Introduction
Fossil woods provide information not only on the botanical affinity, but also the paleoclimatic and paleoevironmental conditions where the trees grew. Fossil woods from the Tronquimalal Group of the Llantenes Depocenter, northern Neuquén Basin add information about the diversity of the flora of Argentina and Gondwana during the Late Triassic, and on the early diversification of modern conifers.
The Tronquimalal Group in the northern Neuquén Basin spans ~3.4 km2 and comprises the fluvial and lacustrine deposits of the Chihuido and Llantenes formations. These formations contain abundant plant fossils of Late Triassic age that are relevant to our understanding of Gondwanan floras. Previous studies of palynomorphs and leaves from the Tronquimalal Group revealed a “Dicroidium Flora” that is typical of the extratropical southwestern Gondwanan assemblages of Triassic age, including evidence of conifers (Volkheimer and Papú, Reference Volkheimer and Papú1993; Zavattieri and Batten, Reference Zavattieri, Batten, Jansonius and McGregor1996; Artabe et al., Reference Artabe, Morel, Spalletti and Brea1998, Reference Artabe, Morel and Spalletti2003; Spalletti et al., Reference Spalletti, Artabe, Morel and Brea1999, Reference Spalletti, Artabe and Morel2003; Zamuner et al., Reference Zamuner, Zavattieri, Artabe, Morel, Artabe, Morel and Zamuner2001). Fossil woods are abundant in the Tronquimalal Group and provide valuable information about forest composition and structure as well as climate, but so far only a handful of species have been recorded. Menéndez (Reference Menéndez1951) described Araucarioxylon sp. from the Chihuido Formation, and Crisafulli and Herbst (Reference Crisafulli and Herbst2010) reported Ginkgoales (Baieroxylon chilensis Torres and Philippe, Reference Torres and Philippe2002) and conifers assigned to the Araucariaceae (Agathoxylon dallonii [Boureau, Reference Boureau1948] Crisafulli and Herbst, Reference Crisafulli and Herbst2010), Podocarpaceae (Podocarpoxylon tikiense Ram-Awata and Rajanikanth, Reference Ram-Awatar and Rajanikanth2007), and Taxaceae (Prototaxoxylon intertrappeum Prakash and Srivastava, Reference Prakash and Srivastava1961) from the Llantenes Formation.
The primary aim of this work is to describe fossil woods collected during three field seasons from 2015 to 2017 in the Chihuido and Llantenes formations and to discuss their significance for understanding the flora of the Tronquimalal Group in the Llantenes Depocenter (Fig. 1). Our secondary aim is to provide new details on the geological and sedimentological contexts of these fossils in order to draw conclusions about the environments in which these plants grew and/or were deposited. We identified four fossil species of conifers from 64 wood fragments and we compared these specimens with those described by Menéndez (Reference Menéndez1951) and Crisafulli and Herbst (Reference Crisafulli and Herbst2010) from the Tronquimalal Group. We determined the systematic positions of these fossils by comparing the anatomy with that of other living and fossil conifers. Finally, we discuss our findings in the context of previous works on the flora of the Chihuido and Llantenes formations (Artabe et al., Reference Artabe, Morel, Spalletti, Artabe, Morel and Zamuner2001) and the significance of these woods for estimating the paleoclimate of the Neuquén Basin at the end of the Triassic.

Figure 1. Generalized outline of the Neuquén Basin, central-western Argentina showing the location of the Llantenes Depocenter, within the fold and thrust belt of Malargüe (modified from Vergani et al., Reference Vergani, Tankard, Belotti and Welsink1995). Image taken from NASA - National Aeronautics and Space Administration, July 2004.
Geological setting
The Neuquén Basin of west-central Argentina is a Mesozoic rifted back-arc basin on the western margin of southern South America, approximately between latitudes 30°S and 41°S (Fig. 1). Its development is attributed to extension during the fragmentation of Gondwana and the opening of the South Atlantic Ocean (Uliana and Biddle, Reference Uliana and Biddle1988). In the northern sector of the Neuquén Basin, a series of unconnected asymmetric fault-bounded depressions began to form during the early rifting stage (Manceda and Figueroa, Reference Manceda, Figueroa and Ramos1993, Reference Manceda, Figueroa, Tankard, Suarez Soruco and Welsink1995; Vergani et al., Reference Vergani, Tankard, Belotti and Welsink1995). The unrelated depocenters were initially filled with continental siliciclastic, pyroclastic, and volcanic Norian–Sinemurian deposits. One of these, the Llantenes Depocenter (35°40′S, 69°35′W), is located at ~20 km south of Malargüe city, on the north-eastern margin of the Andean sector of the Neuquén Basin, south-western Mendoza Province (Arcila Gallego, Reference Arcila Gallego2010) (Fig. 1). The initial Late Triassic rifting phase in the Llantenes Depocenter (Gulisano and Gutiérrez Pleimling, Reference Gulisano and Gutiérrez Pleimling1994, fig. 4, p. 17, 21) is represented by the Tronquimalal Group (Stipanicic, Reference Stipanicic1979), whose limited areal outcrops include continental fluvial and lacustrine deposits that were greatly influenced by pyroclastic and volcanic materials. It was developed on the basinal substrate of magmatic, volcanic, and pyroclastic rocks of the Choiyoi Group (Middle Permian–Middle Triassic) (Llambías et al., Reference Llambías, Kleiman and Salvarredi1993; Kleiman and Japas, Reference Kleiman and Japas2009). The Tronquimalal Group is overlain unconformably by fluvial, pyroclastic, and volcaniclastic Early Jurassic deposits of the Remoredo Formation (Spalletti and Morel, Reference Spalletti and Morel1992; Spalletti, Reference Spalletti1997; Franzese and Spalletti, Reference Franzese and Spalletti2001). The whole sequence was intruded by Cenozoic sub-volcanic andesites and basalt bodies (Menéndez, Reference Menéndez1951; Dessanti, Reference Dessanti1973).
Artabe et al. (Reference Artabe, Morel, Spalletti and Brea1998, Reference Artabe, Morel, Spalletti, Artabe, Morel and Zamuner2001, Reference Artabe, Morel and Spalletti2003), Morel et al. (Reference Morel, Ganuza and Zúñiga2000, Reference Morel, Artabe and Spalletti2003), Zamuner et al. (Reference Zamuner, Zavattieri, Artabe, Morel, Artabe, Morel and Zamuner2001), and Gnaedinger and Zavattieri (Reference Gnaedinger and Zavattieri2015) gave detailed lists of the paleobotanical content of both units integrating the Tronquimalal Group. Artabe et al. (Reference Artabe, Morel, Spalletti, Artabe, Morel and Zamuner2001) described four plant paleo-communities and the corresponding sedimentary paleo-environments of the Chihuido and Llantenes formations (Table 1, PC25-PC28). Their plant assemblages are characterized by components of typical Southern Hemisphere Triassic assemblages, characterized by the “Dicroidium Flora,” together with Jurassic elements (Table 1). Spalletti et al. (Reference Spalletti, Artabe, Morel and Brea1999) assigned the fossil flora of both units of the Tronquimalal Group to the Florian event of the upper Late Triassic (Norian–?Rhaetian) within the paleofloristic biozones of Argentina. Volkheimer and Papú (Reference Volkheimer and Papú1993) analyzed the microfloristic assemblages recorded from the upper levels of the Chihuido Formation, and they assigned the flora to Norian age based on the presence of the genus Classopollis Pflug, together with typical Triassic palynomorphs. In the Triassic basins of Argentina, the genus Classopollis has been recorded previously (sporadically) in late Late Triassic units (=Norian to Rhaetian). These Cheirolepidiacean pollen grains are frequent to dominant in Jurassic strata of Argentina and Gondwana (Grant-Mackie et al., Reference Grant-Mackie, Aita, Balme, Campbell, Challinor, MacFarlan, Molnar, Stevens and Thulborn2000; Cúneo et al., Reference Cúneo, Ramezani, Scasso, Pol, Escapa, Zavattieri and Bowring2013; de Jersey and McKellar, Reference de Jersey and McKellar2013; Olivera et al., Reference Olivera, Zavattieri and Quattrocchio2015, and references therein).
Table 1. Sedimentary paleoenvironments, associated paleoflora and paleoecological interpretation in the Chihuido and Llantenes formations; (1) Artabe et al. (Reference Artabe, Morel, Spalletti, Artabe, Morel and Zamuner2001); (2) Artabe et al. (Reference Artabe, Morel, Spalletti and Brea1998).

Sedimentary environments of the Tronquimalal Group
The type sections of both formations have been described along the Llantenes Creek (=Quebrada de los Llantenes) by Menéndez (Reference Menéndez1951), Spalletti and Morel (Reference Spalletti and Morel1992), and Spalletti (Reference Spalletti1997) (Fig. 2).
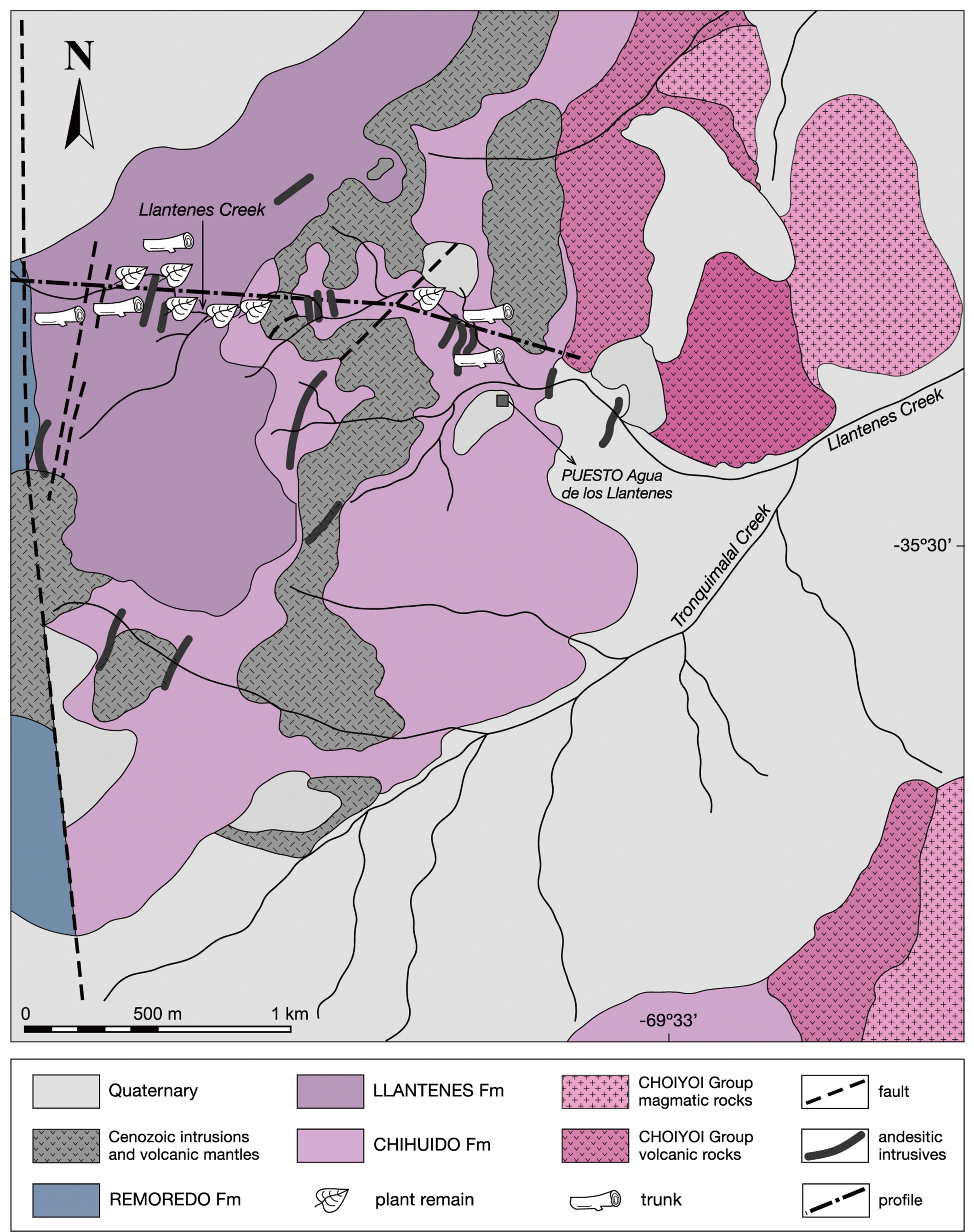
Figure 2. Geologic map of the Llantenes Depocenter showing the outcrops of the Chihuido and Llantenes formations and location of the studied profile (adapted from Menéndez, Reference Menéndez1951).
Chihuido Formation
The type section of the Chihuido Formation (Figs. 2, 3) consists of 190 m thick conglomeratic longitudinal bars, channel, and sheet-flood deposits interstratified with volcanic breccias, mudflow deposits, hyper-concentrated flood deposits, as well as sandy and gravelly transverse bar deposits (Figs. 3, 4.1–4.4). Spalletti (Reference Spalletti1997) interpreted this unit as composed of different proximal volcaniclastic facies. These were deposited in humid and volcanically controlled alluvial fans and braid-plains. The facies associations and depositional processes are summarized in Table 1 and Figure 3. The lower coarse-grained facies with log remains suggest a proximal high-energy system in the marginal parts of the basin (Spalletti, Reference Spalletti1997) (Figs. 2, 3, 4.1–4.3). Plant fossil leaf impressions and palynological assemblages were recorded from the siltstones and mudstones of the middle and upper distal parts of the Chihuido Formation (Fig. 4.5). Large trunks and drifted log fragments are intercalated within volcaniclastic gravelly bar deposits in the coarse-grained, clast-supported conglomerates and/or breccias of basal alluvial fan deposits (Figs. 3, 4.1–4.5).

