Introduction
The modern African dwarf crocodile, Osteolaemus Cope, Reference Cope1861, is a group of at least three crocodylid species (Eaton et al., Reference Eaton, Martin, Thorbjarnarson and Amato2009; Smolensky, Reference Smolensky2015; Smolensky et al., Reference Smolensky, Hurtado and Fitzgerald2015). They have comparatively short, deep snouts and extensive dermal armor. These are some of the smallest extant crocodylians, rarely exceeding 2 m in total length (Eaton, Reference Eaton, Manolis and Stevenson2010), and inhabit streams and pools in rainforests of western and central Africa (e.g., Waitkuwait, Reference Waitkuwait1986; Kofron, Reference Kofron1992; Riley and Huchzermeyer, Reference Riley and Huchzermeyer1999; Eaton, Reference Eaton, Manolis and Stevenson2010; Shirley et al., Reference Shirley, Carr, Nestler, Vliet and Brochu2018).
Extinct relatives of Osteolaemus from the late Cenozoic of Africa and Madagascar represent a much wider range of body size, skull morphology, and (presumably) ecological preference. Some were gharial-like forms with tubular snouts, and others superficially resembled modern generalized crocodylians (sensu Brochu, Reference Brochu2001) such as Crocodylus Laurenti, Reference Laurenti1768, bearing moderately long, broad snouts (Brochu, Reference Brochu2000a, Reference Brochu2007; Storrs, Reference Storrs, Leakey and Harris2003; Llinás Agrasar, Reference Llinás Agrasar2004; Brochu and Storrs, Reference Brochu and Storrs2012; Conrad et al., Reference Conrad, Jenkins, Lehmann, Manthi, Peppe, Nightingale, Cossette, Dunsworth, Harcourt-Smith and Mcnulty2013). Indeed, most such generalized forms were previously referred to Crocodylus (e.g., Fourtau, Reference Fourtau1918; Tchernov and Van Couvering, Reference Tchernov and Couvering1978; Tchernov, Reference Tchernov1986; Pickford, Reference Pickford2003).
Early osteolaemines also had a broader distribution within continental Africa. One species of Osteolaemus formerly occurred as far east as Uganda, but the present range of the genus does not extend into the East African Rift Valley System (EARS; Eaton, Reference Eaton, Manolis and Stevenson2010). The African sharp-nosed crocodile, Mecistops Gray, Reference Gray1844, is also an osteolaemine in some analyses (e.g., Gatesy et al., Reference Gatesy, Amato, Norell, Desalle and Hayashi2003; McAliley et al., Reference McAliley, Willis, Ray, White, Brochu and Densmore2006; Meredith et al., Reference Meredith, Hekkala, Amato and Gatesy2011; Oaks, Reference Oaks2011), and it is likewise a Central and West African lineage not currently found east of Lake Tanganyika or south of the Congo Basin (Shirley et al., Reference Shirley, Carr, Nestler, Vliet and Brochu2018). But osteolaemines are known from the early Miocene of Egypt, Libya, Namibia, and Kenya (Fourtau, Reference Fourtau1918; Tchernov and Van Couvering, Reference Tchernov and Couvering1978; Brochu, Reference Brochu2000a; Pickford, Reference Pickford2003; Llinás Agrasar, Reference Llinás Agrasar2004; Brochu and Storrs, Reference Brochu and Storrs2012; Conrad et al., Reference Conrad, Jenkins, Lehmann, Manthi, Peppe, Nightingale, Cossette, Dunsworth, Harcourt-Smith and Mcnulty2013). Indeed, the only generalized crocodiles known from Africa during the early Miocene are putative osteolaemines.
In the EARS, the generalized osteolaemine Brochuchus pigotti (Tchernov and Van Couvering, Reference Tchernov and Couvering1978) and its tube-snouted close relative, Euthecodon Fourtau, Reference Fourtau and Fourtau1920, have been reported from the ca. 18 Ma Hiwegi Formation on Rusinga Island and correlative units in the Lake Victoria region of Kenya (Tchernov and Van Couvering, Reference Tchernov and Couvering1978; Buffetaut, Reference Buffetaut1979; Tchernov, Reference Tchernov1986; Conrad et al., Reference Conrad, Jenkins, Lehmann, Manthi, Peppe, Nightingale, Cossette, Dunsworth, Harcourt-Smith and Mcnulty2013). By ca. 7 Ma, in the Lower Nawata Formation at Lothagam, near Lake Turkana (Storrs, Reference Storrs, Leakey and Harris2003), the only remaining osteolaemine lineages are Euthecodon and, assuming it is an osteolaemine, Mecistops, both of which persist in the eastern (Gregory) branch of the EARS into the Pleistocene (Joleaud, Reference Joleaud1930; Arambourg, Reference Arambourg and Arambourg1947; Tchernov, Reference Tchernov1986; Storrs, Reference Storrs, Leakey and Harris2003; Brochu, Reference Brochu2020). Although not to the same degree as Euthecodon, Mecistops also has a comparatively slender snout (e.g., Busbey, Reference Busbey and Thomason1994; Pierce et al., Reference Pierce, Angielczyk and Rayfield2008; Sadleir and Makovicky, Reference Sadleir and Makovicky2008; Piras et al., Reference Piras, Colangelo, Adams, Buscalioni, Cubo, Kotsakis, Meloro and Raia2010; Wilberg, Reference Wilberg2017; Groh et al., Reference Groh, Upchurch, Barrett and Day2020). The generalized crocodylids in these units are species of Crocodylus (Brochu and Storrs, Reference Brochu and Storrs2012; Brochu, Reference Brochu2020).
It is unclear why generalized osteolaemines such as B. pigotti appear to have been replaced by Crocodylus, largely because of the 11 million years separating early and late Miocene crocodylian assemblages in the region. Whether these changes occurred suddenly or gradually, and whether they can be correlated with specific tectonic events or environmental changes, depends on knowing when generalized osteolaemines disappeared. This, in turn, is reliant on the description of Miocene crocodylids that are intermediate in age between Rusinga and Lothagam.
Here, we report occurrences of Brochuchus from several sites throughout the early and middle Miocene of Kenya. Some are from the Lake Victoria region in southwestern Kenya, and others are from the Turkana Basin in the northern part of the country (Fig. 1). Specimens from the middle Miocene at Maboko form the basis of a new species, Brochuchus parvidens. These occurrences extend the known stratigraphic range of generalized osteolaemines in East Africa and bear on the timing of crocodylian faunal turnover in the late Cenozoic of the region.

Figure 1. Map showing placements of locations discussed in this manuscript. A: Loncherangan. B: Kalodirr. C: Moruorot. D: Maboko. E: Rusinga Island.
Materials and methods
Phylogenetic analysis
The new species described here was added to a matrix of 189 discrete morphological characters and 31 ingroup taxa. The characters and codings used here are the same as those of Brochu and Storrs (Reference Brochu and Storrs2012) with modifications (Supplemental Data). First, we added Pleistocene Crocodylus ossifragus Dubois, Reference Dubois1908 from Java based on first-hand observation of type and referred specimens. Although it has generally been regarded as a junior synonym of the extant Siamese crocodile (C. siamensis Schneider, Reference Schneider1801; Müller, Reference Müller1923; Brochu, Reference Brochu2000a; Delfino and de Vos, Reference Delfino and De Vos2010), there are subtle but consistent differences between them (Brochu and Sumrall, Reference Brochu and Sumrall2020). We thus treat C. ossifragus as a separate species here.
Codings for B. pigotti were slightly modified from those used in Brochu and Storrs (Reference Brochu and Storrs2012) to reflect a revised interpretation of sutures surrounding the external naris. Previously, B. pigotti was assigned state 0 for character 82, indicating that the nasals contact the naris, but do not bisect it. We no longer hold this interpretation and assign state 2—the nasals do not reach the naris, but the nasals and premaxillae remain in contact—to both species of Brochuchus. This is similar to the condition in some other generalized crocodylids, such as Rimasuchus lloydi (Fourtau, Reference Fourtau1918) and “Crocodylus” gariepensis Pickford, Reference Pickford2003.
As used in this analysis, Crocodylus niloticus Laurenti, Reference Laurenti1768; Crocodylus novaeguineae Schmidt, Reference Schmidt1928; and Mecistops cataphractus (Cuvier, Reference Cuvier1824) can be viewed as representing two species each. All three have been split (Hekkala et al., Reference Hekkala, Shirley, Amato, Austin, Charter, Thorbjarnarson, Vliet, Houck, Desalle and Blum2011; Meredith et al., Reference Meredith, Hekkala, Amato and Gatesy2011; Shirley et al., Reference Shirley, Carr, Nestler, Vliet and Brochu2018; Murray et al., Reference Murray, Russo, Zorrilla and McMahan2019), with Crocodylus suchus Geoffroy, Reference Geoffroy Saint-Hilaire1807 separated from C. niloticus; Crocodylus halli Murray et al., Reference Murray, Russo, Zorrilla and McMahan2019 separated from C. novaeguineae; and Mecistops leptorhynchus (Bennett, Reference Bennett1835) separated from M. cataphractus. The new or resurrected species are morphologically redundant with their pre-split conspecifics for the characters used here; hence, including them as separate terminals would add nothing to the analysis.
