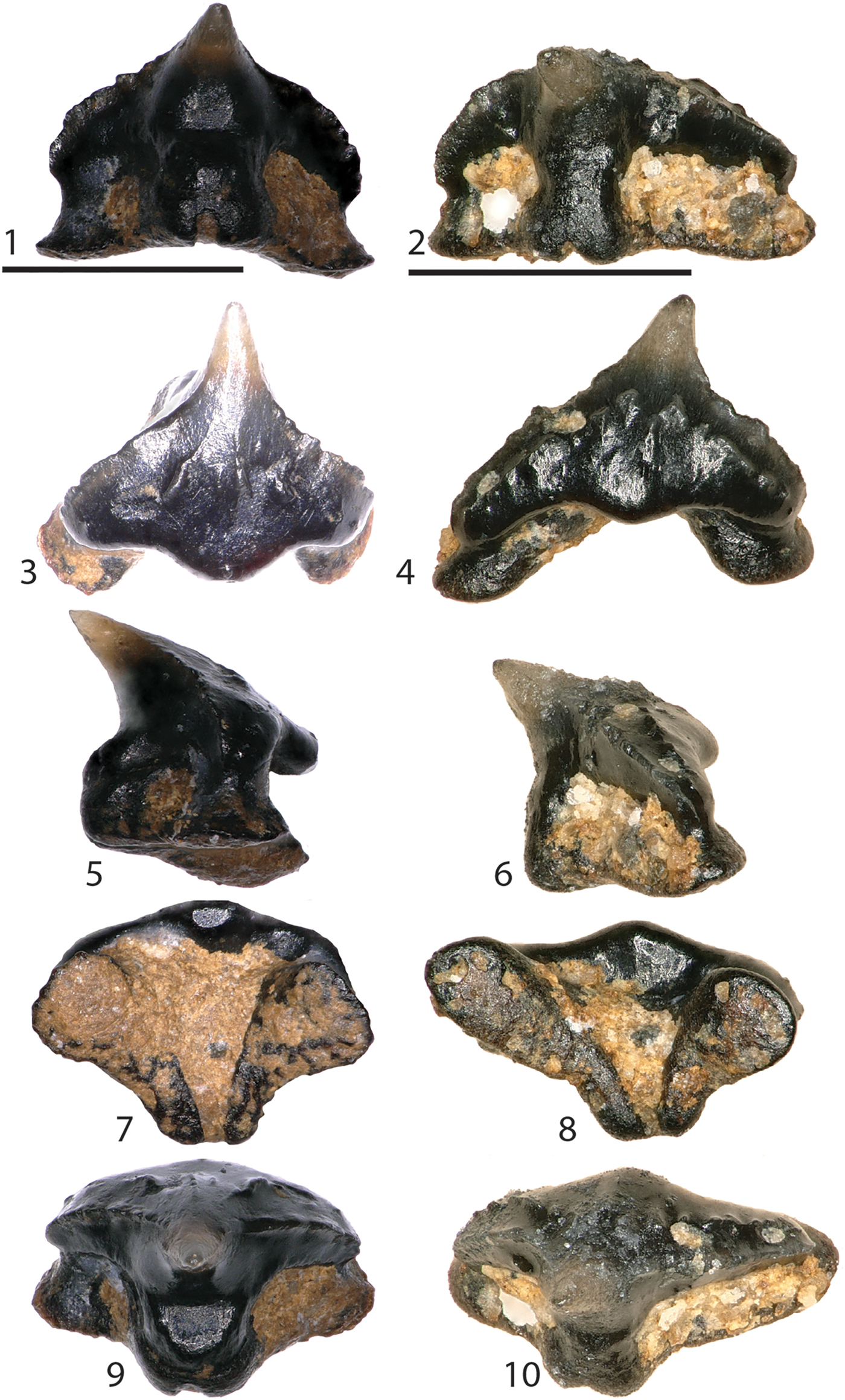
Figure 5. Anterolateral and lateral teeth of Galagadon nordquistae n. gen. n. sp. from the FMNH “SUE” locality. FMNH PF 15768 (1, 3, 5, 7, 9) and 15771 (2, 4, 6, 8, 10) in (1, 2) lingual view; (3, 4) labial view; (5, 6) lateral view; (7, 8) basal view; (9, 10) occlusal view. Scale bars equal 1 mm.