Figure 3. Sedimentologic sections of the Chihuido and Llantenes formations showing the stratigraphic location of the studied fossil trunks and plant paleocommunities (PC 25 to 28) defined by Artabe et al. (Reference Artabe, Morel, Spalletti, Artabe, Morel and Zamuner2001) (adapted from Spalletti, Reference Spalletti1997).

Figure 4. (1–5) Chihuido Formation. (1) Outcrops of the lower part of the Chihuido Formation at Llantenes Creek showing thick and coarse clast-supported conglomerates with sandstone lenses and volcanic breccias intercalated, suggesting proximal high-energy system. Large trunks of A. lamaibandianus are intercalated within volcaniclastic gravelly bar deposits. (2) Detail of the coarse clast-supported conglomerates and/or breccias of alluvial fan deposits with drifted log fragment (glove for scale); (3) detail of the coarse clast-supported conglomerates and/or breccias interpreted as volcaniclastic hyper-concentrated deposits and debris-flow deposits related to conditions of high discharge; probably root fragment (hammer for scale); (4) upper section of the Chihuido Formation at Llantenes Creek, coarse clast-supported conglomerates of alluvial fans (above), coarse to medium, large scale, trough cross-stratification with erosive contact with drifted trunk fragments (hammer for scale) and tabular-planar beds of fine-grained gray levels of proximal braid-plains indicating proximal braided-channel migration. (5) Outcrop of the upper part of the Chihuido Formation at Llantenes Creek showing fine tabular- and planar-bedded sandstones and gray carbonaceous siltstone and mudstone levels intercalated below and above with andesitic breccias. Fossil plants and palynological assemblages recorded in these units come from the fine-grained carbonaceous gray levels (arrow). (6–9) Llantenes Formation. (6) Lacustrine environments. Predominantly gray-black carbonaceous and laminated siltstones, and fine-grained massive sandstones of the distal delta front deposits and subaquatic delta progradation; (7) detail of the finely laminated dark gray siltstones of meromictic lacustrine deposits of one of the lacustrine cycles of the Llantenes Formation; (8) tabular-planar and horizontal lamination and tabular-planar cross-stratification of fine-grained sandstones and siltstones interbedded within fluvial flood-plain deposits; (9) panoramic view of the uppermost part of the Llantenes Formation, high topographic nascent of Llantenes Creek (view from west to east). To the higher parts of this cycle, the content of sandstone increases and conglomeratic intercalations are more frequent, reflecting a deltaic mouth-bar and distributary channel deposits of deltaic progradation of the fluvial system into meromictic lacustrine environments; the black arrows indicate location of the studied trunks. At the bottom of the creek, there are outcrops of the Remoredo Formation (white arrow).
Llantenes Formation
The type section of 180 m thick of the Llantenes Formation is developed in continuity on the Chihuido Formation (Figs. 2, 3). Spalletti (Reference Spalletti1997) described in detail three facies associations, and he interpreted the Llantenes succession as progradations of fluvial-dominated deltaic lobes into lacustrine environments (Figs. 3, 5.1, 5.2) as consequence of tectonic regional subsidence. The lower lacustrine hypolimnic deposits are characterized by thick black to dark-gray organic laminated shales (Figs. 3, 4.6, 4.7), as well as by fine-grained and relatively thin sandstone horizons (Figs. 3, 4.8) that increase frequency of intercalations towards the upper part of the cycle. The black shale associations represent the anoxic bottom of the lake, which bears well-preserved plant remains, spinicaudatans, fish scales, some insect remains, and a few stromatolitic calcareous levels (Brauckmann et al., Reference Brauckmann, Gallego, Haushchke, Martins-Neto, Groening, Ilger and Lara2010). In the higher parts of the sequence, sandstone channels and coarse-grained conglomeratic intercalations become frequent (Figs. 2, 3, 4.9), suggesting coarsening-upward trends (upper deltaic facies associations; see Spalletti, Reference Spalletti1997). The last coarsening-upward cycle is interpreted as deltaic mouth-bar deposits and inter-distributary channels of the upper delta plain (Figs. 2, 3, 4.9). Several trunk remains (Figs. 3, 5.3–5.7), as well as plant leaf fragments (Figs. 3, 5.8–5.10) were found in the upper fluvio-deltaic system of the Llantenes Formation (Figs. 2, 3, 5.3–5.7).

Figure 5. Llantenes Formation. (1) Panoramic view of lacustrine deposits (two of the cycles), mouth-bar deposits, and distributary channel fills, indicating progradations of fluvial dominated deltaic lobes of the Llantenes Formation; (2) predominantly sandy part of the deltaic sequence, showing a channel-like sedimentary body that is in part, tabular cross-bedded and is interpreted as proximal delta-front deposits prograding into lacustrine cycles of the Llantenes Formation; (3) large trunk fragments recorded in the uppermost part of the Llantenes outcrops (hammer for scale); (4) trunk intervals of the uppermost part and upper topographic levels of the Llantenes section showing also the panoramic view of the Llantenes Depocenter downwards; (5–7) several in situ pieces of a broken large trunk, uppermost part of the Llantenes outcrops (hammer for scale); (8) impression-compression of Johnstonia stelzneriana (Geinitz, Reference Geinitz1876) Frenguelli, Reference Frenguelli1943; (9) carbonized foliar remains of Dicroidium crassum recorded in upper fluvio-lacustrine cycle of the Llantenes section; (10) carbonized remains of Sphenobaiera sp. recorded in the carbonaceous finely laminated mudstones of the lacustrine environments of the Llantenes Formation. Scale bars: (8, 9) 4 mm; (10) 8 mm.
Materials and methods
In the present work, we have followed the analysis of the lithofacies and the paleoenvironments of the main depositional systems detailed in many previous contributions (e.g., Menéndez, Reference Menéndez1951; Spalletti and Morel, Reference Spalletti and Morel1992; Spalletti, Reference Spalletti1997, among others). A detailed examination of the sedimentary successions was made, emphasizing the lithological characterization of the strata (texture and composition) and the sedimentary structures. In addition, sedimentary sequences recognized allowed us to follow the superposition of the rock bodies and note their vertical lithologic variation and lateral lithofacies distribution. The collection of fossil trunks was carried out because it indicated the different sedimentary facies. In the profile, we identified and located the levels bearing plants previously studied by Menéndez (Reference Menéndez1951), Artabe and Morel (Reference Artabe and Morel1983), and Artabe et al. (Reference Artabe, Morel, Spalletti and Brea1998).
The 78 wood fragments found in the Tronquimalal Group were analyzed using standard petrographic thin-sections along three planes: radial longitudinal (RLS), tangential longitudinal (TLS), and transverse (TS). In addition, a technique based on acetate peels (Galtier and Phillips, Reference Galtier, Phillips, Jones and Rowe1999) was also used. Sixty-four silicified fragments have good cellular preservation, whereas the remaining woods show compressed secondary xylem caused by taphonomic processes, thus the anatomical characters were not preserved. The thin sections were studied in detail with a Leica microscope (DM500) and microphotographs were taken with a digital camera (Leica ICC50) and a scanning electron microscope (SEM Jeol 5800LV) from the Universidad Nacional del Nordeste, Corrientes, Argentina.
Descriptions of woods are according to the list of microscopic features for softwood identification (García Esteban et al., Reference García Esteban, De Palacios, Guindeo Casasús, Lázaro Durán, González Fernández, Rodríguez Salvador, Fernández García, Bobadilla Maldonado and Camacho Atalaya2002, Reference García Esteban, Guindeo Casasús, Pereza Oramas and de Palacios2003; IAWA Committee, Richter et al., Reference Richter, Grosser, Heinz and Gasson2004), and the standard measurements established by Chattaway (Reference Chattaway1932). At least 20 individual measurements of the anatomical characters were recorded, and values were obtained for means, minimum–maximum (e.g., tracheids diameter: 42 (25–66) μm), and minimum–maximum and the maximum included (e.g., ray height: 3–14, 35 cells). Systems of nomenclature and nomenclatural reviews are those of Philippe (Reference Philippe1993), Bamford and Philippe (Reference Bamford and Philippe2001), and Philippe and Bamford (Reference Philippe and Bamford2008). In this paper, we used the terms araucarian, abietinean, and mixed for wood types (based on the tracheid radial pitting), according to the definitions given by Eckhold (Reference Eckhold1921) (sensu Philippe and Bamford, Reference Philippe and Bamford2008). The supra-generic systematic treatment outlined by Anderson et al. (Reference Anderson, Anderson and Cleal2007) was adopted.
Repository and institutional abbreviations
Specimens and microscopic slides are deposited in the paleobotanic collection of the IANIGLA (Instituto Argentino de Nivología, Glaciología y Ciencias Ambientales), CCT-CONICET-MENDOZA under the acronyms IANIGLA-PB and the microscope slide-numbers are given between parentheses (tr: transversal section, lr: radial longitudinal section and lg: tangential longitudinal section).
Systematic paleobotany
Order Pinales Dumortier, Reference Dumortier1829 (=Coniferales Gorozhankin, Reference Gorozhankin1904)
Genus Agathoxylon Hartig, Reference Hartig1848
Type species
Agathoxylon cordaianum Hartig, Reference Hartig1848, p. 188.
Agathoxylon cozzoi new species
Figures 6, 7
- 1951
Araucarioxylon sp. Cozzo in Menéndez, p. 209, pl. 13, figs. 1–3.
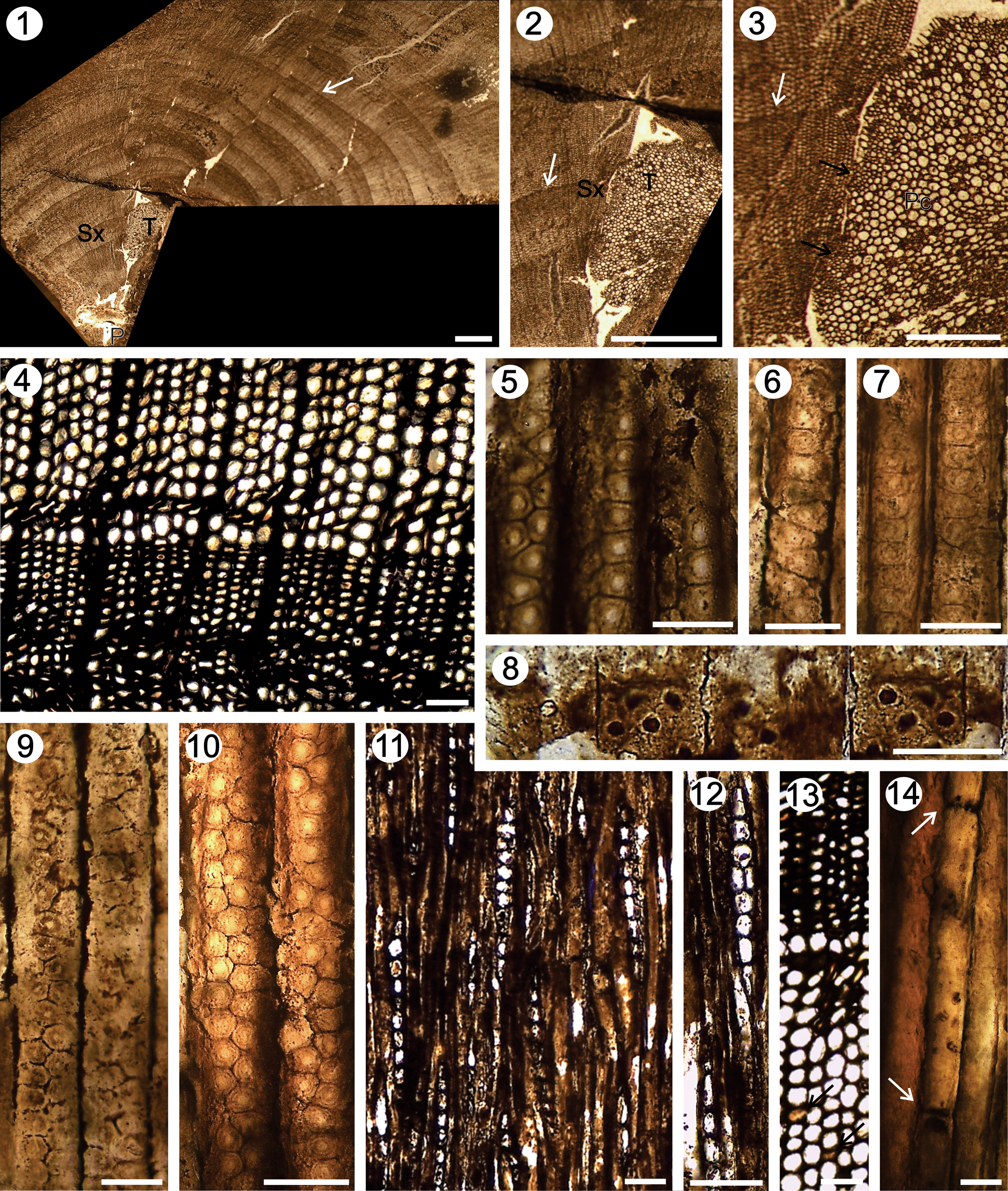
Figure 6. Agathoxylon cozzoi n. sp. (1–3) TS, general view of the petrified wood: P = no preserved pith, T = trace rameal/foliar, SX = secondary xylem, Pc = parenchymatic cells of the trace rameal/foliar, black arrows = primary xylem, white arrows = growth ring; (4) TS, detail of the tracheids and growth ring; (5–7, 9, 10) RLS, pits on tracheid radial walls; (8) RLS, detail of cross-field pits; (11, 12) TLS, detail of the rays; (13) TS, detail of axial parenchyma (black arrows); (14) RLS, detail of axial parenchyma (white arrows). Scale bars: (1, 2) 1.5 mm; (3) 0.75 mm; (4, 11–13) 160 μm; (5–10) 40 μm; (14) 30 μm. IANIGLA PB 794.