The ingroup sample focused on crocodylids and close crocodyloid relatives. One thousand random addition replicate analyses were performed using PAUP 4.0a (build 165; Swofford, Reference Swofford2002). Branches were collapsed if their minimum length was zero. Borealosuchus sternbergii (Gilmore, Reference Gilmore1910) and Leidyosuchus canadensis Lambe, Reference Lambe1907 were used as outgroups to root the trees.
Nomenclatural notes
In this manuscript, we apply clade name definitions from a molecular context (Brochu, Reference Brochu2003). Crocodylidae Cuvier, Reference Cuvier1807 corresponds with the last common ancestor of Crocodylus niloticus and Osteolaemus tetraspis Cope, Reference Cope1861 and all of its descendants, and Crocodylinae Cuvier, Reference Cuvier1807 refers to C. niloticus and all crocodylians more closely related to it than to O. tetraspis. The definition of Osteolaeminae Brochu, Reference Brochu2003—O. tetraspis and all crocodylians more closely related to it than to C. niloticus—is context independent.
We refer to the two living species of Crocodylus in Africa—C. niloticus and C. suchus—as Neoafrican Crocodylus. This is to distinguish the modern forms from an extinct clade including C. anthropophagus Brochu et al., Reference Brochu, Njau, Blumenschine and Densmore2010 and C. thorbjarnarsoni Brochu and Storrs, Reference Brochu and Storrs2012, which is here termed Paleoafrican Crocodylus. Neoafrican Crocodylus may not be monophyletic; some analyses draw C. niloticus closer to a clade of crocodiles from the Western Hemisphere—Neotropical Crocodylus—than to C. suchus (Hekkala et al., Reference Hekkala, Shirley, Amato, Austin, Charter, Thorbjarnarson, Vliet, Houck, Desalle and Blum2011; Meredith et al., Reference Meredith, Hekkala, Amato and Gatesy2011; Oaks, Reference Oaks2011).
Conrad et al. (Reference Conrad, Jenkins, Lehmann, Manthi, Peppe, Nightingale, Cossette, Dunsworth, Harcourt-Smith and Mcnulty2013) informally referred to a group including Osteolaemus and Voay Brochu, Reference Brochu2007 as “osteolaemins.” Here, we define Osteolaemini on the basis of Osteolaemus tetraspis and all taxa more closely related to it than to Mecistops cataphractus, Crocodylus niloticus, or Euthecodon nitriae Fourtau, Reference Fourtau and Fourtau1920. We define Euthecodonini as Euthecodon nitriae and all taxa more closely related to it than to Osteolaemus tetraspis, Mecistops cataphractus, or Crocodylus niloticus. In some published trees (e.g., those of Conrad et al., Reference Conrad, Jenkins, Lehmann, Manthi, Peppe, Nightingale, Cossette, Dunsworth, Harcourt-Smith and Mcnulty2013), Osteolaemini is redundant with Osteolaeminae.
Because we have not studied it first-hand, the ingroup sample in our analysis does not include the type species of Euthecodon, E. nitriae from the Pliocene of Egypt. One could thus argue that our analysis does not actually explore the membership of Euthecodontini, the definition of which is anchored on the type species. However, E. nitriae and one of the species included here, Plio-Pleistocene Euthecodon brumpti (Joleaud, Reference Joleaud1920) from Kenya and Ethiopia, are extremely similar (Ginsburg and Buffetaut, Reference Ginsburg and Buffetaut1978; Tchernov, Reference Tchernov1986). Euthecodon brumpti can reasonably be used as a stand-in for E. nitriae when discerning putative euthecodontins.
Dental abbreviations
Dental alveolar positions are indicated with a letter representing the tooth-bearing bone (p for premaxilla, m for maxilla, d for dentary) and a number indicating the position of the tooth or alveolus in the tooth row.
Repositories and institutional abbreviations
All specimens discussed in this manuscript are housed at the National Museums of Kenya, Nairobi (KNM) or the Natural History Museum, London, UK (NHMUK).
Systematic paleontology
Crocodylia Gmelin, Reference Gmelin1789
Crocodylidae Cuvier, Reference Cuvier1807
Brochuchus Conrad et al., Reference Conrad, Jenkins, Lehmann, Manthi, Peppe, Nightingale, Cossette, Dunsworth, Harcourt-Smith and Mcnulty2013
Type species
Brochuchus pigotti (Tchernov and Van Couvering, Reference Tchernov and Couvering1978).
Revised diagnosis
Crocodylid with flat, slender rostrum. Five premaxillary, 14 maxillary, and 15 dentary teeth; teeth are circular in cross-section. Deep occlusal pits between maxillary alveoli, and the first eight alveoli are widely spaced. Alveoli project beyond surrounding surface, giving them a collared appearance. Nasals excluded from the external naris on dorsal surface of rostrum (shared with most longirostrine crocodylians, including Euthecodon). Naris opens anterodorsally. W-shaped maxillary-premaxillary suture on the palate, with the premaxilla extending back to the level of m2. Maxillary ramus of ectopterygoid forked (shared with Crocodylus). Dentary symphysis extends to a level between d5 and d6. The following characters are true for B. pigotti, but unknown in B. parvidens n. sp.: suborbital fenestra elliptical, and there are no concavities in its outline caused by laminae of the maxilla, palatine, or ectopterygoid. Palatine process slender and pointed, and extends anteriorly to the level of m7. Collared internal choana surrounded by a thin lamina of the pterygoid (shared with other osteolaemines). Prominent preorbital ridges; linear frontoparietal suture; skull table planar, and supratemporal fenestrae not constricted; supraoccipital exposed on dorsal skull table surface. Quadrate foramen aëreum on dorsomedial surface of quadrate ramus. Dorsal margin of axial neural spine oriented anterodorsally.
Remarks
Brochuchus pigotti was a relatively small animal—the largest cranial specimens suggest individuals ~2.5 m in total length (Conrad et al., Reference Conrad, Jenkins, Lehmann, Manthi, Peppe, Nightingale, Cossette, Dunsworth, Harcourt-Smith and Mcnulty2013). Its skull is gracile but not tubular, and like most such Cenozoic crocodyloids, the species was originally referred to Crocodylus (Tchernov and Van Couvering, Reference Tchernov and Couvering1978). The forms described here are broadly similar in size and skull shape.
The type species is known from a large number of specimens from the Hiwegi Formation (early Miocene, ca. 18 Ma) on Rusinga Island that collectively make it one of the best-sampled Neogene crocodylids. Phylogenetic analyses have consistently drawn the type species closer to Osteolaemus than to Crocodylus (Brochu, Reference Brochu2006, Reference Brochu2007; Brochu et al., Reference Brochu, Njau, Blumenschine and Densmore2010; Brochu and Storrs, Reference Brochu and Storrs2012). This prompted Conrad et al. (Reference Conrad, Jenkins, Lehmann, Manthi, Peppe, Nightingale, Cossette, Dunsworth, Harcourt-Smith and Mcnulty2013) to transfer the species to the new genus Brochuchus.
At present, Brochuchus is known with certainty only from sites in the Victoria and Turkana Basins in Kenya. Specimens referred to B. pigotti have been reported from Italy (Kotsakis et al., Reference Kotsakis, Delfino and Piras2004), but these were not figured and, not having seen them, we are unable to assess these referrals. Conrad et al. (Reference Conrad, Jenkins, Lehmann, Manthi, Peppe, Nightingale, Cossette, Dunsworth, Harcourt-Smith and Mcnulty2013) questioned whether mandibular material from the early Miocene of Saudi Arabia referred to B. pigotti (Buffetaut, Reference Buffetaut1984) was sufficient to support this conclusion. Indeed, the Saudi dentaries figured by Buffetaut (Reference Buffetaut1984) differ from Kenyan Brochuchus dentaries in important respects—there is greater size disparity between d4 and much smaller neighboring alveoli, the fourth alveolus is substantially larger relative to other dentary alveoli, alveoli behind the fourth are not as widely separated, the dentary symphysis is narrower, and the tooth row between d1 and d5 is more arcuate. Referral to Brochuchus is possible, but not yet demonstrable.
Tchernov and Van Couvering (Reference Tchernov and Couvering1978) included a dorsal premaxillary process extending to m2 in their diagnosis of B. pigotti. The dorsal premaxillary process extends between the maxilla and nasal on the rostral surface, and it does indeed terminate at the level of m2 in B. pigotti. It is somewhat longer in B. parvidens n. sp. (see below).
The morphological restoration of B. pigotti presented by Conrad et al. (Reference Conrad, Jenkins, Lehmann, Manthi, Peppe, Nightingale, Cossette, Dunsworth, Harcourt-Smith and Mcnulty2013) is generally accurate, but we disagree on one point. Conrad et al. (Reference Conrad, Jenkins, Lehmann, Manthi, Peppe, Nightingale, Cossette, Dunsworth, Harcourt-Smith and Mcnulty2013) reconstructed the skull with nasals that penetrate the narial aperture (Fig. 2.2). This was the condition assigned to B. pigotti by Brochu and Storrs (Reference Brochu and Storrs2012), although they interpreted the nasals as being restricted to a pair of slender processes approaching the naris, much like the nasals of Voay, and not the more robust structures depicted by Conrad et al. (Reference Conrad, Jenkins, Lehmann, Manthi, Peppe, Nightingale, Cossette, Dunsworth, Harcourt-Smith and Mcnulty2013). Further examination of material at the NHMUK (including the holotype) and KNM compels us to reinterpret the rostrum of B. pigotti. The premaxillae meet behind the naris, excluding the nasals from the narial rim (Fig. 2.3).

