Introduction
Phylogenetic studies based on genetic tools have generated important insights into the relationships among ascaridoid nematodes (Nadler and Hudspeth, Reference Nadler and Hudspeth1998; Pereira and Luque, Reference Pereira and Luque2017; Li et al., Reference Li2018). Discussion remains on the validity of the family Raphidascarididae Hartwich, 1954 and its independence from the closely related Anisakidae Railliet & Henry, 1912 (see Pereira and Luque, Reference Pereira and Luque2017). Similarly, the subdivision of the genus Raphidascaris Bloch, 1779 into three subgenera, namely Raphidascaris Bloch, 1779, Ichthyascaris Wu, 1949 and Sprentascaris Petter & Cassone, 1984, has also been debated (Petter and Cassone, Reference Petter and Cassone1984; Bruce, Reference Bruce1990; Moravec et al., Reference Moravec, Kohn and Fernandes1990; Petter, Reference Petter1995; Moravec and Justine, Reference Moravec and Justine2012); however, there is no molecular approach focused on such a discussion.
Currently, Raphidascaris (Sprentascaris) includes six species parasitizing freshwater characiforms (Serrasalmidae), perciforms (Cichlidae) and siluriforms (Loricariidae and Pimelodidae) in the Neotropical region (Petter and Cassone, Reference Petter and Cassone1984; Ramallo, Reference Ramallo2009; Luque et al., Reference Luque2011; Melo et al., Reference Melo2011; Zago et al., Reference Zago2013; Ailán-Choke et al., Reference Ailán-Choke, Ramallo and Davies2017). None of these species have been characterized genetically.
During the first parasitological examination of Gymnogeophagus balzanii (Perugia, 1891) (Cichlidae), collected in the Pantanal wetlands, State of Mato Grosso do Sul, Brazil, several nematodes were found in the intestine of the fish. Detailed observations have revealed that these specimens represent a new species of Raphidascaris (Sprentascaris), described herein based on light and scanning electron microscopy (SEM), and characterized genetically based on four genetic markers. Additionally, phylogenetic reconstructions using representatives of Raphidascarididae were generated to evaluate the position of the new species within the family, its relationships with other taxa, especially with its congeners, and the validity of the subgenera of Raphidascaris.
Materials and methods
Collection and examination of nematodes
Twenty-one specimens of G. balzanii (total body length 50.2–109.5 mm) were collected using casting nets, from September 2015 to August 2017, in small lakes located at the margins of the State highway MS-184, which crosses the Pantanal wetlands, in the municipality of Corumbá, State of Mato Grosso do Sul, Brazil. Fish were kept alive in small water tanks with oxygen pumps, brought to the laboratory, euthanized and dissected. Host nomenclature and classification follow Froese and Pauly (Reference Froese and Pauly2018). Nematodes found alive were washed in saline, fixed in hot 4% formaldehyde solution and preserved in 70% ethanol. For morphological examinations, nematodes were cleared in glycerine. The middle body parts of one male were excised and fixed in molecular-grade 96–99% ethanol for genetic studies; the anterior and posterior parts were fixed in 4% formalin for morphological identification, i.e. hologenophores (the voucher specimens from which the molecular sample is directly derived; see Astrin et al., Reference Astrin, Zhoun and Misof2013, for more details).
Drawings were made using a drawing tube attached to an Olympus BX51 microscope. Measurements are given in micrometers, unless otherwise stated. Specimens used for SEM (two males, one of which the mid body was taken for genetic sequencing, and two females) were dehydrated through a graded ethanol series, dried by evaporation with hexamethyl disilazane, coated with gold and observed in a JEOL JSM 6460-LV at an accelerating voltage of 15 kV. The systematic classification of nematodes adopted follows the recent proposals of Pereira and Luque (Reference Pereira and Luque2017) and Li et al. (Reference Li2018), in which Anisakidae and Raphidascarididae are independent and valid. Parasite specimens were deposited in the Coleção Zoológica da Universidade Federal de Mato Grosso do Sul (ZUFMS).