Figure 7. Anatomical character schemes of the species identified in the Tronquimalal Group. N = nodular end-wall of the radial parenchyma (juniperoid type); P = horizontal wall of the radial parenchyma distinctly pitted; Rt = ray tracheid; arrows = axial parenchyma of the end-wall: smooth (black) and nodular (white).
Holotype
IANIGLA PB 794 (1–4tr; 5–7lr; 8lg).
Diagnosis
Growth rings distinct. Araucarian wood type. Radial pitting of earlywood tracheids contiguous, predominantly biseriate-hexagonal, uniseriate flattened with biseriate hexagonal-alternate portions and uniseriate-flattened (laterally compressed with a rectangular shape); some bi-triseriate hexagonal-alternate and uniseriate flattened with biseriate square shape and opposite. Radial pitting of latewood tracheids contiguous, uni-biseriate, circular and alternate. Cross-fields pitting araucarioid, two to eight circular pits, frequency range five to six pits. Rays homocellular, mostly uniseriate and the average height is medium. Axial parenchyma diffuse.
Occurrence
Chihuido Formation, type section, Tronquimalal Group, Llantenes Creek, Llantenes Depocenter, south of the Mendoza Province, north-eastern border of the Neuquén Basin, Late Triassic (Norian to ?Rhaetian).
Description
Fragments of secondary xylem of 5 cm in larger diameter and of 2.5 cm in smaller diameter, which consist of 16 growth rings (Fig. 6.1). In cross section, a branch trace extending from the pith through the secondary xylem shows its pith formed by parenchymal cells and some wedges of the primary xylem. The size of the trace is 5 mm × 2 mm (Fig. 6.2, 6.3). The growth rings are distinct, the transition from earlywood to latewood gradual (Fig. 6.2–6.4). The tracheid of the earlywood are rectangular in shape (Fig. 6.4). The radial diameter of tracheids is 41 (25–66) μm, while the tangential diameter is 31 (20–44) μm. Latewood tracheids are rectangular, 16 (14–19) μm in radial diameter, and 27 (26–35) μm in tangential diameter. The mean number of rows of tracheids separating the rays is five, ranging from two to nine (Fig. 6.4). Araucarian wood type: in the earlywood tracheids, the pits are contiguous, biseriate-hexagonal (44.1%), uniseriate flattened (rectangular shape) with biseriate hexagonal-alternate portions (25.1%), uniseriate-flattened (laterally compressed with a rectangular shape) (20.04%), uniseriate-flattened (rectangular shape) with biseriate square shape and opposite (9.73%), and some bi-triseriate hexagonal-alternate (1.03%) (Figs. 6.5–6.7, 6.9, 6.10, 7; Tables 2, 3). In the latewood, the pits are circular, uni-biseriate and alternate. The size of the pit ranges from 15–23 μm × 10–12 μm and 13–17 μm × 10–13 μm (height × width, respectively). The pits have circular aperture of 3–5 μm in diameter (Figs. 6.5–6.7, 6.9, 6.10, 7). The cross-fields are araucarioid, having two to eight circular pits, whose frequency range is five to six pits. The pits measure 10–12 μm in diameter, with circular apertures ranging from 4–6 μm in diameter (Figs. 6.8, 7; Table 3). The rays are homocellular, mostly uniseriate (99%), some uniseriate to partially biseriate in one to three cells (1%). The height varies from two to 10, occasionally up to 20 cells, and the average height is medium (mean: six cells) (Figs. 6.11, 6.12, 7; Table 3). Marginal cells are triangular, whereas central cells are ovoid-rectangular in shape, ranging 25–42 μm in height and 23–30 μm in width. Axial parenchyma diffuse, with smooth transverse walls, cells 234–257 μm in height and 28–30 μm in width (Figs. 6.13, 6.14, 7).
Table. 2. Quantitative data expressed in percentages of Agathoxylon cozzoi n. sp. (A.c), Agathoxylon lamaibandianus (A.l), Protojuniperoxylon ischigualastense (P.i), and Cupressinoxylon llantenesense n. sp. (C.ll). The following anatomy characters are combined: seriation, shape, and disposition of the radial pitting: uniseriate (1): circular-contiguous (C-C), flattened-contiguous (F-C), circular-mixed (C-M), circular-separate (C-S). Uniseriate/biseriate (1/2): circular-alternate (C-A), flattened-hexagonal-alternate (F-H-A), rectangular-opposite (R-O), circular opposite (C-O). Biseriate alternate (2) and biseriate/triseriate (2/3): hexagonal-contiguous (H-C), circular-contiguous (C-C) and Groups (gro).

Table. 3. Quantitative data of the anatomy feature expressed in numbers and average percentages of Agathoxylon cozzoi n. sp. (A.c), Agathoxylon lamaibandianus (A.l), Protojuniperoxylon ischigualastense (P.i), and Cupressinoxylon llantenesense n. sp.

Etymology
In recognition of Agronomist D. Cozzo, who described the first petrified trunks of the area.
Remarks
We compared this species with similar species assigned to Agathoxylon Hartig, Dadoxylon Endlicher, and Araucarioxylon Kraus. The Permian–Triassic species, Dadoxylon parenchymatosum Vogellehner, Reference Vogellehner1965 from South Africa and D. tordoxyloides Vozenin-Serra and Salard-Cheboldaeff, Reference Vozenin-Serra and Salard-Cheboldaeff1992 from Australia share characters with the studied specimen, such as uniseriate-flattened and biseriate (rarely triseriate)-hexagonal, alternate (opposite) pits in the radial wall of the tracheids and presence of axial parenchyma. Both South African and Australian species are distinguished by number and frequency of pits in the cross-fields (up to four) and height of the rays. On the other hand, the specimen herein described is comparable to the Lower Cretaceous species, Dadoxylon mandroense Sah and Jain, Reference Sah and Jain1964 from India, and to Araucarioxylon sp. 1 and Araucarioxylon sp. 2 of Antarctica (Falcon-Lang and Cantrill, Reference Falcon-Lang and Cantrill2001) by the flattened and hexagonal pits on the radial tracheid walls of the earlywood, and by the presence of circular pits on latewood tracheids. Thus, the Mendoza specimen can be distinguished from the mentioned species by cross-field number pits, presence/absence of axial parenchyma, and ray height. In accordance with IAWA (Richter et al., Reference Richter, Grosser, Heinz and Gasson2004) and García Esteban et al. (Reference García Esteban, De Palacios, Guindeo Casasús, Lázaro Durán, González Fernández, Rodríguez Salvador, Fernández García, Bobadilla Maldonado and Camacho Atalaya2002, Reference García Esteban, Guindeo Casasús, Pereza Oramas and de Palacios2003), the cross fields (type, number, and frequency pits) and the axial parenchyma are diagnostic features that justify the assignment of the Chihuido Formation specimen to the new species Agathoxylon cozzoi.
From the upper part of the Chihuido Formation at its type section, D. Cozzo (in Menéndez, Reference Menéndez1951) described Araucarioxylon sp. He characterized and assigned the specimen to the genus by the presence of two, and frequently fewer (one or three) rows of pits on the radial wall tracheids. In the first case, they are alternate, polygonal (hexagonal), or oval, and when there is only one row, they are oval and vertically compressed (flattened). Cross-fields contain three to four (five) pits, with medium to large and circular or oval shape. Rays without radial tracheids (Menéndez, Reference Menéndez1951, p. 209–210). Thus, we include the specimens that Cozzo (in Menéndez, Reference Menéndez1951) identified as Araucarioxylon sp. in A. cozzoi n. sp.
The new species is differentiated from A. lamaibandianus, described also in this contribution and recorded in both Chihuido and Llantenes formations, mainly by the shape and arrangement of the radial pitting, the number of pits in the cross-fields, presence of axial parenchyma, and the height of the rays (Figs. 6.5–6.8, 7, 8.3–8.10, 9.1–9.4, 13; Tables 2, 3).
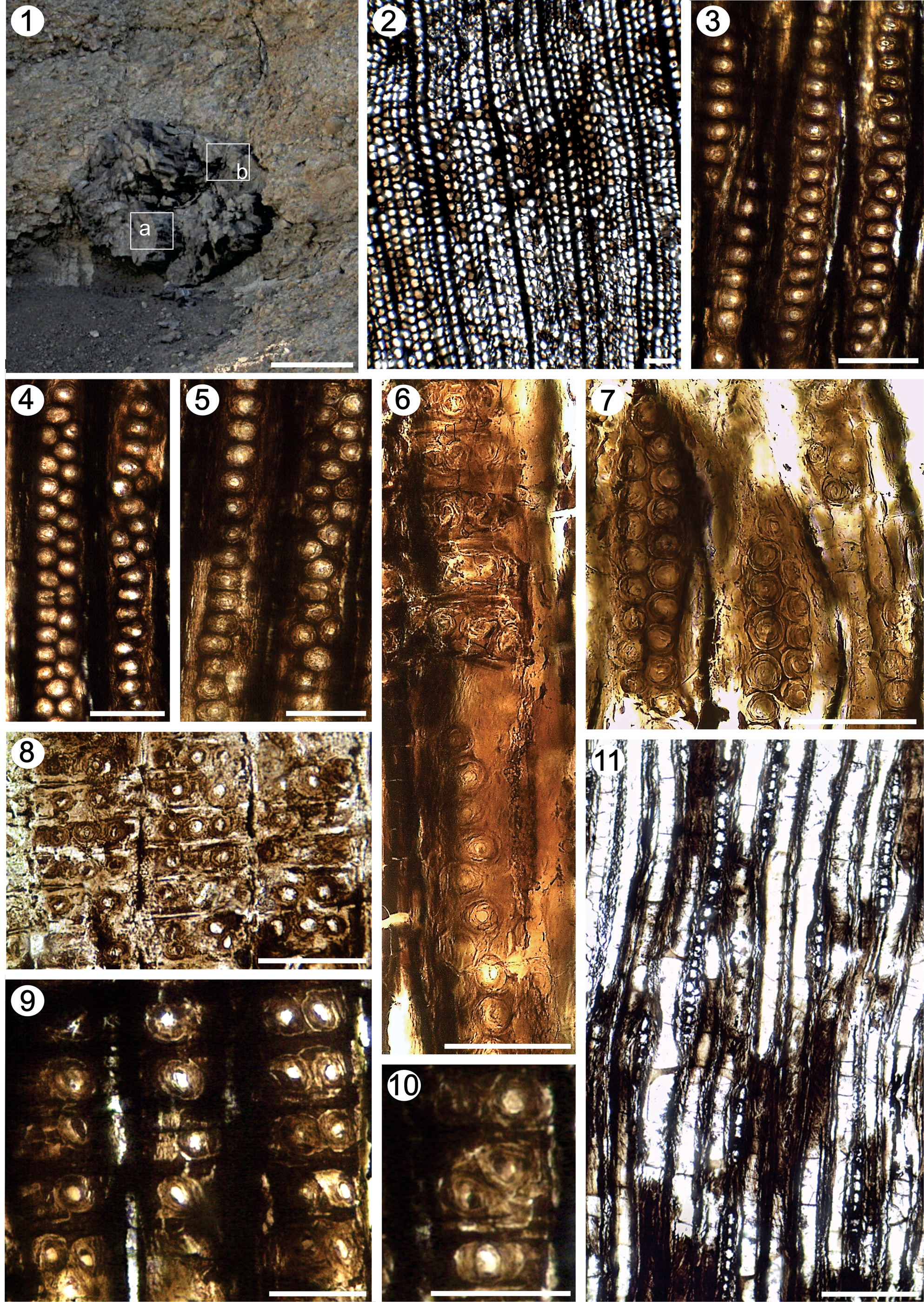
Figure 8. Agathoxylon lamaibandianus. (1) General view of the petrified wood from Chihuido Formation, a = fragments near the central pith (IANIGLA PB 792b), and b = fragments from the periphery of the log (IANIGLA PB 792a); (2) TS, detail of the tracheids; (3–7) RLS, pits on tracheid radial walls; (6, 8–10) RLS, detail of cross-field pits; (11) TLS, detail of the rays. Scale bars: (1) 45 cm; (2) 200 μm; (3–10) 50 μm; (11) 150 μm. (2) IANIGLA PB 786. (6, 7) IANIGLA PB 792b. (3–5, 8–11) IANIGLA PB 783.
