Introduction
Parasitic associations have a long evolutionary history, with the earliest example described from the early Cambrian (Bassett et al., Reference Bassett, Popov and Holmer2004). During the Great Ordovician Biodiversification Event (GOBE), as increasingly complex ecosystems were becoming more extensive and diverse, symbiotic relationships between organisms also evolved, including examples of both parasitism and commensalism (Servais and Harper, Reference Servais and Harper2018). These relationships are often difficult to identify in the fossil record due to a lack of direct evidence, but examples of probable symbiotic relationships between drilling and encrusting symbionts and their hosts are known from the Ordovician in a number of marine invertebrate groups, including graptolites (Bates and Loydell, Reference Bates and Loydell2000), echinoderms (Deline, Reference Deline2008), chitinozoans (Grahn, Reference Grahn1981), bryozoans (Palmer and Wilson, Reference Palmer and Wilson1988), corals (Vinn and Mõtus, Reference Vinn and Mõtus2012), and brachiopods (Vinn, Reference Vinn2005; Zhan and Vinn, Reference Zhan and Vinn2007; Vinn et al., Reference Vinn, Wilson and Toom2014).
The earliest-known brachiopod parasite was found in early Cambrian lingulates from Kazakhstan (Bassett et al., Reference Bassett, Popov and Holmer2004). However, the earliest-known parasitic association with rhynchonelliform brachiopods was found from the Caradocian (Upper Ordovician) of Estonia (Vinn, Reference Vinn2005; Vinn et al., Reference Vinn, Wilson and Toom2014). One example consists of a tubular structure penetrating through the shell into the mantle cavity of a Clitambonitidina brachiopod (Vinn et al., Reference Vinn, Wilson and Toom2014).
In this study, an example of a parasitic association in a rhynchonelliform brachiopod Rongatrypa xichuanensis (Xu, Reference Xu1996) from the Katian (Upper Ordovician) of Henan, central China, is reported. It is slightly younger than the Estonian examples. This trace consists of a pouch-shaped structure composed of secondary shell material, showing that the brachiopod was able to heal and implying that there was a long-term parasitic association. The aims of this paper are to: (1) describe the trace (including boring and bioclaustration) in a brachiopod shell of Rongatrypa xichuanensis, (2) investigate its parasitic origin, (3) discuss the possible drilling organism, and (4) review two types of Paleozoic brachiopod parasitic traces on the basis of previous reports.
Geological setting
Shells examined in this study were collected from the Shiyanhe Formation at the Shiyanhe and the Heishui'an sections in southwestern Henan Province, central China (Fig. 1). This region is part of the eastern Qinling orogenic belt, which formed the northern margin of the South China paleoplate (Rong et al., Reference Rong, Zhan, Wang, Huang, Tang and Luan2015; Jing et al., Reference Jing, Stouge, Ding, Wang and Zhou2017) located in the equatorial region during the Late Ordovician (Torsvik and Cocks, Reference Torsvik and Cocks2017).

Figure 1. Generalized map of central China and studied region in southwestern Henan, modified from Zhang et al. (Reference Zhang, Huang, Zhan, Sproat and Zhou2019a).
The Upper Ordovician is well exposed in the study region. The Shiyanhe Formation is composed of interbedded carbonate siltstones and bioclastic grainstones. Conodonts from the formation belong to the Oulodus ulrichi, the Oulodus robustus, the Aphelognathus grandis, and the Aphelognathus divergens biozones, indicating a Katian age (Ka2-Ka4) (Jing et al., Reference Jing, Stouge, Ding, Wang and Zhou2017).
Both sections are located in Xichuan County. The well-studied Shiyanhe section, north of Shiyanhe Village (111°18′33.6″E, 33°07′37.02″N; Fig. 1), is accessible along a country road. The Shiyanhe Formation is well exposed along a valley with a total thickness of 97 m. The Heishui'an section is located to the north of Heishui'an Village (111°20′34.89″E, 33°07′00.50″N; Fig. 1). The thickness of the Shiyanhe Formation at this section is unknown because the lower part is covered by Quaternary deposits.