Genetic procedures
Genomic DNA was isolated using a DNeasy Blood & Tissue Kit (QIAGEN, Hilden, Germany), following the manufacturer's instructions. Four genetic regions were amplified: the 5′ end of the 18S rDNA, the nuclear ITS1-5.8S-ITS2, the D2 and D3 domains of the 28S rDNA and the cox1 of the mtDNA. All polymerase chain reactions (PCR), cycling conditions and primers are detailed in supplementary material S1. PCR products were purified through an enzymatic treatment with ExoProStar™ (GE Healthcare) and sent for sequencing at ACTGene (Ludwig Biotec, Rio Grande do Sul, Brazil) with the same primers used in the PCR reactions.
Contiguous sequences were assembled in Geneious v. 9.1.5 (created by Biomatters, available from http://www.geneious.com/) and deposited in GenBank (see taxonomic summary for accession numbers). A preliminary BLAST search of the GenBank database (https://www.ncbi.nlm.nih.gov/genbank/) was performed to confirm the genetic proximity between the present sequences and those from representatives of Raphidascarididae.
Sequences chosen for phylogenetic reconstructions were from all representatives of Raphidascarididae, of which genetic regions were congruent with those from the present study (see table 1). Sequences from the same isolate or clones with 100% genetic similarity were not included. The following sequences were excluded from the analyses because they interfered in the alignment, showing high incongruence with the other sequences: 18S and 28S rDNA sequences of Hysterothylacium amoyense (Hsü, 1933) (MF072695/MF094276) and of Raphidascaris gigi Fujita, 1928 (AB558482 and AB558483/AB558480 and AB558481), and cox1 mtDNA sequence of Raphidascaris trichiuri (Yin & Zhang, 1983) (FJ907318). The phylogenetic analyses were based on four different datasets, to compare the present sequences with those from almost all taxa of Raphidascarididae, with maximum accuracy: one including the 18S and 28S rDNA sequences concatenated, one consisting of the nuclear ITS1-5.8S-ITS2, one concatenating the whole 18S, ITS1-5.8S-ITS2 and 28S rDNA, and one with the cox1 mtDNA alone. The outgroups were chosen according to the recent phylogeny by Li et al. (Reference Li2018), following the nature of each dataset (table 1). Sequences were aligned using M-Coffee (Notredame et al., Reference Notredame, Higgins and Heringa2000), then evaluated by the transitive consistency score, to verify the reliability of aligned positions, and based on score values ambiguous aligned positions were trimmed (Chang et al., Reference Chang, Di Tommaso and Notredame2014). Datasets were subjected to maximum likelihood (ML) and Bayesian inference analyses, using PHYML and MrBayes, respectively (Huelsenbeck and Ronquist, Reference Huelsenbeck and Ronquist2001; Guindon and Gascuel, Reference Guindon and Gascuel2003). The model of evolution and its fixed parameters for each dataset were chosen and estimated under the Akaike information criterion with jModelTest 2 (Guindon and Gascuel, Reference Guindon and Gascuel2003; Darriba et al., Reference Darriba2012) and are detailed in supplementary table S1. Nodal supports for Bayesian posterior probability values were determined after running the Markov chain Monte Carlo (2 runs 4 chains) for 4 × 106 generations, with sampling frequency every 4 × 103 generations and discarding the initial ¼ of sampled trees (1 × 106) as burn-in. The same, but for ML, were based on 1000 bootstrap non-parametric replications.
Table 1. Species whose sequences were obtained from GenBank and used in phylogenetic reconstructions, associated with their hosts (habitat), geographical origin, accession number and genetic regions. The 18S, ITS1-5.8S-ITS2, 28S refer to the rDNA and the cox1 to the mtDNA.

*Used as outgroups in the phylogenetic analyses.
NM = not mentioned.
Results
Ascaridoidea Baird, 1853; Raphidascarididae Hartwich, 1954; Raphidascaris (Sprentascaris) Petter & Cassone, 1984; Raphidascaris (Sprentascaris) andersoni n. sp.