Figure 2. Anterior end of rostrum of NHMUK PV R 7729, holotype, Brochuchus pigotti. (1) Photograph of specimen. (2) Sutural interpretation based on Conrad et al., Reference Conrad, Jenkins, Lehmann, Manthi, Peppe, Nightingale, Cossette, Dunsworth, Harcourt-Smith and Mcnulty2013. (3) Sutural interpretation used in this study. dpp = dorsal posterior premaxillary process; en = external naris; if = incisive foramen; mx = maxilla; n = nasal; pmx = premaxilla. Scale = 5 cm.
Conrad et al. (Reference Conrad, Jenkins, Lehmann, Manthi, Peppe, Nightingale, Cossette, Dunsworth, Harcourt-Smith and Mcnulty2013) distinguished Brochuchus from Osteolaemus and Voay on the absence in Brochuchus of anterolateral processes on paramedian osteoderms, but these are absent from all crocodylids, including Osteolaemus and Voay.
Brochuchus parvidens new species Figures 3.3–3.6, 4.
Holotype
KNM-MB 36682, partial rostrum including most of both premaxillae, the right maxilla to m5, the left maxilla to m6, and the nasals between them (Fig. 3.3–3.6).
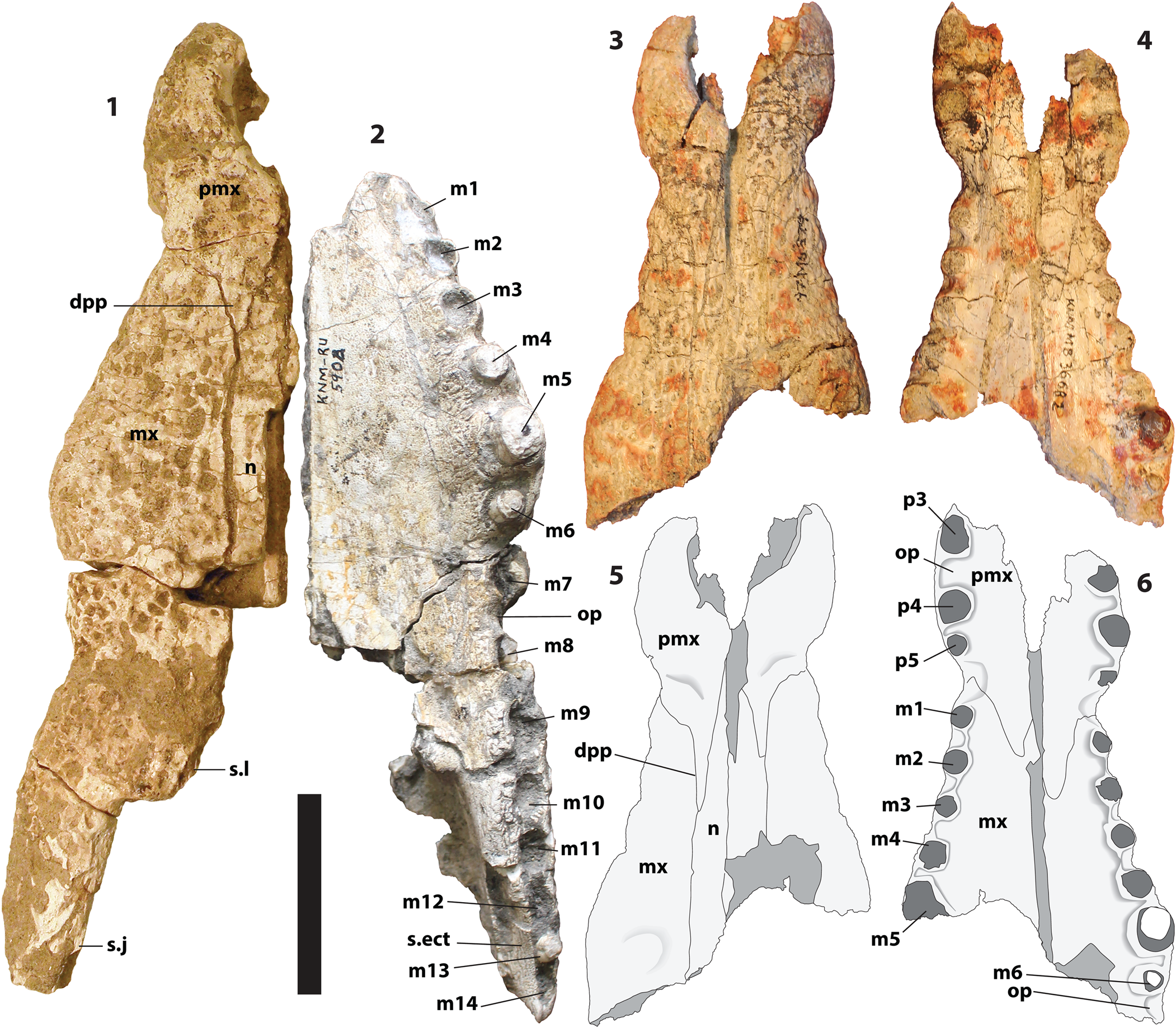
Figure 3. (1) NHMUK PV R 37517, Brochuchus pigotti, partial rostrum, dorsal view. (2) KNM RU-5904, Brochuchus pigotti, partial rostrum, ventral view. KNM-MB 36682, holotype, Brochuchus parvidens n. sp., partial skull in dorsal (3, 5) and ventral (4, 6) view. dpp = dorsal posterior premaxillary process; m1–m14 = maxillary dental/alveolar positions; mx = maxilla; n = nasal; op = occlusal pit; p3–p5 = premaxillary dental/alveolar positions; pmx = premaxilla; s.ect = sutural surface for ectopterygoid; s.j = sutural surface for jugal; s.l = sutural surface for lacrimal. Scale = 5 cm.
Diagnosis
Brochuchus differing from B. pigotti in having slender premaxillary processes on the dorsal surface of the rostrum and more widely spaced maxillary and dentary alveoli; among the first four maxillary alveoli, the gap between two alveoli is approximately as long as the diameter of the alveolus behind the gap.
Occurrence
Middle Miocene Maboko Formation, Maboko Main Locality, Maboko Island, Kenya (Fig. 1). All specimens were excavated in situ from Bed 3 at Maboko Main (see map in Geraads et al., Reference Geraads, McCrossin and Benefit2012). Laser fusion 40Ar/39Ar dating of alkali feldspar from the tuff of Bed 8 (K87-4149) up-section from Beds 3 and at Maboko Cliffs yielded six reasonable isotopic ages with a mean age of 15.4 Ma and most recent date of 14.7 Ma (Feibel and Brown, Reference Feibel and Brown1991). Biostratigraphic markers indicate an age younger than 16–18 Ma deposits at Rusinga (Van Couvering and Van Couvering, Reference Van Couvering, Van Couvering, Isaac and McCown1976; Pickford, Reference Pickford1981, Reference Pickford, Ciochon and Corruccini1983).
Bed 3 is a fossil-rich ~1 m thick unconsolidated oolitic packstone overlying the Bed 2 bentonite and capped by the hard Bed 4 calcrete. Several researchers suggest Maboko was part of a large floodplain (Van Couvering and Van Couvering, Reference Van Couvering, Van Couvering, Isaac and McCown1976; Andrews et al., Reference Andrews, Meyer, Pilbeam, Van Couvering and Van Couvering1981; Pickford, Reference Pickford1981, Reference Pickford, Ciochon and Corruccini1983). Sedimentological analysis of the ooids and oncoids in Bed 3, along with faunal and isotopic evidence, indicate likely deposition as a low energy freshwater beach ramp or littoral environment in a forest or woodland setting (McCrossin et al., Reference McCrossin, Benefit, Gitau, Palmer, Blue, Fleagle, McHenry and Rosenberger1998; Benefit, Reference Benefit1999; Retallack et al., Reference Retallack, Wynn, Benefit and McCrossin2002; Watkins, Reference Watkins2004; Arney et al., Reference Arney, Maclatchy, Benefit, McCrossin and Kingston2018). Water appears to have receded late in the history of Bed 3, leading to deposition of the Bed 4 calcrete. This may explain the absence of Brochuchus above Bed 3. A local habitat change at Maboko Main is indicated by the transition to Bed 4 calcrete overlain by Bed 5b mudstone, both with horizontal bedding (Watkins, Reference Watkins2004). Faunal transitions between Bed 3 and 5b include dramatic increases in the relative abundance of tragulids and the highly arboreal ape Mabokopithecus, accompanied by a decrease in the abundance of the presumably woodland giraffoid Climacoceras (Benefit, Reference Benefit1999; Retallack et al., Reference Retallack, Wynn, Benefit and McCrossin2002). Whereas numerous birds typically associated with large expanses of water occur higher in the sequence in Bed 5w (pelican, stork, cormorant, and flamingo), the only bird known from Bed 5b is a wader (Charadrii) typically associated with mudflats, and the only bird known from Bed 3 is a hornbill, which can occur in a range of habitats from forest to savanna (Mayr, Reference Mayr2014). Bed 5b may have been a forested wetland or swamp environment (Benefit, Reference Benefit1999).