The diverse fossil fauna collected from this formation includes brachiopods, corals, gastropods, cephalopods, and bryozoans, indicating a well-oxygenated tropical shallow marine environment (e.g., Deng, Reference Deng1987; Rong et al., Reference Rong, Zhan, Wang, Huang, Tang and Luan2015). The depositional background in the studied region shows a clear difference from other regions in South China since the majority of the South China paleoplate was dominated by the anoxic black shale of the Wufeng Formation (e.g., Chen et al., Reference Chen, Zhang, Fan, Cheng and Li2010; Chen et al., Reference Chen, Fan, Melchin and Zhang2014; Zhao et al., Reference Zhao, Jin, Jin, Wen, Geng, Yan and Nie2017) during the Katian of the Late Ordovician.
Materials and methods
There are 450 brachiopod specimens with two conjoined valves collected from the upper part of the Shiyanhe Formation of the above-mentioned two sections in the study region. The shells are poorly preserved and often encrusted with hard and resistant matrix material that is difficult to remove. Among our collection, 402 shells were identified as the atrypid brachiopod Rongatrypa xichuanensis with a considerable variation in shell size (Fig. 2; Zhang et al., Reference Zhang, Zhan, Sproat and Huang2019c). Altogether, 13 conjoined shells were serially sectioned to examine the internal morphology. One individual of Rongatrypa xichuanensis from the upper part of the Shiyanhe Formation at the Shiyanhe section has been found with the clear evidence of a drilling trace (Figs. 3–6). Another R. xichuanensis shell collected from the same horizon at the Shiyanhe section was found with a gastropod shell attached on its ventral exterior near the hinge line.

Figure 2. Histograms of the shell length, width, and depth of the brachiopod Rongatrypa xichuanensis from the upper part of the Shiyanhe Formation (Upper Ordovician) at Shiyanhe section and Heishui'an section, southwestern Henan, central China. (1–3) The x-axes indicate (1) the length, (2) the width, and (3) the depth of the shell in millimeters. The y-axes indicate the percentages of shells in the measured quantities. A total of 402 conjoined valves are measured for statistics.
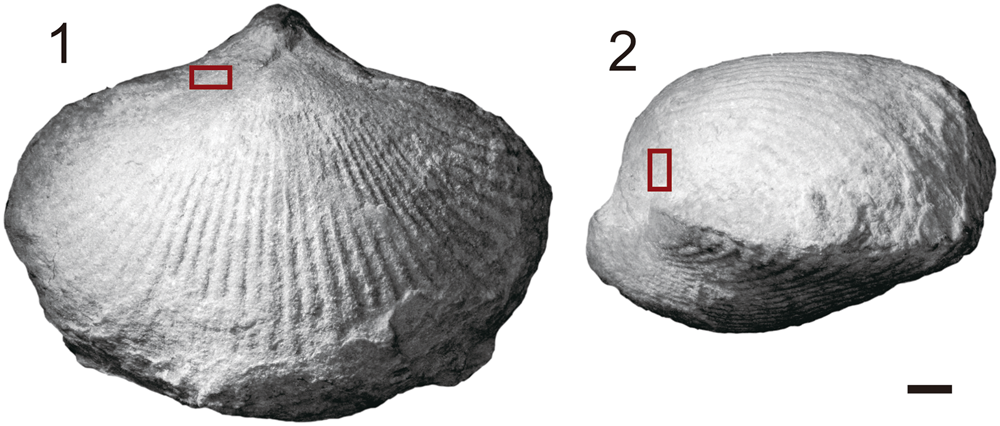
Figure 3. Photos of brachiopod Rongatrypa xichuanensis from the upper part of the Shiyanhe Formation (Upper Ordovician) at Shiyanhe section, southwestern Henan, central China. (1, 2) Dorsal and lateral views of Rongatrypa xichuanensis, specimen catalog number NIGP 168264. Dark red rectangles show the location of the trace on the brachiopod dorsal shell. Scale bar = 2 mm.

Figure 4. Photos of sections showing the trace in the shell of Rongatrypa xichuanensis from the upper part of the Shiyanhe Formation (Upper Ordovician) at Shiyanhe section, southwestern Henan, central China. (1–6) Magnified photos of acetate peels showing the trace and surrounding shell material at (1) posterior boundary of the trace, (2) 0.25 mm, (3) 0.40 mm, (4) 0.55 mm, (5) 0.70 mm, and (6) 0.80 mm from the posterior boundary of the trace, specimen catalog number NIGP 168264. Yellow dashed lines show the boundary of the brachiopod shell; triangular marks in (2–4) point to a series of micro-elevations (i.e., attachment structure) on the attachment region between the secondary shell material and the drilling organism. Scale bars = 0.5 mm.