Description
Small, thin, whitish nematodes. Cuticle thick with fine transverse striations throughout body (fig. 2F–H). Anterior end with three well-developed labia (one dorsal and two subventral), each bearing two long lateral, horn-shaped projections and three pairs of roughly triangular cuticular elevations (one dorsal and two subventral) at each labium base (figs 1C, D and 2D). Dorsal labium with two double papillae and pulp composed of two triangular anterior projections (figs 1C and 2A, B, D). Subventral bearing one double papilla and one amphidial papilla with transversal aperture, its pulp with cuticular projection of irregular edge (figs 1D and 2A–D). Well-developed lateral alae, c. 40 maximum width, originating at base of subventral labia (figs 1A–D and 2A, B, D), extending to near tail tip in females (figs 1G and 2H) and ending well anterior to first pair of caudal papillae in males (fig. 1J). Interlabia absent. Oesophagus muscular, slightly expanded at posterior end (fig. 1A, B). Nerve ring encircling oesophagus somewhat at end of its first 1/3 (fig. 1A, B). Excretory pore slightly posterior to level of nerve ring (fig. 1A). Ventriculus with irregular shape, transversely oval in some specimens; ventricular appendix short and posteriorly directed (fig. 1A, B).
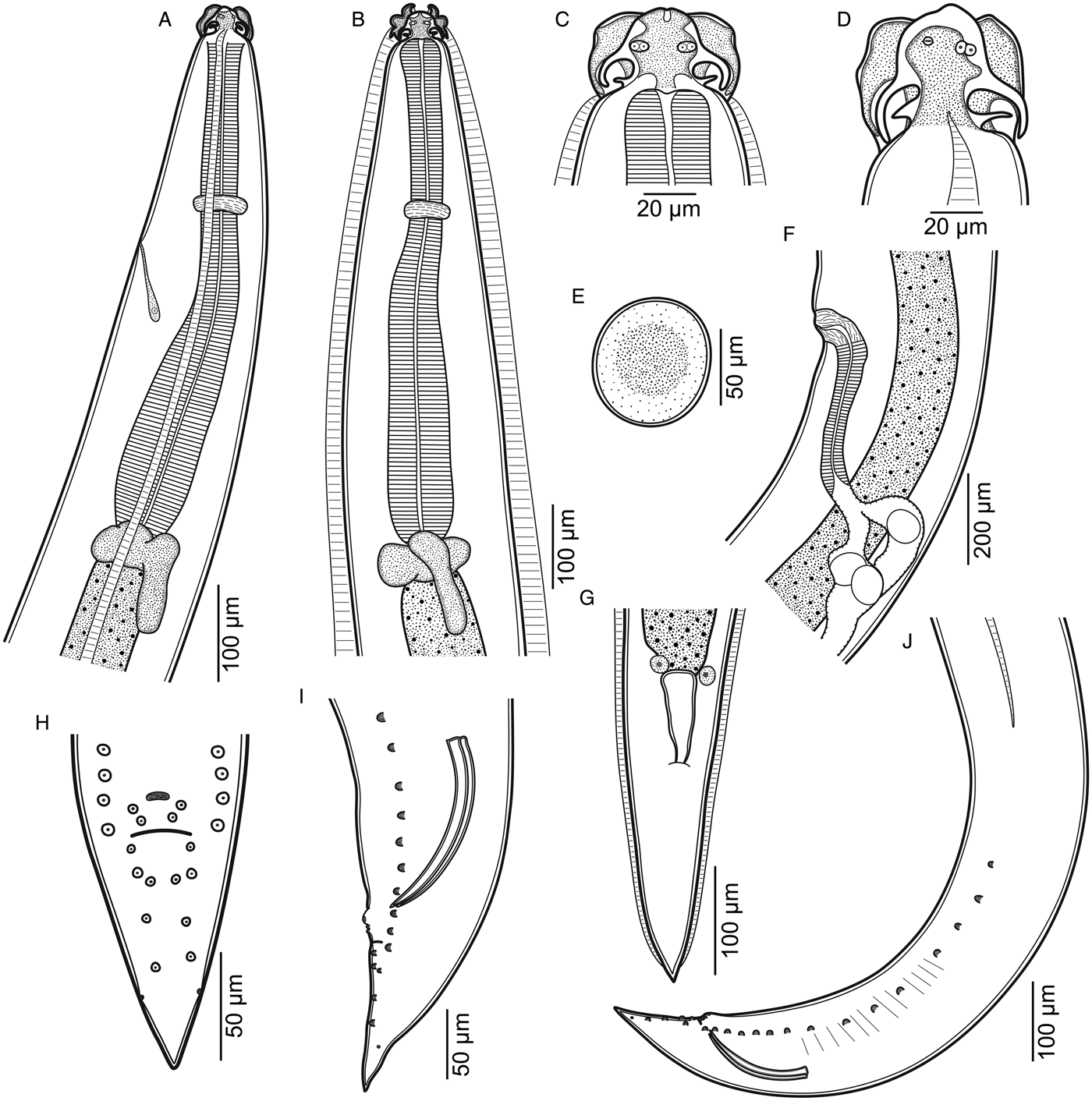
Fig. 1. Raphidascaris (Sprentascaris) andersoni n. sp. (A, B) anterior end, lateral and dorsoventral views, respectively; (C, D) cephalic end, male dorsal and female subventral views, respectively; (E) egg; (F) region of vulva, lateral view; (G) posterior end of female, ventral view; (H, I) tail of male, ventral and lateral views, respectively; (J) posterior end of male, lateral view.

Fig. 2. SEM micrographs of Raphidascaris (Sprentascaris) andersoni n. sp. showing (A, B, D) cephalic end, apical views (arrow indicates lateral ala, arrowheads indicate postlabial cuticular elevations); (C) subventral labium, subapical view; (E) posterior end of male, subventral view; (F, G) tail and cloacal regions of male, ventral views, respectively (arrowhead indicates phasmidial pore); (H) tail of female, subapical view (arrow indicates lateral ala). Abbreviations: a, amphidial papilla; d, dorsal labium; e, excretory pore; p, double labial papilla; s, subventral labium; u, unpaired papilla-like formation.
