Introduction
Antiarch placoderm fishes are probably the most abundant middle Paleozoic vertebrates, known from almost all paleozoogeographic provinces, from the Silurian and Devonian periods (Lebedev and Zakharenko, Reference Lebedev and Zakharenko2010; Young, Reference Young2010). Many Devonian localities have yielded completely preserved skeletons. The morphology of the dermal skull and thoracic armor in these placoderms is well known (for example, Stensiö, Reference Stensiö1948; Hemmings, Reference Hemmings1978; Young, Reference Young1984; Johanson, Reference Johanson1997, Reference Johanson1998; Lukševičs, Reference Lukševičs2001; Béchard et al., Reference Béchard, Arsenault, Cloutier and Kerr2014). However, endoskeletal parts of their skeletons ossified extremely rarely and are usually not preserved (as an exception, Bothriolepis sp. [Young, Reference Young1984]). Dermal gnathal elements are sometimes found in assemblages with other skeletal parts, often being isolated from the skulls. A hypothesis to explain this separation was put forward by Upeniece (Reference Upeniece2011), who suggested that the gnathal elements became lost after death, being blown away by decay gases.
Despite numerous morphological descriptions of the dermal upper (suborbitals) and lower (infragnathals) jaw elements, in the Asterolepidoidei (Asterolepis: Pander, Reference Pander1857; Traquair, Reference Traquair1894; Stensiö, Reference Stensiö1931; Nilsson, Reference Nilsson1941; Lyarskaya, Reference Lyarskaya1981; Upeniece, Reference Upeniece2011; Pterichthyodes: Pander, Reference Pander1857; Hemmings, Reference Hemmings1978; Remigolepis: Nilsson, Reference Nilsson1941; Johanson, Reference Johanson1995, Reference Johanson1997), Bothriolepidoidei (Bothriolepis: Whiteaves, Reference Whiteaves1887; Woodward, Reference Woodward1892; Patten, Reference Patten1904; Stensiö, Reference Stensiö1931, Reference Stensiö1948, Reference Stensiö and Piveteau1969; Gross, Reference Gross1933, and Gross in Stensiö, Reference Stensiö1948; Young, Reference Young1984, Reference Young2008; Johanson, Reference Johanson1998; Moloshnikov, Reference Moloshnikov2008; Rücklin et al., Reference Rücklin, Donoghue, Johanson, Trinajstic, Marone and Stampanoni2012; Grossilepis: Gross, Reference Gross1941; Stensiö, Reference Stensiö1948; Nawagiaspis: Young, Reference Young1990; Livnolepis: Moloshnikov, Reference Moloshnikov2004, Reference Moloshnikov2008), and Yunnanolepidoidei (Phymolepis: Young and Zhang, Reference Young and Zhang1996; Parayunnanolepis: Wang and Zhu, Reference Wang and Zhu2021), the position of the gnathals with respect to the headshield during life is more or less uncertain. Because of this, questions regarding what food items were consumed, feeding mode, and the role of these fishes in the trophic webs of the Siluro-Devonian vertebrate communities remain unresolved.
Here we present a morphofunctional interpretation of the antiarch jaw apparatus exemplified by a better-known member of the Bothriolepidoidei, Bothriolepis sp. from the Upper Devonian of Australia (Young, Reference Young1984), with an attempt to reconstruct its jaw biomechanics and possible function. These widely distributed fishes played an important role in Siluro-Devonian trophic communities, and reconstruction of the antiarch jaw apparatus and its function is crucial for understanding antiarch feeding. This role, and antiarchs’ ecological relationships with respect to the earliest primary consumers, the agnathans, will be discussed.
Materials and methods
The specimens described in the following are infragnathal (lower jaw) bones assigned by Moloshnikov (Reference Moloshnikov2004, Reference Moloshnikov2008) to Livnolepis zadonica (Obrucheva, Reference Obrucheva1983) (specimen PIN 3725/1118) and to Bothriolepis sp. indet. (specimen PIN 3725/1119). Both specimens come from the same locality, a limestone factory quarry by Gornostayevka village, Livny District, Orel Region, Central Russia, 52°23′30.2″N, 37°33′16.8″E (Upper Devonian, lower Famennian, Zadonskian Regional Stage). The Zadonskian Regional Stage correlates to ?Upper triangularis–crepida interval of the Standard Conodont Zonation (Sobolev and Evdokimova, Reference Sobolev and Evdokimova2008).
These infragnathals were CT scanned at the All-Russian Research Geological Oil Institute (VNIGNI) using the ProCon X-Ray CT-compact system. The following scan parameters were used for PIN 3725/1118: AxialCT = 3,840 angles, voxel size = 19.771 μm, exposure time = 0.65 sec, averages = 9, voltage = 90 kV, current = 210 μa, focal spot mode = high resolution, bit depth = 16, detector resolution = 1888:1504, detector pixel size = 127 μm, binning mode = 1×1 12.5 fps high sensitivity; for PIN 3725/1119: AxialCT = 3,840 angles, voxel size = 9.224 μm, exposure time = 1.43 sec, averages = 7, voltage = 90 kV, current = 97 μa, focal spot mode = high resolution, bit depth = 16, detector resolution = 1888:1504, detector pixel size = 127 μm, binning mode = 1×1 12.5 fps high sensitivity. Scans were reconstructed by means of CERA and segmented using VolumeGraphics software, with movies made using VolumeGraphics and Movavi Video Suite software. Before macrophotography, specimens were dusted with ammonium chloride.
Antiarch jaw kinematics were reconstructed on the basis of the best-preserved Bothriolepis sp. specimen CPC25205 illustrated by Young (Reference Young1984). Apart from the dermal jaw bones (suborbital and infragnathal), this specimen also demonstrates the endoskeletal palatoquadrate and Meckelian cartilages. An enlarged jaw model (approximately 5×) was created of acrylic plaster, aluminum plates, and plasticine based mainly on the graphic reconstructions presented by Young (Reference Young1984) but including some corrections regarding the lower jaw and jaw articulation made according to Young's photos of the original specimen CPC25205.
Repository and institutional abbreviation
Figured specimens examined in this study are deposited in the A.A. Borissiak Palaeontological Institute of the Russian Academy of Sciences, Moscow, Russia, in the collection PIN 3725.
Results
Terminology
Inadequate knowledge of the morphology of the antiarch mouth elements and differing ideas as to their life orientation have led to inconsistency and ambiguity in terminology used in descriptions. The upper jaw suborbital and the lower jaw infragnathal bones are dermal derivatives of the mandibular visceral arch (Stensiö, Reference Stensiö1948; Jarvik, Reference Jarvik1980). During the early evolution of the group, the skulls in these fishes became strongly transformed relative to other placoderms. The oral fenestra became displaced, from its hypothetically ancestral terminal position, ventrally and oriented transversely. These changes primarily affected the topographic position of the suborbitals, which reversed topographically. For this reason, we avoid using previously accepted terms “ventral” and “dorsal” (for example, Hemmings, Reference Hemmings1978). The new transverse orientation of the mouth positions the morphologically posterior corner of the suborbital to a more lateral orientation.
Despite these perturbations, morphological interrelationships of the gnathal bones remained unchanged. To refine morphological clarity and consistency, we suggest a terminological scheme presented in Figure 1. However, the morphologically inaccurate term “lateral” widely used in antiarch descriptions, especially with respect to the terms “lateral notch” and “lateral processes” of the suborbital, are well-established terms in the literature and worth preserving. The term “mesial” is used with respect to the direction toward the symphysis, and “distal” toward the mandibular joint. The term “occlusal” is retained to refer to the cutting edge and cutting portion of the jaws (also termed “oral edge” and “oral division,” respectively) but does not imply actual occlusion between the upper and lower jaws.

Figure 1. Terminology used here for the suborbital and infragnathal bones of antiarchs, schematic sketches drawn after Bothriolepis sp. specimens from Gogo, Australia. (1) External view of the suborbital, orientation with the oral margin rostrally. (2, 3) Right infragnathal: (2) oral view; (3) labial view. (1, 3) Redrawn from Young (Reference Young1984). (2) Redrawn from Rücklin et al. (Reference Rücklin, Donoghue, Johanson, Trinajstic, Marone and Stampanoni2012).
Yunnanolepidoidei
The earliest examples of the antiarch jaw elements are presented in the early Devonian members of the Yunnanolepidoidei, Parayunnanolepis xitunensis Zhang, Wang, and Wang, Reference Zhang, Wang and Wang2001 (Wang and Zhu, Reference Wang and Zhu2021) and Phymolepis cuifenshanensis Zhang, Reference Zhang1978 (Young and Zhang, Reference Young and Zhang1996). In the former (Fig. 2.1), the suborbitals are much narrower than in the latter, leaving a large triangular open space. The suborbital antimeres contacted in life, but this contact seems to be quite mobile, considering their postmortem displacement. A weakly developed rostromesial projection is thought to serve as attachment for the ligamentous or cartilaginous connection between the antimeres. In Phymolepis, the large suborbitals, twice as long as deep, occupy almost the whole oral fenestra. These left–right antimeres tightly contact each other in the midline (symphyseally) or are even fused (Fig. 2.2). In both genera, the dermal ornamentation covers the whole external surface of the suborbitals, showing no oral shelf, and the oral margin is slightly inflected inward. The lateral notch is not expressed in either of these species.