Figure 5. Photos of the cellulose acetate film serial sections showing the detailed microstructures of the trace and the surrounding shell of the brachiopod Rongatrypa xichuanensis from the upper part of the Shiyanhe Formation (Upper Ordovician) at Shiyanhe section, southwestern Henan, central China. (1) Magnified photos of acetate peel showing the trace and surrounding shell material at 0.60 mm from the posterior boundary of the trace, specimen catalog number NIGP 168264. (2, 3) Magnification of areas marked by white rectangles in (1). Yellow dashed lines in (1) and (2) show the boundaries of the brachiopod primary shell material; red dashed lines in (1) show the boundaries of the brachiopod secondary shell material; blue dashed lines in (1), (2), and (3) show the boundaries between the primary shell material and the secondary shell material of the brachiopod; triangular marks in (1) point to a series of micro-elevations (i.e., attachment structure) in the region between the secondary shell material and the drilling organism. (1) Scale bar = 0.4 mm; (2, 3) scale bars = 0.1 mm.
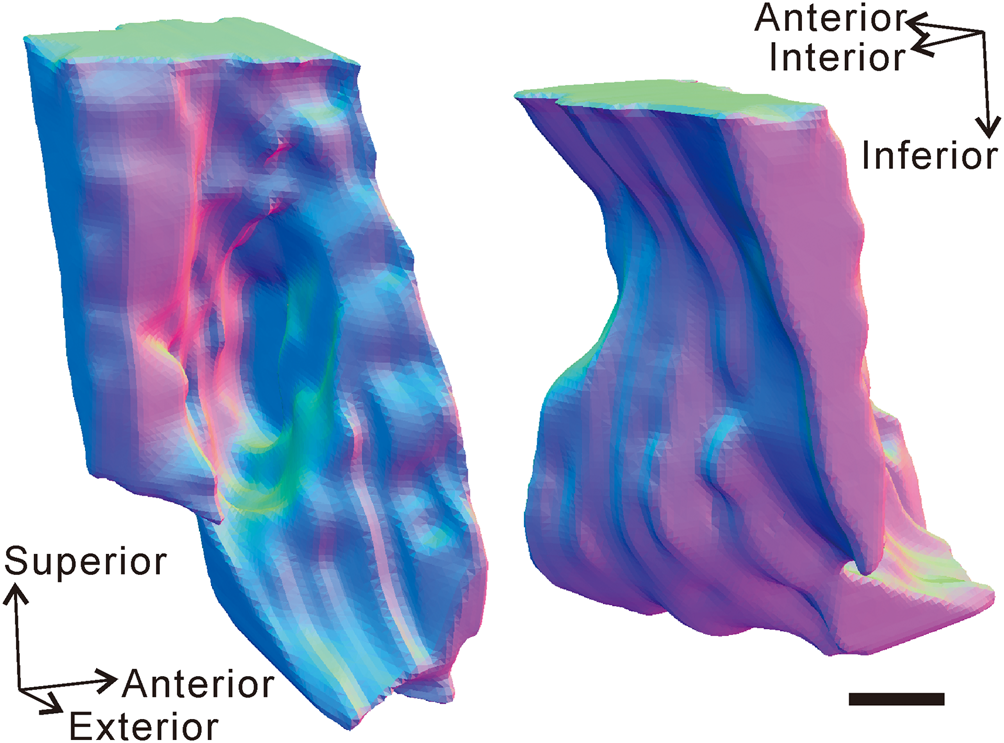
Figure 6. Three-dimensional model showing the external morphology of the trace (including the boring and the bioclaustration) and the surrounding shell of the brachiopod Rongatrypa xichuanensis from the upper part of the Shiyanhe Formation (Upper Ordovician) at Shiyanhe section, southwestern Henan, central China. Arrows point to the directions of the model in 3-D space. Scale bar = 0.2 mm.
A parallel grinder (Zhang et al., Reference Zhang, Sproat, Zhan, Zhang, Luan and Huang2019b) was used for serial sectioning to investigate the structure of the trace and the surrounding microstructure of the shell at intervals of 0.05 mm. The sections were produced by first treating the polished surface with dilute hydrochloric acid to enhance the relief of the shell. A drop or two of acetone was then applied to the surface before applying a thin film of cellulose acetate. Upon drying, the acetate was carefully removed from the surface to avoid warping. The resulting section preserves the fine details of the shell, including the fibrous layers of the shell structure, making this technique ideal for this study.