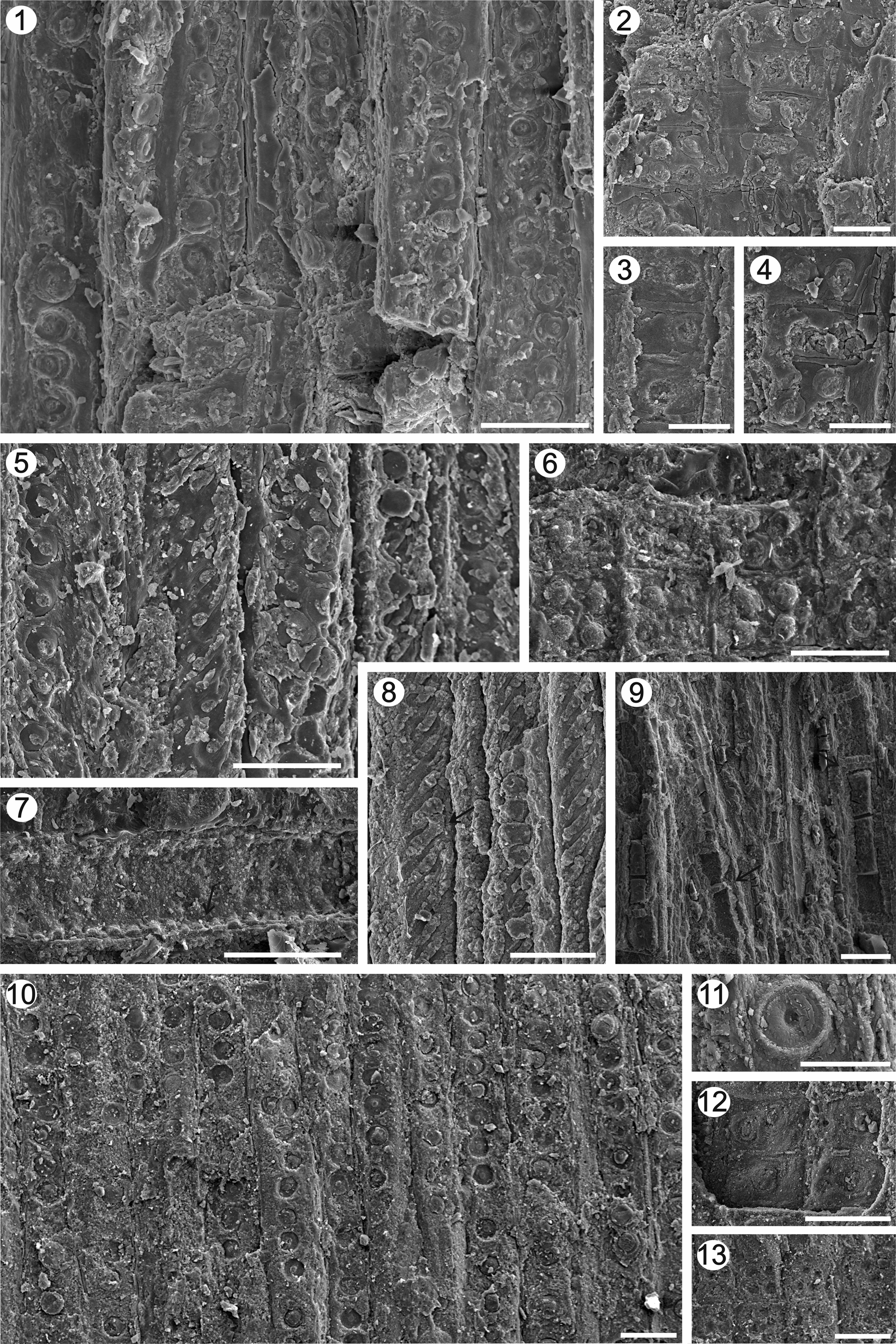
Figure 9. (1–4), Agathoxylon lamaibandianus. (1), RLS, pits on tracheid radial wall; (2–4) RLS, detail of cross-field pits. (5–9), Protojuniperoxylon ischigualastense. (5), RLS, pits on tracheid radial wall; (6) RLS, detail of cross-field pits; (7), RLS, detail of the horizontal walls, pitted of the radial parenchyma (arrow); (8), RLS, spiral-like structure and helical grooves in the macrofibrils of the secondary walls (S2) of the tracheids (arrow); (9), TLS, detail of the axial parenchyma (arrows). (10–13), Cupressinoxylon llantenesense n. sp. (10), RLS, pits on tracheid radial walls; (11), RLS, detail of radial bordered pits and torus-margo thick membranes; (12, 13) RLS, detail of cross-field pits. Scale bars: (1) 50 μm; (2–4) 25 μm; (5–9) 40 μm; (10, 12, 13) 45 μm; (11) 20 μm. (1, 2) IANIGLA PB 792b; (3–5, 8) IANIGLA PB 745; (6, 7, 9) IANIGLA PB 764; (10–12) IANIGLA PB 767; (13) IANIGLA PB 753a.
Agathoxylon lamaibandianus Crisafulli and Herbst, Reference Crisafulli and Herbst2011
Figures 7, 8, 9.1–9.4
- 2010
Agathoxylon dallonii (Boureau) Crisafulli and Herbst; Crisafulli and Herbst, p. 15, fig. 2A–C.
- 2010
Prototaxoxylon intertrappeum Prakash and Srivastava; Crisafulli and Herbst, p. 17, figs. 3F, 4A–D.
- 2011
Agathoxylon lamaibandianus Crisafulli and Herbst, p. 276, fig. 2.1–2.6.
- 2011
Agathoxylon dallonii (Boureau) Crisafulli and Herbst; Crisafulli and Herbst, p. 278, fig. 3.1–3.4
Holotype
CTES PB 14036. CTES PMP: 2964, 2965 and 2966 from Laguna Colorada Formation, Norian, Santa Cruz Province, Patagonia, Argentina (Crisafulli and Herbst, Reference Crisafulli and Herbst2011, p. 276–278, fig. 2.1–2.6).
Description
Fragments of secondary xylem from two large trunks, ~65 cm × 110 cm in diameter are found in the Chihuido Formation (Figs. 4.1, 8.1) and nine fragments were found in the Llantenes Formation. The growth rings are distinct, the transition from earlywood to latewood gradual. The tracheids are rectangular-quadrangular in shape. The radial diameter of tracheids is 50 (33–70) μm, while the tangential diameter is 36 (24–55) μm. The mean number of tracheids separating rays is four, with a range of two to six rows (Fig. 8.2). Araucarian wood type: in the radial wall of tracheids the pits are bordered, mainly uniseriate, circular (47.34% [42.85–57.59%]) and rarely flattened (2.95% [0.98–5.7%]). Other pits are uniseriate with biseriate portions alternate (17.78% [13.96–20%]) and rarely opposite (8.01% [7.14–9.1%]). The biseriate are alternate (15.02% [11.04–21.01%]), sometimes groups of three to six pits are recognized; they start and end with a single pit (6.21% [3.58–8.58%]) (Figs. 7, 8.3–8.7, 9.1; Tables 2, 3). In addition, some parts of the wall of the tracheids without pits can be observed. Pit diameters are 16–25 μm, with the diameter of circular aperture 5–15 μm. The cross-fields show one to four pits, arranged in araucarioid type, whose frequency ranges from one to three pits (Figs. 7, 8.6, 8.8–8.10, 9.2–9.4; Table 3). These are 18–20 μm in size, with circular apertures 6–8 μm in diameter, or elliptical apertures 9 × 5 μm and 10 × 6 μm. From the two large trunks of the Chihuido Formation, fragments near the central pith (IANIGLA PB 792b, 793b), fragments from the periphery of the log (IANIGLA PB 792a, 793a) (Fig. 8.1), and numerous fragments of the Llantenes Formation have been analyzed. These show minimal variability in the frequency of the anatomical features, such as radial pitting seriation and cross-field pits (Tables 2, 3). The rays are homocellular, uniseriate. Ray height varies from 3–20, 30 cells, the average height is medium (mean: 12 cells) (Figs. 7, 8.11, Table 3). Marginal cells are triangular, while central cells are rectangular in shape, 20–27 μm in height and 11–15 μm in width.
Materials
Chihuido Formation: IANIGLA PB 792a (1–2lr), IANIGLA PB 792b (1tr; 2lr), IANIGLA PB 793a (1–2tr; 3–4lr; 5–9lg), IANIGLA PB 793b (1–4tr; 5–7lr; 8lg). Llantenes Formation: IANIGLA PB 771 (1–2tr; 4–7lr; 8–9lg), IANIGLA PB 772 (1tr; 2–3lr; 4lg), IANIGLA PB 781 (1tr; 2–5lr), IANIGLA PB 782 (1tr; 2–5lr), IANIGLA PB 783 (1–8lr), IANIGLA PB 784 (1tr; 2–4lr; 5–6lg), IANIGLA PB 785 (1tr; 2–3lr; 4–6lg), IANIGLA PB 786 (1–3tr; 4–11lr; 12–13lg), IANIGLA PB 833 (1–3tr; 4–5lr; 7lg).
Remarks
From comparisons made with most fossil species of the genera Araucarioxylon, Dadoxylon, and Agathoxylon, the diagnostic characters of the Chihuido and Llantenes specimens allow their assignment to Agathoxylon lamaibandianus (Gnaedinger et al., Reference Gnaedinger, Garcia Massini, Becchis and Zavattieri2015; Kloster and Gnaedinger, Reference Kloster and Gnaedinger2018). This species has been characterized by araucarian wood with uniseriate to biseriate, alternate, circular, and flattened tracheid radial pitting, rays of 3–23 cells in height, cross-fields with one to four cupressoid pits arranged in araucarioid pattern (Crisafulli and Herbst, Reference Crisafulli and Herbst2011).
Agathoxylon lamaibandianus is similar to A. allanii (Kraüsel, Reference Kräusel and Plumstead1962) Gnaedinger and Herbst, Reference Gnaedinger and Herbst2009; A. protoaraucana (Brea, Reference Brea1997) Gnaedinger and Herbst, Reference Gnaedinger and Herbst2009; A. termieri (Attims, Reference Attims1965) Gnaedinger and Herbst, Reference Gnaedinger and Herbst2009; and A. liguaensis Torres and Philippe, Reference Torres and Philippe2002. However, A. lamaibandianus is distinguished by seriation and distribution of radial pitting and number of pits in the cross-fields, whereas A. liguaensis is distinguished by ray height (Gnaedinger et al., Reference Gnaedinger, Garcia Massini, Becchis and Zavattieri2015; Kloster and Gnaedinger, Reference Kloster and Gnaedinger2018).
The anatomical features of the secondary xylem in the analyzed specimens are identical to those of Agathoxylon malaimbandense (Marguerier, Reference Marguerier1976) Gnaedinger and Herbst (Reference Gnaedinger and Herbst2009) described from the Permian–Triassic of Malaimbandi, Madagascar Island (south-eastern Africa) by Marguerier (Reference Marguerier1976). According to Crisafulli and Herbst (Reference Crisafulli and Herbst2011), the material type of A. malaimbandense has preserved the pith, and thus should not be included in Agathoxylon, which is a genus that lacks pith as diagnostic character.
Crisafulli and Herbst (Reference Crisafulli and Herbst2010, Reference Crisafulli and Herbst2011) described A. dallonii (Boureau, Reference Boureau1948) from the Llantenes Formation and from the Laguna Colorada Formation (Late Triassic of El Tranquilo Group, Patagonia) and Prototaxoxylon intertrappeum from the Llantenes Formation. Both taxa are herein included as synonyms of A. lamaibandianus because they have uni-biseriate circular radial pitting and 1–2 pits in the cross-fields as frequent features.
Agathoxylon lamaibandianus is distinguished from the holotype A. dallonii described from the Jurassic–Cretaceous of Africa by the cross-fields with large or elliptical simple pit (Boureau, Reference Boureau1948; Duperon-Laudoueneix and Lejal-Nicol, Reference Duperon-Laudoueneix and Lejal-Nicol1981). On the other hand, A. lamaibandianus differs from the holotype of P. intertrappeum described from the Upper Cretaceous by the high number of pits in the cross-fields as well as the seriation and height of the rays (Prakash and Srivastava, Reference Prakash and Srivastava1961; Gnaedinger and Herbst, Reference Gnaedinger and Herbst2006).
In the Chihuido Formation, Menéndez (Reference Menéndez1951) gave the main characteristics of Araucarioxylon sp., based on the descriptions made by D. Cozzo, and herein we assign and describe the Chihuido specimen as a new species, Agathoxylon cozzoi. It is distinguished from A. lamaibandianus by the shape of radial pitting, axial parenchyma, and by the number of pits in the cross-fields (Figs. 6.5–6.8, 7, 8.3–8.10, 9.1–9.4; Tables 2, 3).
Genus Protojuniperoxylon Eckhold, Reference Eckhold1921
Type species
Protojuniperoxylon maidstonense (Stopes, Reference Stopes1915) Eckhold, Reference Eckhold1921.
Protojuniperoxylon ischigualastense (Bonetti, Reference Bonetti1966) Bodnar and Artabe, Reference Bodnar and Artabe2007
Figures 7, 9.5–9.9, 10, 11

Figure 10. Protojuniperoxylon ischigualastense. (1) General view of petrified wood from Llantenes Formation; (2) TS, detail of growth ring and tracheid; (3, 4) RLS, pits on tracheid radial walls; (5–8) RLS, detail of cross-field pits; (6) RLS, detail of axial parenchyma (arrows); (9), TLS, detail of the rays; (9, 10) TLS, detail of the nodular end-wall of the radial parenchyma (juniperoid thickening); (11, 12) RLS, detail of the nodular (juniperoid thickening) end wall (white arrow) and pitted horizontal walls of the radial parenchyma cells (black arrows); (13) TS, the end wall of the radial parenchyma cells nodular (juniperoid thickening) (white arrow) and pitted horizontal walls of the radial parenchyma cells (black arrow); (14) RLS, the end wall of the radial parenchyma cells nodular (juniperoid thickening) (arrow). Scale bars: (1) 2.5 cm; (2) 140 μm; (3–5, 7, 8, 11–14) 40 μm; (6) 80 μm; (9)110 μm; (10) 30 μm. (1) IANIGLA PB 775; (2, 3, 5–8, 12) IANIGLA PB 763; (4, 9–11, 13, 14) IANIGLA PB 742.