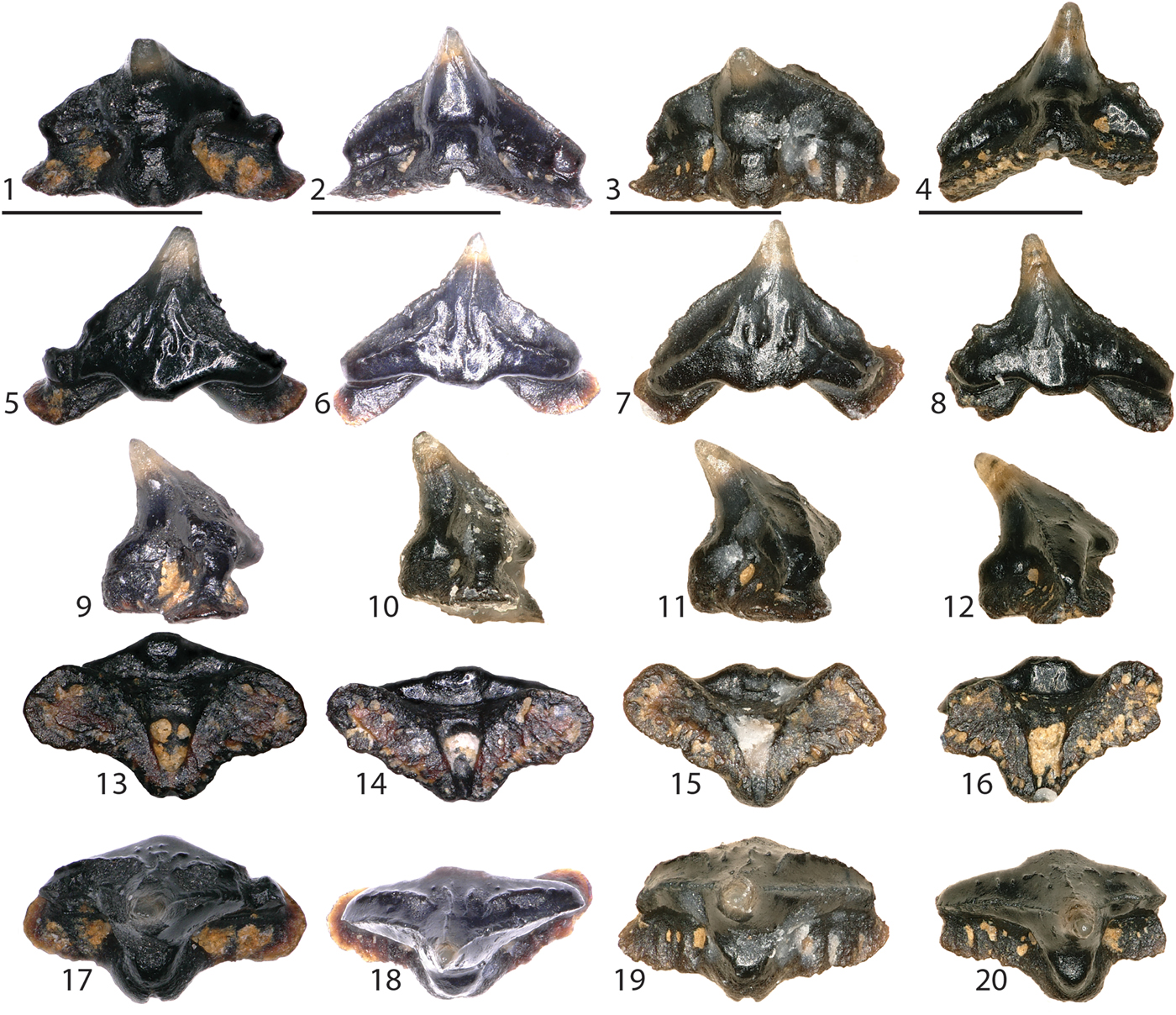
Figure 6. Lateral teeth of Galagadon nordquistae n. gen. n. sp. from the FMNH “SUE” locality. FMNH 15762 (1, 5, 9, 13, 17); FMNH 15763 (2, 6, 10, 14, 18); FMNH 15764 (3, 7, 11, 15, 19); FMNH PF 15983 (4, 8, 12, 16, 20). Specimens in lingual view (1–4), labial view (5–8), lateral view (9–12), basal view (13–16), and occlusal view (17–20). Scale bars equal 1 mm.
Lingually, Galagadon n. gen. teeth have a convex central cusp that tapers to a fine tip. Anterior teeth (Figs. 3, 4) have a taller central cusp than lateral teeth when compared to root width (Figs. 5, 6), and lateral tooth central cusps tend to recurve distally. The central cusp is swollen compared to the root, which produces a distinct constriction of neck between the tooth regions. Mesial and distal heels slope basally from the central cusp. In anterior teeth (Figs. 3, 4), the heels originate near the tip of the central cusp, whereas on lateral teeth the heels originate lower on the central cusp (Figs. 5, 6). The general shape of the heel changes posteriorly—the anterior teeth have mesio-distally narrower heels than lateral teeth, as a result of the narrower crown base compared to the laterals, and they show a distinct convex angle midway towards the tips (Figs. 3, 4, 5.1, 5.2, 6.1–6.4). Sharpness of this angle varies between specimens. Laterally, the heels largely lose the convex angle and become less steep. Some of the heels in the lateral series have slightly serrate apical surfaces. A small, diminutive cusplet is present on some teeth (Fig. 6.1). Development of this feature does not seem to follow a pattern through the tooth series. On both mesial and distal margins of the tooth, the central cusp-root intersection is positioned at the mid-point of a deep embayment formed by the heel and the root lobes. Such a feature is common in most orectolobiform teeth in which the root is as wide or wider than the crown. Depth of the constriction between the tooth central cusp and root similar to that of Galagadon n. gen. (well developed on the type specimen FMNH PF 15769) is visible on teeth of Cantioscyllium markaguntensis Kirkland, Eaton, and Brinkman, Reference Kirkland, Eaton, Brinkman, Titus and Loewen2013 (Kirkland et al., Reference Kirkland, Eaton, Brinkman, Titus and Loewen2013, fig. 9.9) and Palaeobrachaelurus spp. (Cappetta, Reference Cappetta and Schultze2012, fig. 154).
The lingual bulge is prominent. When a tooth is viewed laterally, the lingual bulge can be seen to extend lingually equal to the lingual extent of the central cusp. In other words, a vertical line drawn from the tip of the central cusp to the base would form a tangent to the lingual bulge. A single, large lateral foramen is present on each side of the lingual protuberance. There is much variation in the size of this lateral foramen among orectolobiform species (Cappetta, Reference Cappetta and Schultze2012). That seen on Galagadon n. gen. is one of the larger lateral foramina, similar to Eometlaouia spp., Hemiscyllium ocellatum, and many members of the Ginglymostomidae.