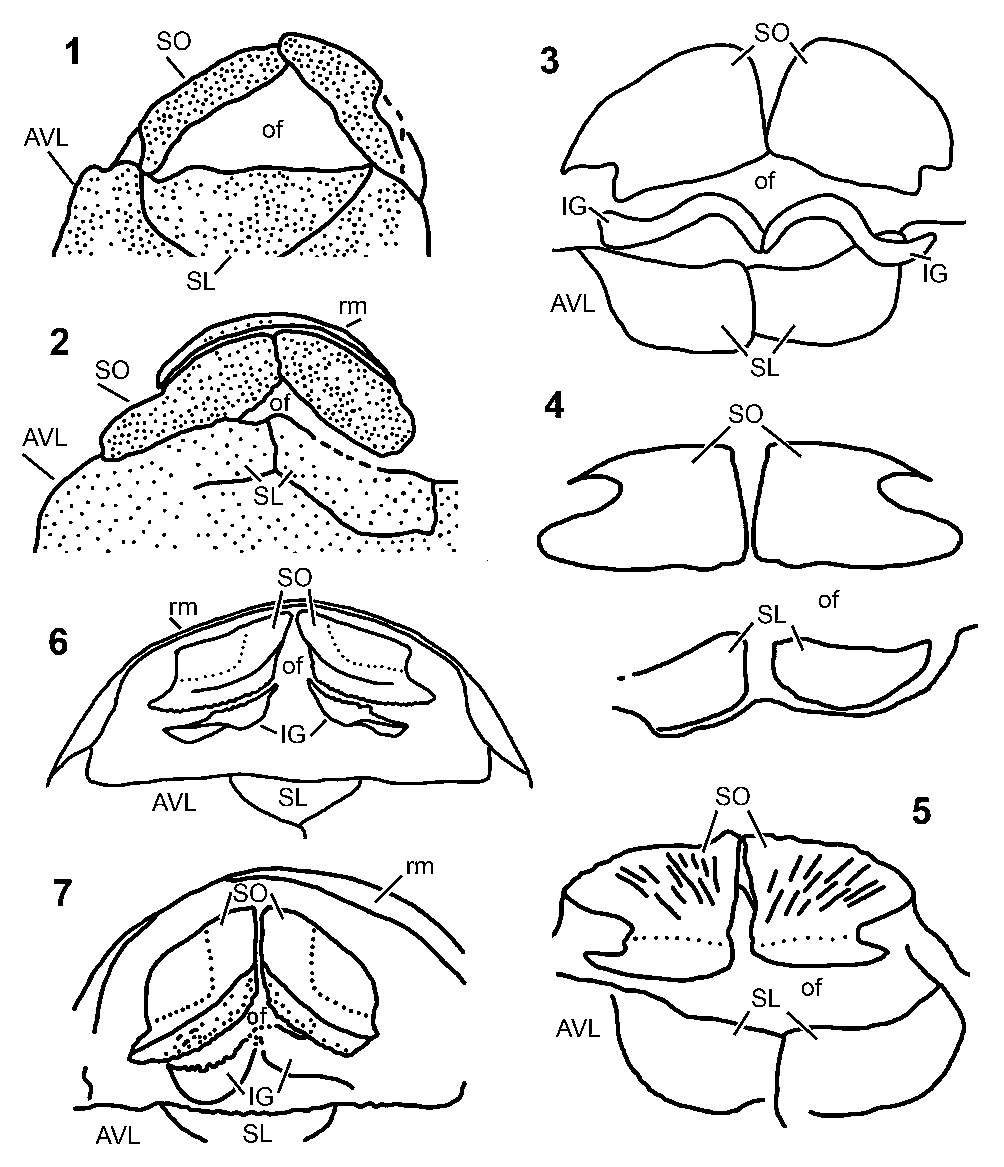
Figure 2. Oral fenestrae and jaw elements in antiarchs: (1, 2) Yunnanolepidoidei; (3–5) Asterolepidoidei; (6, 7) Bothriolepidoidei. (1) Parayunnanolepis xitunensis Zhang, Wang, and Wang, Reference Zhang, Wang and Wang2001. (2) Phymolepis cuifenshanensis Zhang, Reference Zhang1978. (3) Pterichthyodes milleri (Miller, Reference Miller1841). (4) Asterolepis scabra (Woodward, Reference Woodward1891). (5) Asterolepis ornata Eichwald, Reference Eichwald1840. (6) Bothriolepis canadensis (Whiteaves, Reference Whiteaves1880). (7) Bothriolepis sp. from Gogo. All figures are redrawn from the original reconstructions and photographs: (1) from Wang and Zhu (Reference Wang and Zhu2021); (2) from Young and Zhang (Reference Young and Zhang1996); (3) from Hemmings (Reference Hemmings1978); (4) from Nilsson (Reference Nilsson1941); (5) from Upeniece (Reference Upeniece2011); (6) from Stensiø (Reference Stensiö1948); (7) from Young (Reference Young1984). In (4), the right suborbital is mirrored from the left one for better comparison with other figures. AVL = anterior ventrolateral; IG = infragnathal; SL = semilunar; SO = suborbital; of = oral fenestra; rm = rostral margin of the skull roof. Not to scale.
Asterolepidoidei
In Pterichthyodes milleri (Miller, Reference Miller1841), the midline contact between the opposing suborbitals is fairly straight (Figs. 2.3, 3.1), and the antimeres fit together without forming an oral or aboral notch (Hemmings, Reference Hemmings1978). The posterior ridges supporting the palatoquadrate on the visceral side of the suborbitals are well marked, but the oval pit is poorly developed. The occlusal margin is mostly smooth; in some specimens it bears small crenulations. Hemmings (Reference Hemmings1978) claimed that the occlusal margin of the infragnathals appeared to have a large tooth-like structure just behind the rostromesial process of the oral division like that in Bothriolepis canadensis (Stensiö, Reference Stensiö1931, p. 64), but did not illustrate it. The distal division of the infragnathals is thin and shorter than the oral division.
Lyarskaya (Reference Lyarskaya1981) described the visceral elements in Asterolepis ornata Eichwald, Reference Eichwald1840 from the upper Givetian of Latvia. Unfortunately, the photos are not clear enough and the specimen drawings are too generalized, as is the reconstruction of the oral elements of the visceral arches, all requiring reexamination (E. Lukševičs, personal communication, 2021). The shape of the suborbital plate of the adult individual illustrated by Lyarskaya (Reference Lyarskaya1981, fig. 73) does not correspond to the material (Upeniece, Reference Upeniece2011).
Upeniece (Reference Upeniece2011) briefly described the suborbital bones of juvenile individuals of Asterolepis ornata. The shape of the bone in juveniles in general is similar to that in the adults. The aboral margins of these bones are convex, and the oral is straight (Figs. 2.5, 3.2). The posterolateral process is only slightly longer than the orolateral one, and the lateral notch is deep. The external surface in juveniles bears a fine-meshed and reticulate ornament; in adults the surface is stripped, characteristic of the dermal bones. Only one adult specimen (LDM 260/1a) shows infragnathal plates, but it is still undescribed.
Nilsson (Reference Nilsson1941) redescribed the internal mold of the suborbital plate in Asterolepis scabra (Woodward, Reference Woodward1891) originally described by Stensiö (Reference Stensiö1931) (Figs. 2.4, 3.3). The midline margin is straight, suggesting close fusion between the antimeres; the posterior (aboral) margin presumably contacting the rostral margin of the skull roof is convex. For comparison, Nilsson (Reference Nilsson1941) described homologous elements from the Upper Devonian of East Greenland ascribed to Remigolepis sp. In this specimen (Fig. 3.4), the lateral notch is strongly expressed but is much shallower than that in Asterolepis scabra. In contrast to the former, in the latter the left and right antimeres are firmly sutured.
Johanson (Reference Johanson1997) provided a thorough description of numerous individual skeletons of Remigolepis walkeri Johanson, Reference Johanson1997 from the Mandagery Sandstone (late Devonian) near Canowindra, NSW, Australia. In contrast to other antiarchs, in R. walkeri (Fig. 3.5) the suborbitals are separated caudosymphyseally by a deep V-shaped notch, a feature otherwise observed, although to a much smaller extent, only in Pterichthyodes milleri. The lateral processes are almost equal in size, in contrast to other antiarch taxa, where one of them is normally smaller. The lateral notch is expressed to various degrees. The visceral surfaces demonstrate oval areas and ridges interpreted as structures marking the attachment of the palatoquadrate. Configuration of these structures suggests that mandibular adductor muscles would have attached in the suborbital area below the metapterygoid region of the palatoquadrate. This restricted area suggested smaller muscle size and, consequently, a reduced biting force in R. walkeri with respect to Bothriolepis. The infragnathals are sinuous, slender, and similar in structure to homologous elements in other antiarchs, with a smooth blade of the oral division mesially (Fig. 4.3). These variations in the structure of gnathal elements are interpreted as being due to a different mode of life and feeding habits compared with other antiarchs.
Bothriolepidoidei
Stensiö (Reference Stensiö1931, Reference Stensiö1948, Reference Stensiö and Piveteau1969) studied a vast collection of Bothriolepis canadensis (Whiteaves, Reference Whiteaves1880) specimens from the Frasnian deposits of Miguasha (Quebec, Canada). Abundance of material, perfect preservation, and careful preparation resulted in the unearthing of several specimens that demonstrated mostly intact or only somewhat damaged elements of the oral apparatus, including well-preserved suborbitals (termed the “mentals” by Stensiö and his early successors), the infragnathals, and several dermal elements of unclear function: the infraprelaterals, extramandibulars, submandibulars, and subhyoids. All these were claimed by Stensiö (Reference Stensiö1948) to be located near their natural life position and are included in his reconstruction of the jaw apparatus (Fig. 2.6). No doubt, all these elements participated in the formation of the apparatus, but the fossil record of these elements in other antiarchs is almost completely missing, hindering comparison.
The suborbitals in Bothriolepis canadensis are almost separated in the midline by the oral notch thought to house the mesial tips of the infragnathals (Stensiö, Reference Stensiö1948). The lateral notch is shallow; thus, the posterolateral process is not expressed (Fig. 3.8). The oral margin bears a row of bony crenulations. Considering the absence of dermal ornament over the oral shelf and its inset position with respect to the rest of the bone, Stensiö (Reference Stensiö1948) suggested that this shelf was covered in life by a thick layer of soft tissue continuing laterally to form the labial fold. The visceral side of the suborbital bone orally bears a ridge for the contact to the palatoquadrate. The morphology of the infragnathal was described extensively earlier by Stensiö (Reference Stensiö1931). In B. canadensis there is a sharp separation of the oral division from the distal nonbiting division by means of a pronounced distal flange of the former (Fig. 4.9, 4.10). The distal end of the vertical blade clearly defines the posterolateral margins of the oral cavity.

Figure 3. Suborbital plates in various antiarchs, redrawn from the original illustrations and photographs. If only one antimere is known, it is mirrored to demonstrate the shape of the upper oral margin. (1–5) Asterolepidoidei: (1) Pterichthyodes milleri (Miller, Reference Miller1841); (2) Asterolepis ornata Eichwald, Reference Eichwald1840; (3) Asterolepis scabra (Woodward, Reference Woodward1891); (4) Remigolepis sp.; (5) Remigolepis walkeri Johanson, Reference Johanson1997. (6–12) Bothriolepidoidei: (6) Nawagiaspis wadeae Young, Reference Young1990; (7) Grossilepis tuberculata (Gross, Reference Gross1941); (8) Bothriolepis canadensis (Whiteaves, Reference Whiteaves1880); (9) Bothriolepis cellulosa (Pander in Keyserling, Reference Keyserling1846); (10) Bothriolepis sp. from Gogo; (11) Bothriolepis maxima Gross, Reference Gross1933; (12) Bothriolepis yeungae Johanson, Reference Johanson1998.
(1) From Hemmings (Reference Hemmings1978); (2) from Upeniece (Reference Upeniece2011); (3, 4) from Nilsson (Reference Nilsson1941); (5) from Johanson (Reference Johanson1997); (6) from Young (Reference Young1990); (7, 9) from Gross (Reference Gross1941); (8) from Stensiö (Reference Stensiö1948); (10) from Young (Reference Young1984); (11) from Gross (Reference Gross1933); (12) from Johanson (Reference Johanson1998). Not to scale.

Figure 4. Infragnathal elements in various antiarchs, redrawn from the original illustrations and photographs. (1, 2) Pterichthyodes milleri (Miller, Reference Miller1841). (3) Remigolepis walkeri Johanson, Reference Johanson1997. (4, 5) Nawagiaspis wadeae Young, Reference Young1990. (6–8) Grossilepis tuberculata (Gross, Reference Gross1941). (9, 10) Bothriolepis canadensis (Whiteaves, Reference Whiteaves1880). (11–13) Bothriolepis sp. from Gogo. (14–16) Bothriolepis sp. from Livny. (17–19) “Livnolepis zadonica” (Obrucheva, Reference Obrucheva1983). Not to scale.