All peels were observed and photographed under an optical microscope (Zeiss Microsystems SYCOP 3, Germany). These images were then traced using the vector graphics program CorelDraw X8. A selection of 14 of these sections that include the trace and the surrounding shell was processed for three-dimensional (3-D) reconstruction using the software Geomagic Studio (version 12) and 3-Matic (version 12.0).
Repository and institutional abbreviation
The remains of the brachiopod specimen, all acetate peels, and an uncut brachiopod specimen with a gastropod attached examined in this study are deposited in Nanjing Institute of Geology and Paleontology (NIGP), Chinese Academy of Sciences, Nanjing, China (NIGP 168264, 169048).
Description of the shell and trace
The brachiopod shell with the trace is 18.7 mm long, 24.4 mm wide, and 12.3 mm high—large relative to other specimens of this species from the same horizon (Fig. 2). The boring is located on the lateral flanks of the dorsal valve (Fig. 3), 3.1 mm from the commissure. Secondary shell material reinforces the damaged primary shell, isolating the boring from the interior of the shell and forming a pouch-shaped cavity open to the exterior of the shell (Figs. 4, 6). The oval-shaped aperture of the cavity is perpendicular to the shell exterior, and the boring extends almost vertically toward the mantle cavity of the brachiopod. The aperture measures 1.0 mm by 0.6 mm, and the pouch-shaped cavity has a depth of 1.0 mm.
Secondary shell material around the boring thickens around the aperture (Figs. 4.2–4.6, 5.1). A series of micro-elevations and micro-depressions are located on the inner surface of the pouch-shaped cavity (Figs. 4.2–4.4, 5.1). The outer surface of the pouch-shaped cavity is smooth and lacking any ornamentation. The secondary shell material is fibrous and continuous and is overall finer and denser compared with the primary layer (Fig. 5).
A 3-D model of the trace and part of the brachiopod is shown in Figure 6 in two orientations.
Discussion
The identification and interpretation of parasitic drilling
Interpretation of biotic relationships from fossils is difficult due to the lack of direct evidence of the interaction between organisms in the fossil record. Nonetheless, three broad categories have been established: postmortem bioerosion, predation, and symbiotic relationships.
Postmortem bioerosion involves drilling of dead shells by a benthic organism (Richards and Shabica, Reference Richards and Shabica1969). Boring predation implies that boring drillers act as predators that prey on the host shells, ultimately leading to the death of the brachiopod when successful (e.g., Kowalewski et al., Reference Kowalewski, Simoes, Torello, Mello and Ghilardi2000; Leighton, Reference Leighton2001, Reference Leighton, Kelley, Kowalewski and Hansen2003; Hoffmeister et al., Reference Hoffmeister, Kowalewski, Bambach and Baumiller2003; Robson and Pratt, Reference Robson and Pratt2007; Chattopadhyay, Reference Chattopadhyay2011). Symbiotic relationships can be subdivided into three types: (1) parasitism (when one symbiont benefits from living in association with the other, and the other incurs losses); (2) mutualism (when both organisms benefit from the relationship); and (3) commensalism (when one benefits and the other incurs neither losses nor benefits) (Taylor and Wilson, Reference Taylor and Wilson2003).
Interpreting the origin of borings in fossil brachiopod shells is often difficult due to the lack of preserved in situ interactions between the organisms.
Despite relatively few examples of these relationships, some criteria have been established for distinguishing these associations. In postmortem bioerosion, the borings can extend through brachiopod shells and the hard/soft substrate below. Shells are commonly disturbed by the drilling organisms, indicated by bioturbation in the substrate adjacent to the shell (Richards and Shabica, Reference Richards and Shabica1969). A single boring with the smooth and sharp edge in the shell with no evidence of shell repair implies a short-termed association that may have been fatal for the brachiopod, which often implies predation (Cameron, Reference Cameron1967; Rohr, Reference Rohr1976; Chatterton and Whitehead, Reference Chatterton and Whitehead1987; Leighton, Reference Leighton2001; Robson and Pratt, Reference Robson and Pratt2007).
Symbiosis can be differentiated from predation when there is evidence of an attachment structure, borings that do not penetrate the brachiopod shell, or multiple healed drill sites that can indicate a long-term association between the host shell and the symbiont (Baumiller, Reference Baumiller1990; Brown and Alexander, Reference Brown and Alexander.1994; Bassett et al., Reference Bassett, Popov and Holmer2004; Vinn et al., Reference Vinn, Wilson and Toom2014).
