Introduction
Although the Mediterranean Sea is one of the most investigated regions of the World Ocean in relation to the presence of Monogenea (Platyhelminthes) (Euzet et al., Reference Euzet, Combes and Caro1993; Strona et al., Reference Strona, Stefani and Galli2010), and Gyrodactylus von Nordmann, 1832 is one of the most species-rich genera within monogeneans – 409 species sensu Harris et al. (Reference Harris, Shinn, Cable and Bakke2004), the first finding of gyrodactylids in the Mediterranean Sea occurred only at the beginning of the 21st century, with six species found on sand gobies (Gobiidae) in the western Mediterranean and Adriatic seas, and all had previously been observed in the north-eastern Atlantic Ocean (Huyse et al., Reference Huyse, Pampoulie, Audenaert and Volckaert2006). Later, Paladini et al. (Reference Paladini, Cable, Fioravanti, Faria, Di Cave and Shinn2009, Reference Paladini, Hansen, Fioravanti and Shinn2011) described two new Gyrodactylus spp. from farmed juveniles of Sparus aurata L. in the Tyrrhenian and Adriatic seas, and Paladini et al. (Reference Paladini, Cable, Fioravanti, Faria and Shinn2010) another one from aquarium-held Syngnathus typhle L. caught off Marseille (France). Recently, this latter species has also been found on Gobius cobitis Pallas off Sardinia (Dmitrieva et al., Reference Dmitrieva, Gerasev, Garippa, Piras and Merella2015).
Thus, nine species of Gyrodactylus are formally known for the Mediterranean Sea: G. arcuatus Bychowsky, 1933; G. branchialis Huyse, Malmberg & Volckaert, 2004; G. corleonis Paladini, Cable, Fioravanti, Faria & Shinn, 2010; G. gondae Huyse, Malmberg & Volckaert, 2004; G. longipes Paladini, Hansen, Fioravanti & Shinn, 2011; G. orecchiae Paladini, Cable, Fioravanti, Faria, Di Cave & Shinn, 2009; G. ostendicus Huyse & Malmberg, 2004; G. rugiensis Gläser, 1974; and G. rugiensoides Huyse & Volckaert, 2002 (Huyse et al., Reference Huyse, Pampoulie, Audenaert and Volckaert2006; Paladini et al., Reference Paladini, Cable, Fioravanti, Faria, Di Cave and Shinn2009, Reference Paladini, Cable, Fioravanti, Faria and Shinn2010, Reference Paladini, Hansen, Fioravanti and Shinn2011; Dmitrieva et al., Reference Dmitrieva, Gerasev, Garippa, Piras and Merella2015).
Fourteen species of Gyrodactylus are known for the Black Sea: G. alviga Dmitrieva & Gerasev, 2000; G. anguillae Ergens, 1960; G. arcuatus; G. atherinae Bychowsky, 1934; G. bubyri Osmanov, 1965; G. crenilabri Zaika, 1966; G. flesi Malmberg, 1957; G. ginestrae Kvach, Ondračková, Seifertová & Hulak, 2019; G. harengi Malmberg, 1957; G. mugili Zhukov, 1970; G. mulli Gerasev & Dmitrieva, 2005; G. proterorhini Ergens, 1967; G. rarus Wagener, 1910; G. sphinx Dmitrieva & Gerasev, 2000 (Ergens, Reference Ergens and Bauer1985; Dmitrieva & Gerasev, Reference Dmitrieva and Gerasev1997; Maltsev & Miroshnichenko, Reference Maltsev and Miroshnichenko1998; Gerasev & Dmitrieva, Reference Gerasev and Dmitrieva2004, Reference Gerasev and Dmitrieva2005; Stoyanov et al., Reference Stoyanov, Huyse, Pankov and Georgiev2016; Kvach et al., Reference Kvach, Ondračková, Seifertová and Hulak2019).
In particular, G. sphinx, found on the gills, skin and fins of the sphinx blenny Aidablennius sphynx (Valenciennes) in the Black Sea off Crimea, was firstly reported as Gyrodactylus sp. 2 (Dmitrieva & Gerasev, Reference Dmitrieva and Gerasev1997). Later, its validity was confirmed, and the new species was named as G. sphinx Dmitrieva & Gerasev, 2000. This species has previously been reported only on the sphinx blenny in the Black Sea, and unsuccessful experimental attempts to infect other fish species of the same biotope have led to the conclusion that this G. sphinx was strictly specific to A. sphynx (Dmitrieva, Reference Dmitrieva2003).