Description
Each premaxilla of the holotype preserves the distalmost three alveoli (Fig. 3.4, 3.6). The incisive foramen was evidently small, but its outline is not preserved. A deep occlusal pit separates p3 and p4, and there was evidently a large pit on the palatal surface mesial to p3. The fourth and fifth alveoli are close together. The premaxillae are dorsoventrally narrower anteriorly than posteriorly, and the naris opens anterodorsally.
The left and right sides of the holotype are displaced, with the left side elevated relative to the right. The premaxillae are no longer in contact, and the nasals separate anteriorly. As a result, sutural relationships around the naris are open to interpretation. Nevertheless, we are reasonably certain the nasals did not reach the naris on the external surface (Fig. 3.3, 3.5). The anterior end of the left nasal, as preserved, is acute. The medial sutural surface on the premaxilla behind the naris is parasagittal, suggesting it was contacting something on the sagittal plane. The apparent groove posterior to the naris is caused by depression of the right nasal and premaxilla relative to their left counterparts.
The dorsal premaxillary processes are very slender and appear to be rather long (Fig. 3.3, 3.5). That it appears shorter on the right is because the anterior tip of the right nasal is broken away. Despite their length, they only reach the level of m3. This can be seen both on the holotype and on KNM-MB 25736, which does not preserve the premaxilla itself, but which preserves a clear sutural surface for it (Fig. 4.1). Indeed, on the right side of the holotype, the process appears to reach a level between m2 and m3. Normally, processes as long as these would extend beyond m3. In this case, the alveoli are more widely spaced than in most other crocodylians. In effect, the alveoli moved posteriorly relative to the process.

Figure 4. KNM-MB 25736, Brochuchus parvidens n. sp., partial left maxilla, dorsal (1) and ventral (2) view. (3) KNM-RU 2583, Brochuchus pigotti, right dentary, dorsal view. KNM-MB 28142, Brochuchus parvidens n. sp., left dentary, dorsal (4), lateral (5), and medial (6) view. (7) KNM-MB 22102, Brochuchus parvidens n. sp., partial right dentary, dorsal view. d4 = fourth dentary tooth/alveolus; m1–m5 = maxillary dental/alveolar positions; s.pmx = sutural surface for premaxilla; sym = dentary symphysis. Scale = 5 cm.
The premaxillary-maxillary sutural surface on the palate is convex posteriorly, with the premaxilla extending to the level of the second maxillary alveolus (Fig. 4.2). The suture as a whole is W-shaped (Fig. 3.4, 3.6).
Four complete alveoli are preserved on the right maxilla of the holotype (Fig. 3.4, 3.6). The fifth alveolus is partially preserved. The same is true of KNM-MB 25736 (Fig. 4.2). The left maxilla of the holotype preserves six alveoli and an occlusal pit posterior to the sixth. The tooth row has an almost linear arrangement from m1 through m5 (Fig. 3.4, 3.6). The fifth alveoli are the largest, and the left m5 bears a slender, lingually recurved tooth with low unserrated mesiodistal carinae. The alveoli are widely separated, and occlusal pits between the first four alveoli are almost as large as the preceding alveolus. The fourth and fifth alveoli are not as widely spaced from each other. A small boss for the fifth tooth root is preserved on the dorsal surface of the left maxilla of the holotype and, indistinctly, KNM-MB 25736.
The medial wall of the caviconchal recess is exposed on KNM-MB 25736. There are three or four shallow pits dorsal to the first two alveoli, but the surface is smooth otherwise.
The preserved dentaries are from substantially smaller animals than the holotype. Neither preserves the anteriormost alveoli, but we assume the largest preserved on both specimens is d4 (Fig. 4.4, 4.7); in other generalized crocodylids, there is minimal difference in diameter among other mesial dentary alveoli. Based on this, the dentary symphysis extends either to the level of d6 (KNM-MB 28142; Fig. 4.4) or to a level between d5 and d6 (KNM-MB 22102; Fig. 4.7). The symphyseal part of the mandible would have been mediolaterally narrow, and the dentary posterior to the symphysis remains slender, with only a modest amount of dorsal expansion approaching d10 and d11. Alveoli are widely spaced, with a larger diastema between d8 and d9. The third and fourth alveoli are also widely separated. The splenial did not reach the symphysis, and based on the sutural surface on KNM-MB 22102 (Fig. 4.6), it terminated at the level of d7.
Etymology
parvi, from Latin parvus, small; dens, Latin, tooth. The name refers to the relatively small alveoli in the maxilla and dentary compared with homologues in B. pigotti.
Materials
KNM-MB 22099, partial maxilla; KNM-MB 22102, partial right dentary (Fig. 4.7); KNM-MB 25736, partial left maxilla (Fig. 4.1, 4.2); KNM-MB 28142, left dentary (Fig. 4.4–4.6).
Remarks
Differences between B. pigotti and B. parvidens n. sp. are modest but consistent across multiple specimens. The most obvious is the shape of the premaxillary process on the dorsal surface. The process is triangular in both species, but it is visibly narrower in B. parvidens n. sp. than in B. pigotti. The condition in B. parvidens n. sp. is most evident in the holotype (Fig. 3.3), which preserves the premaxillae, but it can also be discerned on the much smaller isolated left maxilla (KNM-MB 25736), which preserves the sutural surface for the premaxilla (Fig. 4.1). The process is consistently more robust in B. pigotti (Figs. 2.3, 3.1). That the premaxillary process of B. parvidens n. sp. is slender in two specimens of very different size, the largest of which are of similar size to large specimens of B. pigotti, argues against an ontogenetic explanation.
The slender premaxillary process may reflect a mediolaterally contracted snout overall. The holotype of B. parvidens n. sp. is of approximately equivalent size with the holotype and several referred specimens of B. pigotti, but the rostrum appears broader between the fifth maxillary teeth in B. pigotti than in B. parvidens n. sp. The symphysial surface of the dentary is also visibly narrower in B. parvidens n. sp., although because the only known dentaries of B. parvidens n. sp. are from small individuals, this could be a matter of scaling.
Alveolar separation also distinguishes the two species. The second, third, and fourth maxillary alveoli are only slightly wider than the diastemata anterior to them; the average ratio between the diameter of an alveolus and the length of the diastema in front of it (e.g., diameter of m2/distance between m1 and m2) is 1.16 (m2), 1.15 (m3), and 1.29 (m4) for two specimens of B. parvidens n. sp. (KNM-MB 36682 and KNM-MB25736). Conversely, the same alveoli are more than twice as wide as their preceding diastemata in B. pigotti, with ratios 2.10 (m2), 2.06 (m3), and 2.32 (m4) for three specimens (NHMUK PV R 7729, KNM-RU 5904, KNM-RU 2597). The third and fourth dentary alveoli also seem to be more widely spaced in B. parvidens n. sp.
Relative distances between centers of maxillary alveoli are the same in both species. Wider separation in B. parvidens n. sp. results from smaller alveolar diameter, which increases interalveolar space. This, too, might reflect decreased snout width; most living and extinct slender-snouted crocodylians, including gharials and Mecistops, have comparatively gracile teeth, although these forms also have elongated snouts that, in most cases, spread the alveoli further apart.
The dentary symphysis might be longer in B. parvidens n. sp. than in B. pigotti. On the more complete B. parvidens n. sp. dentary (KNM-MB 28142; Fig. 4.4–4.6), its posterior limit is somewhat closer to d6 than in most B. pigotti dentaries (e.g., Fig. 4.3). But this is not the case with the other dentary fragment referred to B. parvidens n. sp. (Fig. 4.7), and this is subject to minor amounts of variation among living crocodylians (Brochu, Reference Brochu2000b). A larger sample is needed to assess the consistency of this difference.
Brochuchus sp. Figure 5.1–5.3.
Occurrence
Kalodirr Member, Lothidok Formation, Moruorot (Fig. 1). Deposits at this site west of Lake Turkana are correlative with those at Kalodirr to the southeast (see below).
Materials
KNM-MO 91, right maxilla (Fig. 5.1–5.3).