The suborbital of Bothriolepis maxima Gross, Reference Gross1933 (Gross, Reference Gross1933, also Gross in Stensiö, Reference Stensiö1948) (Fig. 3.11) does not differ in morphology from that in Bothriolepis canadensis.
Gross (Reference Gross1941) described two isolated suborbital specimens thought to belong to Bothriolepis cellulosa (Pander in Keyserling, Reference Keyserling1846). Both show an oblique oral margin bearing a smooth shelf with a row of bony crenulations running along it (Fig. 3.9). The lateral notch is only slightly expressed. The mesial margin is directed at an acute angle to the aboral one, suggesting that a rather wide gap was present orally between the antimeres as in B. canadensis (Stensiö, Reference Stensiö1931, Reference Stensiö1948, Reference Stensiö and Piveteau1969). The structure of the suborbital in Grossilepis tuberculata (Gross, Reference Gross1941) is similar in general features to that in Bothriolepis but differs in the absence of the lateral notch (Fig. 3.7). The single small infragnathal was assigned by Gross (Reference Gross1941) to Grossilepis tuberculata. However, his comment that this small specimen was a Bothriolepis cellulosa juvenile, known from the same locality, remained neglected by later authors (e.g., Stensiö, Reference Stensiö1948). This specimen is distinguished by a tubercular ornament on the external surface of its oral division (Fig. 4.6–4.8). Gross stressed that the massive and rough tubercles of the occlusal edge are the same as those of the dermal ornamentation and are different from crenulations set along the oral margin of the suborbital, and it is unlikely that these two types of tubercles could interact directly. The aboral side of the oral division shows a very deep furrow, which housed the mesial part of the Meckel's cartilage.
Young (Reference Young1984) described a uniquely preserved specimen of Bothriolepis sp. from the Frasnian-aged Gogo locality (Western Australia). Among other bones of the skull, this specimen demonstrates several visceral elements: the suborbitals, the infragnathals, and the infraprelaterals (Fig. 2.7). The first two are associated with partly mineralized palatoquadrates and Meckel's cartilage, respectively. In this individual, the lateral notch of the suborbital plate is pronounced, the orolateral process is elongated, and the posterolateral one is almost undefined (Figs. 2.7, 3.10). The oral margin shows a number of crenulations (“denticulation”) running along it and forms a V-shaped notch between the antimeres. The oral shelf is said to house “soft tissues forming an upper lip” (Young, Reference Young1984, p. 636). The palatoquadrate mostly adjoins the oral half of the suborbital; thin perichondral lining shows two connections to the braincase named by Young (Reference Young1984) as the orbital and the palatobasal; the anterolateral connection of the autopalatine part of the palatoquadrate is hypothetical. The condyle of the mandibular articulation projects beyond the margin of the suborbital. The occlusal margin of the oral division of the infragnathals bears a row of crenulations (Fig. 4.11–4.13). An important feature of the infragnathal not commented on by Young (Reference Young1984) but clearly seen in a photo is a high level of porosity of the oral division, especially well expressed on its lingual surface (Young, Reference Young1984, pl. 58, fig. 2). A slender mesial process of the Meckel's cartilage occupies a furrow running along the aboral side of the mesial division of the infragnathal; in the distal division this furrow flattens.
Instead of a simple lateral notch (Fig. 3.6), in Nawagiaspis wadeae Young, Reference Young1990 the lateral margin of the suborbital is irregularly embayed and shows overlapping surfaces (Young, Reference Young1990). The occlusal margin is convex in approximately the same manner as in other bothriolepidoids, while the ridge supporting the palatoquadrate is similar to that in Bothriolepis. The infragnathals differ from those in Bothriolepis in showing a shorter distal division, and the porosity is significantly less expressed (Fig. 4.4, 4.5). In contrast to Bothriolepis, no crenulations are observed on the occlusal margin.
Johanson (Reference Johanson1998) described the morphology of well-preserved, mostly complete skeletons known from natural casts of Bothriolepis yeungae Johanson, Reference Johanson1998 from the Upper Devonian Canowindra locality. Here, the suborbital imprints made it possible to see an unusually wide smooth oral shelf comprising about a third of the total plate depth and limited by a well-pronounced ridge (Fig. 3.12). The infragnathals are S-shaped and similar in structure to those known in other species of Bothriolepis.
In 2004, Moloshnikov provided a short description of an antiarch infragnathal from the Upper Devonian (lower Famennian) Livny (Gornostayevka) locality of Central Russia. This locality had provided numerous isolated skeletal parts of the antiarch placoderm Livnolepis zadonica (Obrucheva, Reference Obrucheva1983), thus Moloshnikov assigned the specimen PIN 3725/1118 to this taxon (Figs. 4.17–4.19, 5). Moloshnikov suggested that the unusually porous and grooved surface of the oral blade of the infragnathal was related to capping by a keratinous sheath in life, comparing it to the structure of the jaw bones in modern turtles. Later, Moloshnikov (Reference Moloshnikov2008) described more antiarch material from the same fossiliferous strata (Bothriolepis sosnensis Moloshnikov, Reference Moloshnikov2008, B. sp. indet., Bothriolepis cf. B. leptocheira Traquair, Reference Traquair1893, Rossolepis brodensis Moloshnikov, Reference Moloshnikov2008, and Remigolepis (?) sp.) and a new infragnathal element assigned by him to Bothriolepis sp. (PIN 3725/1119) (Figs. 4.17–4.19, 6). The number of antiarch taxa in this locality makes a secure assignment of isolated jaw bones to either species problematic; thus, we use his identifications but underline their doubtful taxonomic status. Apart from these briefly described infragnathals, one more element (PIN 2266/48) from the lower Frasnian of the Kursk Region in Central Russia was identified as belonging to Asterolepis sp. indet. However, its morphological identification is dubious because of poor preservation.
Description
As the original description provided by Moloshnikov (Reference Moloshnikov2004, Reference Moloshnikov2008) was too short, we here redescribe these infragnathal bones PIN 3725/1118 and PIN 3725/1119. Although somewhat incomplete and isolated, these two specimens provide a wealth of new morphological information on the structure of the lower jaw bones in antiarchs and provoke new functional and ecological interpretations.
The right infragnathal of “Livnolepis zadonica” (PIN 3725/1118, Figs. 5, 6, Supplementary video 1, 2) is mostly well preserved, except for the distalmost part of the distal division and the mesial extremity of the oral division. In its general features, the structure of this bone follows the pattern shared by other antiarchs.

Figure 5. Right infragnathal of “Livnolepis zadonica” (Obrucheva, Reference Obrucheva1983), specimen PIN 3725/1118, from the Zadonskian Regional Stage, lower Famennian, Upper Devonian, a limestone factory quarry by the Gornostayevka village, Livny District, Orel Region, Central Russia. Photographs and explanatory specimen drawings in the following projections: (1, 2) lingually; (3, 4) labially; (5) enlargement of the mesial portion of the vertical blade of the oral division, labially; (6, 7) orally; (8, 9) aborally. bod = blade of the oral division; bvn = blood vessel notches; dlf = distal lingual flange; mam = suggested insertion of the m. adductor mandibulae; mcg = groove occupied by the Meckel's cartilage; mqm? = suggested insertion of the m. quadratomandibularis; om = occlusal margin; vl = imprints of vascular loops. Scale bars = 10 mm.

Figure 6. Right infragnathal of “Livnolepis zadonica” (Obrucheva, Reference Obrucheva1983), specimen PIN 3725/1118, from the Zadonskian Regional Stage, lower Famennian, Upper Devonian, a limestone factory quarry by the Gornostayevka village, Livny District, Orel Region, Central Russia. Virtual sections in the oral–aboral plane: (1–3) in labial view; (4–6) in oral view.
Scale bar = 10 mm.
The blade of the oral division (bod, Fig. 5.1–5.5) is very deep, about half of its length, in contrast to Bothriolepis canadensis, B. sp. from Gogo, and Grossilepis tuberculata (Stensiö, Reference Stensiö1931, Reference Stensiö1948; Gross, Reference Gross1941; Young, Reference Young1984) in which the blade depth ranges from a third to a half its length (Fig. 4). The occlusal margin (om) is strongly arched and slightly deflected labially (Fig. 5.6, 5.7). The labial surface of the oral division is ornamented by plentiful rough, wide, branching vascular grooves, the bases of which are penetrated by numerous small vascular pores. The occlusal edge of the blade is subdivided by terminating vascular grooves directed normal to the occlusal margin into an irregular row of small, spaced projections of bone (Fig. 5.3–5.5). Blood vessels entered this vascular network at the sharp flanges embracing the Meckel's cartilage, their entries forming two or three notches at the margin of these flanges (bvn, Fig. 5.3–5.5, 5.8, 5.9). Distally, the blade of the oral division turns lingually across the mesial part of the distal division and reaches the lingual surface of the bone (Fig. 5.1, 5.2, 5.6, 5.7). The slightly concave labial surface of this part of the blade is much smoother and bears only small vascular foramina (Fig. 5.3, 5.4). The labial flange flanking the Meckel's cartilage groove is continued by a short oblique crest mesially delimiting a narrow rugose strip, most likely a muscle scar (presumably m. intermandibularis) running along the labial margin of the distal division (mim, Fig. 5.3, 5.4, 5.6, 5.7). The oral side of the distal division is more or less smooth, bearing only scarce small vascular foramina and grooves (Fig. 5.6, 5.7). A subdivision of this surface into the lateral and mesial parts by the continuation of the occlusal margin, as in Bothriolepis sp. from Gogo and Grossilepis tuberculata (Fig. 4, also compare Young, Reference Young1984), is lacking.
The lingual face of the mesial division is strongly porous only in its oral half; more aborally, the size and density of pores diminishes (Fig. 5.1, 5.2, 5.6, 5.7). The sharp margin of the lingual flange limiting the aboral surface is interrupted by a subvertical vascular groove situated close to the broken mesial part (bvn, Fig. 5.8, 5.9). The distoaboral corner of the lingual face of the oral blade at its junction with the distal division is occupied by a triangular muscle scar (presumably m. adductor mandibulae) with its right angle pointed orodistally (mam, Fig. 5.3–5.5, 5.8, 5.9).
In the oral division, the furrow that housed the symphyseal part of Meckel's cartilage is limited by deep and sharp flanges (mcg, Fig. 5.8, 5.9). Distally, this groove becomes almost interrupted from the lingual side by a massive swelling. From this point and more distally, the groove turns into a wide depression limited lingually by a sharp-edged flange (dlf, Fig. 5.8, 5.9) lacking in other antiarch infragnathals. On the labial side, the bottom of the depression curves gently orally forming a pronounced ridge facing labially. The aboral surface presents numerous imprints of vascular loops (vl) on the generally smooth surface of the cartilage–bone contact. In the distal division, the surfaces of the sharp-edged flange limiting the depression lingually and the labial margin are penetrated by numerous vascular foramina interconnected by vermiculating vascular grooves.