Figure 11. Protojuniperoxylon ischigualastense. (1–3) RLS, heterocellular rays: detail of the radial tracheids (white arrows) (IANIGLA PB 745); (4–6, 8) rameal and/or foliar traces: (4) TLS, parenchyma cells (IANIGLA PB 764); (6) RLS, pith and preserved secondary xylem with growth rings (black arrow) (IANIGLA PB 775); (5, 8) RLS, primary xylem (white arrows) and parenchyma cells (black arrow) in TLS (IANIGLA PB 777); (7) RLS, spiral-like structure (arrow) (IANIGLA PB 745); (9) TLS, detail of the rays (IANIGLA PB 745). Scale bars: (1–3, 7, 8) 50 μm; (4) 120 μm; (5) 80 μm; (6) 1 mm; (9) 130 μm.
Holotype
LPPB 4375 (LPPM 367, 368 and 369) from the Ischigualasto Formation, early-Late Triassic, San Juan Province, Argentina (Bonetti, Reference Bonetti1966; Bodnar and Artabe, Reference Bodnar and Artabe2007).
Description
Fragments of secondary xylem with distinct growth rings and abrupt transition from earlywood to latewood (Fig. 10.1, 10.2). Earlywood tracheids are quadrangular to rectangular in shape (Fig. 10.2). The radial diameter of tracheids is 49 (30–78) μm, while the tangential diameter is 41 (28–53) μm. Latewood tracheids are rectangular, 19 (16–26) μm in radial diameter and 30 (26–32) μm in tangential diameter. The mean number of tracheids separating the rays is five, with a range of 2–12 rows (Fig. 10.2). Mixed wood type: in the radial wall of tracheids the pits are bordered, circular (96.29%), and occasionally flattened (3.71%), contiguous (53.01%), contiguous and separate (32.46%), and separate (14.53%). The radial pittings are uniseriate contiguous, circular (29.66% [22.23–34.78%]) and flattened (3.17% [0–4.34%]), mixed (14.85% [7.4–21.05%]) and separate (14.53% [8.69–26.31%]). Other radial pittings are uniseriate with biseriate portions alternate (9.55% [5.29–18.5%]) and opposite (17.61% [10.52–22.11%]), and biseriate alternate (10.06% [5.26–14.2%]) (Figs. 7, 9.5, 10.3, 10.4; Tables 2, 3). Pit diameters are 14–21 μm, with circular aperture 4–5 μm in diameter. Cross-fields are cupressoid with two to six cupressoid pits, frequency ranges two to four, arranged in one and two horizontal rows of pits (Figs. 7, 9.6, 10.5–10.8; Table 3). These are 13–17 μm in size with circular or elliptical apertures 7–9 μm in diameter. The radial system is heterogeneous with homocellular and some heterocellular rays. Rays are uniseriate (43.26%), uniseriate partially biseriate in one to eight cells (35.13%), biseriate (16.21%), and rarely triseriate (5.4%) (Figs. 7, 10.9, 10.10, 11.9; Table 3). The height varies from 2–32, 56 cells, the average height is high (mean: 16 cells) (Table 3). Marginal cells are triangular and central cells are ovoid-rectangular, 26–39 μm in height and 23–30 μm in width. The radial parenchyma cells with distinctly pitted horizontal and end walls nodular (juniperoid thickening) (Figs. 7, 10.9–10.14). The radial tracheids are located in the middle part of the rays or in their extremes (Figs. 7, 11.1–11.3). Axial parenchyma with diffuse arrangement. The transverse end walls of axial parenchyma cells are nodular. The parenchyma cells have dark content and the size range from 95–130 μm high and 17–21 μm width (Figs. 7, 9.9, 10.6).
In the specimens IANIGLA PB 744–747, the horizontal walls pitted and the end nodular (juniperoid thickenings) walls of the ray parenchyma cells are not observed, probably due to the removal of the tracheid and ray walls caused by fungal attack and evidenced by the presence of fungal hyphae in the tracheids and rays. These materials are included in this species by coincidence with other anatomical diagnostic characters (e.g., Fig. 11.1–11.3, 11.9; Table 2). Also, some other specimens (IANIGLA PB 744–745) show spiral-like structure orientated with an angle of 45°, with Z-shaped and helical grooves in the microfibrils of the secondary walls (S2) of the tracheids (Figs. 9.8, 11.7). These features may be caused by the compression wood produced in branches, in trees that grew in slopes with pronounced steepness, subjected to strong winds, or any other kind of stress conditions (Yoshizawa et al., Reference Yoshizawa, Itoh and Shimaji1982, Reference Yoshizawa, Itoh and Shimaji1985; García Esteban et al., Reference García Esteban, Guindeo Casasús, Pereza Oramas and de Palacios2003; Burgert et al., Reference Burgert, Fruhman, Keckes, Fratzl and Stanzl-Tschegg2004; Schweingruber et al., Reference Schweingruber, Börner and Schulze2006; Schweingruber, Reference Schweingruber2007). It can also be the product of decay processes by fungi (García Esteban et al., Reference García Esteban, Guindeo Casasús, Pereza Oramas and de Palacios2003; Schweingruber et al., Reference Schweingruber, Börner and Schulze2006; Schweingruber, Reference Schweingruber2007).
Rameal and/or foliar traces
In TLS, the traces are rhomboidal in shape, measuring 938 μm high × 318 μm wide formed by parenchymatic cells, circular-oval in the transverse section of 16–25 μm diameter (Fig. 11.4: IANIGLA PB 764). In other larger traces of 3.5 cm high × 2.5 cm wide, it is constituted by pith and preserved secondary xylem with five growth rings (Figs. 10.1, 11.6: IANIGLA PB 775). In RLS, parenchyma cells 79–116 μm high × 35–63 μm wide were observed and the tracheids of the primary xylem with scalariform thickening are 24–26 μm in width (IANIGLA PB 745). In another specimen, the trace of 830 × 491 μm shows primary xylem in the longitudinal section with tracheids 17–25 μm wide, and in TLS section parenchyma cells of circular-polygonal shape and filled by opaque content are 20–25 μm in diameter (Fig. 11.2, 11.5, 11.8: IANIGLA PB 777).
Materials
Llantenes Formation: IANIGLA PB 742 (4tr; 5–7lr; 8lg), IANIGLA PB 744 (1tr; 2–3lr; 4lg), IANIGLA PB 745 (1–3tr; 4–5lr; 6–8lg), IANIGLA PB 746 (1–6tr; 7–12lr; 13–14lg), IANIGLA PB 747 (1tr; 2lr; 3–4lg), IANIGLA PB 748, IANIGLA PB 749 (1–2tr; 3–7lr; 8lg), IANIGLA PB 750 (1–2lr), IANIGLA PB 751 (1–2lr), IANIGLA PB 752, IANIGLA PB 754 (1tr; 2–4lr; 5lg), IANIGLA 755 (1-5tr; 6-9lr; 10lg), IANIGLA PB 756 (1tr; 2–4lr; 5lg), IANIGLA PB 757 (1–2tr; 3lr; 4lg), IANIGLA PB 759 (1tr; 2–6lr), IANIGLA PB 761 (1–3tr; 4–6lr; 7lg), IANIGLA PB 762 (1tr; 2–4lr; 5lg), IANIGLA PB 763 (1–3lr), IANIGLA PB 764 (1–6lr; 7–12lg), IANIGLA PB 775 (1–5tr; 6–9lr;10lg), IANIGLA PB 777 (1–2tr; 3–10lr; 11lg), IANIGLA PB 778, IANIGLA PB 787 (1–3tr; 4–5lr, 6-7lg), IANIGLA PB 788 (1tr; 2–4lr); IANIGLA PB 817, IANIGLA PB 818, IANIGLA PB 819.
Remarks
The analyzed specimens are identified with Protojuniperoxylon by the following main diagnostic features: araucarian to araucarian-abietinean (mixed) tracheid radial pitting, horizontal walls of ray parenchyma cells distinctly pitted and end walls of ray parenchyma cells nodular (juniperoid thickenings) (Eckhold, Reference Eckhold1921; Bonetti, Reference Bonetti1966; Bodnar and Artabe, Reference Bodnar and Artabe2007; Philippe and Bamford, Reference Philippe and Bamford2008).
Protojuniperoxylon includes two species to date, P. maidstonense (Stopes) Eckhold (type species) and P. ischigualastense (Bonetti) Bodnar and Artabe. By the presence of the anatomical features, such as radial pitting seriation and number of pits in the cross-fields, the Llantenes Formation specimens are identified as P. ischigualastense.
Genus Cupressinoxylon Göppert, Reference Göppert1850 (nomen conservandum, see Bamford et al., Reference Bamford, Zijlstra and Philippe2002)
Type species
Cupressinoxylon gothani Kräusel, Reference Kräusel1920.
Cupressinoxylon llantenesense new species
Figures 7, 9.10–9.13, 12.1–12.15

Figure 12. Cupressinoxylon llantenesense n. sp. (1) General view of petrified wood from Llantenes Formation; (2) TS, detail of the tracheids; (3–7) RLS, pits on tracheid radial walls; (3–10) detail of the torus-margo pit thick membranes (black arrows) (2–7, 9) RLS, (8) TS, (10) TSL; (11, 12) RLS, detail of cross-field pits (arrow); (13, 14) TLS, detail of the rays (torus-margo pit thick membranes = black arrows); (15) TLS, detail axial parenchyma. Scale bars: (2, 13) 180 μm; (3–9, 11–12) 45 μm; (10) 30 μm; (14) 100 μm; (15) 90 μm. (2, 8) IANIGLA PB 767; (3–6, 9–11, 13, 14) IANIGLA PB 769c; (7, 12, 15) IANIGLA PB 765.
Holotype
IANIGLA PB 769 (1tr; 2–5lr; 6 lg).
Paratype
IANIGLA PB 765 (1–4tr; 5–9lr; 10–12lg).
Diagnosis
Secondary xylem with distinct growth rings. Abietinean wood type. Radial pitting of tracheids bordered, circular and separate (rarely contiguous), predominantly uniseriate, some uniseriate with biseriate portions opposite. The torus-margo pit with thick membrane present. Tangential wall of tracheids uniseriate and separate pits. Cross-fields cupressoid-type, in one and two horizontal rows of pits (sensu Richter et al., Reference Richter, Grosser, Heinz and Gasson2004) with one to four cupressoid pits, frequency range is two to four per field. Rays uniseriate, uniseriate to partially biseriate, and rarely biseriate, and the average height is medium. Axial parenchyma diffuse and scarce.
Occurrence
Upper part of the Llantenes Formation (Tronquimalal Group), in the type section, Llantenes Creek, Llantenes Depocenter, south of the Mendoza Province, north-eastern border of the Neuquén Basin, Late Triassic (Norian to ?Rhaetian).
Description
Fragments of secondary xylem with distinct growth ring boundaries and abrupt transition from early to latewood (Fig. 12.1, 12.2). The earlywood tracheids are quadrangular-rectangular in shape. The radial diameter of tracheids is 44 (26–60) μm, while the tangential diameter is 46 (21–80) μm. Latewood tracheids are rectangular, 15 (12–18) μm in radial diameter and 35 (24–46) μm in tangential diameter. The mean number of tracheids separating the rays is four, with a range of 2–10 rows (Fig. 12.2). Abietinean wood type: in the radial wall of tracheids the pits are bordered, circular, and separate (70.62% [66.22–76.36%]); mixed (15.28% [11.45–17.36%]); and rarely contiguous (7.37% [5.45–8.63%]); predominantly uniseriate (93.27%), some being uniseriate with biseriate sections opposite (6.71% [1.83–13.15%]) (Figs. 7, 9.10, 9.11, 10.13, 10.14, 12.3–12.10; Tables 2, 3). The pits have circular and elliptical aperture and torus-margo thick membrane. Pit diameters 16–22 μm with circular aperture of 4–5 μm in diameter and elliptical aperture of 12 × 4 μm. Cross-fields show one to four cupressoid pits, with frequency ranges from two to four, arranged in cupressoid type, in one and two horizontal rows of pits, sensu Richter et al. (Reference Richter, Grosser, Heinz and Gasson2004) (Figs. 7, 9.12, 9.13, 12.3, 12.11, 12.12; Table 3). These are 10–14 μm in size with elliptical apertures of 8 × 4 μm (height × width) and circular ones of 4 μm in diameter. The tangential wall of tracheids have uniseriate and separate pits. Rays homocellular, mainly uniseriate (83.64%), some being uniseriate to partially biseriate (12.73%), and rarely biseriate (3.64%), having 1–13, 22 cells high and the average height is medium (mean: 5) (Table 3). The central cells are rectangular, 21–31 μm high and 22–26 μm wide. The cell extremes have triangular to elliptic contours, 30 μm high and 19 μm wide (Figs. 7, 12.13, 12.14). Axial parenchyma is diffused and scarce and with smooth terminal walls (Figs. 7, 12.15).
Etymology
In reference to the Llantenes Formation, Mendoza, Argentina, where the analyzed wood was found.