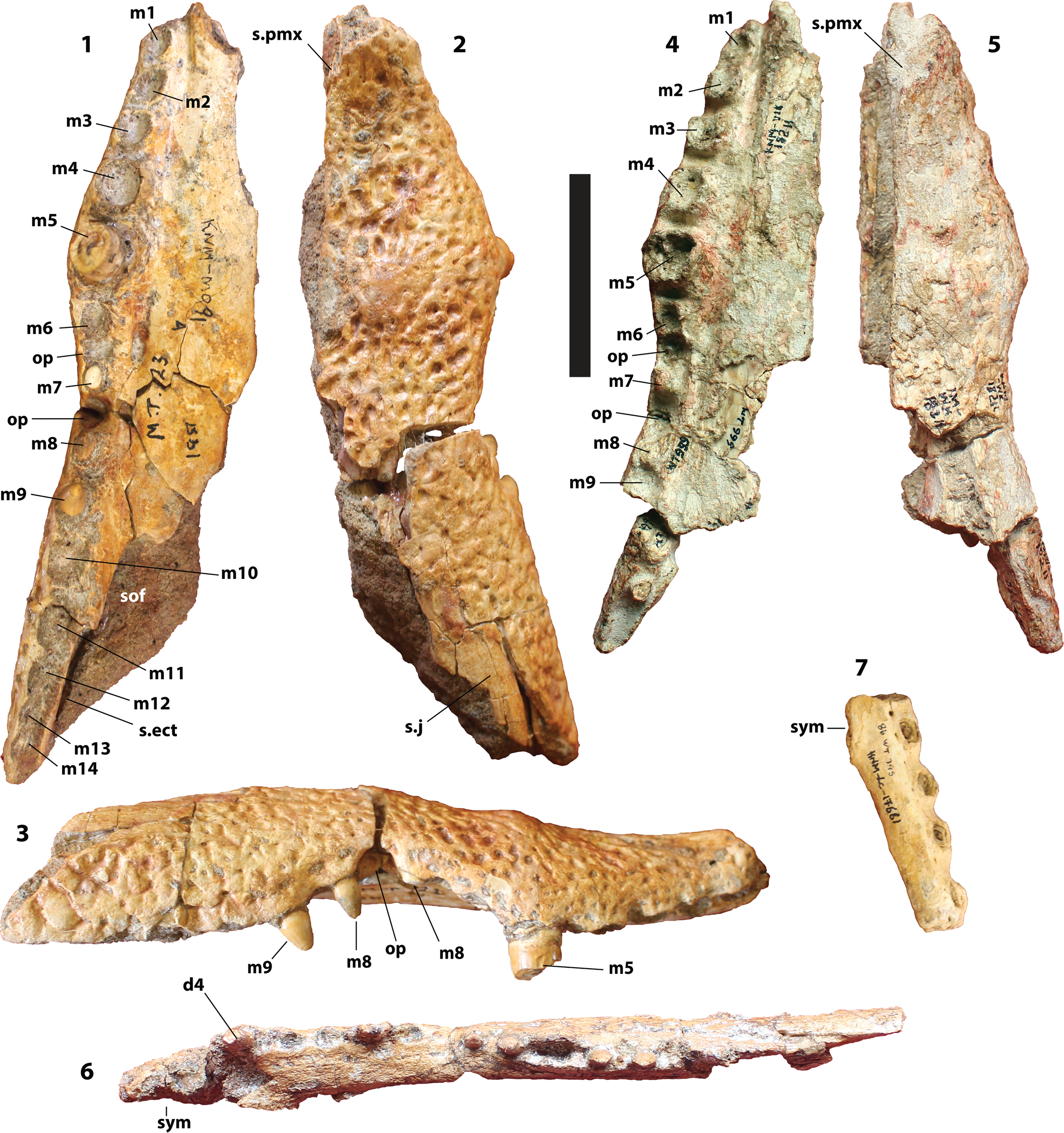
Figure 5. KNM-MO 91, Brochuchus sp. from Morurorot, right maxilla, ventral (1), dorsal (2), and lateral (3) view. KNM-WK 18211, Brochuchus sp. from Kalodirr, right maxilla, ventral (4) and dorsal (5) view. (6) KNM-WK 18210, Brochuchus sp. from Kalodirr, partial right dentary, dorsal view. (7) KNM-LC 17661, Brochuchus sp. from Loncherangan, partial right dentary, dorsal view. d4 = fourth dentary tooth/alveolus; m1–m14 = maxillary dental/alveolar positions; op = occlusal pit; s.ect = sutural surface for ectopterygoid; s.j = sutural surface for jugal; s.pmx = sutural surface for premaxilla; sof = suborbital fenestra; sym = dentary symphysis. Scale = 5 cm.
Remarks
This specimen preserves, either completely or in part, fourteen maxillary alveoli surrounded by thin crests, imparting a collared appearance (Fig. 5.1). There are deep occlusal pits between m6 through m8, and the pit between m7 and m8 is especially deep with a scalloped lateral surface expressed as a discrete concavity on the ventral margin of the bone in lateral view (Fig. 5.1, 5.3). The suborbital fenestra extends anteriorly to a level between m9 and m10, and the ectopterygoid was adjacent to the last four maxillary alveoli. Matrix covers most of the medial surface, preventing us from assessing the shape of the ectopterygoid, and although a small uncovered section of the medial surface at the anterior end lacks pits on the caviconchal recess wall, we do not know whether such pits were absent posteriorly. The maxilla-premaxilla suture is not preserved on the palate.
The sutural surface for the premaxilla is visible dorsally (Fig. 5.2). It suggests a rather robust premaxillary process. The surface appears to be oriented medially more than anteriorly toward its posterior end, which would suggest a slender process, but it is unclear whether this is an actual sutural surface or breakage. In either case, even if the process was slender posteriorly, it was robust anteriorly. The jugal sutural surface is visible as a smooth patch toward the posterior end of the specimen.
Brochuchus sp. Figure 5.4–5.6.
Occurrence
Kalodirr Member, Lothidok Formation, Kalodirr, west of Lake Turkana (Fig. 1). These deposits have been constrained by radiometric dates from under- and overlying tuffs to between 17.7 Ma and 16.6 Ma (Boschetto et al., Reference Boschetto, Brown and Mcdougall1992).
Remarks
The maxilla (KNM-WK 18211; Fig. 5.4, 5.5) preserves sutural contacts for the premaxilla anteriorly, nasal medially, and ectopterygoid posteromedially. The medial surface is covered with matrix. Thirteen or 14 alveoli were present; the number is unclear because the posterior end of the maxilla is not attached to the rest of the element, but the alveoli are surrounded by thin crests and separated by deep occlusal pits. The sutural contact for the premaxilla is preserved on the maxilla, but the dorsal premaxillary process itself is not preserved.
The symphysis of the right dentary (Fig. 5.6) extends to a level between d5 and d6. The splenial terminated behind the symphysis at the level of d7. Alveoli are widely spaced and were evidently surrounded by tall collars, although these are imperfectly preserved.
The teeth preserved with the maxilla and dentary are slender and, where the apex is preserved, conical. They bear modest unserrated mesiodistal carinae.
Brochuchus sp. Figure 5.7.
Occurrence
Loncherangan, west of Lake Turkana (Fig. 1). An underlying ash bed gives a radiometric age of ca. 17.5 Ma, and the mammalian fauna is similar to those of Kalodirr and Moruorot (Anyonge, Reference Anyonge1991).
Materials
KNM-LC 17661, partial right dentary (Fig. 5.7); KNM-LC 17672, maxilla fragment; KNM-LC 18458, left dentary fragment; KNM-LC 18460, maxilla fragment.
Remarks
Although fragmentary, this material is sufficient to establish a form of Brochuchus at Loncherangan. The dentary symphysis (Fig. 5.7) extended to d6, and the alveoli are widely spaced and collared. Maxillary alveoli are likewise surrounded by collars, and there are deep occlusal pits between them. KNM-LC 17672 may preserve m5—one of the two preserved alveoli is large, and the dorsal surface is expanded in the manner of a boss over the fifth tooth root.
Comparisons
Several character states allow referral of these specimens to Brochuchus. The most notable is the configuration of the maxillary alveoli. They are surrounded by prominent collars and separated by deep occlusal pits (Figs. 2.3, 3.2, 3.4, 4.2, 5.1, 5.4). Most crocodylid maxillary teeth are separated by occlusal pits and surrounded by collars, but the pits of Brochuchus are especially deep, and their depth is accentuated by the more prominent circumalveolar collars.
These specimens share additional features with B. pigotti, although these may have a broader distribution among crocodylids. The nasals did not reach the external naris, for example, but this is also true for Rimasuchus lloydi, “Crocodylus” gariepensis, and almost any tube-snouted crocodyliform, including Euthecodon and Mecistops.
Moruorot and Kalodirr are equivalent in age and in close physical proximity, and we would expect Brochuchus from both to be conspecific. In both cases, the maxillae more closely resemble those of B. pigotti. In particular, the dorsal premaxillary process appears to be robust. Distances between alveoli on the Moruorot form are also similar to those of B. pigotti (Fig. 5.1). At first glance, they appear to be greater and more like those of B. parvidens n. sp. on the Kalodirr maxilla (Fig. 5.4), but the appearance of greater distance results from better preserved alveolar collars.
Referral of the Moruorot and Kalodirr material to B. pigotti might be a reasonable approach, but we are hesitant because the maxillae from Moruorot and Kalodirr appear to be somewhat narrower than in B. pigotti. This is most apparent on the Kalodirr maxilla, which preserves the midline palatal suture (Fig. 5.4). But this is also from a smaller individual than most maxillae from Rusinga. The difference could be ontogenetic. In the absence of a larger sample, we would rather not identify this material beyond the level of Brochuchus.
Results
The analysis recovered 46 equally optimal trees with a length of 214, CI excluding uninformative characters of 0.541, and an RI of 0.719 (Fig. 6). Most aspects of the optimal trees are consistent with those from previous analyses—extant Crocodylus forms a clade with Paleoafrican Crocodylus and Crocodylus checchiai Maccagno, Reference Maccagno1948, and there is a well-supported Crocodylidae excluding mekosuchines and “Crocodylus” megarhinus Andrews, Reference Andrews1905. Moreover, as with most recent analyses using this matrix (e.g., Brochu and Storrs, Reference Brochu and Storrs2012; Drumheller and Brochu, Reference Drumheller and Brochu2016), although there are groups including Osteolaemus and Voay (Osteolaemini) and Euthecodon, Brochuchus, and “Crocodylus” gariepensis (Euthecodonini), this analysis failed to unambiguously support a monophyletic Osteolaeminae including both groups. In fact, relationships at the root of Crocodylidae appear poorly resolved.