Internally, the trabecular bone composing the infragnathal is penetrated by numerous vascular cavities and canals (Fig. 6; Supplementary videos 2–4). Only a few of them attain the diameter of the superficially located vascular grooves; most of them are much smaller. Porosity is concentrated mostly in the central part of the bone. The external layer, especially covering the distal portion of the labial side of the vertical blade, is more compact (Fig. 6.3, 6.5, 6.6). Within the vertical blade, most of the canals are oriented vertically (i.e., in the oral–aboral direction), few larger ones branching from the superficial grooves rise subvertically and taper toward the occlusal margin. In the distal division, canals are concentrated predominantly in its center and are generally subparallel to the external surfaces (Fig. 6.2, 6.3, 6.5, 6.6).
Although more complete (only a small part of the mesial extremity of the oral division is broken off), the infragnathal of Bothriolepis sp. (PIN 3725/1119) is more weathered superficially (Fig. 7; Supplementary videos 5–8). Especially damaged is the mesial area of the labial face of the oral division.

Figure 7. Left infragnathal of Bothriolepis sp., specimen PIN 3725/1119, from the Zadonskian Regional Stage, lower Famennian, Upper Devonian, a limestone factory quarry by the Gornostayevka village, Livny District, Orel Region, Central Russia. Photographs and explanatory specimen drawings in the following projections: (1, 6) labially; (2, 7) mesiolabially; (3, 8) orally; (4, 9) lingually; (5, 10) aborally. bod = blade of the oral division; dlf = distal lingual flange; mam = suggested insertion of the m. adductor mandibulae; mqm? = suggested insertion of the m. quadratomandibularis; om = occlusal margin. Scale bar = 5 mm.
The maximum depth of the blade of the oral division, as in PIN 3725/1118, is about half of its length (bod, Fig. 7.1–7.4, 7.6–7.9). The length of the distal division is almost two times smaller than that of the oral. The occlusal margin (om) is strongly arched and deflected labially. In contrast to the specimen PIN 3725/1118, its continuation distally forms a sharp ridge running lingually to meet the lingual margin of the distal division. At the junction of the oral blade with the labial margin of the distal division, there is a small oblique crest similar in shape, position, and orientation to that in specimen PIN 3725/1118, also interpreted here as a possible muscle scar for insertion of m. intermandibularis (mim, Fig. 7.1, 7.2, 7.6, 7.7). The oral side of the distal division is convex in cross section and bears only small pores of the vascular canals.
The distal part of the blade of the oral division on the labial side is slightly concave and penetrated by numerous tiny vascular foramina. At its base, at the junction with the sharp lingual margin of the distal division, there is a small crest similar in orientation to that observed in PIN 3725/1118 and possibly delimiting the insertion of m. adductor mandibulae (mam, Fig. 7.4, 7.9). The generally concave lingual side of the oral blade becomes convex distally. In cross section, this surface is also convex due to the labial deflection of the occlusal margin. A better preserved mesiobasal area bears only small, slightly expressed imprints of the vascular network while the whole occlusal half of the blade is superficially eroded, making it possible to see short sections of internal vascular canals.
No furrow housing the Meckel's cartilage is expressed along the base of the oral division; instead, a well-developed distal lingual flange (dlf, Fig. 7.5, 7.10) and a sharp margin of the distal division meet at an acute angle approximately in the mid-length of the aboral surface of the oral division, forming a slit-like pocket. This pocket turns into a wide, shallow groove running distally along the labial margin. The remaining area of the aboral side of the distal division is smooth; its surface bears only small vascular foramina. The mesial part of the aboral surface is narrow and possibly rounded by abrasion.
The internal structure of this specimen differs slightly from that in the specimen PIN 3725/1118 (Fig. 8; Supplementary videos 6–8). The average diameter of internal cavities is smaller, but these occupy almost the whole of the bone, without compaction along the bone surface. In the oral blade, canals form a rather uniform network (Fig. 8.2, 8.5, 8.6). The distal division is spongy, and the cavities and canals are small (Fig. 8.3). Along the distal margin of this division, the vascular canals directed at an acute angle to the bone margin are arranged in a subparallel pattern and interconnect to each other forming a network. Several subparallel interconnecting canals oriented at an almost right angle to those previously described run along the distal margin (Fig. 8.6).
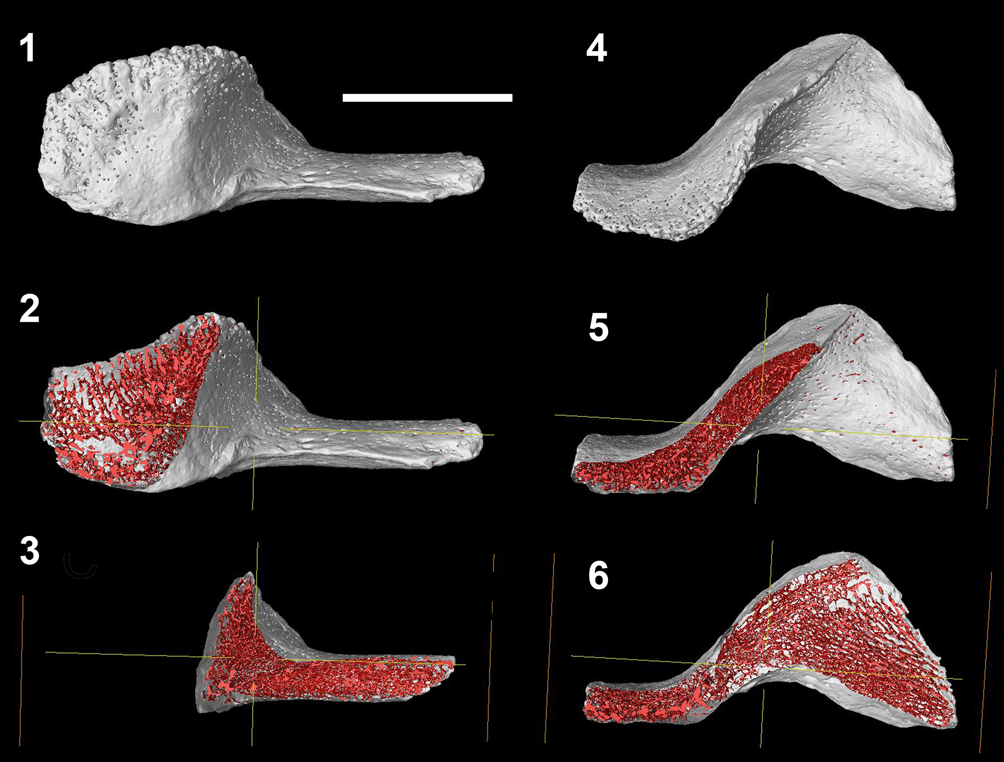
Figure 8. Left infragnathal of Bothriolepis sp., specimen PIN 3725/1119, from the Zadonskian Regional Stage, lower Famennian, Upper Devonian, a limestone factory quarry by the Gornostayevka village, Livny District, Orel Region, Central Russia. Virtual sections in the oral–aboral plane: (1–3) in the labial view; (4–6) in the oral view. Scale bar = 5 mm.
Discussion
Morphological variability of dermal elements of the mandibular arch in antiarchs
Although generally following the same bauplan, the jaw elements in antiarch fishes present a variety of morphologies (Figs. 2–4). The Yunnanolepidoidei differ from the Asterolepidoidei and the Bothriolepidoidei in the absence of the oral shelf and complete ornamentation of the external surface of the suborbitals, as well as the absence of the lateral notch. Symphyseal fusion between the suborbital antimeres is variable (Fig. 2.1, 2.2).
The characteristic feature of the Asterolepidoidei is a close (Asterolepis ornata, Upeniece, Reference Upeniece2011; Pterichthyodes milleri, Hemmings, Reference Hemmings1978; Remigolepis walkeri, Johanson, Reference Johanson1997) or even sutural contact between the suborbitals (A. scabra, Nilsson, Reference Nilsson1941; Remigolepis sp. from East Greenland, Nilsson, Reference Nilsson1941), suggesting the functional importance of this rigid connection (Figs. 2.3–2.5, 3.1–3.5). In contrast to yunnanolepidoids and in accordance with the bothriolepidoids, a significantly wide, smooth band devoid of dermal ornamentation runs along the oral margin of the suborbitals (Fig. 3.1, 3.2, 3.6–3.12) being unknown due to preservation in A. scabra, Remigolepis sp. from East Greenland (Nilsson, Reference Nilsson1941), and R. walkeri (Johanson, Reference Johanson1997). In Asterolepidoidei, the mesial notch on the oral side of the suborbitals is missing; thus, the oral margins of these bones are only slightly convex.
In the Bothriolepidoidei, the suborbitals are more or less lozenge shaped with the mesial (symphyseal) margin subparallel to the lateral one (Fig. 3.7–3.12), with the exception of Nawagiaspis (Fig. 3.6), in which the latter is directed at a right angle to the posterior (aboral) margin (Young, Reference Young1990). In this antiarch, the mesial part of the oral margin externally is occupied by a rugose area most probably for attachment of connective tissues serving to form an elastic connection of antimeres. In other bothriolepidoids, this area is ornamented superficially with regular dermal sculpturing suggesting placement of this connection on the visceral surface, if any. When connected, the oral margins of the suborbitals taken together would make a mesial notch, in contrast to the Yunnanolepidoidei and Asterolepidoidei, in which the oral margin is almost straight. In well-preserved and chemically prepared specimens, such as Bothriolepis sp. from Gogo (CPC 25205), the groove separating the oral shelf from the dermally ornamented aboral part demonstrates numerous vascular foramina of various sizes (Young, Reference Young1984, pl. 58, fig. 1, text-fig. 6), which suggests an active blood supply. In some species (B. canadensis, Stensiö, Reference Stensiö1948, text-figs. 35A, C; B. cellulose, Gross, Reference Gross1941, text-fig. 6A; Stensiö, Reference Stensiö1948, text-fig. 35D; Bothriolepis sp. from Gogo, Young, Reference Young1984, text-figs. 2, 3, 5, 6, pls. 58, figs. 1, 59, figs. 1, 2; Grossilepis tuberculate, Gross, Reference Gross1941, text-fig. 26G; Stensiö, Reference Stensiö1948, text-fig. 35F), this oral margin bears bony spikes arranged at an approximately regular distance from each other. This row of crenulations continues mesially, occupying approximately half of this margin.
Morphological variability of antiarch infragnathals can be grouped by the following features: (1) ratio between the length of the oral and distal divisions, (2) curvature of the oral division, (3) oral blade depth, (4) curvature of the labial margin of the distal division, and (5) porosity and superficial ornamentation of the oral division (Fig. 4).
The oral division of the infragnathal is morphologically marked by the posterior extremity of its oral blade. In Bothriolepis canadensis, the oral blade terminates distally, forming a deep semicircular flange (Stensiö, Reference Stensiö1948, text-fig. 36A).
The length of the oral division exceeds that of the distal in Pterichthyodes milleri (Fig. 4.1, 4.2), Grossilepis tuberculata (Fig. 4.6–4.8), and Nawagiaspis wadeae (Fig. 4.4, 4.5); in Bothriolepis canadensis (Fig. 4.9, 4.10), these parts are of approximately the same length. In other species, the oral division is shorter than the distal one. However, in Remigolepis walkeri (Fig. 4.3) and Bothriolepis sp. from Gogo (Fig. 4.11–4.13), the oral divisions are arched in such a manner that the lingual side is concave and the labial one is convex; this gives an impression of their shortness in the labial projection.