Materials
Llantenes Formation: IANIGLA PB 740 (1–2tr; 3–14lr; 15–16lg), IANIGLA PB 741 (1–2tr; 3–8lr), IANIGLA PB 743 (1–2tr; 3–4lr; 5–6lg), IANIGLA PB 753 (1–3tr; 4–8lr; 9–12lg), IANIGLA PB 766 (1–2tr; 3–4lr), IANIGLA PB 767 (1tr; 2–3lr; 4lg), IANIGLA PB 768 (1–2lr), IANIGLA PB 770 (1–2tr; 3–6lr; 7–9lg), IANIGLA PB 773 (1–2tr), IANIGLA PB 774 (1lr; 2–3lg), IANIGLA PB 776 (1–2tr; 3–6lr; 7lg), IANIGLA PB 779 (1tr; 2lr; 3–4lg), IANIGLA PB 789 (1–2tr; 3–4lr), IANIGLA PB 790 (1–2lr). IANIGLA PB 815 (1-5lr), IANIGLA PB 816 (1-ct; 2lr), IANIGLA PB 820, IANIGLA PB 821, IANIGLA PB 824 (1-6lr), IANIGLA PB 825, IANIGLA PB 834.
Remarks
The anatomical features of the described specimens like radial pitting circular and uniseriate to biseriate (abietinean), presence of axial parenchyma, low and uniseriate homocellular rays, and cupressoid cross-fields indicate that the fossil is a conifer with abietinean secondary structure and assigned to Cupressinoxylon according to Göppert (Reference Göppert1850) (see Philippe and Bamford, Reference Philippe and Bamford2008).
By comparisons made between species of Cupressinoxylon (Vaudois and Privé, Reference Vaudois and Privé1971; Kłusek, Reference Kłusek2014; Bodnar et al., Reference Bodnar, Ruiz, Artabe, Morel and Ganuza2015; Brea et al., Reference Brea, Bellosi, Umazano and Krause2016; Ruiz et al., Reference Ruiz, Brea, Raigemborn and Matheos2017), the specimens of the Triassic in Argentina are closely related with those species that share the parenchyma axial and number of one to four pits in the cross-fields. These species are: C. kotaense Rajanikanth and Sukh-Dev, Reference Rajanikanth and Sukh-Dev1989 (Jurassic, India); Cupressinoxylon sp. (Cretaceous, Argentina); C. hallei Kraüsel, Reference Kraüsel1924 (Cretaceous and Cenozoic, Argentina and Antarctica); C. gothani Kraüsel, Reference Kräusel1920 (Miocene, Polonia); C. canadense (Schroeter, Reference Schroeter1880) Kraüsel, Reference Kräusel1949 (=C. wellingtonioides [Prill, Reference Prill1913] Kraüsel, Reference Kräusel1949) (Miocene, Romania and Polonia); and C. kostyniukii Dolezych and Schneider, Reference Dolezych and Schneider2006 (Miocene, Germany) (Kraüsel, Reference Kraüsel1919; Kownas, Reference Kownas1951; Rajanikanth and Sukh-Dev, Reference Rajanikanth and Sukh-Dev1989; Dolezych and Van der Burgh, Reference Dolezych and Van der Burgh2004; Dolezych and Schneider, Reference Dolezych and Schneider2006; Kłusek, Reference Kłusek2014; Pujana et al., Reference Pujana, Santillana and Marensi2014; Brea et al., Reference Brea, Bellosi, Umazano and Krause2016; Egerton et al., Reference Egerton, Williams and Lacovara2016). The specimens of the Llantenes Formation are distinguished from species of Cupressinoxylon mentioned above by the arrangement of tracheid radial pitting, presence of the crassulae, lack of torus in the pits, axial parenchyma wall nodular, and by the height of the rays. For all these reasons, the new species Cupressinoxylon llantenesense is established.
The Cupressinoxylon species in the early Mesozoic strata are scarce. They were recorded in Triassic–?Jurassic as Cupressinoxylon sp. of Argentina (Bodnar and Falco, Reference Bodnar and Falco2018) and in Jurassic–?Cretaceous as C. kotaense Rajanikanth and Sukh-Dev, C. (Taxodioxylon) rajmahalense Bhardwaj, Reference Bhardwaj1953 (Bhardwaj, Reference Bhardwaj1953; Rajanikanth and Sukh-Dev, Reference Rajanikanth and Sukh-Dev1989) from India, and C. jurassica Lutz, Reference Lutz1930 from North America (Lutz, Reference Lutz1930). Cupressinoxylon llantenesense n. sp. is differentiated by the set of diagnostic features described above.
Discussion
Anatomy and taxonomic considerations
The combination of anatomical characters, such as wood type (shape, seriation, and arrangement of the tracheid radial pitting), cross-fields type, rays height, and the presence of axial parenchyma are useful for identification (Greguss, Reference Greguss1955; Boureau, Reference Boureau1956; García Esteban et al., Reference García Esteban, De Palacios, Guindeo Casasús, Lázaro Durán, González Fernández, Rodríguez Salvador, Fernández García, Bobadilla Maldonado and Camacho Atalaya2002, Reference García Esteban, Guindeo Casasús, Pereza Oramas and de Palacios2003; Richter et al., Reference Richter, Grosser, Heinz and Gasson2004). Anatomical characteristics of the woods were quantified in accordance with criteria proposed by Falcon-Lang and Cantrill (Reference Falcon-Lang and Cantrill2000), Poole and Cantrill (Reference Poole and Cantrill2001), Gnaedinger (Reference Gnaedinger2012), Gnaedinger et al. (Reference Gnaedinger, Garcia Massini, Becchis and Zavattieri2015), and Kloster and Gnaedinger (Reference Kloster and Gnaedinger2018). Tables 2 and 3 show percentage differences between specimens of the same species and compare the four taxa identified in the Tronquimalal Group. Plotted dispersion graphics based on quantitative data for each specimen (Tables 2, 3) clearly reflect the differences between identified genera and species where these species show single entities in “morphology space” (Falcon-Lang and Cantrill, Reference Falcon-Lang and Cantrill2000, Reference Falcon-Lang and Cantrill2001; Gnaedinger, Reference Gnaedinger2012; Gnaedinger et al., Reference Gnaedinger, Garcia Massini, Becchis and Zavattieri2015; Kloster and Gnaedinger, Reference Kloster and Gnaedinger2018) (Tables 2, 3). Thus, the taxa can be distinguished by their different percentages of tracheid radial pitting shapes and distribution (seriation) (araucarian, mixed, and abietinean wood), as well as by the range and mean number of pits in the cross-fields. Tables 2 and 3 show three wood types corresponding to Agathoxylon, Protojuniperoxylon, and Cupressinoxylon. These conifers and conifer-like gymnosperm wood types are distinguished for the Mesozoic based on tracheid radial pitting (Eckhold, Reference Eckhold1922; Bamford and Philippe, Reference Bamford and Philippe2001). The araucarian wood type (Group B in Philippe and Bamford, Reference Philippe and Bamford2008) is characteristic of the Paleozoic gymnosperms and in the extant Araucariaceae, whereas the abietinean wood type (Group C in Philippe and Bamford, Reference Philippe and Bamford2008) is widely encountered in other families of the conifers from Cretaceous to the Present (Bamford and Philippe, Reference Bamford and Philippe2001). The third wood type is mixed (Group D in Philippe and Bamford, Reference Philippe and Bamford2008), having contiguous, mixed, and separate radial pitting, and is commonly (but not exclusively) recorded in Jurassic to Lower Cretaceous conifers (Boureau, Reference Boureau1956; Lepekhina, Reference Lepekhina1972; Bamford and Philippe, Reference Bamford and Philippe2001; Philippe and Bamford, Reference Philippe and Bamford2008). In the Chihuido Formation, two araucarian wood type (Agathoxylon lamaibandianus and A. cozzoi n. sp.) are described, but in the Llantenes Formation, araucarian (A. lamaibandianus), abietinean (Cupressinoxylon llantenesense n. sp.), and mixed (Protojuniperoxylon ischigualastense) wood types are herein identified (Figs. 7, 13.2, 13.3; Tables 2, 3).

Figure 13. (1, 2) Plotted dispersion graphics based on quantitative data (in percentages) (Table 1) of specimens for Agathoxylon cozzoi n. sp. (A.c), Agathoxylon lamaibandianus (A.l), Protojuniperoxylon ischigualastense (P.i), and Cupressinoxylon llantenesense n. sp. (C.ll). (1) Percentages of the seriation, shape, and disposition of the radial pitting; (2) percentages of the uniseriate radial pitting contiguous and separate (araucarian, mixed, and abietinean wood type). (3, 4) Plotted dispersion graphics based on average percentages (Table 2) from species; (3) abietinean versus araucarian wood type; (4) uniseriate radial pitting shape: circular versus flattened.
Agathoxylon lamaibandianus and A. cozzoi n. sp. have a secondary araucarian structure type and cross-fields of the araucarioid type (Tables 2, 3; Fig. 13). Both species are distinct because the first one has circular-flattened radial pitting and cross-fields with one to four pits (the greater frequency being one to two pits), while A. cozzoi n. sp. shows flattened, hexagonal radial pitting and cross-fields with three to eight pits (greater frequency of five to six pits) (Figs. 6, 7, 8, 9.1–9.4, 13; Tables 2, 3). According to IAWA Committee (Richter et al., Reference Richter, Grosser, Heinz and Gasson2004), the frequency range of the number of pits per cross-field is a characteristic diagnostic feature. On the other hand, Falcon-Lang (Reference Falcon-Lang2005) suggested the ontogenetic pattern by which, the number of pits per cross-field increases with decreasing age. Mature wood typically has fewer pits per field compared with juvenile wood in the upper trunk, branches, and roots. The specimens from the Chihuido Formation identified as A. cozzoi n. sp. have a range of three to eight pits per cross-field, which may correspond to a juvenile wood or to a branch, while A. lamaibandianus has one to four pits in the adult trunk. Large adult logs (65 × 120 cm in diameter) of A. lamaibandianus were identified, and a fragment of 8 cm in diameter that was calculated by the curvature of the growth rings, would correspond to a branch or juvenile stem assigned to the new species A. cozzoi. However, these fossil species are distinguished by the shape of the pits, which is an anatomical character used to differentiate fossil species (Figs. 7, 13; Tables 2, 3). In the literature, there are no data from extant or fossil specimens regarding changes in the shape of the pits in the trunk with respect to those of mature wood, branches, and roots. The extant species of Agathis have polygonal pits, while some species of Araucaria are characterized by circular shapes and others by polygonal radial pitting (Greguss, Reference Greguss1955; García Esteban et al., Reference García Esteban, De Palacios, Guindeo Casasús, Lázaro Durán, González Fernández, Rodríguez Salvador, Fernández García, Bobadilla Maldonado and Camacho Atalaya2002). According to Erasmus (Reference Erasmus1976) and Giraud (Reference Giraud1991), the tracheid radial pittings show modifications in the gymnosperms from Paleozoic to Present. These changes are referred to a reducing in the seriation of pits: 1–5 seriate in the Paleozoic (Glossopteridales, Pentoxylales, Cordaitales, and other plant groups); 1–2, 3 seriate in Mesozoic (Conifers extinct and fossil Araucariaceae) to 1–2 seriate in Cretaceous to Recent (extant Araucariaceae). Agathoxylon lamaibandianus and A. cozzoi n. sp. have similitudes with modern Araucariaceae by the presence of one to two seriate radial pitting (contiguous and alternate) and cross-fields of araucarioid type. Such affinities are supported by the known fossil Triassic record assigned to the Araucariaceae from the palynological data (Araucariacites australis Cookson, Reference Cookson1947 ex Couper, Reference Couper1953 and Inaperturopollenites reidi de Jersey, Reference de Jersey1964) of Australia and Argentina, and from the seed cone scales (Araucarites parsorensis Lele, Reference Lele1956) from India (Zamuner et al., Reference Zamuner, Zavattieri, Artabe, Morel, Artabe, Morel and Zamuner2001; Ottone et al., Reference Ottone, Mancuso and Resano2005; Kunzmann, Reference Kunzmann2007; Taylor et al., Reference Taylor, Taylor and Krings2009, and references therein). The Araucariaceae fossil woods recorded from Argentina were first described as Agathoxylon protoaraucana (Brea) Gnaedinger and Herbst in the “Darwin Forest,” Paramillos Formation, Precordillera of the Mendoza Province (Brea, Reference Brea1997; Brea et al., Reference Brea, Artabe and Spalletti2008, Reference Brea, Artabe and Spalletti2009). In the Northern Hemisphere, Agathoxylon (=Araucarioxylon) arizonicum Knowlton, Reference Knowlton1888 was described in the Upper Triassic “Petrified Forest,” Arizona (United States) (Ash and Creber, Reference Ash and Creber2000; Kunzmann, Reference Kunzmann2007).
Protojuniperoxylon ischigualastense has mixed wood types (Fig. 13; Tables 2, 3), but the other specific anatomical characters that are shared with the Cupressaceae s.l. are: axial parenchyma with nodular walls, horizontal walls of radial parenchyma cells distinctly pitted, end-walls of radial parenchyma cells nodular (=juniperoid-thickenings), radial tracheids, and cupressoid cross-fields (Bonetti, Reference Bonetti1966; Bodnar and Artabe, Reference Bodnar and Artabe2007; Table 3).