Male (based on holotype and eight paratypes; measurements of holotype in parentheses)
Body length 3.8–6.3 (5.1) mm, maximum width 171–232 (216). Labia 22–28 (28) long. Oesophagus 456–624 (624) long, with maximum width 63–91 (74). Entire length of oesophagus representing 9.6–12.6 (12.2) % of body length. Nerve ring and excretory pore 173–207 (204) and 203–242 (215), respectively, from anterior body end. Ventriculus 32–51 (35) long and 32–67 (45) wide; ventricular appendix 94–146 (94) long and 25–51 (34) wide. Spicules equal, well-sclerotized, with straight proximal end and sharp distal tip, 116–137 (117) long, representing 2.2–3.4 (2.3) % of total body length (fig. 1I, J). Gubernaculum and caudal alae absent. Precloacal papillae: 15 pairs similar in size, 13 of which are subventral and two ventral near cloacal aperture, somewhat asymmetric; one ventral unpaired papillae-like ellipsoid formation anterior to ventral pairs of precloacal papillae (figs 1H–J and 2E–G). Postcloacal papillae: five ventral pairs, asymmetrically arranged in two lines (figs 1H–J and 2E, F). Pair of lateral, small and inconspicuous phasmidial pores, posterior to last pair of postcloacal papillae (figs 1H–J and 2F). Tail conical, 103–132 (132) long (figs 1H–J and 2E).
Female (based on allotype and eight paratypes, all gravid; measurements of allotype in parentheses)
Body length 4.4–8.7 (6.6) mm, maximum width 193–348 (264). Labia 21–39 (24) long. Oesophagus 549–797 (777) long, with maximum width 73–108 (86). Entire length of oesophagus representing 9.1–12.5 (11.7) % of body length. Nerve ring and excretory pore 172–285 (231) and 283–370 (296), respectively, from anterior body end. Ventriculus 34–58 (53) long and 45–70 (68) wide; ventricular appendix 94–160 (154) long and 21–44 (44) wide. Vulva 1.2–2.6 (2.2) mm from anterior end, at 28–35 (33) % of body length, vulval lips slightly elevated (fig. 1F). Vagina posteriorly directed, with striated musculature, followed by strong muscular ovijector c. 230 long and didelphic opisthodelphic uterus (fig. 1F). Eggs oval with thin smooth shell, non embryonated, measuring 53–74 × 50–70 (from allotype) (fig. 1E). Tail conical with pointed end, 226–317 (317) long (figs 1G and 2H). Phasmids not observed.