The curvature of the oral division might be roughly estimated by means of an angle between two planes, one tangential to the mesial and the second to the distal part of the vertical blade (lines in two dimensions). Geometrically, this angle is known as the central angle of an arch. In Pterichthyodes milleri and Bothriolepis sp. from Livny (PIN 3725/1119) and “Livnolepis zadonica” (PIN 3725/1118) these planes meet at an approximately right angle, in Remigolepis walkeri, Nawagiaspis wadeae, and Bothriolepis sp. from Gogo this angle is more acute, and in B. canadensis and Grossilepis tuberculata the angle is obtuse. An obtuse angle implies a smaller, and an acute angle a larger, curvature of the oral division.
Data on the vertical blade depth are available only when appropriate views have been provided in the descriptions. The largest depth exceeding that of the distal division is observed in Bothriolepis sp. from Livny (Fig. 4.14–4.16) and “Livnolepis zadonica” (Fig. 4.17–4.19), in which it is approximately twice as large as that of the distal division. In other available species, the vertical blade depth is much smaller and approximately equals or only slightly exceeds that of the distal division.
The labial margin of the distal division is curved to various degrees. In Pterichthyodes milleri, Bothriolepis canadensis, Bothriolepis sp. from Gogo, Bothriolepis sp. from Livny, and Grossilepis tuberculata it is almost straight or only slightly concave, in Remigolepis walkeri it is slightly sinusoid, while in Nawagiaspis wadeae it is very strongly convex. This character might reflect the difference in adductor muscle arrangement.
Another character is the degree of porosity of the vertical blade of the oral division and its superficial ornamentation. Unfortunately, in most specimens, data on the internal structure are unavailable, and all that is possible is visual evaluation of this feature from photos or drawings. In Bothriolepis canadensis the labial side of the vertical blade demonstrates feeble granulation or pitting, although this is not clearly seen in the drawing provided by Stensiö (Reference Stensiö1948, text-fig. 36A) and not commented on in the text, while in Grossilepis tuberculata this area is sculptured by clearly marked, rough tubercles (Gross, Reference Gross1941; Stensiö, Reference Stensiö1948) said to be indistinguishable from those of the ornamentation of the other dermal bones. The marginal tubercles of the occlusal margin are massive and blunt; unfortunately, data on their wear are missing and thus it is hard to judge whether they participated in food processing. In Bothriolepis sp. from Gogo, the lingual surface is strongly porous, except closer to the aboral margin; on the contrary, in Nawagiaspis wadeae, the occlusal part of the lingual side is almost smooth and the basal one is strongly porous (Young, Reference Young1990). Bothriolepis sp. from Livny demonstrates only slight porosity closer to the occlusal margin (Fig. 7.1, 7.2, 7.6, 7.7) while in “Livnolepis zadonica” the lingual side is slightly porous (Fig. 5.1, 5.2) while the labial one bears numerous rough, mostly vertically oriented branching canals opening to the surface of the bone predominantly in its mesial portion (Fig. 5.3–5.5).
The structure of the suborbital–infragnathal morphofunctional unit in antiarchs can be separated into four main groups based mostly on the morphology of the oral (occlusal) margins: (1) this least known group includes the Yunnanolepidoidei in which the oral margin of the suborbitals is devoid of the oral shelf, comparatively straight and incurved; (2) in the Asterolepidoidei, the deep suborbitals contact tightly in the midline or are even connected at a suture, presenting a straight or slightly convex oral margin; (3) in most of the Bothriolepidoidei, the oral margin is strongly convex and forms a mesial notch; on the contrary, the outline of the oral margin of the occlusal portion of the infragnathals is more or less straight or concave; (4) in the fourth group, based on two infragnathals from Central Russia described previously, the oral margin of this bone is strongly convex rather than concave due to its increase in depth toward the middle of the blade.
Thus, the infragnathals in antiarchs demonstrate a wide spectrum of characters related to their configuration and proportions, such as the ratio between the length of the oral and distal divisions, curvature of the oral margin, vertical blade depth, and curvature of the labial margin of the distal division. These differences may reflect the shape and size of the mouth cavity and might be closely related to the general skull outline. Deep skulls in Livnolepis, as described by Moloshnikov (Reference Moloshnikov2004, Reference Moloshnikov2008), might be correlated with the increased depth of the blade of the oral division. Superficial ornamentation as exemplified by Grossilepis tuberculata infragnathals suggests an external position and superficial coating by only a thin epidermal cover (Gross, Reference Gross1941). It is not impossible that in yunnanolepidoids there was no keratinized sheath at all as may be deduced from the absence of the oral shelf on their suborbitals. Sutured suborbitals in asterolepidoids suggest different jaw kinematics from that in bothriolepidoids, but currently there are insufficient data on these groups to perform kinematic studies.
Presence of a keratinous sheath on the gnathal elements in antiarchs
In all studied specimens, the oral surfaces of the gnathal elements are more or less porous. In those Bothriolepis species in which the external surface of the suborbital is adequately known (B. canadensis, B. cellulosa, B. maxima, and B. sp. from Gogo), in Grossilepis tuberculata, and in Nawagiaspis wadeae, the groove along the oral shelf shows variously expressed porosity reflecting an extensive lifetime blood supply. Superficially located vascular foramina and grooves opening on the oral surfaces of the infragnathals and reflecting trabecular spongy inner structure are especially well expressed in Bothriolepis sp. from Gogo (Young, Reference Young1984, pl. 58, figs. 2, 3), Nawagiaspis wadeae (Young, Reference Young1990, text-fig. 9C), Bothriolepis sp. from Livny (Fig. 7.1, 7.2; Supplementary videos 6–8), and especially in “Livnolepis zadonica” (Fig. 5.1, 5.3, 5.5; Supplementary videos 2–5). These foramina suggest the presence of an external cover during life protecting these superficially located blood vessels. This cover should be rigid to interact with food items and, at the same time, protect these vulnerable external vessels and bone surfaces. We suggest that the nature of this cover might have ranged from a comparatively thin layer of hard skin to even a keratinous cap forming the working jaw surfaces. Apart from protective function, a hard keratinoid substance might bear shearing, self-sharpening margins used for scraping.
To support this hypothesis, we considered recent animals. Intense superficial bone vascularization is characteristic of taxa (turtles and birds) in which teeth are missing and the jaw edges are furnished by keratin beaks known as rhamphothecas. For example, in the bill of a long-tailed duck Clangula hyemalis (Linnaeus, Reference Linnaeus1758) (Fig. 9.1–9.3, specimen PIN 41-7-5), the smallest blood vessels ending with capillaries on the ventral margin of the premaxilla are placed in superficial grooves separated by unaffected bony margin; in “Livnolepis zadonica” (Fig. 9.4), there is a strikingly similar structure of the labial side of the oral blade with branching superficial vascular grooves coming into canals at the base.

Figure 9. Vascularization of the oral margins in recent birds compared with antiarchs: (1–3) Clangula hyemalis (Linnaeus, Reference Linnaeus1758): (1) dorsal view of the rostral margin of the premaxilla; (2) ventral view of the rostral margin of the premaxilla; (3) an enlarged area of (2). (4) “Livnolepis zadonica” (Obrucheva, Reference Obrucheva1983); occlusal area of the vertical blade of the oral division of the infragnathal, labially. (1, 2) Scale bars = 2 mm; (3) scale bar = 1 mm; (4) scale bar = 3 mm.
Although keratinous structures are never preserved in the fossils except in Pleistocene mammals obtained from permafrost and asphalt sites (for example, Tridico et al., Reference Tridico, Rigby, Kirkbride, Haile and Bunce2014), presence of gnathic keratinization is widely accepted in the fossil dinosaurs considered the closest relatives of birds and in turtles (for example, Davit-Béal et al., Reference Davit-Béal, Tucker and Sire2009; Louchart and Viriot, Reference Louchart and Viriot2011). Among fossil lower vertebrates, presumed keratinous structures had been described in lampreys and hagfishes (Janvier, Reference Janvier2008). Moreover, genes for keratins are shared across extant vertebrates, including lampreys (e.g., Kimura and Nikaido, Reference Kimura and Nikaido2021), suggesting that these genes would also be expressed in antiarchs (stem gnathosomes; Zhu et al., Reference Zhu, Giles, Young, Hu, Bazzi, Ahlberg, Zhu and Lu2021), including in structures associated with the jaws.
Despite a widely held opinion that no keratin is present in fishes with the exception of horny “teeth” in the mouth sucker in the agnathan cyclostomes (Grassé, Reference Grassé1958), numerous members of the teleost fish family Cyprinidae, the specialized scrapers, possess a sharpened keratinous sheath for scraping off the periphyton (Lyovin, Reference Lyovin2007). This author also indicated that scraping in keratin-bearing Cyprinidae is often observed in those fishes in which skulls are shortened and mandibles are transverse or horseshoe shaped. This corresponds well to characteristics of the antiarch infragnathals described above. In the European periphytonophagous Chondrostoma nasus (Linnaeus, Reference Linnaeus1758), the lower jaw bears a keratinoid sheath (Vostradovsky, Reference Vostradovsky1973). Tripathi and Mittal (Reference Tripathi and Mittal2010) described horny sheaths covering jaws in a cypriniform scraper Puntius sophore (Hamilton, Reference Hamilton1822). Agrawal and Mittal (Reference Agrawal and Mittal1992) observed keratinized cone-like structure on the horny upper jaw sheath and on the horny lower jaw sheath associated with the lips of an herbivorous column feeder, Labeo rohita (Hamilton, Reference Hamilton1822). Pinky et al. (Reference Pinky, Mittal, Yashpal, Ojha and Mittal2004) reported the keratinization in lips and associated structures of Garra lamta (Hamilton, Reference Hamilton1822), and Qi et al. (Reference Qi, Chao, Guo, Zhao, Li, Wei and Zhao2012) in the members of the subfamily Schizothoracinae.
Beginning with Gross (Reference Gross1941), authors described bony crenulations on the occlusal margins of the antiarch suborbitals and infragnathals. Gross stressed that these “Zacken” (crenulations) are not denticles and are not composed of dentine (Gross, Reference Gross1941, p. 14). The groove on the external side of the suborbital (for example, in Young, Reference Young1984, pl. 58, fig. 1) might represent the base of this keratinous case housing its germinal cells. Young (Reference Young1984, text-fig. 6) also presented a photo of a perfectly preserved anterior edge of the semilunar bone forming the medial part of the anterior margin of the oral fenestra bearing tiny crenulations of the same size and distribution type as those on the oral margin of the suborbital. This might suggest the presence of a narrow keratinous cover protecting the posterior margin of the oral fenestra, as would be expected in a bottom-dwelling animal. In various turtles and tortoises, for example in Agrionemys horsfieldii (Gray, Reference Gray1844), very similar crenulations are found along the occlusal margin of the dentary (Fig. 10.1, 10.2). The same type of crenulation is observed on both jaw margins in the Arrau turtle Podocnemis expansa (Schweigger, Reference Schweigger1812) (Fig. 10.3). Formation of these spikes might result from concentration of bone tissue between capillaries supplying blood to subkeratinous dermis.