Another comparison between Galagadon n. gen. and other species is the apical angle between the crown and the lingual protuberance. Variation in this angle seems wide-ranging, even among supposedly closely related species. For instance, Galagadon n. gen. has an arch generally similar in aspect to some specimens of Chiloscyllium missouriense (Case, Reference Case1979) (Kirkland et al., Reference Kirkland, Eaton, Brinkman, Titus and Loewen2013, fig. 9.10CC), Restesia americana (Cook et al., Reference Cook, Newbrey, Brinkman, Kirkland, Wilson, Clemens, Horner and Hartman2014, fig. 3), or Eometlaouia numidica (Cappetta, Reference Cappetta and Schultze2012, fig. 145D). This is in contrast to the wider arch of Brachaelurus waddi or the narrower arch of the brachaelurid Eostegostoma spp. (Cappetta, Reference Cappetta and Schultze2012, fig. 153C, G). Given the variation seen in this trait, it seems unlikely that it will be useful for morphological phylogenetic analysis.
The labial face of Galagadon n. gen. teeth is more or less triangular. An absence of cusplets creates an uninterrupted edge from the apex to the lateral corners of the crown. The labial face of each Galagadon n. gen. central cusp is flat to slightly convex (Figs. 3–6). The plications and raised ridges of the labial face can also be seen. In some teeth, the labial face has a large section raised to form a slight plateau-like feature that can occupy nearly the entire central portion of the central cusp. Along the base of the labial face, the apron extends basally for only a short distance, but spreads widely laterally. There is a difference between the anterior and lateral teeth in that the apron is slightly more extended in anterior teeth (Figs. 3, 4, 5.3, 5.4, 6.5–6.8). The basal aspect of the apron ranges in shape from rounded to flat to faintly bifid and shows no particular pattern among tooth positions. The apico-basal shortness of the apron differs from the elongated aspect observed in brachaelurids, and is much more similar to species of ginglymostomids and some orectolobids (although there is much more variation in apron morphology among this clade). Across the labial face of the crown are numerous enameloid folds that form bumps, short ridges, and elongate ridges, all coalescing into a chevron with the terminal ends occupying the basal mesial and distal corners of the labial face, and the apex positioned along the midline at various places between the central cusp apex and base of the apron, depending on the individual tooth (Figs. 3, 4, 5.3, 5.4, 6.5–6.8). Some teeth, such as FMNH PF 15773 (Fig. 4.2) show a well-defined triangular ridge with an accessory ridge spanning from the apex of the triangular ridge to the apex of the central cusp. Most other teeth exhibit a lesser degree of enameloid folding than is seen on the aforementioned specimen. Nonetheless, all specimens have ornate labial central cusps and the plications form the same basic shape of an arc or triangle in the center of the crown (except FMNH PF 15770 whose labial face has a single apically oriented ridge).
A labial crown face adorned with bumps, ridges, and plications is a common morphological feature of species within the Ginglymostomidae and other orectolobiform species (Cappetta, Reference Cappetta and Schultze2012). Protoginglymostoma, as illustrated in Cappetta (Reference Cappetta and Schultze2012, fig. 163A) exhibits small folds that look similar to those observed on several specimens of Galagadon n. gen., although these folds are too few in number and in a different position, with exception of the Protoginglymostoma (Cantioscyllium) estesi specimen UALVP 53531 (Cook et al., Reference Cook, Newbrey, Brinkman, Kirkland, Wilson, Clemens, Horner and Hartman2014), which shows no ornamentation. Other ginglymostomid species that show ornamentation similar to Galagadon n. gen. include Plicatoscyllium, which possesses ridges in a general triangular pattern, and Cantioscyllium spp., which possesses apico-basally oriented ridges, especially on C. markaguntensis (Kirkland et al., Reference Kirkland, Eaton, Brinkman, Titus and Loewen2013). Outside of the Ginglymostomidae, Orectoloboides has irregularly apico-basally oriented ridges (Cappetta, Reference Cappetta1977). Guinot et al. (Reference Guinot, Cappetta and Adnet2014) illustrated a specimen of Ornatoscyllium that exhibits a raised ridge following the heels in a similar fashion to Galagadon n. gen., and specimens figured in Underwood and Ward (Reference Underwood and Ward2004) have less ornamentation. Also, Neuman and Brinkman (Reference Neuman, Brinkman, Currie and Koppelhus2005, fig. 9.1) figured an orectolobiform tooth (TMP 93.116.2) that has ample ornamentation.
Four teeth that may belong to Galagadon n. gen. (Fig. 7) are less certain with respect to their affinities. They could represent upper teeth of a heterodont dentition, ontogenetic or sex differences, or simply individual variation. The heel ridges on these teeth are more distinct—forming incipient cusplets—and the ornamentation is varied more than in the other teeth, albeit still similar in overall appearance. FMNH PF 15777 (Fig. 7.1, 7.5, 7.13, 7.17) has the most divergent labial ornamentation in that they are small bumps arrayed in an arc.

Figure 7. Teeth of unknown affinity of Galagadon nordquistae n. gen. n. sp. from the FMNH “SUE” locality. FMNH PF 15777 (1, 5, 9, 13, 17); FMNH PF 15778 (2, 6, 10, 14, 18); FMNH PF 15775 (3, 7, 11, 15, 19); FMNH PF 15774 (4, 8, 12, 16, 20). Specimens in lingual view (1–4), labial view (5–8), lateral view (9–12), basal view (13–16), and occlusal view (17–20). Scale bars equal 1 mm.
Etymology
The species name honors Ms. Karen Nordquist for her decades of support and volunteering at the FMNH, including as a matrix sorter, during which she discovered the teeth described here.
Materials
FMNH PF 15758, isolated tooth; FMNH PF 15759, isolated tooth; FLMNH PF 15760, isolated tooth; FMNH PF 15761, isolated tooth; FMNH PF 15762, isolated tooth; FMNH PF 15763, isolated tooth; FMNH PF 15764, isolated tooth; FMNH PF 15765, isolated tooth; FMNH PF 15766, isolated tooth; FMNH PF 15767, isolated tooth; FMNH PF 15768, isolated tooth; FMNH PF 15770, isolated tooth; FMNH PF 15771, isolated tooth; FMNH PF 15772, isolated tooth; FMNH PF 15773, isolated tooth; FMNH PF 15774, isolated tooth; FMNH PF 15775, isolated tooth; FMNH PF 15776, isolated tooth; FMNH PF 15777, isolated tooth; FMNH PF 15778, isolated tooth; FMNH PF 15780, isolated tooth; FMNH PF 15781, isolated tooth; FMNH PF 15783, isolated tooth; FMNH PF 15784, isolated tooth.