Symbiotic associations in the fossil record are difficult to specifically classify as parasitic, commensal, or mutual (Taylor and Wilson, Reference Taylor and Wilson2003). Parasitism emphasizes the biotic interaction that benefits the parasite and is detrimental to the host, which should be distinguished from commensalism and mutualism. However, there is usually little evidence in the fossil record that can be used to determine whether the host is harmed.
The material reported here shows that the boring in the brachiopod has been healed (Figs. 4, 5). The growth of the secondary shell material strengthens the primary shell in the damaged area (Fig. 5), and the attachment structure can be identified in the trace as a series of micro-elevations and micro-depressions (Figs. 4.2–4.4, 5), indicating the brachiopod was colonized by the drilling organism earlier in development and the damage to the shell did not cause the death of the brachiopod. The growth of the fine-crystalized secondary layer and the presence of micro-elevations and micro-depressions can be interpreted as the evidence of a long-term association between the brachiopod and the drilling organism because the calcite shell needs time for growth to repair the drilling, form the pouch-shaped cavity, and develop the attachment structure on the margin of the two organisms. Given that the brachiopod must have been alive during and after the drilling, it is not an example of either postmortem bioerosion or predation.
Most brachiopods are interpreted to have had inhalant currents drawn in from the lateral flanks of the shell and an exhalant current exiting the shell medially at the anterior (Rudwick, Reference Rudwick1970; LaBarbera, Reference Labarbera1977). The location of the trace near the lateral commissure of brachiopod on the dorsal valve implies the driller might have benefited from the inhalant feeding current generated by the lophophore by filtering food particles from the incurrent before they reached the lophophore. In addition, shell repair would have been a metabolic cost for the brachiopod, decreasing its overall fitness. However, potential loss of nutrition or shell repair would not have affected the brachiopod to any significant degree as no clear growth disruption evidence was found in the shell. Given the lack of any conceivable benefit to the brachiopod in the association, the evidence points to a parasitic relationship between the driller and the brachiopod.
It should be noted that the trace in the brachiopod shell in this study is actually a combination of a trace fossil (i.e., the classically defined boring) and a bioclaustration (i.e., the pouch-shaped cavity composed of the secondary shell material). Similar compound traces have been previously described in some Cenozoic coral reefs (Edinger and Risk, Reference Edinger and Risk1994). These traces consist of both the boring by parasites and the bioclaustration structure (i.e., the thickened secondary layers of the shell for repairing the damage) developed by the host organisms (Taylor, Reference Taylor1990). This compound structure is not considered a true trace fossil (ichnofossil) because the shape of the bioclaustration on a specific host can be variable and unpredictable when it was affected by different parasites (Bertling et al., Reference Bertling2006).
Although the trace and associated bioclaustration are well defined, some aspects of the parasite remain uncertain. The size of the parasite in this study, for example, cannot be predicted on the basis of the current material. It is also uncertain whether the parasite used the trace as a shelter, living completely within the boring, or whether it would have been attached to the surface (although the second shell with an attached gastropod favors the latter interpretation; see Fig. 7).