Figure 10. Crenulations along the jaw margins in modern tortoises. (1) Rostral part of the lower jaw of Agrionemys horsfieldii (Gray, Reference Gray1844), specimen PIN, unnumbered. (2) A scanning electron microscope photo showing an enlargement of bony spikes in (1). (3) The skull of Podocnemis expansa (Schweigger, Reference Schweigger1812), Morphosource-2021-08-18-025423. All figures in lateral view. Scratch marks on the bone surface in (2) are produced by dermestid larvae. (1) Scale bar = 2 mm; (2) scale bar = 1 mm; (3) scale bar = 3 mm.
Reconstruction of the jaw function
Uncertainty with respect to the position of the antiarch gnathal elements results from their bizarre structure and loose connection to the skull, as well as from postmortem displacement. The earliest author to describe the antiarch gnathal elements (Pander, Reference Pander1857) correctly identified these in Pterichthyodes as the mouth parts, but was uncertain which elements belonged to the upper and which to the lower jaws. Traquair (Reference Traquair1888), in his reconstruction of Asterolepis, placed the mouth opening between the rostral margin of the skull roof and jointed suborbital plates considered by him as the lower jaw. Woodward (Reference Woodward1892) was the first to suggest the connection of the suborbitals to the rostral margin of the skull roof by means of connective tissue and skin. The problem remaining is the natural orientation of the suborbitals in the positions “mouth closed” and “mouth opened.”
In fossils, the suborbitals are either found detached or occupy a position within the oral fenestra roughly in the same plane with the ventral part of the thoracic armor, ornamented surface facing ventrally, as exemplified by Phymolepis (Young and Zhang, Reference Young and Zhang1996), Parayunnanolepis (Wang and Zhu, Reference Wang and Zhu2021), Asterolepis ornata (Lyarskaya, Reference Lyarskaya1981; Upeniece, Reference Upeniece2011), Bothriolepis canadensis (Stensiö, Reference Stensiö1931, Reference Stensiö1948), and Bothriolepis sp. from Gogo (Young, Reference Young1984). In Stensiö's reconstruction of the Bothriolepis canadensis head (Stensiö, Reference Stensiö1948, text-figs. 4, 5), the plane of the oral fenestra is inclined anteroventrally with respect to the ventral surface of the trunk shield (Stensiö, Reference Stensiö1948, text-fig. 114), and thus the mouth position was subterminal. The position of the suborbital plates during life was demonstrated by Young and Zhang (Reference Young and Zhang1996) and Young (Reference Young2008); in their reconstructions, the ventral mouth opening position in Bothriolepis contrasts with that presented by Stensiö (Reference Stensiö1948). This anatomical correction is important in the revision of the hypotheses regarding the antiarch feeding mode.
In the yunnanolepidoid Phymolepis cuifenshanensis and in the asterolepidoids Pterichthyodes milleri and Remigolepis sp., the suborbital antimeres tightly contact or even fuse at the symphysis (Nilsson, Reference Nilsson1941; Hemmings, Reference Hemmings1978; Zhu, Reference Zhu1996; Figs. 2.2, 3.1, 3.4). This suggests their action as a single functional unit. In the Bothriolepidoidei, on the contrary (Fig. 2.6, 2.7), the adjoining suborbitals are separated in the midline (Young, Reference Young1984; Zhu, Reference Zhu1996) so that they could function separately, for example, to respond to the size and density of the food object differently.
Johanson (Reference Johanson1997, Reference Johanson1998) interpreted the mouth position in Bothriolepis and Remigolepis as strictly ventral; Béchard et al. (Reference Béchard, Arsenault, Cloutier and Kerr2014), using three-dimensional surface-scanning technology to reconstruct the armor of Bothriolepis canadensis, also clearly demonstrated that the oral fenestra faced strictly ventral.
In Phymolepis as well as in the Asterolepidoidei and the Bothriolepidoidei, the aboral (posterior) margin of the suborbital is always slightly arched (Bothriolepis canadensis, Stensiö, Reference Stensiö1948, text-fig. 35A and Nawagiaspis, Young, Reference Young1990, text-figs. 8A, 9A, B being exceptions). This bend fits the curvature of the rostral margin of the oral fenestra. This suggests that (1) in the natural “mouth closed” position, the suborbitals were situated in such a way that their external (labial) surface was oriented ventrally and in a plane with the ventral side of the trunk armor; and (2) the suborbitals were mobile and could rotate in the rostral direction during mouth opening. However, this rotation would have been limited by palatoquadrate articulations with the braincase, as well as by sensory canal, skin, and other soft tissues surrounding these bones forming no sutured connections to the adjoining ones.
Young (Reference Young1984) suggested that the position of the suborbitals in the Bothriolepis sp. specimen from Gogo was due to postmortem rotation. In the partly prepared state (Young, Reference Young1984, text-fig. 6), the suborbitals are somewhat displaced and depressed inside the cranium, but the infragnathals remain in proper position. This author also provided a reconstruction of the Bothriolepis head in lateral view; however, the upper and lower jaw elements are omitted there.
In a later paper (Young, Reference Young2008, text-fig. 3A), a new reconstruction modified the orientation of the mouth opening at the expense of a slight lifting of the snout and small posteroventral inclination of the suborbitals, thus giving more space for these within the oral fenestra. Considering the repeatedly observed placement of the suborbitals in the same plane with that of the ventral side of the trunk armor in the fossils, we suggest that this had been their natural “mouth closed” position.
Location of the mandibular joint was estimated as inside the prelateral plate, with the dorsal articular surface facing toward the lateral oval pit but some distance below it (Young, Reference Young1984). The palatoquadrate lies rostromesial to the articular process of the submarginal plate, the slope of its lingual surface in the quadrate region conforming fairly well to the slope of the articular process. Two points of articulation between the palatoquadrate and the braincase, the orbital and palatobasal connections, had been reconstructed in Bothriolepis sp. from Gogo (Young, Reference Young1984). Those provided an axis for a limited palatoquadrate + suborbital complex hinge rotation.
To overcome the difference in the transverse width of the longer suborbitals and shorter infragnathals, Young (Reference Young1984) had to assume the presence of a nonpreserved or at least nonossified (?calcified) cartilaginous basimandibular, an unpaired copula of the mandibular arch connecting the two Meckel's cartilage antimeres. If so, the mesial tips of the infragnathal oral divisions became widely separated. However, on the specimen (Young, Reference Young1984, text-fig. 6), the suborbitals and the infragnathals closely fit each other in the midline, with the mesial tips of the latter strongly bound inside the mouth, although this configuration might be explained by postmortem displacement. Wide separation of the mesial tips of the infragnathals is unlikely as in this case they would not fit between the suborbitals. To do so, any mesial connection should be very elastic to make possible rotation of each unit in the lingual–labial direction at the mouth closure/opening.
Creation of our jaw apparatus model was aimed at physical manipulation for defining the most parsimonious and noncontradictory interrelations of its parts and functioning of the mechanism on the whole during life (Supplementary Fig. 1). In our opinion, this “hardware modeling,” as compared with software modeling, allows us to make more definite conclusions as to what positions and movements of the jaws were possible and what were not. For example, our hardware model of acrylic plaster and plasticine has immediately allowed us to exclude any occlusal interactions between the antiarch upper and lower jaws, which were found to be incompatible with the integrity of the jaw articulation.
The jaw articulation reconstructed here might belong to a ball-and-socket type as no plausible opening and closing jaw movements could be achieved otherwise with the model created (Figs. 11, 12). The ball of the joint readily found its place on the oral side of the palatoquadrate part protruding distally (laterally) beyond the suborbital. Here, there is a gap in the mineralized lining of the palatoquadrate cartilage. There is a reciprocal glenoid fossa incompletely ossified as a gap in the mineralized perichondral lining on the distal end of the Meckel's cartilage. This gap is shaped as a wide notch seen from both the oral and aboral sides of the lower jaw (Fig. 12, gf). In our reconstruction, the mandibular joint socket occupies this notch completely. This more closely resembles the specimen's shape and is more parsimonious than Young's (Reference Young1984) original reconstruction with the jaw articulation on the oral side of the notch and articulation with hyoid on its aboral side (his “ventral articular area”). Neither epihyal (hyomandibula) nor ceratohyal is known in the specimen CPC25205 or any other antiarchs. Moreover, there are no biomechanical reasons to hypothesize the presence of a hyostylic jaw suspension. This seems to have been either absent or nonfunctional, for example, reduced to compliant ligaments that did not restrict or guide jaw motions.

Figure 11. Reconstruction of Bothriolepis (based on specimen CPC 25205 from Gogo) jaws and jaw muscles within the oral fenestra in ventral view: (1) “mouth closed” position; (2) “mouth opened” position. Gray: dermal bones (suborbital and infragnathal); hatched: reconstructed keratinous sheath. The right side adductor mandibulae muscle is not shown to expose the ball-and-socket jaw articulation. AVL = anterior ventral lateral bone of the trunk carapace; IG = infragnathal; MC = Meckel's cartilage; PQ = palatoquadrate; SL = semilunar bone of the trunk carapace; SO = suborbital; mam = m. adductor mandibulae; mcm = m. coracomandibularis; mim = m. intermandibularis; mlpre (mlpal) = m. levator palatoquadrati or m. levator prelabialis. Not to scale.

Figure 12. Schematic presentation of Bothriolepis (based on specimen CPC 25205 from Gogo) jaw apparatus and jaw muscles: (1) kinematic scheme of mouth opening, anterolateral view from the right side; (2) lingual view of the right mandible showing the adductor mandibulae muscle and the glenoid fossa. IG = infragnathal; MC = Meckel's cartilage; PQ = palatoquadrate; SO = suborbital; gf = glenoid fossa; ks = keratinous sheath; mam = m. adductor mandibulae; mcm = m. coracomandibularis; mim = m. intermandibularis; mlpre (mlpal) = m. levator palatoquadrati or m. levator prelabialis. Not to scale.
The typical archaic palatoquadrate connection to the braincase in gnathostomes includes two points, the orbital anteriorly and palatobasal posteriorly. In the antiarchs, the jaws are very short and placed on the ventral side of the head, while the nares and eyes are tightly packed on the dorsal side. As a result, the palatoquadrates are placed far ventrally from the ethmoid region of the braincase. To overcome this incongruency, Stensiö (Reference Stensiö and Piveteau1969) reconstructed a high orbital process on the palatoquadrate. Young (Reference Young1984) refuted this high orbital process and suggested, instead, the presence of short orbital and palatobasal connections, on the basis of two respective gaps in the mineralized perichondral lining on the lingual (inner) side of the palatoquadrate, one closer to its mesial end and the other to the jaw joint, level to the distal (lateral) edge of the suborbital. For it to preserve palatoquadrate connections in the absence of its high processes, Young (Reference Young1984) hypothesized a certain anteroventral extension of the braincase toward the palatoquadrates, beneath the anterior wall of the skull roof, and termed this extension the subnasal shelf. In fact, it corresponds to the rostrum (“rostral process of ethmoid region”) of Stensiö (Reference Stensiö and Piveteau1969), and the presumed articulations of the palatoquadrate to it cannot be treated as orbital or palatobasal but rather preorbital or even prenasal.