Taxonomic summary
Type host. Gymnogeophagus balzanii (Perugia) (Actinopterygii: Cichlidae).
Site of infection. Intestine.
Type locality. Lakes on the margin of State highway MS-184, Pantanal wetlands, municipality of Corumbá, State of Mato Grosso do Sul, Brazil (19°28′S, 57°02′W).
Prevalence. Six infected fish of 21 examined (28.5%).
Mean intensity of infection (range). 5.16 ± 5.45 (1–17) nematodes per infected host.
Specimens deposited. Holotype male (ZUFMS-NEM00062), allotype female (ZUFMS-NEM00063), female paratypes (ZUFMS-00064), male paratypes (ZUFMS-00065).
Genetic data (GenBank accession numbers). 18S rDNA (MK141033), 28S (MK141031) rDNA, ITS1-5.8S-ITS2 (MK141032), cox1 mtDNA (MK14343).
Etymology. The specific name is a homage to Roy Clayton Anderson, who made important and valuable contributions to the knowledge of the nematode parasites of vertebrates.
Remarks
According to the conception of Moravec et al. (Reference Moravec, Kohn and Fernandes1990), the subgenus Raphidascaris (Sprentascaris) includes species with postlabial cuticular elevations and mature eggs in the uterus containing fully formed larvae. The present specimens have clear postlabial cuticular elevations and, even though no eggs were found containing larvae, they were allocated in Raphidascaris (Sprentascaris), as the subgenus is exclusive to freshwater fishes in the Neotropical region. Non-embryonated eggs were also reported in R. (S.) lanfrediae Melo, Santos, Giese, Santos & Santos, 2011 and in R. (S.) pimelodi (Petter & Cassone, 1984) (Petter and Cassone, Reference Petter and Cassone1984; Pereira and Luque, Reference Pereira and Luque2017) suggesting that this character may be unsuitable for subgeneric diagnosis, as it was amended by Moravec (Reference Moravec1998).
Raphidascaris (S.) andersoni n. sp. is the only species in the subgenus that has a conspicuous unpaired papilla-like formation anterior to the cloacal aperture. A structure that may resemble it was described in R. (S.) lanfrediae, a parasite of cichlid fishes from northern Brazil (Pereira and Luque, Reference Pereira and Luque2017); however, this feature appears as a small button-like formation without striations in R. (S.) lanfrediae, whereas in the new species it is large and striated. Moreover, the new species differs from R. (S.) lanfrediae because the caudal alae are absent in males (vs present), the lateral alae end far anterior from the first pair of precloacal papillae (vs at level of ninth pair of precloacal papillae pairs), by having two pairs of subventral papillae slightly anterior to cloacal opening (vs only one pair) and vulva far posterior (at 28–35% vs14–22% of body length) (Melo et al., Reference Melo2011; Pereira and Luque, Reference Pereira and Luque2017), among other morphometric differences.
Of the remaining five species of Raphidascaris (Sprentascaris), R. (S.) hypostomi (Petter & Cassone, 1984) and R. (S.) pimelodi have no lateral alae, clearly differing from the new species (Petter and Cassone, Reference Petter and Cassone1984; Moravec et al., Reference Moravec, Kohn and Fernandes1990). Furthermore, R. (S.) hypostomi has digitiform conspicuous phasmids (vs pore-like inconspicuous in the new species) (Moravec et al., Reference Moravec, Kohn and Fernandes1990) and males of R. (S.) pimelodi have ventral cuticular surface on the posterior end of body ornamented (vs smooth in the new species) (Petter and Cassone, Reference Petter and Cassone1984). Raphidascaris (S.) mahnerti has well-developed lateral alae as in R. (S.) andersoni n. sp., but males of the first have long and conspicuous caudal alae, which are absent in the new species (Petter and Cassone, Reference Petter and Cassone1984; Moravec et al., Reference Moravec, Kohn and Fernandes1990). In contrast, R. (S.) marano Ramallo, 2009 and R. (S.) saltaensis Ailán-Choke, Ramallo & Davies, 2017, both parasites of loricariid fishes in Argentina, also have conspicuous lateral alae but males lack caudal alae as in the new species; however, these two species differ from R. (S.) andersoni n. sp. by having males with only three pairs of postcloacal papillae (vs five pairs), one pair of adcloacal papillae (vs absence of adcloacal papillae), larger spicules (220–300 μm at maximum vs 116–137 μm) and embryonated eggs with rugged shell (vs non-embryonated with smooth shell) (Ramallo, Reference Ramallo2009; Ailán-Choke et al., Reference Ailán-Choke, Ramallo and Davies2017).