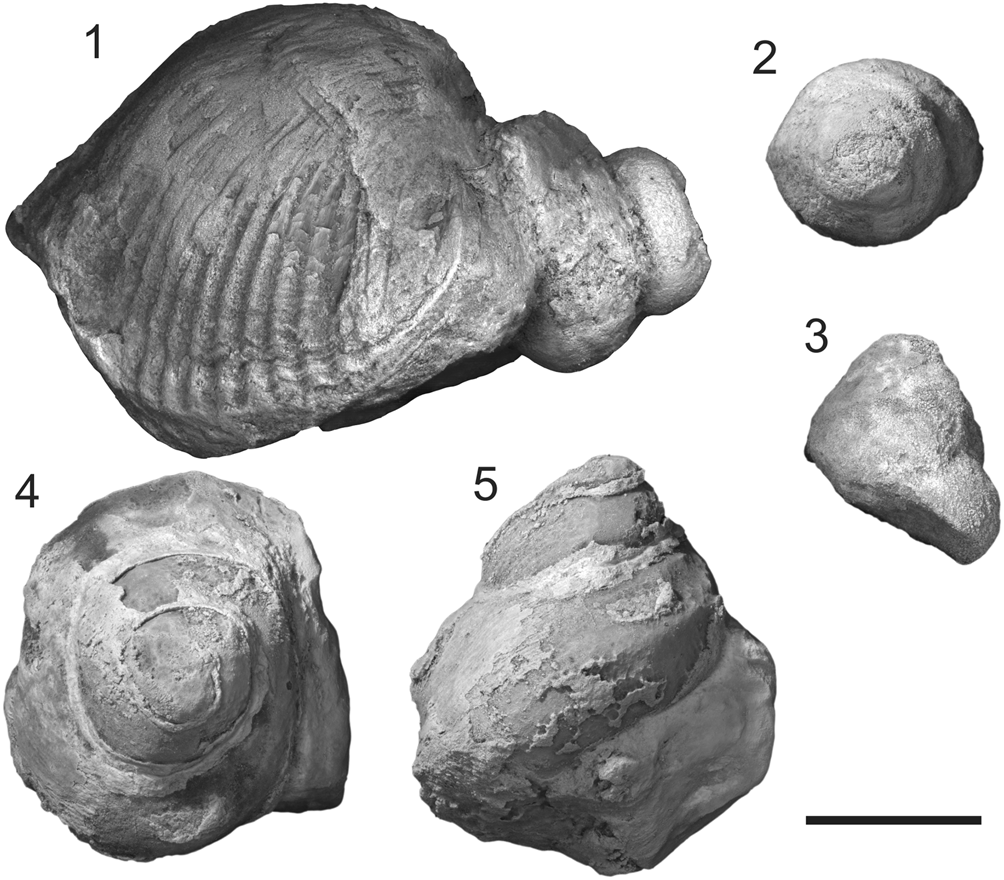
Figure 7. Photos of a conjoined shell of brachiopod Rongatrypa xichuanensis with a gastropod shell attached on its surface and two endocasts of platyceratid gastropods Cyclonema? sp. from the upper part of the Shiyanhe Formation (Upper Ordovician) at Shiyanhe section, southwestern Henan, central China. (1) Lateral view of a conjoined shell of brachiopod Rongatrypa xichuanensis with a gastropod shell Cyclonema? sp. attached on its ventral exterior near the hinge line, specimen catalog number NIGP 169048. (2–5) Lateral and vertical views of two specimens of platyceratid gastropods Cyclonema? sp. collected from the same horizon as specimens NIGP 168264 and NIGP 169048. Scale bar = 5 mm.
Platyceratid gastropods as the perpetrator
Platyceratide gastropods were geologically long-lived (Ordovician to Permian), abundant, and occurred across a broad range of marine settings (Bowsher, Reference Bowsher1955). These fossil gastropods have been found attaching to and drilling the shells of a variety of taxa, including crinoids (e.g., Bowsher, Reference Bowsher1955; Baumiller, Reference Baumiller1990; Baumiller and Gahn, Reference Baumiller and Gahn2002), blastoids (Baumiller, Reference Baumiller1993, Reference Baumiller1996; Baumiller and Macurda, Reference Baumiller and Macurda1995), cystoids (Kluessendorf, Reference Kluessendorf1983), and brachiopods (Baumiller et al., Reference Baumiller, Leighton and Thompson1999). The relationship between platyceratids and crinoids, at least, has been suspected of being parasitic (Lane, Reference Lane1984; Baumiller, Reference Baumiller1990, Reference Baumiller2003; Baumiller and Gahn, Reference Baumiller and Gahn2002; Gahn and Baumiller, Reference Gahn and Baumiller2003, Reference Gahn and Baumiller2006) as they have been found attached to the anal vent of stalked crinoids (Gahn and Baumiller, Reference Gahn and Baumiller2006), stealing nutrition from the calyx (Rollins and Brezinski, Reference Rollins and Brezinski1988). In this position, the parasite would have negatively affected the balance of the crinoid in the water column. The gastropods may even have directly penetrated into the guts of the crinoid to steal nutrients directly from the digestive system (Gahn and Baumiller, Reference Gahn and Baumiller2003).
In terms of brachiopods, platyceratids are known to have drilled Brachythyris and Spirifer from the Mississippian of Kentucky, USA (Baumiller et al., Reference Baumiller, Leighton and Thompson1999). The diameter of borings in those shells varies from 1 mm to 3.5 mm (Baumiller et al., Reference Baumiller, Leighton and Thompson1999), similar to the size of the boring in this study.