The fossil species described here has a greater affinity with extant Juniperus L. (Cupressoideae) and Fitzroya Hook. f. ex Lindl. (Callitroideae) due to the presence of horizontal walls of radial parenchyma cells distinctly pitted, end-walls of radial parenchyma cells nodular (juniperoid-thickenings), and nodular transverse end wall of axial parenchyma. In addition, it has affinity with extant Sequoia and Sequoiadendron (Sequoioideae), due to the presence of uniseriate and biseriate, with rarely triseriate rays, and mainly partially biseriate with >30 cells in height (Richter et al., Reference Richter, Grosser, Heinz and Gasson2004; Román-Jordán et al., Reference Román-Jordán, García Esteban, Palacios and Fernández2017). In accordance with Bodnar and Artabe (Reference Bodnar and Artabe2007), we interpret the fossil genus Protojuniperoxylon to correspond to a paraphyletic clade of the Cupressaceae s.l., despite its mixed wood type.
The abietinean wood type (Fig. 13; Tables 2, 3) identified as Cupressinoxylon has been related to Cupressaceae (Dolezych and Van der Burgh, Reference Dolezych and Van der Burgh2004; Dolezych and Schneider, Reference Dolezych and Schneider2006; Kłusek, Reference Kłusek2014; Bodnar et al., Reference Bodnar, Ruiz, Artabe, Morel and Ganuza2015; Brea et al., Reference Brea, Bellosi, Umazano and Krause2016; Egerton et al., Reference Egerton, Williams and Lacovara2016; Bodnar and Falco, Reference Bodnar and Falco2018; among others), and some species to Podocarpaceae (Kräusel, Reference Kräusel1949; Vaudois and Privé, Reference Vaudois and Privé1971; Pujana et al., Reference Pujana, Santillana and Marensi2014). Cupressinoxylon llantenesense n. sp. shows anatomical characteristics of the Cupressaceae s.s., such as cross-fields with cupressoid pits (Richter et al., Reference Richter, Grosser, Heinz and Gasson2004) arranged in one or two rows and the presence of axial parenchyma (Boureau, Reference Boureau1948; Bodnar and Artabe, Reference Bodnar and Artabe2007; Bodnar and Falco, Reference Bodnar and Falco2018). In addition, this new species has torus/margo pits, and thick membrane on radial tracheid walls like other members of the Cupressaceae s.s. (Hacke et al., Reference Hacke, Sperry and Pittermann2004; Brodribb et al., Reference Brodribb, Pittermann and Coomes2012; Bodnar et al., Reference Bodnar, Escapa, Cúneo and Gnaedinger2013). The Pinaceae have pits with porous margo (Bauch et al., Reference Bauch, Liese and Schultze1972; Bodnar et al., Reference Bodnar, Escapa, Cúneo and Gnaedinger2013).
The oldest generally accepted fossil assigned to the Cupressaceae is a permineralized cone Parasciadopitys from the Middle Triassic of Antarctica (Yao et al., Reference Yao, Taylor and Taylor1997; Taylor et al., Reference Taylor, Taylor and Krings2009). However, Bomfleur et al. (Reference Bomfleur, Decombeix, Escapa, Schwendemann and Axsmith2013), based on structural similarities between Parasciadopitys and the permineralized state of a Telemachus cone, included Parasciadopitys within the Voltziales. Bodnar and Falco (Reference Bodnar and Falco2018) pointed out that some species of Cupressinoxylon have affinity with the Cupressaceae s.l. Studies based on 16 fossil calibration points and three molecular dating methods show that Cupressaceae originated during the Triassic, when Pangaea was intact (Mao et al., Reference Mao, Milne, Zhang, Penga, Liu, Thomas, Mill and Renner2012). The vicariance between the two subfamilies (the Laurasian Cupressoideae and the Gondwanan Callitroideae) occurred when southern and northern hemisphere continents were separated (Mao et al., Reference Mao, Milne, Zhang, Penga, Liu, Thomas, Mill and Renner2012; Brea et al., Reference Brea, Bellosi, Umazano and Krause2016).
The fossil woods from the Tronquimalal Group show anatomical features similar to extant Araucariaceae and Cupressaceae s.l. (not to genus-level). Leslie et al. (Reference Leslie, Beaulieu, Holman, Campbell, Mei, Raubeson and Mathews2018) compared phylogenetic relationships and estimated divergence ages with the paleobotanical records. From their analysis, they estimated late Paleozoic (Permian) to early Mesozoic (Triassic) divergence ages for the extant family-level conifer clades.
The petrified forests of the Tronquimalal Group
The fossil records of impressions/compressions, wood petrifications, and pollen grains of the Tronquimalal Group suggest that in the Late Triassic, the conifers were represented by the Voltziaceae (impression/compression leaves of Heidiphyllum Retallack) and Cheirolepidiaceae (Classopollis Pflug pollen) transitional conifers. Other fossils with affinity to modern families were related to the Araucariaceae, Podocarpaceae, and Cupressaceae s.l. (Menéndez, Reference Menéndez1951; Volkheimer and Papú, Reference Volkheimer and Papú1993; Artabe et al., Reference Artabe, Morel, Spalletti and Brea1998; Zamuner et al., Reference Zamuner, Zavattieri, Artabe, Morel, Artabe, Morel and Zamuner2001).
In this study, the petrified woods described are related to the Araucariaceae (22%) and Cupressaceae s.l. (78%), and were part of the arboreal stratum of the Tronquimalal forests together with representatives of the transitional conifers (Heidiphyllum, Classopollis), ginkgoaleans (Sphenobaiera Florin emend. Harris and Millington; Baiera Braun; Baieroxylon Greguss), and gymnosperms of uncertain affinity (Linguifolium Arber) (Table 1) (Artabe et al., Reference Artabe, Morel, Spalletti and Brea1998; Zamuner et al., Reference Zamuner, Zavattieri, Artabe, Morel, Artabe, Morel and Zamuner2001; Crisafulli and Herbst, Reference Crisafulli and Herbst2010). Remarkably, the permineralized stem genus referred to Corystospermaceae (Rhexoxylon Bancroft emend. Archangelsky and Bret, Tranquiloxylon Herbst and Lutz, and Cuneumxylon Artabe and Brea), commonly recorded in the Triassic Argentinean basins, is not present within the Tronquimalal forests.
In the Chihuido Formation, trunks of Agathoxylon lamaibandianus (80%) and A. cozzoi n. sp. (20%) are present. Some species, such as Araucaria araucana (Molina, Reference Molina1782) K. Koch, Reference Koch1873, the extant representative of the Araucariaceae in Patagonia, can grow on soils of volcanic origin (Tortorelli, Reference Tortorelli2009). The association of the Araucariaceae with volcanic soils is consistent with the volcaniclastic sediments of the proximal fluvial systems of the Chihuido Formation (Spalletti, Reference Spalletti1997; Artabe et al., Reference Artabe, Morel, Spalletti and Brea1998; Artabe et al., Reference Artabe, Morel, Spalletti, Artabe, Morel and Zamuner2001) (Table 1).
The large trunks of the Chihuido Formation were quickly buried within the fluvial volcaniclastic hyper-concentrated deposits and debris-flow deposits related to conditions of high discharge and steep slopes associated with volcanic reliefs (Spalletti et al., Reference Spalletti, Artabe and Morel2003). Artabe et al. (Reference Artabe, Morel, Spalletti, Artabe, Morel and Zamuner2001) interpreted that these thick deposits, characteristic of proximal areas, allowed the preservation of petrifications in a medium where the sediments formed a rock-mass that prevented the degradation of woody material by biological attack. The woods were buried quickly and they were mineralized quickly by the profuse silica-rich solutions probably linked to volcanic activity.
In the Llantenes Formation, Artabe et al. (Reference Artabe, Morel, Spalletti, Artabe, Morel and Zamuner2001) described three “plant paleocommunities” that inhabited fluvial and deltaic environments, based on the composition of compression/impression fossil assemblages (taphocenosis) (Fig. 3; Table 1). Agathoxylon lamaibandianus was found in the middle levels of the stratigraphic profile, between two horizons corresponding to the 27 and 28 “paleocommunities” of Artabe et al. (Reference Artabe, Morel, Spalletti, Artabe, Morel and Zamuner2001) (Fig. 3; Table 1). These middle levels correspond to epilimnic facies association of marginal lacustrine environments underlying progradational deposits of deltaic mouth bars. The Cupressaceae s.l., Protojuniperoxylon ischigualastense (54%) and Cupressinoxylon llantenesense n. sp. (46%) were found in the upper levels of the Llantenes section above “paleocommunity” 28 (Fig. 3; Table 1). The coarser upper sections of the unit are interpreted as fluvial channels, distributary channels, and mouth-bar deposits of delta front (Spalletti, Reference Spalletti1997; Artabe et al., Reference Artabe, Morel, Spalletti, Artabe, Morel and Zamuner2001). On the stable fine-grained subaerial deposits formed in upland and uniform conditions within fluvial flood-plains and upper deltaic plains grew the conifers indicating well-established vegetation in higher areas of the system (Figs. 3, 5).
The trunks collected in the Tronquimalal Group investigation revealed that during the transport and burial processes they underwent compression in different directions, which produced deformation of the secondary xylem as a result of the compression of the sediment before the wood was permineralized (Fig. 14). The resulting distortions are observed clearly in cross-section (García Esteban et al., Reference García Esteban, Guindeo Casasús, Pereza Oramas and de Palacios2003; Schweingruber et al., Reference Schweingruber, Börner and Schulze2006; Schweingruber, Reference Schweingruber2007; Schweingruber and Börner, Reference Schweingruber and Börner2018). The deformation of the secondary xylem, “shearing zones” (Erasmus, Reference Erasmus1976), have been observed in all petrified specimens analyzed and are referred as a region with collapsed tracheids of the earlywood, following the typical oblique course for a short distance (Fig. 14.1). The collected specimens of the upper part of the Llantenes Formation also show deformation of the secondary xylem as “s-shaped linear rows” (Maheshwari, Reference Maheshwari1972). Under such physical state, the earlywood zones are strongly compressed and arranged in a zig-zag pattern together with the slightly distorted latewood (Schweingruber et al., Reference Schweingruber, Börner and Schulze2006; Schweingruber, Reference Schweingruber2007; Schweingruber and Börner, Reference Schweingruber and Börner2018) (Fig. 14.2, 14.4). In some cases, the compressed wood structures are recognizable from the rays with an evident zig-zag pattern (Fig. 14.3). Other wood fragments show compression in different directions without pattern (Fig. 14.5, 14.6). These deformations could be interpreted as a result of the dynamic processes related to mouth-bar deposits and distributary channels of fluvial-dominated deltaic lobes prograding into lacustrine environments.
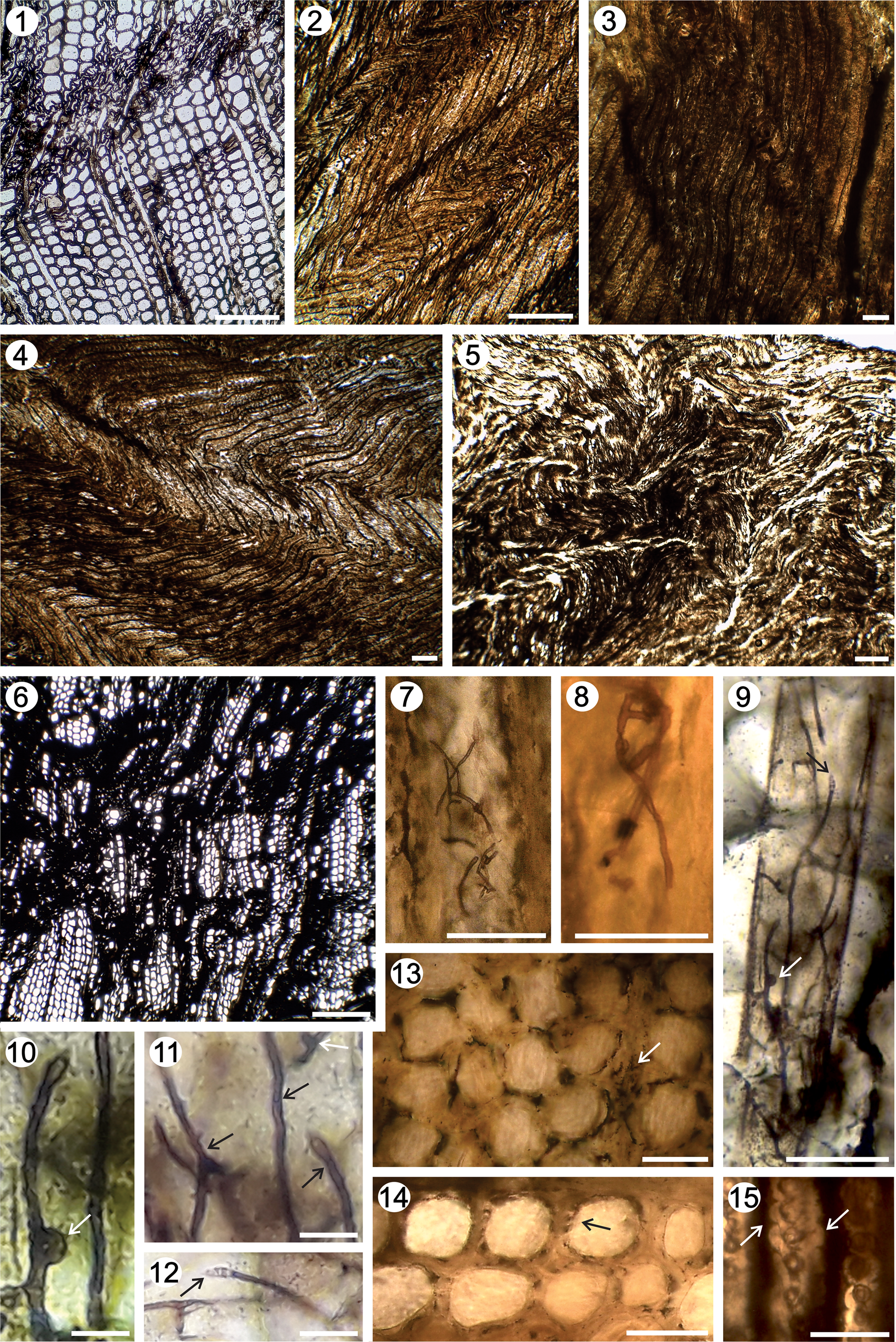
Figure 14. Secondary xylem deformation by compressed wood. (1) TS, “shearing zones;” (2, 4) TS, “s-shaped linear rows;” (3) TS, rays with an evident zig-zag pattern; (5, 6) TS, compressed wood in different directions; (7–9) RLS, hyphae simple or dichotomous; (9–11) RLS, hyphae with clamp connections (white arrows); (11) RLS, hyphae with septa (black arrows); (9, 12) RLS, hyphae with basidium septate (black arrows); (13) TS, decayed tissue displaying differentially decayed cells; arrow = lamella, primary wall, secondary wall; (14, 15) cells with simultaneously decay (arrow = radial notches, U-shaped in cell lumen in TS and in RLS). Scale bars: (1–6) 140 μm; (7–9, 13–15) 45 μm; (10–12) 23 μm. (1) IANIGLA PB 767; (2, 3) IANIGLA PB 777; (4) IANIGLA PB 749; (5) IANIGLA PB 770; (6) IANIGLA PB 754; (7, 8) IANIGLA PB 745; (9–15) IANIGLA PB 792a.