Molecular characterization and phylogenetic analyses
The partial sequences of the 18S (779 bp) and 28S (728 bp) rDNA, nuclear region ITS1-5.8S-ITS2 (927 bp) and cox1 (384 bp) mtDNA, were obtained for the new species. As expected, R. (S.) lanfrediae was most genetically similar to R. (S.) andersoni n. sp. regarding the nuclear genes 18S and 28S rRNA (sequence identity 96.7%). Without R. (S.) lanfrediae in the alignment, R. (S.) andersoni n. sp. was most genetically similar to the two taxa labelled as Raphidascaris sp. in the dataset of the ITS1-5.8S-ITS2 (78.8% and 77.17%, respectively). When concatenating the whole nuclear region 18S, ITS1-5.8S-ITS2 and 28S rDNA, the new species was most genetically similar to Raphidascaris (Raphidascaris) acus (Bloch, 1779), followed by Hysterothylacium tetrapteri (Bruce & Cannon, 1989) and Raphidascaris (Ichthyascaris) lophii (Wu, 1949) (86.54%, 85.75% and 85.45%, respectively). In the alignment using sequences of the cox1 mtDNA, R. (S.) andersoni n. sp. was most similar genetically to H. tetrapteri (83.85%), R. (I.) lophii and R. (I.) longispicula Li, Liu, Liu & Zhang, 2012 (each 83.59%).
The phylogenetic reconstruction using the 18S and 28S rDNA sequences concatenated confirmed the separation of R. (S.) andersoni n. sp. from its closely related R. (S.) lanfrediae; both species formed a full-supported, monophyletic and external clade within Raphidascarididae (fig. 3A). In this phylogeny, species of the subgenus Ichthyascaris formed a monophyletic highly supported assemblage as sister group of H. longilabrum Li, Liu & Zhang, Reference Li, Liu and Zhang2012. A similar pattern was noted in the phylogeny generated from sequences of the ITS1-5.8S-ITS2; after inclusion of R. (I.) trichiuri the clade formed by species of Raphidascaris (Ichthyascaris) continued with full support; however, two taxa identified only as Raphidascaris sp. were also in the same cluster (fig. 3B). In the same tree, the subgenus Raphidascaris, represented by two species, appeared as non-monophyletic (fig. 3B). The trees generated from the alignments of the 18S, ITS1-5.8S-ITS2, 28S rDNA concatenated and from the cox1 also showed the monophyly of Raphidascaris (Ichthyascaris) as sister group of H. longilabrum (fig. 4A), even though nodal support values were different according to each dataset (fig. 4A, B).
In all the phylogenetic reconstructions, R. (S.) andersoni n. sp. was separated from the other taxa, but its phylogenetic position within Raphidascarididae changed according to each dataset and the genus Hysterothylacium Ward & Magath, 1917 was not monophyletic (figs 3A, B and 4A, B). Similarly, Raphidascaroides Yamaguti, 1941 was not monophyletic in the tree generated from 18S and 28S rDNA sequences concatenated, appearing in two highly supported, separate clades (fig. 3A).