In our collection, 32 specimens of platyceratid gastropods were collected from the same unit of the Shiyanhe Formation as the specimens shown here (Fig. 7). One of these specimens was found attached to the ventral posterior of the brachiopod Rongatrypa xichuanensis (Fig. 7.1) across the hinge line. We cannot determine whether the gastropod bored into the brachiopod, but its position on the shell supports our hypothesis that platyceratid gastropods may have been parasitic even if the gastropod was not found attached to this sectioned shell.
The elongate shape of the opening and the general pouch-shaped morphology of the cavity are also similar to the ichnofossil Rogerella isp., thought to have been produced by acrothoracid barnacles. Acrothoracids are capable of drilling on a range of shelly organisms, for example, echinoids (Donovan and Jagt, Reference Donovan and Jagt2013; Donovan et al., Reference Donovan, Jagt and Goffings2014, Reference Donovan, Jagt and Nieuwenhuis2016), oysters (El-Sabbagh et al., Reference El-Sabbagh, Mansour and El-Hedeny2015), crinoids (Mirantsev, Reference Mirantsev, Zamora and Rábano2015), and gastropods (Schlaudt and Young, Reference Schlaudt and Young1960). Acrothoracids are filter feeders (Lin et al., Reference Lin, Kobasov and Chan2016) and thought to have a parasitic relationship that took advantage of the hosts (Baird et al., Reference Baird, Brett and Tomlinson1990; Mirantsev, Reference Mirantsev, Zamora and Rábano2015). In this study, acrothoracids could benefit from the inhalant current of the brachiopod to increase their nutrient availability and would have benefited from the stable substrate of the brachiopod shell in a dominantly muddy environment. However, the traces that acrothoracids bore, that is, Rogerella isp., have been known to range only from Devonian to Recent (Baird et al., Reference Baird, Brett and Tomlinson1990; Wilson, Reference Wilson and Miller2007). A few questionably assigned Silurian traces were described as Rogerella isp. (Bundschuh, Reference Bundschuh2000). However, their taxonomy has not been widely agreed on because the original illustrations (Bundschuh, Reference Bundschuh2000) are not very convincing (Bromley, Reference Bromley and McIlroy2004).
The trace in this study might also be interpreted as a gall-like formation induced by endolithic sponges growing on brachiopods (Bromley et al., Reference Bromley, Beuck, Ruggiero, Wisshak and Tapanila2008). However, typically there are many irregular chambers and branched threads developed in the gall-like formation (Bromley et al., Reference Bromley, Beuck, Ruggiero, Wisshak and Tapanila2008) that are not seen here. In addition, the size of the cyst (Bromley et al., Reference Bromley, Beuck, Ruggiero, Wisshak and Tapanila2008) caused by the sponge is much larger than that in this study.
Comparison to other examples of Paleozoic brachiopod parasitism
Although uncommon, several other examples of Paleozoic brachiopod parasitism have been identified thus far. These parasitic traces can be classified into two main types: constructive association and destructive association. The criterion for distinguishing the two is whether parasites damage the host brachiopod shells. By establishing a framework for classifying these associations, we can more easily interpret their ecological and evolutionary significance going forward.
Constructive association occurs when the parasite cements itself to the inner surface of the shell and constructs a tube-shaped structure with a single aperture, most commonly on the interior of shell. Examples of this type in the fossil record range from Cambrian to Carboniferous in age, including a tubular structure projecting into the mantle cavity of the lingulate brachiopod Linnarssonia constans Koneva in Goryansky and Koneva, Reference Goryansky and Koneva1983 from the lower Cambrian of Kazakhstan (Bassett et al., Reference Bassett, Popov and Holmer2004), another example found in the ventral mantle cavity of the Late Ordovician rhynchonelliform brachiopod Clitambonites squamatus Pahlen, Reference Pahlen1877 from Estonia (Vinn et al., Reference Vinn, Wilson and Toom2014), several open-ended tubes extending inward from the dorsal interiors of Early Devonian spiriferid brachiopods in Australia (Chatterton, Reference Chatterton1975), and several tubular infestation structures in the dorsal interiors of early Carboniferous spine-bearing brachiopods in South China (Baliński and Sun, Reference Baliński and Sun2010). These tubes that open into the mantle interior cavities of brachiopods are most likely shelters of suspension-feeding parasites, variously interpreted as Polychaeta, Cnidaria, Tunicata, or Phoronida, that took advantage of the feeding currents generated by the lophophore. The shell of the brachiopod host offered protection from predators (Vinn et al., Reference Vinn, Wilson and Toom2014). Although the parasite would have robbed the brachiopod of food and nutrients, there is no indication of direct damage to the host shell.