Climate and age
During the Triassic, the supercontinent Pangea was at its greatest size, in terms of aggregated continental crust. The extended exposed land area was divided symmetrically about the paleoequator, between the northern and southern hemispheres (Dubiel et al., Reference Dubiel, Parrish, Parrish and Good1991; Artabe et al., Reference Artabe, Morel and Spalletti2003; Spalletti et al., Reference Spalletti, Artabe and Morel2003). These conditions were ideal for maximizing monsoonal circulation, which was characterized by strongly seasonal climate (Parrish, Reference Parrish1993; Scotese et al., Reference Scotese, Boucot and McKerrow1999). During this time, the phytogeographic and floristic provincialism of Gondwana was strongly related to paleolatitudinal positions and therefore, to paleoclimatic zones. Spalletti et al. (Reference Spalletti, Artabe and Morel2003) and Artabe et al. (Reference Artabe, Morel and Spalletti2003) analyzed the paleogeographical reconstructions of southwestern Gondwana based on paleomagnetic and tectonic information, suggesting that in the Late Triassic, South America was displaced by 10° towards the equator, favoring the existence of dry subtropical and temperate-warm strongly seasonal climates in the interior basins of the mega-continent. The humid basins located in the western margin of southern South America supported a subtropical humid to sub-humid climate influenced by Pacific Ocean currents and developed with more humid conditions (Artabe et al., Reference Artabe, Morel, Spalletti, Artabe, Morel and Zamuner2001, Reference Artabe, Morel and Spalletti2003; Spalletti et al., Reference Spalletti, Artabe and Morel2003). The sedimentological evidence obtained from the study of the Llantenes Depocenter supports this paleoclimatic interpretation (Spalletti and Morel, Reference Spalletti and Morel1992; Spalletti, Reference Spalletti1997). The subtropical or warm-temperate humid climates favored the development of “extratropical” forests (Artabe et al., Reference Artabe, Morel and Spalletti2003; Spalletti et al., Reference Spalletti, Artabe and Morel2003).
Most of the petrified woods analyzed were compressed in vertical or horizontal orientations during fossilization. Hence, it was not possible to measure the width of the growth rings (Fig. 13.1–13.6). However, according to the classification given by Creber and Chaloner (Reference Creber and Chaloner1984) and illustrated by Brison et al. (Reference Brison, Philippe and Thevenard2001), the logs analyzed belong to Type D (Figs. 6.4, 8.2, 10.2, 12.2), reflecting relatively uniform seasonal growth, but each one has a terminal event representing a cessation or delay of the cambium activity. The growth rings suggest that the conifers identified in the Tronquimalal Group had growth interrupted by seasonal variations of temperature (winters and summers) and with a relatively uniform water supply.
This environment provided opportune conditions for fungal decay of wood. The fungal attack of wood requires sufficient temperature conditions and the availability of moisture in the wood (Schmidt, Reference Schmidt and Czeschlik2006). Such moisture exists as hygroscopic water within the wall or lumen cells, or it is obtained by extraction from the substrate, depending on the prevailing weather conditions (Engelund et al., Reference Engelund, Thygesen, Svensson and Hill2013; Thybring et al., Reference Thybring, Kymäläinen and Rautkari2018). The majority of the Tronquimalal Group woods analyzed show abundant hyphae and fungal decay. The hyphae are simple or dichotomous (Fig. 14.7–14.9), some with septa (Fig. 14.11), and others bearing clamp connections (Fig. 14.10) and with basidium septate (Fig. 14.12). The wood decay is white rot, manifested by the removal of tracheid walls, resulting of the degradation of lignin and cellulose (Stubblefield et al., Reference Stubblefield, Taylor and Beck1985; Stubblefield and Taylor, Reference Stubblefield and Taylor1986; Schwarze, Reference Schwarze2007; Gnaedinger et al., Reference Gnaedinger, Garcia Massini, Becchis and Zavattieri2015). In the transverse section, the cells show walls differentially decayed, appearing as whitened and deformed disconnected units (i.e., the middle lamella and primary wall) (Fig. 14.13). Other cells show simultaneous wall decay, observed as radial notches from the lumen outwards in transverse section and similar U-shaped features and erosion troughs in longitudinal section (Fig. 14.14, 14.15). The hyphae structures, presence of the clamp connections, and basidium in the fossil, associated with the white-rot, are comparable to decay caused by extant basidiomycetes. The presence of the basidium septate would relate them with the heterobasidiomycetes, a group of common wood-inhabiting fungi (Wright and Albertó, Reference Wright and Albertó2002; Zhang et al., Reference Zhang, Yang, Tu, Gao and Zhao2008).
In southwestern extratropical areas of Gondwanaland (30–60°S), three major floristic events were recognized by Spalletti et al. (Reference Spalletti, Artabe, Morel and Brea1999, Reference Spalletti, Artabe and Morel2003), Morel et al. (Reference Morel, Stipanicic, Ganuza, Stipanicic and Marsicano2001, Reference Morel, Artabe and Spalletti2003), and Artabe et al. (Reference Artabe, Morel, Spalletti, Artabe, Morel and Zamuner2001, Reference Artabe, Morel and Spalletti2003) for the Triassic sedimentary basins of Argentina. The third Florian Stage or DML Biozone of the Late Triassic (Norian to ?Rhaetian) (Spalletti, Reference Spalletti1997; Artabe et al., Reference Artabe, Morel, Spalletti and Brea1998; Spalletti et al., Reference Spalletti, Artabe, Morel and Brea1999; Morel et al., Reference Morel, Artabe and Spalletti2003) where the Tronquimalal Group is included, shows substantial changes in plant composition of communities of the long-lasting endemic Triassic Dicroidium-dominated floras. During the Florian event, Corystospermaceae, Peltaspermaceae, and ginkgoaleans declined while some taxa became dominant during the Jurassic across the Southern Hemisphere (Anderson and Anderson, Reference Anderson, Anderson, Lucas and Morales1993; Anderson et al., Reference Anderson, Anderson, Archangelsky, Bamford, Chandra, Dettmann, Hill, McLoughlin and Rosler1999; Spalletti et al., Reference Spalletti, Artabe, Morel and Brea1999; Zamuner et al., Reference Zamuner, Zavattieri, Artabe, Morel, Artabe, Morel and Zamuner2001; Morel et al., Reference Morel, Artabe and Spalletti2003), such as conifers and bennettitaleans (McLoughlin, Reference McLoughlin2001). Thus, the Florian Stage shows the development of deciduous forests where the corystosperms disappeared from the arboreal stratum (Artabe et al., Reference Artabe, Morel, Spalletti, Artabe, Morel and Zamuner2001). The fluvial areas supported seasonal deciduous forests with arboreal vegetation of voltzialeans, ginkgoaleans, and the Araucariaceae and Cupressaceae conifers (Agathoxylon lamaibandianus and Cupressinoxylon llantenesense n. sp.), whereas the corystosperms were only present in the understory (Artabe et al., Reference Artabe, Morel, Spalletti, Artabe, Morel and Zamuner2001). The margins of the lacustrine systems were characterized by deciduous forests with clear predominance of Linguifolium (Artabe et al., Reference Artabe, Morel, Spalletti, Artabe, Morel and Zamuner2001) and by Cupressaceae, Protojuniperoxylon ischigualastense (Table 1). Similarly, the modern representatives of these families, such as Juniperus L. and Fitzroya Hook.f. ex Lindl., develop under humid oceanic climates (Paull and Hill, Reference Paull and Hill2010).
Conclusions
The present study is an important contribution to the knowledge on the Upper Triassic lignoflora from the Tronquimalal Group, northeastern border of the Neuquén Basin, south of Mendoza Province, Argentina.
Four additional species are reported from the Tronquimal and the whole Neuquén Basin based on fossil woods. Fossil woods from the Chihuido Formation include Agathoxylon lamaibandianus and A. cozzoi n. sp. The Llantenes Formation preserves coniferous trunks assigned to Agathoxylon lamaibandianus (middle levels of the stratigraphic profile), Protojuniperoxylon ischigualastense, and Cupressinoxylon llantenesense n. sp. (upper levels).
From the analysis of new log specimens, we conclude that previously described wood from the Chihuido Formation assigned by Cozzo (in Menéndez, Reference Menéndez1951) to Araucarioxylon sp. is here identified as Agathoxylon cozzoi n. sp. Those recorded by Crisafulli and Herbst (Reference Crisafulli and Herbst2010) as Agathoxylon dallonii and Prototaxoxylon intertrappeum are considered in this study as being synonymous with A. lamaibandianus. This is the second occurrence of the species previously known from Upper Triassic strata of Argentina (Laguna Colorada Formation, El Tranquilo Group, Santa Cruz Province, Patagonia).
The genus Protojuniperoxylon ischigualastense is herein described for the second time for Argentina and therefore for Gondwana. It was first described from the Upper Triassic Ischigualasto Formation, San Juan Province, Argentina.
The new species Cupressinoxylon llantenesense is the oldest fossil wood species of the genus worldwide. Some species were described from the Jurassic and Cretaceous, and the Cupressinoxylon is especially species-rich in Cenozoic deposits.
In the Tronquimalal forests, the new record of the Araucariaceae and Cupressaceae s.l. are adding to the known composition fossil assemblage integrated by ginkgoaleans, Conifers (Voltziaceae, Cheirolepidiaceae), some Corystospermaceae, and gymnosperms incertae sedis (Linguifolium). The plant communities of the Llantenes Depocenter were developed under seasonal subtropical climate and experienced mostly temperate-warm humid to subhumid conditions, receiving certain marine influence from the west, as is suggested by paleogeographical reconstructions (Artabe et al., Reference Artabe, Morel and Spalletti2003; Spalletti et al., Reference Spalletti, Artabe and Morel2003).
The compositional differences of fossil woods and the numbers of individuals (richness) recorded in the Chihuido and Llantenes formations were caused by different paleoenvironments developed in the tectonic and sedimentary evolution of the Llantenes Depocenter. In the marginal areas related to high-energy conditions and associated with volcanic reliefs represented by the Chihuido Formation, just the Araucariaceae could be developed. In contrast, in more distal and lower-energy areas of the depocenter infilling the lacustrine systems, flood plains and deltaic inter-distributary plains allowed development of more diverse forests (Araucariaceae and Cupressaceae s.l.) when the middle and upper Llantenes Formation was deposited.
According to the wood type and particular anatomic characteristics, the coniferous woods from the Tronquimalal Group show similarity to some extant Araucariaceae and Cupressaceae s.l. This information is important for understanding the Triassic–Jurassic transition of the flora, because it supports the hypothesis that modern families of conifers originated in the Late Triassic and they were well established in the Early to Middle Jurassic flora in Gondwana (McLoughlin, Reference McLoughlin2001; Zamuner et al., Reference Zamuner, Zavattieri, Artabe, Morel, Artabe, Morel and Zamuner2001; Taylor et al., Reference Taylor, Taylor and Krings2009; Leslie et al., Reference Leslie, Beaulieu, Holman, Campbell, Mei, Raubeson and Mathews2018).
Acknowledgments
This contribution was partially funded by the Agencia Nacional de Promoción Científica y Tecnológica (ANPCyT–FONCyT) Argentina (PICT 2011–2546 to AMZ) and by the Secretaría General de Ciencia y Técnica, SGCYT-UNNE, Corrientes Province (PI 2015–2018, Q005–2014 to SCG) and CONICET (PIP 2014–2016. 112 201301 00317 to SCG). The authors acknowledge B. Cariglino and M.B. Lara regarding the field work, as well as collaboration in the collection of fossil plants and logs. Grateful acknowledgement is extended to E. Zavattieri for the improvement of the photo-micrographic figures. Thanks are given to A. Boura, anonymous reviewers, and particularly to the Associate Editor, N. Jud, for their suggestions and corrections that substantially improved the final version of this manuscript.