Fig. 3. Phylogenetic trees of Raphidascarididae generated from Bayesian inference (BI) analysis of sequences of the (A) 18S + 28S rDNA concatenated and (B) ITS1-5.8S-ITS2. Nodal supports of Bayesian posterior probabilities (BPP) were generated after running the Markov chain Monte Carlo (two runs four chains, 4 × 106 generations, sampling frequency = 4 × 103, burn-in = 1 × 106); those of maximum likelihood (ML) were generated after 1000 non-parametric bootstrap replications. Taxon in bold represents the new species; subgenera of Raphidascaris are highlighted. Squares represent BPP = 1 and ML = 100%; circles represent 0.96 < BPP < 1 and 90% ≤ ML ≤ 100%.

Fig. 4. Phylogenetic trees of Raphidascarididae generated from Bayesian inference (BI) analysis of sequences of the (A) 18S + ITS1-5.8S-ITS2 + 28S rDNA concatenated and (B) cox1 mtDNA. Nodal supports of Bayesian posterior probabilities (BPP) were generated after running the Markov chain Monte Carlo (two runs four chains, 4 × 106 generations, sampling frequency = 4 × 103, burn-in = 1 × 106); those of maximum likelihood (ML) were generated after 1000 non-parametric bootstrap replications. Taxon in bold represents the new species; subgenera of Raphidascaris are highlighted. Squares represent BPP = 1 and ML = 100%; filled circles represent 0.96 < BPP < 1 and 90% ≤ ML ≤ 100%; unfilled circle represents BPP = 0.94 and ML = 88%.
Discussion
Recent studies have shown the monophyly of Raphidascarididae and its clear separation from Anisakidae (Pereira and Luque, Reference Pereira and Luque2017; Li et al., Reference Li2018). Such a discussion was not the focus here, but we agree with these previous studies and this was the reason why anisakids were used as outgroups in the present phylogenetic analyses.
The nuclear rDNA genes, which are more conserved than those from the mtDNA, seem to show more resolution pertaining to the phylogenetic relationships among genera (and subgenera) of Raphidascarididae according to the present phylogenies. In this sense, the absence of monophyly of Hysterothylacium and Raphidascaroides was confirmed, as shown in other studies (Nadler and Hudspeth, Reference Nadler and Hudspeth2000; Pereira and Luque, Reference Pereira and Luque2017; Li et al., Reference Li2018). Furthermore, it should be noted that the more taxa included in the analysis, the better the phylogenetic resolution.
When more than one taxon belonging to Ichthyascaris and Sprentascaris was included in the phylogenetic reconstructions, both subgenera formed well-supported monophyletic assemblages. It was interesting that R. (S.) andersoni n. sp. and R. (S.) lanfrediae clustered far from Raphidascaroides brasiliensis Moravec & Thatcher, 1997 and Ro. moraveci Pereira, Tavares, Scholz & Luque, 2015, even though all these species parasitize freshwater fishes from Brazil (fig. 3A) (Pereira et al., Reference Pereira2015), indicating a clear separation between these genera. Conversely, the two Raphidascaris spp. isolated from a perciform fish off Brazil (Pantoja et al., Reference Pantoja2015), were closer to species of Raphidascaris (Ichthyascaris) parasitic in marine fishes from different orders in Europe and Asia (Li et al., Reference Li, Liu and Zhang2012a; Xu et al., Reference Xu2012; Pérez-i-García et al., Reference Pérez-i-García2015) (see fig. 3B and table 1). Both sequences of Raphidascaris sp. (KJ634267/KJ634266) were isolated from larval stages (L3) (Pantoja et al., Reference Pantoja2015), and they belong to Ichthyascaris. Therefore, at least the subgenera Sprentascaris and Ichthyascaris seem to have a consistent monophyly, different from Raphidascaris.
The lack of monophyly in the subgenus Raphidascaris observed in the phylogenetic reconstruction using the ITS1-5.8S-ITS2 dataset cannot be explained with certainty. However, it should be mentioned that Raphidascaris (R.) acus was isolated from a catadromous anguiliform fish in the eastern Mediterranean (off Turkey) (Simsek et al., Reference Simsek2016) (KT633862), whereas the two isolates of R. (R.) macrouri Pérez-i-García, Constenla, Carrassón, Montero, Soler-Membrives & González-Solís, 2015 are from marine gadiform fishes in the western Mediterranean (off Spain) (Pérez-i-García et al., Reference Pérez-i-García2015) (KR232377, KR232376). In contrast, there is consistent close relatedness of R. (I.) longispicula and R (I.) lophii with H. longilabrum (figs 3A and 4A), which may be explained by their same geographical origin (China) (Li et al., Reference Li, Liu and Zhang2012a,Reference Li, Liu and Zhangb; Xu et al., Reference Xu2012).