Destructive association differs in the bioerosion done to the host shell by the parasite (e.g., boring, nicking, or invagination). Examples of this are rare in the fossil record. There are cavities filled with globose callosities and V-shaped invaginations on the valves of the linguliformean brachiopod Botsfordia from the early Cambrian of Greenland (Peel, Reference Peel2015). Multiple borings in a Cambrian linguliformean brachiopod from South Dakota, USA (Robson and Pratt, Reference Robson and Pratt2007), indicate a parasitic origin as they are too tiny to be related to predation. Borings in the Carboniferous spiriferide brachiopods Brachythyris and Spirifer in Kentucky, USA, may have been drilled by platyceratid gastropods (Baumiller et al., Reference Baumiller, Leighton and Thompson1999); however, their predatory/parasitic origin is still uncertain due to the lack of direct evidence. The example described here belongs to this type as the brachiopod shell has been partly damaged through boring.
Given that there are so few examples of either type of parasitism, it is difficult to draw any definitive conclusions from what has been documented thus far. Despite this, the preceding classification system still has some utility.
Occurrence of constructive association or destructive association may be related to brachiopod shell size and thickness. In taxa with a relatively thin shell, or under environmental stresses that might favor production of thin shells in brachiopods, drilling organisms may have been able to establish relatively easily due to the reduced protection offered to the host by the thinner shell (Robson and Pratt, Reference Robson and Pratt2007; Peel, Reference Peel2015). In larger and/or thicker shells that are more difficult to penetrate, drilling parasites would not have been able to establish so easily, and parasites that can simply attach themselves to the shell interior may have been comparatively more common among a host population (Chatterton, Reference Chatterton1975; Baliński and Sun, Reference Baliński and Sun2010; Vinn et al., Reference Vinn, Wilson and Toom2014).
Additional examples of both types are needed to confirm whether the appearance of the parasitic trace is a signal of shell size and thickness or influenced by other unknown reasons given the few examples that have been documented thus far, and a much larger collection is needed to confirm the significance of these early trends.
Conclusion
This early example of parasitic drilling of a rhynchonelliform brachiopod Rongatrypa xichuanensis was found from the Katian (Upper Ordovician) of Henan, central China. The boring extends almost perpendicular to the shell with an oval-shaped aperture. Secondary shell material surrounds the boring, forming a pouch-shaped cavity that is isolated from the interior of the brachiopod dorsal cavity.
Attachment structure and the growth of secondary shell material by the brachiopod support a long-term relationship between the brachiopod and the borer. Such a relationship is thought to be parasitic, with the borer capturing food particles from the inhalant feeding current before it reaches the brachiopod lophophore.
Platyceratid gastropods are known to be capable of drilling on a variety of organisms, including brachiopods, and have been found in association with the brachiopod in this study from the same horizon of the formation. Acrothoracid barnacles are also known to make a similar structure (Rogerella isp.), but no widely accepted examples are known from rocks earlier than the Devonian.
Two types of Paleozoic brachiopod parasitic traces, constructive association and destructive association, are classified depending on whether parasites damage the brachiopod shell. Constructive association is normally identified by a tube-shaped structure with a single aperture on the interior of the shell attached only to the shell surface. Destructive association is typified by this example, characterized by bioerosion of the shell by the parasite (e.g., boring, nicking, or invagination). The paleoecological implications of these two types are difficult to determine given the small number of preserved examples but may be useful to consider as new materials might be discovered and described in the future.
Acknowledgments
We are grateful to J.Y. Rong for his constructive suggestions. We also thank M. Wisshak, J. Huntley, and one anonymous reviewer for their helpful comments and suggestions. The 3-D model of the trace was created by Sichuan Tianxingshun Science and Technology Ltd. Financial support was provided by the Chinese Academy of Sciences (XDB 26000000), the National Natural Science Foundation of China (41530103), the Natural Science Foundation of Jiangsu Province (BK20191101), and the State Key Laboratory of Paleobiology and Stratigraphy. This paper is also a contribution to IGCP project 653 ‘The Onset of the Great Ordovician Biodiversification Event.’