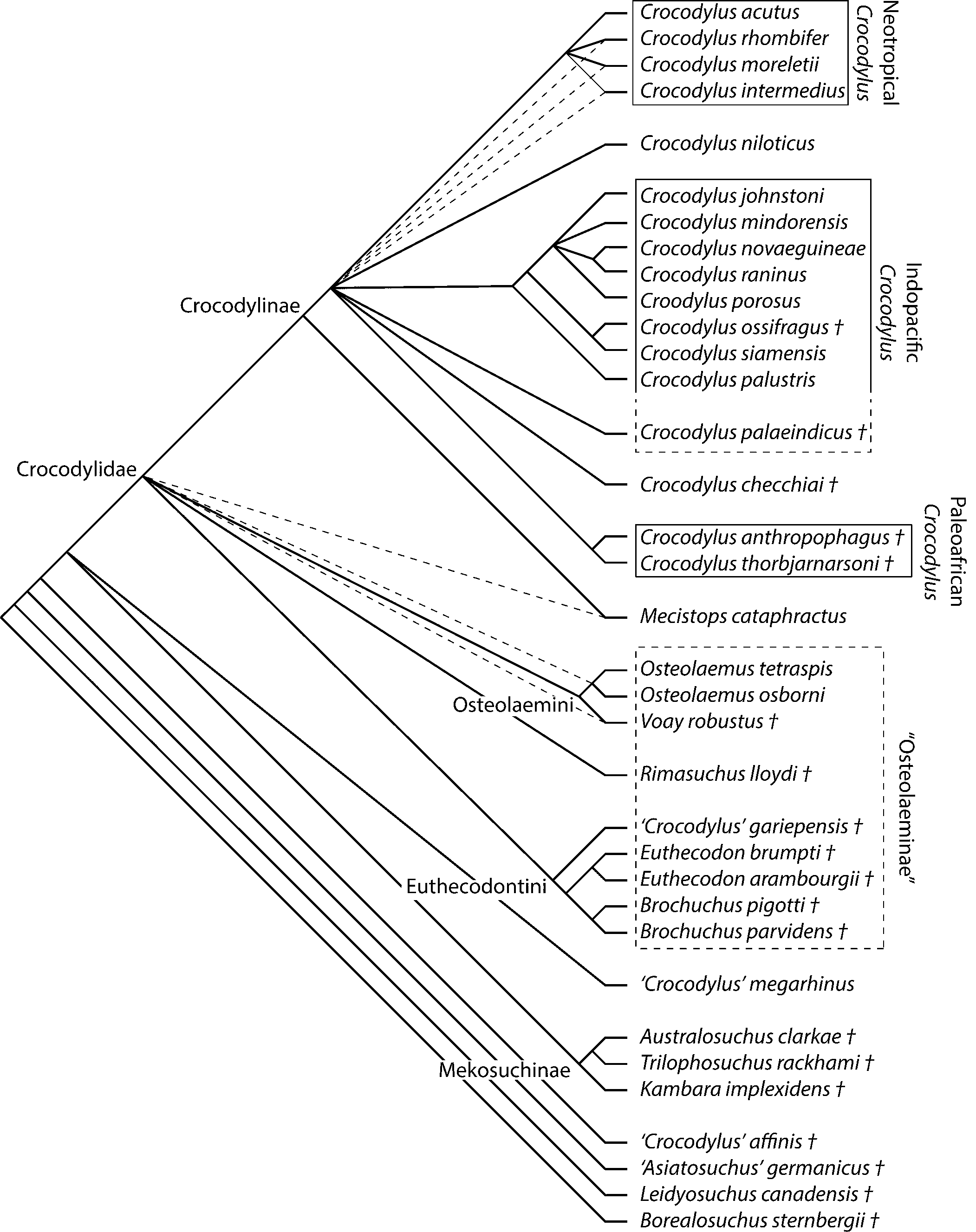
Figure 6. Adams consensus of 46 equally optimal trees (length = 214, CI = 0.54, RI = 0.719) based on maximum parsimony analysis of 33 ingroup taxa and 189 morphological characters. Borealosuchus sternbergii and Leidyosuchus canadensis were used as outgroups. Dashed branches on tree indicate collapsed resolution in a strict consensus of these trees.
Dampened resolution arises in part from incomplete coding of fossils, but character conflict is also a factor. One of the topological miscreants is Crocodylus checchiai, which shares a derived midrostral boss (character 95) with Neotropical Crocodylus, but a plesiomorphic sutural configuration around the infratemporal fenestra (character 145) with Paleoafrican Crocodylus. Paleoafrican Crocodylus and C. checchiai are basal to extant Crocodylus in some optimal trees, but C. checchiai and sometimes Paleoafrican Croodylus are closer to Neotropical Crocodylus in others.
The relationships among Crocodylus, Mecistops, osteolaemins, and euthecodonins are unresolved in the strict consensus tree (Fig. 6), but the number of optimal trees is small compared with number of possible resolutions of this polytomy, suggesting conserved structure masked by a strict consensus approach. Mecistops is closer to Crocodylus than to other crocodylids in the Adams consensus tree, and Mecistops is always closer to Crocodylus than to Osteolaemus in the individual optimal trees. This is incongruent with molecular analyses (e.g., Gatesy et al., Reference Gatesy, Amato, Norell, Desalle and Hayashi2003; McAliley et al., Reference McAliley, Willis, Ray, White, Brochu and Densmore2006; Li et al., Reference Li, Wu, Ji, Yan and Amato2007; Meganathan et al., Reference Meganathan, Dubey, Batzer, Ray and Haque2010; Meredith et al., Reference Meredith, Hekkala, Amato and Gatesy2011; Oaks, Reference Oaks2011), but consistent with previous morphological analyses (e.g., Brochu, Reference Brochu2000a, Reference Brochu2007; Piras et al., Reference Piras, Delfino, Del Favero and Kotsakis2007; Brochu and Storrs, Reference Brochu and Storrs2012; Scheyer et al., Reference Scheyer, Aguilera, Delfino, Fortier, Carlini, Sánchez, Carrillo-Briceño, Quiroz and Sánchez-Villagra2013; Wu and Brinkman, Reference Wu and Brinkman2015; Narváez et al., Reference Narváez, Brochu, Escaso, Pérez-Garcia and Ortega2016; Massonne et al., Reference Massonne, Vasilyan, Rabi and Böhme2019; Groh et al., Reference Groh, Upchurch, Barrett and Day2020).
Relationships at the root of Crocodylidae collapse because Euthecodontini assumes two different positions in the set of optimal trees. This group is either closer to M. cataphractus (Fig. 7.1) or to Ostelaemini (Fig. 7.2). Rimasuchus Storrs, Reference Storrs, Leakey and Harris2003 is always an osteolaemine, but whether it is an osteolaemin depends on the placement of euthecodontins. Bimodally labile taxa cause a loss of resolution disproportionate to the number of trees recovered (e.g., Sumrall et al., Reference Sumrall, Brochu and Merck2001; Wilkinson and Thorley, Reference Wilkinson and Thorley2001; Sharkey et al., Reference Sharkey, Stoelb, Miranda-Esquivel and Sharanowski2013), though in this case, the labile taxon is a clade rather than a single wildcard terminal.
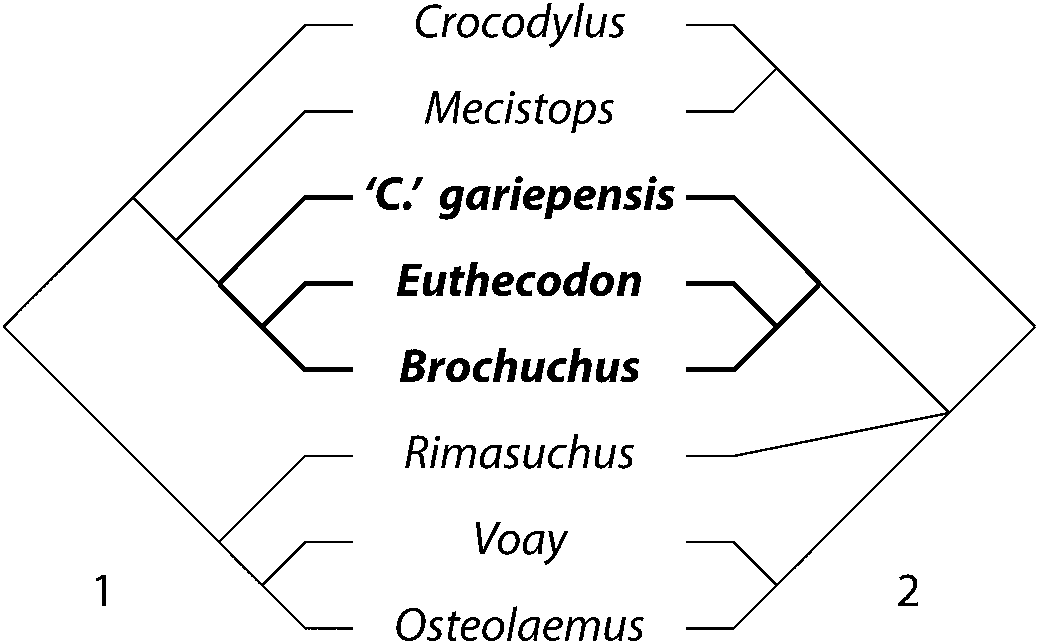
Figure 7. Alternative phylogenetic positions of Euthecodontini in the optimal trees recovered in this analysis. (1) Euthecodontini includes Mecistops and is closer to Crocodylus. (2) Euthecodontini excludes Mecistops and is closer to Osteolaemus. Euthecodontini is subordinate to Crocodylinae in (1) and Osteolaeminae in (2).