The validity of the subgenera of Raphidascaris has been discussed previously and some diagnostic features have been demonstrated to be inconsistent (e.g. egg development in ovijector, presence/absence of lateral alae). For example, Bruce (Reference Bruce1990) and Bruce et al. (Reference Bruce, Adlard and Cannon1994) re-established Ichthyascaris as an independent genus based on the structure of labia and the presence of lateral alae, but later Moravec & Nagasawa (Reference Moravec and Nagasawa2002) verified that several species were wrongly allocated in Ichthyascaris by the previous authors. Furthermore, one of the diagnostic features of Raphidascaris (Sprentascaris) is the presence of eggs containing larvae in gravid females (Moravec et al., Reference Moravec, Kohn and Fernandes1990); however, in the new species, in R. (S.) lanfrediae and in R. (S.) pimelodi, the eggs are not embryonated (Petter and Cassone, Reference Petter and Cassone1984; Pereira and Luque, Reference Pereira and Luque2017; present study). Therefore, Moravec (Reference Moravec1998) amended the subgeneric diagnosis of Sprentascaris as “eggs in uterus embryonated or non-embryonated.”
Based on our results, mainly those of the phylogenetic reconstructions, Raphidascaris, Ichthyascaris and Sprentascaris should be considered as independent genera for a while, even though the subgenus Raphidascaris is apparently not monophyletic. In this sense, an updated generic diagnosis is give herein. It should be highlighted that this is the first parasitological survey in Gymnogeophagus balzanii.
Diagnosis of the genera Raphidascaris, Ichthyascaris and Sprentascaris, updated from Moravec et al. (Reference Moravec, Kohn and Fernandes1990) and Moravec and Nagasawa (Reference Moravec and Nagasawa2002):
Raphidascaris Bloch, 1779: Postlabial cuticular projections in interlabial space absent; only a feebly developed elevation may be present in ventral labial region. Lateral alae absent. Parasites of freshwater and marine fishes throughout the world.
Ichthyascaris Wu, 1949: Labia without lateral membranous flanges. Lateral alae present and united ventro-anteriorly at short distance from subventral labium base. Parasites of marine fishes throughout the world.
Sprentascaris Petter & Cassone, 1984: Labia with lateral membranous flanges. Three pairs of conspicuous postlabial cuticular projections, directed to interlabial space. Lateral alae present or absent. Parasites of freshwater fishes in the Neotropical region.
Supplementary material
To view supplementary material for this article, please visit https://doi.org/10.1017/S0022149X18001153
Acknowledgements
The authors would like to thank Dr Elias Nogueira de Aguiar from the Laboratório Multiusuário de Análises de Materiais do Instituto de Física (MULTILAM-INFI), Universidade Federal de Mato Grosso do Sul (UFMS), for help with scanning electron microscopy procedures.
Financial support
This work was financed in part by the Coordenação de Aperfeiçoamento de Pessoal de Nível Superior-Brasil (CAPES)-Finance code 001. LSM was supported by a Master fellowship from Fundação de Apoio ao Desenvolvimento do Ensino, Ciência e Tecnologia do Estado de Mato Grosso do Sul (acronym FUNDECT-05/2016). FBP was supported by a post-doctoral fellowship PNPD-CAPES (Programa Nacional de Pós-Doutorado-Coordenação de Aperfeiçoamento de Pessoal de Nível Superior-CAPES, Brazil).
Conflict of interest
None.
Ethical standards
All procedures involving animal manipulation were permitted by Sistema de Informação e Autorização em Biodiversidade (license number 54895) and were in strict accordance with the recommendations of the Colégio Brasileiro de Experimentação Animal.
Author ORCIDs
F.B. Pereira 0000-0002-7921-5965