Remarks
The Galagadon n. gen. teeth from the “SUE” site are some of the lowest orectolobiform teeth yet discovered in the Hell Creek Formation. More discoveries of carpet sharks throughout the formation will yield information on the dynamics of the Cretaceous Western Interior Seaway and its influence of the preserved chondrichthyan fauna.
Class Chondrichthyes Huxley, Reference Huxley1880
Subclass Elasmobranchii Bonaparte, Reference Bonaparte1838
Order Rajiformes Berg, Reference Berg1940
Suborder Rhinobatoidei Fowler, Reference Fowler1941
Family Rhinobatidae incertae sedis sensu Cappetta, Reference Cappetta and Schultze2012
Genus Myledaphus Cope, Reference Cope1876
Materials
FMNH PF 15989, isolated denticle; FMNH PF 15990, isolated denticle.
Remarks
Included within the rhinobatoid remains from this site are two dermal denticles (FMNH PF 15989, FMNH PF 15990; Fig. 8.13–8.18). These dermal denticles are virtually identical to those described by Neuman and Brinkman (Reference Neuman, Brinkman, Currie and Koppelhus2005), but no comparative material exists that is definitely attributable to Myledaphus pustulosus in order to define dermal denticle morphology beyond Myledaphus sp. (sensu Cook et al., Reference Cook, Newbrey, Brinkman, Kirkland, Wilson, Clemens, Horner and Hartman2014).
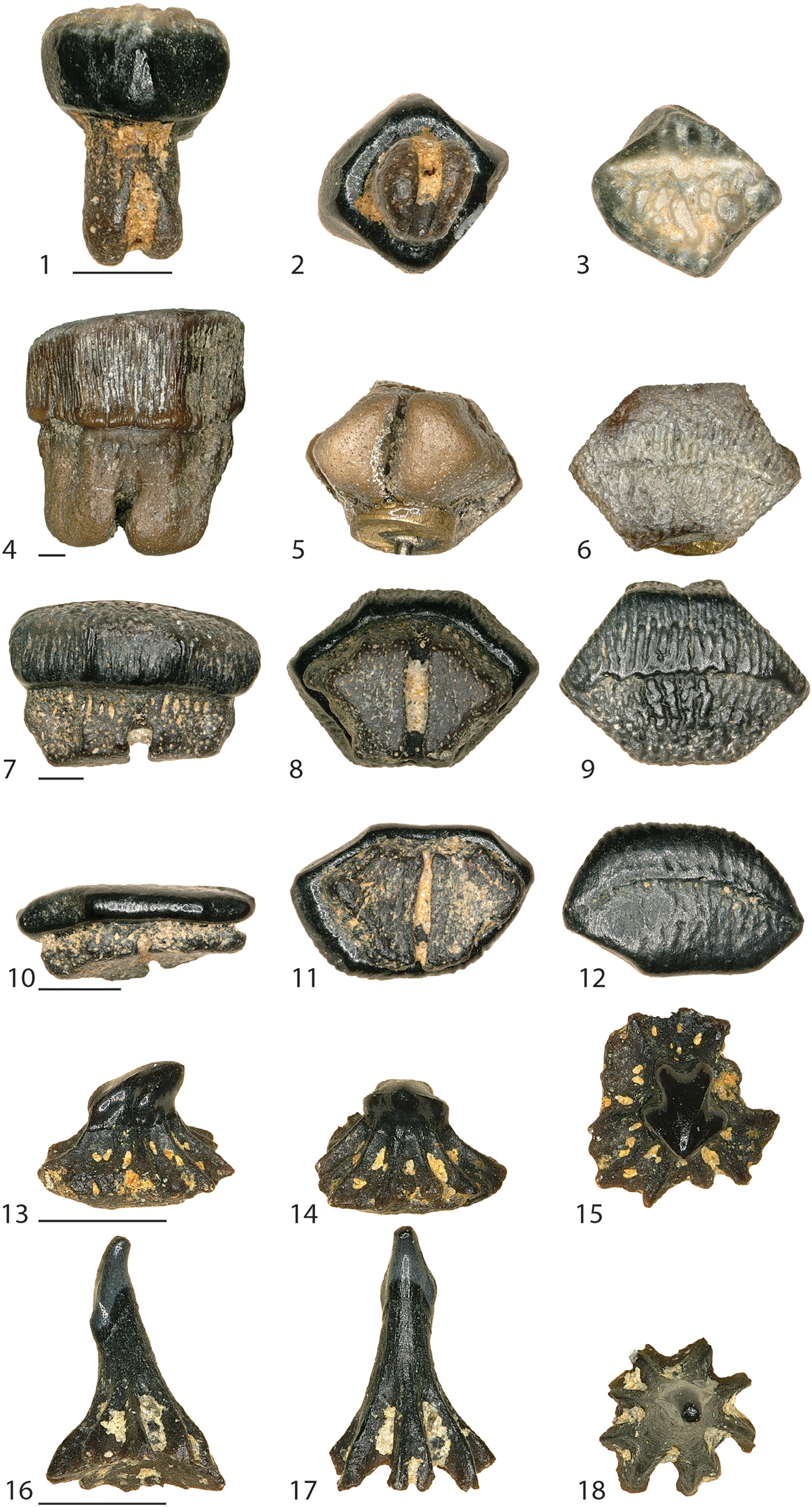
Figure 8. Examples of ray teeth and dermal denticles from the FMNH “SUE” locality. FMNH PF 15787 (1–3); FMNH PF 15313 (4–6); FMNH PF 15786 (7–9); FMNH PF 15788 (10–12) in lingual view (1, 4, 7, 10), basal view (2, 5, 8, 11), and occlusal view (3, 6, 9, 12). FMNH PF 15990 (13–15); and FMNH PF 15989 (16–18) in lateral view (13, 16), caudal view (14, 17), and apical view (15, 18). Scale bars equal 1 mm.