There are compelling reasons to question a grouping of Euthecodontini (including Mecistops) and Crocodylus. The three unambiguous synapomorphies supporting this clade are problematic. One is a wasp-waisted iliac blade (character 34; Brochu, Reference Brochu2000a). The ilium is currently unknown for Euthecodon and “C.” gariepensis, and although an ilium has been referred to B. pigotti, its blade is missing (Conrad et al., Reference Conrad, Jenkins, Lehmann, Manthi, Peppe, Nightingale, Cossette, Dunsworth, Harcourt-Smith and Mcnulty2013).
The second is the absence of ventral armor (character 42). The known absence of such armor in Mecistops and Crocodylus, along with its known presence in Osteolaemus and in the outgroups used in this study, does render the condition in Crocodylus and Mecistops derived; but a broader ingroup sample (including tomistomines) would have called this polarity into question, and although definitive ventral osteoderms have never been found associated with the fossil crocodylids in this analysis, we cannot say these forms lacked ventral armor.
The third unambiguous synapomorphy, passage of the surangular-angular suture along the posteroventral margin of the mandibular fenestra on the lateral surface of the mandible (character 60), has a complex distribution among crocodylids. Euthecodon brumpti was coded on the basis of Plio-Pleistocene specimens from Omo (where the holotype was collected) and Koobi Fora; although the derived state pertains to this material, it does not to Euthecodon from earlier Pliocene deposits at Kanapoi (CAB, personal observation), and the condition is unknown in Euthecodon arambourgii Ginsburg and Buffetaut, Reference Ginsburg and Buffetaut1978. The derived state is also found in Osteolaemus tetraspis, but not O. osborni (Schmidt, Reference Schmidt1919).
A fourth character state may diagnose this group—a truncated ascending surangular lamina (character 67). This refers to a structure adjacent to the posterior wall of the glenoid fossa. In most crocodylians, it extends all the way to the dorsal tip of the articular. In Crocodylus and Mecistops, the lamina is truncated and does not extend substantially beyond the adjacent dorsal surface of the mandible (Brochu, Reference Brochu2000a). This is true of “C.” gariepensis, E. brumpti, and B. pigotti, but also of Voay robustus (Grandidier and Vaillant, Reference Grandidier and Vaillant1872), and so the derived state could either pertain to Crocodyidae with a reversal in Osteolaemus or independent gains in Crocodylus + Euthecodontini and Voay.
Support for a basal Mecistops among euthecodontins is equally problematic. In some trees, this group is united by external exclusion of the nasals from the naris (character 82). But this is only unambiguously true when Crocodylus checchiai, which also shares this feature, is close to Neotropical Crocodylus and not part of a basal Paleoafrican Crocodylus assemblage. Mecistops and Euthecodon also share an anterior surangular spur extending along the lingual margin of at least one dentary alveolus (character 62), but this is true of many tube-snouted crocodylians (Brochu, Reference Brochu1997) and is unknown in Brochuchus or “C.” gariepensis.
In trees uniting osteolaemins and euthecodontins, osteolaemine monophyly is supported by three unambiguous synapomorphies—prominent preorbital ridges (character 97); a thin bony crest, or “neck,” surrounding the internal choana (character 123); and extension of the squamosal over the dorsal surface of the quadrate ramus (character 159). This last character was discussed and figured by Brochu (Reference Brochu2007). Expanded sampling of these taxa suggests more variable expression than previously thought, and continued use of this character may not be advisable.
In all optimal trees, Euthecodontini (excluding Mecistops) is supported by an anterodorsally opening external naris (character 81). The naris ancestrally opens dorsally in crocodyloids, and this is the condition in Crocodylus, Mecistops, and osteolaemins. In euthecodontins, the anterior margin of the naris is depressed relative to the posterior margin. Euthecodonini is supported by exclusion of the nasals from the naris when the group is within Osteolaeminae, and by preorbital ridges when linked to Mecistops and Crocodylus.
Euthecodon and B. pigotti share a linear frontoparietal suture (character 151). The suture is anteriorly concave in most crocodylids, including R. lloydi and V. robustus, though it is also linear in all forms of Osteolaemus. The absence of a septum within the internal choana (character 125), evident in both species of Euthecodon and in B. pigotti, may also diagnose a Euthecodon + Brochuchus clade, although this is also true for Crocodylus thorbjarnarsoni.
In all trees, B. pigotti and B. parvidens n. sp. unambiguously share a notch on the dorsal surface of the premaxilla posterolateral to the external naris (character 86). Beyond Brochuchus among crocodylians, such a notch is only seen in alligatoroids (Brochu, Reference Brochu1999; Piras and Buscalioni, Reference Piras and Buscalioni2006). Brochuchus pigotti is characterized by a wedge-shaped palatine process (character 116) and tall, prominent posterior pterygoid processes lateral to the eustachian foramina (character 171). Neither of these could be assessed for B. parvidens n. sp., and the tall pterygoid processes are a reversal to the plesiomorphic eusuchian condition.
Comparison with other studies
Conrad et al. (Reference Conrad, Jenkins, Lehmann, Manthi, Peppe, Nightingale, Cossette, Dunsworth, Harcourt-Smith and Mcnulty2013) and some subsequent analyses (e.g., Lee and Yates, Reference Lee and Yates2018; Groh et al., Reference Groh, Upchurch, Barrett and Day2020) recovered Brochuchus as an osteolaemine, but Euthecodon as a close relative of Mecistops, with Euthecodon + Mecistops closer to Crocodylus than to Osteolaemus. This is not surprising because Euthecodon and Mecistops are both derived crocodylids with long, slender snouts, and some of the characters used in crocodyliform phylogenetics are correlated with this condition (e.g., Clark, Reference Clark, Fraser and Sues1994; Pol and Gasparini, Reference Pol and Gasparini2009; Iijima, Reference Iijima2017; Drumheller and Wilberg, Reference Drumheller and Wilberg2020; Groh et al., Reference Groh, Upchurch, Barrett and Day2020). Moreover, because Lee and Yates (Reference Lee and Yates2018) drew much of their information for B. pigotti from Conrad et al. (Reference Conrad, Jenkins, Lehmann, Manthi, Peppe, Nightingale, Cossette, Dunsworth, Harcourt-Smith and Mcnulty2013), whose analysis separated B. pigotti from Euthecodon, a similar outcome would be expected.
Although the matrix used here and that used by Conrad et al. (Reference Conrad, Jenkins, Lehmann, Manthi, Peppe, Nightingale, Cossette, Dunsworth, Harcourt-Smith and Mcnulty2013) are both based on the data set of Brochu and Storrs (Reference Brochu and Storrs2012), there are several differences. Conrad et al. (Reference Conrad, Jenkins, Lehmann, Manthi, Peppe, Nightingale, Cossette, Dunsworth, Harcourt-Smith and Mcnulty2013) included two additional terminals—what they called “Crocodylus” niloticus from the Pliocene of Kanapoi and a specimen from the late Miocene of Lothagam (KNM-LT 24081), both of which fell out as related to Osteolaemus in their analyses. This material is all referable to Crocodylus (Brochu and Storrs, Reference Brochu and Storrs2012; Brochu, Reference Brochu2020).
Brochuchus and Euthecodon take different positions in Conrad et al. (Reference Conrad, Jenkins, Lehmann, Manthi, Peppe, Nightingale, Cossette, Dunsworth, Harcourt-Smith and Mcnulty2013) and our analysis because of different codings for B. pigotti in the two studies. We replaced ours for theirs, and in the absence of their interpretations of Kanapoi and Lothagam Crocodylus (and in the absence of B. parvidens n. sp.), we replicated their results. Many of the differences reflect a more conservative approach to character assessment in this study; in several cases, Conrad et al. (Reference Conrad, Jenkins, Lehmann, Manthi, Peppe, Nightingale, Cossette, Dunsworth, Harcourt-Smith and Mcnulty2013) made a determination we were unwilling to make. Others indicate differences of opinion about the character state preserved in the material. All such differences are indicated in the supplemental data.