It is not impossible that the two gaps in palatoquadrate perichondral lining mark the insertion of two ligaments attaching the upper jaw directly to the visceral side of the anterior wall of the skull roof, at the rostral margin of the oral fenestra. Whatever was the connection, the kinematics of the upper jaw seem unequivocal. Available data suggest that the upper jaw antimeres might protract and retract in a manhole-cover manner. In the retracted position, the suborbital was placed in the oral fenestra plane; in the protracted position, it became depressed at a right angle to this plane. The rotation axes of the left and right antimeres of the upper jaw, determined by the double suspensions (connections) of the palatoquadrates, were oriented at an obtuse angle to each other. Therefore, each half was depressed anteriorly and slightly laterally. In those antiarch genera in which the suborbitals were fused in the symphysis, they might become protracted only together with each other along the strictly transversal common axis of rotation.
As to the lower jaw mobility, manipulations with the model have shown that mouth opening was performed by means of combined depression and inward rotation of each lower jaw antimere around its longitudinal axis (Fig. 12.1). Mouth closing was performed by combined adduction and outward rotation. Only the ball-and-socket type of jaw articulation could provide this combined mobility simultaneously involving two degrees of freedom. Furthermore, the antimeres of the lower jaw are located at an angle to each other. Although this angle is obtuse, rotation of the two halves around their longitudinal axes not aligned with each other requires a compliant symphyseal ligament. In fact, the jaws of Bothriolepis and a number of other antiarchs consisted of four mutually mobile quadrants.
Four muscles are reconstructed (Figs. 11, 12; Supplementary Fig. 1). The one responsible for the upper jaw retraction presumably originated from the visceral surface of the anterior wall of the skull roof or from the rostral part of the braincase and passed below the aboral edge of the suborbital to insert on the aboral side of the palatoquadrate between two points of its suspension. This muscle might be homologous either to the elasmobranch m. levator palatoquadrati (innervated with mandibular branch of trigeminal nerve, V3) or to the chimaeriform m. levator prelabialis (innervated with maxillary branch of trigeminal nerve, V2) (Figs. 11, 12.1, mlpre (mlpal)). In extant gnathostomes, the latter muscle is the last remnant of the preoral visceral striated musculature (Mallatt, Reference Mallatt1996).
The function of the second reconstructed muscle is lower jaw depression. It might have belonged to hypobranchial somatic musculature (with hypoglossal innervation) preserved in all gnathostomes. Following the shark nomenclature, this muscle should be termed the m. coracomandibularis. Presumably, the m. coracomandibularis originated from the visceral side of the ventral trunk shield and ran rostrally along the midline, in close association with the contralateral muscle, to insert on the aboral margin of the lower jaw close to the symphyseal end of the Meckelian cartilage (Figs. 11, 12.1, mcm). This position of the m. coracomandibularis implies that depression caused by it was restricted, limited by the rostral margin of the trunk carapace. The position of the m. coracomandibularis implies that it might depress the lower jaw no more than at right angles to the ventral plane of the trunk shield, the maximum possible range of mouth opening.
The third muscle is the m. adductor mandibulae (innervated with the mandibular branch of trigeminal nerve, V3) connecting the upper and the lower jaws and closing the mouth. Gross (Reference Gross1941) reconstructed this muscle in Grossilepis tuberculata as running directly from the upper to the lower jaw. However, with such a placement, the length difference of muscle fibers between the mouth-closed and mouth-opened positions would exceed the known properties of striated musculature, or otherwise, the mouth opening would be improbably restricted by the shortening range of the muscle fibers. Therefore, we reconstruct the m. adductor mandibulae as extending over the labial margin of the Meckel's cartilage to insert across its expanded aboral surface up to the scars found in specimens PIN 3725/1118 and PIN 3725/1119 on the lingual side of the infragnathal bone at the distal end of its curved oral division (Figs. 11, 12.2, mam). It might be hypothesized that the muscle was bipennate, with a single tendon of origin from which the deeper muscle fibers ran to the Meckel's cartilage, and the superficial muscle fibers ran to the superficial aponeurosis, which terminated on the aforementioned scars on the infragnathal. To avoid traumatizing the muscle itself by the distal part of the oral margin (presumably covered with a keratin blade) of the suborbital at mouth closure, we reconstruct the m. adductor mandibulae origin on the palatoquadrate in close proximity to the mandibular joint. Thus, the origin of the m. adductor mandibulae was closer to the jaw articulation than to the insertion. As a result, the muscle extended from the upper to the lower jaw obliquely, somewhat along the lower jaw. Consequently, contraction of this muscle pulled the lower jaw not only upward and forward, but also laterally. The shape of the jaw joint in the specimen CPC25205 supports this conclusion since the notch on the distal end of Meckel's cartilage is exactly what is required to counterbalance the lateral pull of the obliquely reconstructed m. adductor mandibulae.
The fourth muscle is the m. intermandibularis (innervated with the mandibular branch of trigeminal nerve, V3) preserved in all gnathostomes. It might have run transversely, connecting the aboral margins of the infragnathal antimeres (Figs. 11, 12.1, mim). This muscle is normally composed of two contralateral muscles meeting in the floor of the mouth and forming a tendinous interconnection along the midline. Most probably, this muscle attachment on the lower jaw was associated with the scars and notch found in specimens PIN 3725/1118 and PIN 3725/1119 on the labial side of the infragnathal bone at the distal end of its curved biting portion, as well as on the adjacent segment of the Meckel's cartilage. Thus, the m. intermandibularis attachment might have occupied an area between the insertion of the m. coracomandibularis and the belly of the m. adductor mandibulae wrapped around the distal expanded part of the Meckel's cartilage. The proposed function for the m. intermandibularis in antiarchs is the outward rotation of the mandibular antimeres during mouth closure. The muscle should have been strong enough as its presumed force of contraction should be concordant with the extensive development of the aforementioned scars especially developed in the Livnolepis specimen.
Thus, the m. adductor mandibulae is an antagonist for the other three jaw muscles. Its retracting of the upper jaw is opposed by protraction by the levator muscle, adducting the lower jaw—by depressing it by the coracomandibularis muscle, rotating the lower jaw inwards—by its outward rotation by the intermandibularis muscle. The last antagonism is visibly indicated by opposition of the respective m. adductor mandibulae and m. intermandibularis scars on the infragnathal. The m. adductor mandibulae should have been the largest muscle, followed by the m. intermandibularis and further by two subequal ones, the m. levator palatoquadrati (or m. levator prelabialis) and m. coracomandibularis.
With respect to feeding modes, the cartilaginous branchial arches themselves are not preserved; nevertheless, some consideration of the space in which these were held (and so size and potential complexity of the apparatus) can be made. In yunnanolepids, the anterior transverse crista, located on the visceral surface of the anterior ventral trunk shield, occurs along with an ornamented postbranchial lamina (e.g., Wang and Zhu, Reference Wang and Zhu2018). This ornamented lamina is located at the rostral margin of the trunk shield, marking the rear of the branchial cavity, comparable to the mesially directed and ornamented flange of the pectoral girdle in actinopterygians such as Acipenser (Jessen, Reference Jessen1972, fig. 5.2). In other antiarch groups, the postbranchial lamina has been lost, with the anterior transverse crista more posteriorly placed relative to the anterior margin of the trunk shield (e.g., Bothriolepis canadensis, Stensiö, Reference Stensiö1948, text-fig. 164A). This suggests additional space for the branchial arches, compared with the yunnanolepids. The submarginal is located at the lateral margin of the head shield and effectively forms the moveable bony plate covering the branchial region. With a larger branchial cavity (putatively longer branchial arches) and a moveable submarginal in the Asterolepidoidei and the Bothriolepidoidei, these antiarchs might have generated a significant water flow through the orobranchial chamber that made restricted suction feeding possible.
Despite Young's (Reference Young1984) opinion on the possibility of occlusion, manipulations with the reconstruction have shown that no occlusive interactions of the blades of the upper and the lower jaw were possible. The dermal bones of the jaws, capped by hard keratinous blades, might have acted like half-round wood chisels scraping the substrate as they moved toward each other but never met. In the lateral view, the protracted upper jaw is two times shallower than the depressed mandible. Therefore, if the fish attempted to scrape the horizontal aquatic beds, it should have inclined its body head first at approximately 45° (Fig. 13). The peculiar feature of jaw mobility found during manipulations with the model is a combination of the lower jaw adduction with the outward rotation of its antimeres powered by the m. intermandibularis. An opposite movement combining closure with inward rotation was recently suggested in Ferromirum oukherbouchi Frey et al., Reference Frey, Coates, Tietjen, Rücklin and Klug2020, a Late Devonian symmoriiform chondrichthyan (Frey et al., Reference Frey, Coates, Tietjen, Rücklin and Klug2020). Inward rotation of the lower jaw during a bite, like in Ferromirum, is apparently advantageous to pierce the prey. Outward rotation of the lower jaw during mouth closure, like in Bothriolepis, could help in maintaining an optimal cutting angle between the lower jaw half-round wood chisel and the substrate throughout mouth closure while scraping. This scraping action might have been enhanced by mutual outward–inward rotating motion of the curved infragnathal half-round wood chisel resulting from antagonistic interaction of the adductor mandibulae and intermandibularis muscles during mouth closure.

Figure 13. Whole-head reconstruction of Bothriolepis in feeding position in the right-side view with mouth open. IG = infragnathal; MC = Meckel's cartilage; PQ = palatoquadrate; SO = suborbital; mam = m. adductor mandibulae; mcm = m. coracomandibularis; mlpre (mlpal) = m. levator palatoquadrati or m. levator prelabialis. Not to scale.
The closest analogue for the feeding mechanism suggested here for antiarchs is found in the extant anuran tadpoles (e.g., de Jongh, Reference de Jongh1968; Bonacci et al., Reference Bonacci, Brunelli, Sperone and Tripepi2008; Venesky et al., Reference Venesky, Wassersug, Jorgensen, Riddle and Parris2011), despite their much smaller size. While scraping the substrate, they simultaneously suck in the scraped particles by uninterrupted breathing movements. Similarly, antiarchs would not cease to pump water through their mouth and pharynx by oscillatory movements of the submarginal bone. In anuran tadpoles, such a scraping mouth makes possible a wide range of derived applications as presented by Candioti (Reference Candioti2007). Similarly, antiarchs, by small morphological variations, could change the use of their jaw apparatus from picking algae to a wide range of feeding specializations, probably even carnivory as demonstrated by Occidozyga baluensis (Boulenger, Reference Boulenger1896) tadpoles (Haas et al., Reference Haas, Pohlmeyer, McLeod, Kleinteich, Hertwig, Das and Buchholz2014).
Feeding mode of antiarchs
In his Reference Stensiö1931 paper, Stensiö noted that nothing could be stated about the feeding mode of the antiarchs, although he and later authors focused on the morphofunctional interpretation of the shape of the antiarch trunk shield and pectoral fins, consistently interpreting these animals as bottom dwellers capable of fast occasional swimming, possibly escaping from predators.