Myledaphus pustulosus Cook et al., Reference Cook, Newbrey, Brinkman, Kirkland, Wilson, Clemens, Horner and Hartman2014
Figure 8.1–8.12
Type specimen
UCMP 197869, isolated tooth.
Description
Ray teeth attributed to Myledaphus pustulosus dominate the selachian fauna at the “SUE” locality. This species is diagnosed on the basis of tubercles on the labial side of the tooth occlusal surface. Cook et al. (Reference Cook, Newbrey, Brinkman, Kirkland, Wilson, Clemens, Horner and Hartman2014) further described M. pustulosus as bearing transverse ridges that are discontinuous toward the lingual edge of the tooth. Figure 8 shows teeth from the “SUE” locality that possess each of the autapomorphic traits of M. pustulosus.
Materials
FMNH PF 15313, isolated tooth; FMNH PF 15786, isolated tooth; FMNH PF 15787, isolated tooth; FMNH PF 15788, isolated tooth; FMNH PF 17991, 44 isolated teeth.
Remarks
Myledaphus pustulosus is the taxon with the most numerous remains from this locality, to date.
Results
Phylogenetic analysis
Many extant shark families and subfamilies are thought to have arisen in the Mesozoic based on the fossil record (Frickhinger, Reference Frickhinger1999; Cappetta, Reference Cappetta and Schultze2012); however, there has been disagreement regarding the phylogenetic affinities of Mesozoic Orectolobidae. Cappetta (Reference Cappetta and Schultze2012) classified the Cretaceous taxa Cretorectolobus olsoni and Cederstroemia triangulata as orectolobids, but other authors (e.g., Siverson, Reference Siverson1995; Underwood and Mitchell, Reference Underwood, Mitchell and Unwin1999; Underwood et al., Reference Underwood, Mitchell and Veltcamp1999) have treated these as Orectolobiformes incertae sedis. The earliest record of orectolobids within their present day biogeographic distribution appears to be Miocene in age (Kemp, Reference Kemp, Vickers-Rich, Monaghan, Baird and Rich1991), and molecular analysis suggests that the extant taxa are members of a comparatively recent and rapid radiation, likely starting in the Miocene with many species originating as recently as the Pleistocene (Corrigan and Beheregaray, Reference Corrigan and Beheregaray2009).
Parsimony analysis
The first analysis resulted in 468 Most Parsimonious Trees (MPTs) with a length of 263, Consistency Index of 0.692, Rescaled Consistency Index of 0.617, and Retention Index of 0.891 (the strict consensus trees are shown in Figure 9.1). Galagadon n. gen., as well as the other WIS fossil taxa Cretorectolobus olsoni and Cederstroemia triangulata, are nested deep within the Orectolobidae, thus supporting the placement originally proposed by (Cappetta, Reference Cappetta and Schultze2012), despite prior publications placing these genera as Family incertae sedis (e.g., Siverson, Reference Siverson1995; Underwood and Mitchell, Reference Underwood, Mitchell and Unwin1999; Underwood et al., Reference Underwood, Mitchell and Veltcamp1999). Our results agree furthermore with those of Goto (Reference Goto2001) and Corrigan and Beheregaray (Reference Corrigan and Beheregaray2009) in finding that the genus Orectolobus is polyphyletic. Surprisingly, our analysis recovers the three WIS taxa as successive sister taxa to a more exclusive clade that includes some, but not all, living wobbegong species rather than forming an endemic clade, although this result is not robust and is not recovered in Bayesian analyses (see below). Another relevant result of our phylogenetic analysis is that the two fossil taxa attributed to Ginglymostomidae, Protoginglymostoma ypresiensis, and Ornatoscyllium freemani, are recovered within a clade with other nurse sharks, albeit in a polytomy (Fig. 9.1).

Figure 9. Phylogenetic trees positing position of Galagadon nordquistae n. gen. n. sp. among Orectolobiformes. (1) Majority Rules consensus tree for maximum parsimony analysis. (2) Bayesian Inference maximum clade credibility tree. For Figure 9.1, regular font numbers are frequencies of clades in the consensus tree, numbers in italics are the bootstrap values calculated in PAUP with the same settings described in body text for 100 replicates, and numbers in bold are the decay/Bremer values calculated in TNT 1.1 (Goloboff et al., Reference Goloboff, Farris and Nixon2008).
Our second analysis, which enforced monophyly of the three fossil species from the WIS, is only one step longer than the MPTs recovered from the first analysis. The constrained monophyletic clade still occupies the same position relative to living species where the three fossil species were recovered in unconstrained analyses; that is, sister to the remaining species of Orectolobus and Eocrossorhinus and the Orectolobus wardi and Sutorectus tentaculatus clade serving as the earliest diverging sister group to other orectolobids.
With a molecular scaffold constraint imposed on the data, resolution among orectolobid species is reduced in the strict consensus of the resulting 6318 MPTs. Only two nodes within Orectolobidae are recovered across all MPTs: (1) the family is recovered as monophyletic and sister to Brachaelurus; and (2) the late diverging extant species Orectolobus japonicus, O. maculatus, O. ornatus, Sutorectus tentaculatus, and Eocrossorhinus dasypogon form a clade to the exclusion of O. wardi and the three fossil orectolobids, which still fall within the crown group.