Three characters appear to be most responsible for the different outcomes. First, Conrad et al. (Reference Conrad, Jenkins, Lehmann, Manthi, Peppe, Nightingale, Cossette, Dunsworth, Harcourt-Smith and Mcnulty2013) agreed with Brochu (Reference Brochu2007) and Brochu and Storrs (Reference Brochu and Storrs2012) in believing the nasals contributed to the narial rim in B. pigotti. Exclusion of the nasals from the naris thus links Euthecodon and Mecistops to the exclusion of Brochuchus in their study. Second, Conrad et al. (Reference Conrad, Jenkins, Lehmann, Manthi, Peppe, Nightingale, Cossette, Dunsworth, Harcourt-Smith and Mcnulty2013) interpreted the surangular-angular suture as intersecting the mandibular fenestra posteriorly rather than posteroventrally. Whether the derived condition (posteroventral intersection) actually pertains to Euthecodon as a whole is an open question (see above), but it does link Euthecodon and Mecistops in their study. We disagree that the specimens available are sufficient to make a determination for B. pigotti.
Third, Conrad et al. (Reference Conrad, Jenkins, Lehmann, Manthi, Peppe, Nightingale, Cossette, Dunsworth, Harcourt-Smith and Mcnulty2013) coded B. pigotti has having an incisive foramen that abuts the premaxillary toothrow (character 89). This is actually not the case in either species of Brochuchus, but it would have linked B. pigotti with Euthecodon, which has this condition. Lee and Yates (Reference Lee and Yates2018) further assigned this state (incorrectly) to other crocodyloids, including M. cataphractus, all extant species of Crocodylus except C. acutus Cuvier, Reference Cuvier1807, Paleoafrican Crocodylus, both Osteoalemus, Kambara Willis et al., Reference Willis, Molnar and Scanlon1993, and “Crocodylus” megarhinus. In all of these except “C.” megarhinus, the incisive foramen is demonstrably situated farther back on the palate and does not abut the premaxillary tooth row; none of the specimens of “C.” megarhinus has a completely preserved incisive foramen, and the state cannot be assessed. Lee and Yates (Reference Lee and Yates2018) also miscoded B. pigotti as unknown for the extent of the dorsal surangular lamina—nearly flush with the surrounding dorsal mandibular surface rather than extending to the dorsal tip of the glenoid fossa—which further diminished similarities between Brochuchus and Euthecodon in their analysis.
New characters added by Lee and Yates (Reference Lee and Yates2018) supporting a close relationship between Mecistops and Euthecodon are generally correlated with having a long, slender snout, such as deep occlusal sulci on the lateral margin of the premaxilla. Slender-snouted forms, such as Euthecodon and Mecistops, generally do have deeper sulci in the premaxillary outline, especially between p1 and p2 or p3. There is less room in the rostrum for occlusal pits between alveoli, and so the dentary teeth occlude externally to a greater degree. Nearly all slender-snouted forms in their analysis were coded as having this condition. It is unclear, though, why Lee and Yates (Reference Lee and Yates2018) scored Brochuchus this way, as the condition in Brochuchus is indistinguishable from that of the majority of generalized crocodyliforms.
Discussion
Differences between Brochuchus parvidens n. sp. and B. pigotti are minor. Although some might regard them as insufficient to support species-level separation, we now know that many extant crocodylids are cryptic complexes of two or more species with very similar morphology (e.g., Eaton et al., Reference Eaton, Martin, Thorbjarnarson and Amato2009; Meredith et al., Reference Meredith, Hekkala, Amato and Gatesy2011; Smolensky, Reference Smolensky2015; Milián-García et al., Reference Milián-García, Castellanos-Labarcena, Russello and Amato2018; Shirley et al., Reference Shirley, Carr, Nestler, Vliet and Brochu2018; Murray et al., Reference Murray, Russo, Zorrilla and McMahan2019). Osteological distinctions between these, where documented, are very subtle. Differences between B. pigotti and B. parvidens n. sp. may be modest, but they are consistent among multiple specimens of similar size and can be used to distinguish morphologically distinct assemblages.
One is tempted to regard Brochuchus parvidens n. sp. as part of a continuously evolving lineage, also including B. pigotti. The two forms are very similar, and they occur in close geographic proximity; indeed, isolation of the Lake Turkana depositional basin postdates the Miocene, and sites proximate to present-day Lake Victoria and Lake Turkana might have been fluvially connected in the early to middle Miocene (e.g., Feibel, Reference Feibel2011; Macgregor, Reference Macgregor2015). This might even be seen as the most parsimonious explanation, and depending on the species concept one chooses to adopt (e.g., Polly, Reference Polly1997; Hunt and Rabosky, Reference Hunt and Rabosky2014; Allmon, Reference Allmon, Allmon and Yacobucci2016; Haug and Haug, Reference Haug and Haug2017), B. parvidens n. sp. would be a late-occurring form of B. pigotti.
Such interpretations—that fossils in stratigraphic sequence reflect discontinuous sampling of a continuous lineage—may no longer be tenable. In part, this arises from the ongoing discovery of cryptic species complexes among crocodylians. Because geographic ranges of species within these complexes, including Neoafrican Crocodylus, are known to have shifted during historical times (Hekkala et al., Reference Hekkala, Shirley, Amato, Austin, Charter, Thorbjarnarson, Vliet, Houck, Desalle and Blum2011), we cannot assume that the crocodiles found in a particular depositional sequence represent an indigenous lineage (Brochu and Sumrall, Reference Brochu and Sumrall2020). Brochuchus parvidens n. sp. and B. pigotti may indeed be end members of a continuum, but other explanations cannot be discounted.
However one wishes to perceive the underlying evolutionary pattern, this study extends the range of Brochuchus into the middle Miocene. Among generalized crocodylids, neither Crocodylus nor anything unambiguously more closely related to it than to Osteolaemus has been found prior to the late Miocene in the region. This is consistent with previous suggestions that endemic crocodylid faunas were replaced by Crocodylus at some point during the Miocene (Brochu, Reference Brochu2007; Brochu and Storrs, Reference Brochu and Storrs2012).
But the details have grown murky. Based on our phylogenetic analysis, a division between osteolaemines, Mecistops, and Crocodylus among late Cenozoic African crocodylids (e.g., Brochu, Reference Brochu2007) may be an oversimplification. Two distinct groups of erstwhile osteolaemines can be recognized—one including Osteolaemus, another including Euthecodon—but whether these are closely related is ambiguous. We believe the characters supporting osteolaemine affinities for euthecodontins are more compelling, but without additional character evidence, we cannot reject equally parsimonious alternatives. This, along with conflicting phylogenetic hypotheses for Mecistops, makes biogeographic assessment difficult.
Nevertheless, there appears to be a fundamental difference in crocodylid faunas between the last known appearance of Brochuchus at 15 Ma and the first known appearance of Crocodylus at ca. 7 Ma. This interval in the EARS preserves profound climatic and tectonic change. A period of increased basaltic volcanism began ca. 12 Ma in the eastern branch of the EARS (Rooney, Reference Rooney2020). Continuous forest cover began to give way to open grasslands and savannahs at this time, largely in response to drier conditions (Jacobs et al., Reference Jacobs, Pan, Scotese, Werdelin and Sanders2010; Feibel, Reference Feibel2011; Feakins et al., Reference Feakins, Levin, Liddy, Sieracki, Eglinton and Bonnefille2013; Wichura et al., Reference Wichura, Jacobs, Lin, Polcyn, Manthi, Winkler, Strecker and Clemens2015; Linder, Reference Linder2017). Unlike Crocodylus, modern Osteolaemus is found only in forested wetlands (Eaton, Reference Eaton, Manolis and Stevenson2010). If these ecological preferences apply to putative extinct relatives of Osteolaemus, the change from osteolaemine- or euthecodontin-dominated to Crocodylus-dominated crocodylid faunas in the EARS may reflect changes in vegetation cover. These changes are often thought to have played a role in human origins (e.g., Bonnefille, Reference Bonnefille2010; Marean et al., Reference Marean, Anderson, Bar-Matthews, Braun, Cawthra, Cowling, Engelbrecht, Esler, Fisher, Franklin, Hill, Janssen, Potts and Zahn2015). If so, then evolutionary pathways followed by early humans and crocodylids may have been linked to similar causal factors.
Such conclusions are preliminary. Further testing requires improved sampling in three areas. First, additional crocodylid material from the early and middle Miocene of the EARS must be described. Brochuchus is the only generalized crocodylid currently known from this interval, but it was a comparatively small animal, and larger generalized crocodylids may have occurred in the region at the time. Second, discovery and description of crocodylids between 7 and 15 Ma is needed to more precisely document the timing and manner of Miocene crocodylid replacement. Third, and most importantly, additional morphological characters expressing variation among late Cenozoic African crocodylids are needed to clarify the phylogeny of this assemblage.
Acknowledgments
The preparation and collections staff at the National Museums of Kenya in Nairobi are acknowledged for superlative assistance and friendship. L. Steel, S. Maidment, D. Schwarz, and N. den Ouden are thanked for collections access. Interactions with K. McNulty, C. Ward, J.M. Plavcan, T. Lehmann, A. Grossman, E. Hekkala, C. Liutkus-Pierce, J. Conrad, and M. Leakey were especially helpful in better understanding regional stratigraphy, paleoenvironments, and phylogenetic issues. Comments by two anonymous reviewers and the editor improved this contribution. This work was supported by NSF DEB 1257786 (to CAB) and the Leakey Foundation (to CAB and SKD).
Accessibility of supplemental data
Data available from the Dryad Digital Repository: https://doi.org/10.5061/dryad.15dv41nvf.