Denison (Reference Denison1941, Reference Denison1961) described the alimentary tract in Bothriolepis canadensis noting its straightness and absence of a stomach but presence of a large spiral valve in the intestines. This structure had been reconstructed on the basis of its infilling; part of it is composed of fine muddy or silty rock usually present in the posterior part of the reconstructed gut. Frequent presence of carbonaceous material indicated that plant food formed an important part of the diet. Because of these data, Denison suggested that Bothriolepis was a mud-grubber, living much as does an earthworm on digestible material in the mud it swallows. As such, it was a bottom feeder, indicated independently by the ventral position of its mouth. Since this paper, this interpretation became canonical. Interpretation of Bothriolepis canadensis and other antiarchs as benthivorous, bottom-dwelling fishes was later supported by many authors (for example, Watson, Reference Watson1961; Moloshnikov, Reference Moloshnikov2008; Cloutier et al., Reference Cloutier, Proust and Tessier2011).
Johanson (Reference Johanson1998) suggested that “weak” ventral mouths of Bothriolepis and Remigolepis had been adapted for taking small food particles from the sediment surface, or even processing the sediment itself.
Upeniece (Reference Upeniece2001, Reference Upeniece2011) described distinctive concentrations of mineral matter different from the surrounding matrix within the anterior part of the trunk of juvenile Asterolepis ornata individuals seen in more than 100 specimens. A similar position of these concentrations in numerous specimens suggested that these structures might represent the lifetime position of the digestive tract. These infillings consist of coprolitic-like matter otherwise found separately outside the specimens as discrete coprolites in the same clay lens, being composed of quartz grains, carbonaceous matter, and detritic-like bone material. Upeniece (Reference Upeniece2001, Reference Upeniece2011) followed Denison's (Reference Denison1941) interpretation of this content as demonstrating a mud-grubbing mode of feeding in Asterolepis, similar to that in Bothriolepis. The main argument to support this was the observation that the presumable gut infillings in the trunk differed in lithology from that of the surrounding sediment but agreed with the content of isolated coprolites. However, if antiarchs consumed sediment as it was, the gut content would be indistinguishable in composition from the surrounding sediments. Differentiation of the sediment might be achieved only in the case that it was primarily sorted in the mouth, then smaller particles removed through the gill opening. Cloutier et al. (Reference Cloutier, Proust and Tessier2011) and Cloutier (Reference Cloutier2013) studied the gut contents of Bothriolepis canadensis and found the valves of the conchostracan Asmusia membranacea (Martens, Reference Martens, Schultze and Cloutier1996), which most likely lived in the water column and near the bottom, but these might be swallowed together with any other food mass. Strictly speaking, any substance scraped off the bottom surface is mud, containing inclusions.
A significant amount of the nutritional matter is constituted by various algae in the sea or any other plants in the continental basins. This plant matter could be scraped off the substrate and afterward mechanically processed. This transformation might be regarded as a transitional stage between mud filtering and herbivory. If we regard predation as a derived filter feeding, that is, extraction of a single rather than numerous prey objects from the environment, eating plants may be regarded as a derived type of filtration of sediment rich in organic matter. Partial herbivory is supported by an immense spiral valve in Bothriolepis canadensis (Denison, Reference Denison1941) used to house a large quantity of reducing bacteria and to slowly transport a large amount of food matter, maximizing the removal of nutrients.
Most researchers studying antiarch feeding and supporting the mud-grubbing hypothesis (Romer and Grove, Reference Romer and Grove1935; Denison, Reference Denison1941, Reference Denison1961; Upeniece, Reference Upeniece2001) focused on the “weak” structure of the infragnathals in Bothriolepis canadensis and other members of this group. Our survey of jaw apparatuses revealed a wide morphological spectrum of antiarch gnathals. Oral blades of “Livnolepis,” the strongest biter among antiarchs, and Bothriolepis sp. infragnathals from Livny are robust, arched, and deep, in contrast to those known in many other antiarchs, and capable of scratching and/or shearing tough food objects. We suggest here that an observed variety of jaw morphologies in various antiarch taxa reflects a wide spectrum of their trophic adaptations, most probably including herbivory.
In the aquatic assemblage, these food objects might be classified according to their resistance to mechanical processing by consumers into the following groups: (1) hard (shelled mollusks, hard-shelled arthropods and brachiopods, corals, and most echinoderms, such as sea urchins), (2) tough or dense (thallus algae, vascular plants, thin-shelled mollusks, arthropods, and brachiopods), and (3) soft (hydrozoan and scyphozoan coelenterates, ctenophores, and various worms). A separate nutritional group (4) includes micromeric objects dispersed in water and bottom sediments (bacteria, unicellular algae, protozoans, small arthropods, and various larvae).
Of these, hard-shelled animals might be easily excluded from the list of the potential antiarch food items. It is not impossible that some antiarchs fed on micromeric objects; however, we have neither direct morphological nor paleoecological evidence for this. Soft-bodied animals seem to be suitable food objects for antiarchs with less-arched gnathal elements. For those with deeper and arched oral blades, we may suggest nutritional specialization on tougher food objects, including thallus algae and arthropods with thin carapaces. Highly abundant genera, such as Bothriolepis and Remigolepis, were most probably herbivorous and fed on thallus algae scraped from the substrate.
The dispersal of antiarchs during the Devonian
Vertebrate assemblages presenting the oldest antiarchs are described from the Silurian of China (Zhao and Zhu, Reference Zhao and Zhu2010) from the territory of the Pan-Cathaysian galeaspid province. The oldest antiarchs (Telychian, late Llandovery) have been found in association with the agnathan galeaspids and other vertebrates (Fig. 14.1). Starting from the Lochkovian, the composition of the communities including antiarchs changed. Galeaspid agnathans still dominated numerically, but antiarchs had become the second most numerous vertebrate group. All known localities are thought to be formed in shallow-marine conditions. The percentage of antiarch taxa in assemblages indicated they became prevalent starting from the late Eifelian; from this time until the end of the Famennian, the depositional environments varied from shallow-water marine to lacustrine (Zhao and Zhu, Reference Zhao and Zhu2010). Increasing numbers of antiarchs suggests their success in the occupation of the new ecological niches and a certain degree of euryhalinity.

Figure 14. Paleogeographic dispersal of antiarchs and armored agnathans. (1) Global dispersal during the Silurian–Devonian. (2) Diversity curves for antiarch placoderms and osteostracan, cyathaspidiform, and pteraspidiform agnathans of Laurussia. Note the Eifelian–Frasnian increase of antiarchs versus the decline of agnathan groups. Diversity curves based on unpublished database in PIN RAS.
During the Emsian, the antiarchs expanded to Gondwana, and at the beginning of the Eifelian, to Euramerica (Young, Reference Young1990, Reference Young2010; Young et al., Reference Young, Burrow, Long, Turner and Choo2010) (Fig. 14.1). The extinction of the cyathaspidiform and most of the pteraspidiform agnathans by the Eifelian in Euramerica coincided with the antiarch invasion to these new territories (Fig. 14.2) rich in resources. Filter-feeding agnathans (Denison, Reference Denison1961) and mainly macrophytophagous scraping bothriolepidoid antiarchs exploited different food types. These vertebrates co-occurred within the same trophic webs and might have competed for common occupation of bottom-dwelling environments or some other yet unknown ecological parameter. During the Eifelian–Frasnian interval, antiarchs successfully shared space with the osteostracans, anaspids, and especially remaining pteraspidiform psammosteids.
We suggest here that antiarchs became the first phytophagous vertebrates that occupied the trophic level of primary consumers during the late Silurian–Early Devonian. This novel way of antiarch feeding might have influenced the water plant community and ecosystem as a whole. Previously existing simple predator–prey structure of vertebrate trophic chains between filter-feeding agnathans and predatory gnathostomes thus became remodeled.
Conclusions
The suborbital–infragnathal morphofunctional unit in antiarchs is classified into four main types based mostly on the morphology of the oral (occlusal) margins. The first, exemplified by the Yunnanolepidoidei, shows the absence of the oral shelf on the comparatively straight and incurved oral margin of the suborbitals. In the second, represented by the Asterolepidoidei, the oral margin is straight or slightly convex, and suborbitals are deep and tightly connected, even by a suture in the midline. In the third group, including most of the Bothriolepidoidei, the oral margins are strongly convex and form a median notch. On the contrary, the outline of the oral margin of the oral portion of the infragnathals is more or less straight or even concave. The fourth group, demonstrating a reversed configuration of the mouth opening, is exemplified by two infragnathals from Central Russia in which the oral margin is strongly convex rather than concave. These variations reflect differences in the structure of the jaw apparatus, suggested to be closely related to food processing.
The structure of all antiarch gnathal elements is more or less porous. This porosity suggests active blood supply to the bone surface and, correspondingly, the presence of an external cover protecting them and requiring extensive blood supply. This cover should be hard to reinforce vulnerable oral surfaces during their contact with food and substrate. We suggest that the nature of this cover ranged from a comparatively thin layer of hard skin to a keratinous cap.
The curvature of the aboral margin of the suborbitals fits that of the rostral margin of the oral fenestra. Repeated observed placement of the suborbitals in the same plane with that of the ventral side of the trunk armor suggests that this had been their natural “mouth closed” position. Manipulations with a physical model result in a hypothesis that mouth opening was performed by combined depression and inward rotation of each lower jaw antimere, while mouth closure was by combined adduction and outward rotation. Only the ball-and-socket type of mandibular articulation could provide this combined mobility simultaneously involving two degrees of freedom.
A variety of jaw structures in antiarchs suggests a wide spectrum of their food items. It is suggested that reconstructed keratinous caps and structure of the jaw apparatuses at least in the Bothriolepidoidei served as tools scraping ground surface for plant food, such as thallus algae. In the redescribed specimens of “Livnolepis zadonica” and Bothriolepis sp. from Central Russia, robust and deep oral blades of the infragnathals present strongly expressed versions of this scraping tool.
Arising during the Silurian within the agnathan-dominated vertebrate communities, in the Emsian the antiarchs expanded to Gondwana and, at the beginning of the Eifelian, to Euramerica. During the Eifelian–Frasnian interval, antiarchs became dominant in number within the vertebrate communities. Bothriolepid antiarch macrophytophagy is suggested to have become a novelty within the vertebrates; these fishes were the first to occupy the primary consumer's trophic level. This remodeled the only previously existing simple trophic interrelationships between filter-feeding agnathans and predatory gnathostomes.
Acknowledgments
Osteological materials on the recent Clangula hyemalis birds had been kindly presented for our comparative studies by N. Zelenkov and on Agrionemys horsfieldii turtles by E. Syromyatnikova (PIN RAS). This study was funded by RFBR and the Royal Society of London (RS), project number 21–54–10003. Excellent macrophotography was performed by S.V. Bagirov (PIN RAS). The authors acknowledge kind assistance from R.A. Rakitov (PIN RAS), who performed the SEM studies.
Data availability statement
Data available from the Dryad Digital Repository: https://doi.org/10.5061/dryad.9zw3r22gw.
Competing interests
The authors declare none.