Bayesian analysis
In the favored single partition model (Table 3), all major clades are generally retained as reconstructed in MP analyses. Several differences, however, are found within the major clades because the hierarchical ordering of certain taxa differs from the MP analyses. For example, O. wardi is recovered as the sister taxon to G. nordquistae n. gen. n. sp. and the rest of the orectolobids in the MP topology, but in the BI topology, O. wardi is nested within orectolobids and as the sister taxon to S. tentaculatus with a posterior probability of 95%.
Table 3. Model scores for the various Bayesian phylogenetic inferences produced for this study. The merged cells represent a single data partition. Abbreviations: LnL, harmonic mean log likelihood values.

Based on comparisons of Bayes Factors across analyses, results of the analysis with separate partitions for non-dental and dental anatomical traits, with equal/variable rates model for the former partition, is preferred (Table 3). The maximum clade credibility (MCC) tree (Fig. 9.2) from that analysis places the fossil taxa Cederstroemia and Cretorectolobus as stem orectolobids, with all extant species forming a monophyletic clade, in contrast to the MP results. This is potentially more consistent with respect to the timing of the radiation of extant wobbegongs, which has been calibrated to be Miocene and younger based on analyses of mitochondrial DNA (Corrigan and Beheregaray, Reference Corrigan and Beheregaray2009), than the MP results that nest these Cretaceous fossils within the crown and thus dictate a Mesozoic age for the earliest divergences between extant lineages.
A major difference between the BI and MP analyses regards the placement of the new taxon, Galagadon nordquistae n. gen. n. sp. In all MP analyses, it groups unequivocally as a wobbegong close to the other two WIS orectolobids Cederstroemia and Cretorectolobus. In the BI results, however, it is consistently recovered as a stem hemiscyllid (bamboo shark) regardless of which combination of analytical parameters is employed. However, the posterior probability is relatively low for its current placement (~30%) and adjacent nodes suggest some ambiguity for this new taxon near the orectolobid (wobbegong) and hemiscyllid (bamboo) shark split (posterior probability of ~28% at the node uniting both clades). In order to assess whether this difference could be due to potential biases in the placement of taxa with large amounts of missing data in Bayesian Inference analyses (Goloboff and Pol, Reference Goloboff and Pol2005), we ran a series of tests in which fossil taxa were serially excluded, but no change in tree topology was noted. Taxon exclusion experiments conducted in MP suggest that the nested position of Galagadon n. gen. within Orectolobidae is dependent on inclusion of at least one of the other Cretaceous WIS species. When both Cederstroemia and Cretorectolobus are excluded, the strict consensus is unresolved to a large degree with neither Orectolobidae nor Hemiscyllidae recovered as monophyletic groups across all MPTs, indicating that Galagadon n. gen. can group with either of these shark families in the absence of the two other WIS fossil taxa. The discrepancy between BI and MP results is thus best explained as the result of different optimality criteria operating on a taxon characterized by homoplastic characters that can support at least two distinct phylogenetic positions.
Biogeographic Analysis.—Results of the biogeographic analysis (Table 4) of the BAYAREALIKE + j model on the BI time-scaled topology reconstruct the western Pacific as the ancestral area for both the crown plus stem Orectolobidae and crown plus stem Hemiscyllidae, with a subsequent single dispersal to the WIS for Galagadon n. gen. (as a hemiscyllid) and another for the common ancestor of Cretorectolobus and Cederstroemia (as orectolobids) during the Late Cretaceous (Fig. 10.1, 10.2). However, specific routes for these independent dispersal events into the WIS are unclear in the best-fitting analyses that rely on the j founder-event parameter to explain the distributions of the endemic WIS Cretaceous taxa, given that there is a lack of close relatives spanning the areas between the western Pacific Ocean and the WIS (see Discussion). Subsequent dispersals from the western Pacific to the Indian Ocean are reconstructed in the Neogene to explain the current distributions of carpet and bamboo sharks in tropical to temperate parts of the western Pacific and Indian Oceans.

Figure 10. Phylogenetic trees with predicted geographic distributions labeled at the nodes, edges, and tips. (1) Bayesian Inference tree with BAYAREALIKe + j model; (2) maximum parsimony tree with BAYAREALIKE + j model; (3) Bayesian Inference tree with BAYAREALIKE model; (4) maximum parsimony tree with DEC model. W, western Pacific Ocean; E, eastern Pacific Ocean; I, Indian Ocean; A, Atlantic Ocean; T, Para/Tethys Seaway; S, Western Interior Seaway.
Table 4. Model scores for the Maximum Parsimony and Bayesian Inference trees used in the biogeographic analysis. Abbreviations: AIC, Akaike Information Criterion; LnL, Log likelihoods.

Biogeographic reconstructions on the MP scaffold tree differ in reconstructing the WIS as the ancestral area to the orectolobid crown and Cretaceous stem taxa (Fig. 10.2). Two separate dispersal events to the western Pacific in the Late Cretaceous are invoked to account for the present-day distribution of O. wardi and the Sutorectus-Eucrossorhinus-Orectolobus (pars) clade. As in the results of the BI analysis, this could also indicate a more widespread ancestral area for the orectolobids given that the reconstructed ancestral areas include two disconnected areas as defined in the current analysis.
Both the BI and MP analyses using the BAYAREALIKE + j model find the founder-effect speciation (i.e., the ‘j’ parameter [Matzke, Reference Matzke2013]) serves as a significant and more frequent mechanism to explain the observed distributions than dispersal, extinction, or vicariance. Founder-effect speciation is often invoked in instances of island biogeography (e.g., Matzke, Reference Matzke2013), especially in regards to oceanic islands, for which there is scant evidence of historic range expansions or connections to other areas. As such, this model parameter may be ill suited to explain distributions of marine organisms distributed in oceans and epeiric seaways that were never isolated from each other, and which, although ephemeral in some instances, persisted for tens of millions of years or more.
In light of this concern, we also considered the best fitting alternative model that did not employ the j parameter to examine potential biogeographic histories for orectolobiform sharks: the DEC model (Ree and Smith, Reference Ree and Smith2008) for the MP scaffold tree and the BAYAREALIKE model for the BI tree. For the MP topology, ancestors to clades that encompass the Cretaceous WIS taxa as well as extant orectolobids, are reconstructed as being widespread and occurring in both the western Pacific and WIS, and including the (Para)Tethys for the node from which Eometlaouia branches (Fig. 10.3, 10.4). Furthermore, for the BI topology, the clades that include the WIS taxa and their respective extant carpet- and bamboo-shark relatives, the ancestral areas are also reconstructed to be more widespread and include the WIS and western Pacific, implying a wider geographic range than the current distribution suggests. These reconstructions then explain the present-day distributions of carpet and bamboo sharks as resulting from local extirpation of WIS taxa and secondary range expansion into the Indian Ocean as it achieved its current geography following the docking of India and extrusion of Indochina.
Discussion
Our study of the chondrichthyan fauna of the “SUE” quarry yielded both common Late Cretaceous taxa such as the batoid M. pustulosus and the hybodont shark Lonchiodon, but also an unexpected diversity of shark teeth. This includes a specimen that might mark a First Appearance Datum (FAD) for the carcharhiniform genus Carcharhinus, which had fossil records restricted to the Cenozoic (Maisey, Reference Maisey2012; Fig. 3). A recent molecular analysis of shark relationships posited the last common ancestor of hemigaleids and carcharhinids as sister to the triakid sharks (Veléz-Zuazo and Agnarsson, Reference Vélez-Zuazo and Agnarsson2011), which have a fossil record extending into the Late Cretaceous (see Becker et al., Reference Becker, Chamberlain and Wolf2006; Maisey, Reference Maisey2012; Sorenson et al., Reference Sorenson, Santini and Alfaro2014).
Recovery of the carcharhinid from the “SUE” Locality is surprising given the freshwater environment from which the fossils derive. This specimen shows clear evidence of reworking (rounded edges and a shiny patina), suggesting that it was not originally deposited at the same time as the Tyrannosaurus rex fossil. That said, there are three modern species of carcharhinid shark that either regularly invade euryhaline habitats or are restricted to freshwater ecosystems: Carcharhinus leucus (Bull shark) (Müller and Henle, Reference Müller and Henle1839) and two species of Glyphis (including the Ganges shark) (Compagno and Cook, Reference Compagno and Cook1995; Martin, Reference Martin2005). The combination of evidence for taphonomic reworking with the known occurrence of some modern relatives invading freshwater habitats makes it difficult to infer life habits of this shark.
The second novel discovery is Galagadon nordquistae n. gen. n. sp. As mentioned above, there are currently three species of orectolobiform chondrichthyan known from the Hell Creek Formation: Protoginglymostoma estesi (Herman, Reference Herman1977) (Cappetta, Reference Cappetta and Riegraf2006), Restesia americana (Cook et al., Reference Cook, Newbrey, Brinkman, Kirkland, Wilson, Clemens, Horner and Hartman2014), and Chiloscyllium sp. (Cook et al., Reference Cook, Newbrey, Brinkman, Kirkland, Wilson, Clemens, Horner and Hartman2014). Galagadon n. gen. is the only Hell Creek Formation orectolobiform currently known that possesses abundant folds and plications on the labial tooth surface. With the inclusion of Galagadon n. gen., a fourth species can now be included within the freshwater ecosystems. Increasing the species-richness of aquatic ecosystems is not surprising when one considers the complexity and heterogeneity of habitats available to orectolobiformes during the Late Cretaceous. For over 30 million years prior to the deposition of the Hell Creek Formation, the WIS inundated the central portion of North America, generating marine and estuarine ecosystems for orectolobiforms to occupy. Despite all extant orectolobiforms living strictly in marine ecosystems (Martin, Reference Martin2005), tolerance to varying salinity levels within this group is documented by occurrences of orectolobiform fossils discovered across salinity gradients from the marine into upland river systems (e.g., Kirkland et al., Reference Kirkland, Eaton, Brinkman, Titus and Loewen2013), and the “SUE” locality is considered a freshwater environment based on both its distance to the paleoshoreline and the occurrence of amphibian and baenid turtle remains. Terrestrial landscape heterogeneity due to topographic and climatic microcosms during the Late Cretaceous in Laramidia is posited to have caused an increase in vertebrate biodiversity (Gates et al., Reference Gates, Sampson, Zanno, Roberts, Eaton, Nydam, Hutchison, Smith, Loewen and Getty2010a, Reference Gates, Prieto-Márquez and Zanno2012), which is similar to biozonation identified by Nicholls and Russell (Reference Nicholls and Russell1990) for the seaway itself. As such, it seems reasonable that a number of small-bodied sharks could live together in such diverse habitats, or perhaps they are occurring in short, successive stratigraphic intervals. Many extant carpet-and bamboo shark species also exhibit overlapping or peripatric distributions (Corrigan and Beheregaray, Reference Corrigan and Beheregaray2009; Compagno et al., Reference Compagno, Dando and Fowler2005).
Past taxonomic practice often has been to assign fossil shark teeth to modern families based on morphological similarity, but the phylogenetic accuracy of such referrals has been questioned, especially for very old records including fossil Orectolobiformes (Glikman, Reference Glikman1967; Maisey, Reference Maisey2012). Here we have attempted to move beyond this concern by providing the first quantitative phylogenetic analysis that combines fossil and living Orectolobiformes. Although we have been unable to sample all Mesozoic fossils that have historically been assigned to Orectolobiformes, our analyses provide support for the phylogenetic placement of several contentious Mesozoic shark taxa within Orectolobiformes and its subclades. For example, both our Bayesian and MP analyses recovered Cretorectolobus and Cederstroemia as orectolobid relatives, which were originally designated by Siverson (Reference Siverson1995) as simply Family Orectolobiformes incertae sedis. We also found support for grouping fossil and extant ginglymostomatids (nurse sharks).
Concerns related to phylogenetic assignments based on similarity alone are highlighted by our case study of Galagadon n. gen. All our MP analyses, whether constrained or not, posited Galagodon as an orectolobid closely related to the other WIS taxa and most crown clade species, whereas our BI analyses consistently recovered it as a stem hemiscyllid (however, relatively low posterior probability at the nodes uniting and nested within carpet and bamboo sharks indicates its phylogenetic placement with less precision). Taxon exclusion experiments suggest that the differences in topological results are not a result of methodological bias, but instead reflect how different models handle taxa with conflicting character data. Such insights regarding possible phylogenetic placement of fossils can only be gained by applying a range of quantitative phylogenetic analyses.
The placement of fossil species relative to modern ones differed notably between BI topologies and MP ones. In the latter, Cretaceous fossil species were recovered inside the orectolobid crown group forcing a Cretaceous minimum date on its earliest divergences. The BI results, on the other hand, place all WIS fossil species as stem taxa to the extant diversity of carpet and bamboo sharks, thus allowing all divergences among extant species to be much younger, in better agreement with the molecular divergence estimates reported by Corrigan and Beheregaray (Reference Corrigan and Beheregaray2009). While the latter study was not calibrated against ingroup fossils, its estimates of very young (Plio-Pleistocene) divergences for most orectolobid species is consistent with the geological history and oceanography of the Indopacific region.
Our combined fossil and extant phylogenies allow for an examination of the biogeographic history of parts of the orectolobiform radiation. Results of the best-supported models suggest that a series of long distance dispersals or founder events account for most of the distribution of orectolobiformes over geological history, with only minor roles for dispersal through range expansion and vicariance. This result runs counter to most biogeographic theory in which the latter processes are thought to account for the bulk of distribution patterns among organisms (e.g., Ree and Smith, Reference Ree and Smith2008), with founder events playing a rare though important role, especially for island systems (Matzke, Reference Matzke2013). When considering other models that omit the stochastic founder event parameter, the best-fit models indicate that present-day orectolobid and hemiscyllid distributions are relicts of wider ancestral ranges with a secondary, but more limited radiation within the Indopacific region. This result is more consistent with traditional interpretations of orectolobiform history based on the fossil record, which comprises many named fossil species from shallow marine deposits in Europe, North Africa, the Middle East, and even Antarctica, as well as from the WIS (Compagno et al., Reference Compagno, Dando and Fowler2005; Maisey, Reference Maisey2012; Engelbrecht et al., Reference Engelbrecht, Mörs, Reguero and Kriwet2017). We hypothesize that our initial results are biased by the patchy nature of the orectolobiform record (Maisey, Reference Maisey2012), which is further exacerbated by our sampling of only those fossil species for which descriptions allow for character coding. The oldest fossils are Tethyan, whereas all Cretaceous fossils come exclusively from the WIS. Because these seaways disappear in the Mesozoic and thus can neither share extant taxa nor be invoked in the dispersal of extant crown clades, the analysis forces founder events to explain past and present day distributions. This problem may be remedied by better sampling of the fossil record and we hope that future work will expand our preliminary phylogenetic analysis to include more critical fossils and in turn allow for a more comprehensive analysis and understanding of shark biogeography.
Conclusions
Orectolobiform sharks held a rich ancient biodiversity within both strictly marine ecosystems through freshwater riverine habitats. The freshwater “SUE” locality from the latest Cretaceous Hell Creek Formation of South Dakota preserves a new orectolobiform shark, Galagadon nordquistae n. gen. n. sp., diagnosed based on a series of unique and shared traits, such as a constriction between the central cusp and root and a convex angle on the heels. In addition to Galagadon n. gen., other chondrichthyes species found at the site include Lonchidion selachos, Myledaphus pustulosus, and an unidentified tooth from the family Carcharhinidae. The latter species marks a new occurrence for the respective family, firmly pushing the fossil record for their origination into the Maastrichtian. Taken together, these finds add to an already considerable diversity of WIS chondrichthyans and corroborate that Mesozoic epeiric seaways played an important role in the diversification of modern sharks and rays, as suggested by Maisey (Reference Maisey2012).
We provide the first analytically supported phylogenetic positions for several Mesozoic orectolobiform taxa by incorporating both modern and ancient taxa through combined evidence data matrices, allowing for preliminary exploration of the evolution and biogeography of the clade. This study adds to others (e.g., Adent and Capetta, Reference Adnet and Cappetta2001; Peart et al., Reference Peart, Gates and Campione2015) that combine extant and fossil species to explore the history of shark evolution.
Accessibility of supplemental data
Data available from the Dryad Digital Repository: https://doi.org/10.5061/dryad.m276f51
Acknowledgments
We thank the Field Museum's Section of Earth Sciences for supporting this study from funds dedicated to research on SUE. EG's work was supported by a gift from the Kenneth A. Griffin Foundation. Sediment washing was made possible by B. Simpson of the Field Museum who saved the matrix from SUE jackets, and who also cataloged the materials described here. N. Bourne inspired the orectolobiform name. K. Seymour (Royal Ontario Museum), L. French and R. Robbins (Florida Museum of Natural History), and G. Hogue (NC Museum of Natural Sciences) allowed access to collections. N. Bourne, B. Argall, and C. Clark helped photograph shark specimens. T. Cook and D. Ehret provided very helpful reviews that improved the manuscript, and H.-D. Sues provided efficient editorial assistance. Funding for TAG was provided by R. Dunn and L. Zanno through NSF MSP #1319293.