In the present study, representatives of Gyrodactylus, morphologically similar to G. sphinx, were found on the gills, skin and fins of the peacock blenny Salaria pavo (Risso) in the Black Sea near Crimea, and on the same host and on aquarium-held Salaria basilisca (Valenciennes) in the Mediterranean Sea off Sardinia, and additionally on A. sphynx in the Black Sea off the Caucasian coast. In this context, the aims of this paper are to clarify whether the specimens found in hosts and localities other than the type ones of G. sphinx belong to the same species. To reach this goal, their morphological and genetic variability were studied in order to reconstruct the phylogenetic relationships with other species of this genus and the phylogeographic patterns among the allelic variants found in the samples collected in the present study for the nuclear internal transcribed spacer 2 (ITS2) and 5.8S ribosomal DNA (rDNA) regions. Indeed, the ITS rDNA region has been suggested as a valuable tool to discriminate among species of Gyrodactylus, which is almost invariable in conspecific samples, and homozygous and homogeneous in the most specimens studied, with the 5.8S gene as highly conservative and informative for subgenera division, and the ITS1 and ITS2 useful to resolve phylogeny at species level (Cable et al., Reference Cable, Harris, Tinsley and Lazarus1999; Ziętara et al., Reference Ziętara, Arndt, Geets, Hellemans and Volckaert2000, Reference Ziętara, Huyse, Lumme and Volckaert2002). Moreover, the rDNA ITS marker has been shown as rather stable in geographically distant populations of some widespread Gyrodactylus species (Ziętara et al., Reference Ziętara, Arndt, Geets, Hellemans and Volckaert2000).
Materials and methods
Sampling and parasitological examination
In the summers of 2015 and 2018, a total of 78 specimens of blenniid fish from two sites in the Mediterranean Sea and four sites in the Black Sea (fig. 1, table 1), were examined under a dissecting microscope for the presence of monogeneans. All monogeneans were collected alive, some of them were immediately mounted in glycerine jelly (prepared with 0.5 g carbolic acid) after Gusev (Reference Gusev1983), and others were fixed in 70–96% ethanol and stored at 5°C for molecular analysis.

Fig. 1. Localities of sampling: 1, Cala Gonone, off central eastern Sardinia; 2, Naracu Nieddu, off north-eastern Sardinia; 3, Sevastopol, off central coast of Crimea; 4, Batiliman, off central coast of Crimea; 5, Karadag, off south-eastern coast of Crimea; 6, Gelendzhik, off western coast of Caucasus. See table 1 for details on localities and host species sampled.
Table 1. Sampling localities, hosts examined and levels of infection of Gyrodactylus spp.

Type materials of G. sphinx (collection nunbers: 702.M.8 s.h, 703.M.8 s.p1-8–707.M.8 s.p20-22) from the Marine Parasites Collection of the A.O. Kovalevsky Institute of Biology of the Southern Seas, Sevastopol, Russia (IBSS collection, http://marineparasites.org) were included in the morphometric analysis.
Morphometric analysis
Measurements and light micrographs were made with Olympus BX51 and CX41 microscopes (Olympus Corporation, Tokyo, Japan), at magnifications of ×800–2000, using phase-contrast optics and cellSens Imaging Software (Olympus Corporation, Tokyo, Japan).
In total, 169 monogeneans were used for the morphometric analysis. The measuring scheme, including 22 characters of haptoral structures (fig. 2), mainly followed Malmberg (Reference Malmberg1970). Abbreviations of the linear measurements are as follows: bA, pharynx anterior bulb; bP, pharynx posterior bulb; DBL, dorsal bar length; DBW, dorsal bar width; HA, hamulus aperture distance; HPL, hamulus point length; HRL, hamulus root length; HSL, hamulus sickle inner length; HShL, hamulus shaft length; HW, hamulus proximal shaft width; MA, marginal hook aperture; MDW, marginal hook sickle distal width; MPW, marginal hook sickle proximal width; MSL, marginal hook sickle length; MShL, marginal hook shaft length; ML, marginal hook total length; MTW, marginal hook sickle toe width; MCO, male copulatory organ; VBAW, ventral bar anterior width; VBBL, ventral bar basal length; VBL, ventral bar total length; VBML, ventral bar membrane length; VBPW, ventral bar lateral process width; VBW, ventral bar basal width. The terms ventral and dorsal bar length and width are used in relation to the longitudinal axis of the worm's body. The point angle of hamulus sickle was produced using the cosine calculated through the sides of the triangle formed by the HPL, HA and HSL (fig. 2). Body size is given for mounted and flattened but unbroken worms; width was measured at the level of the uterus.
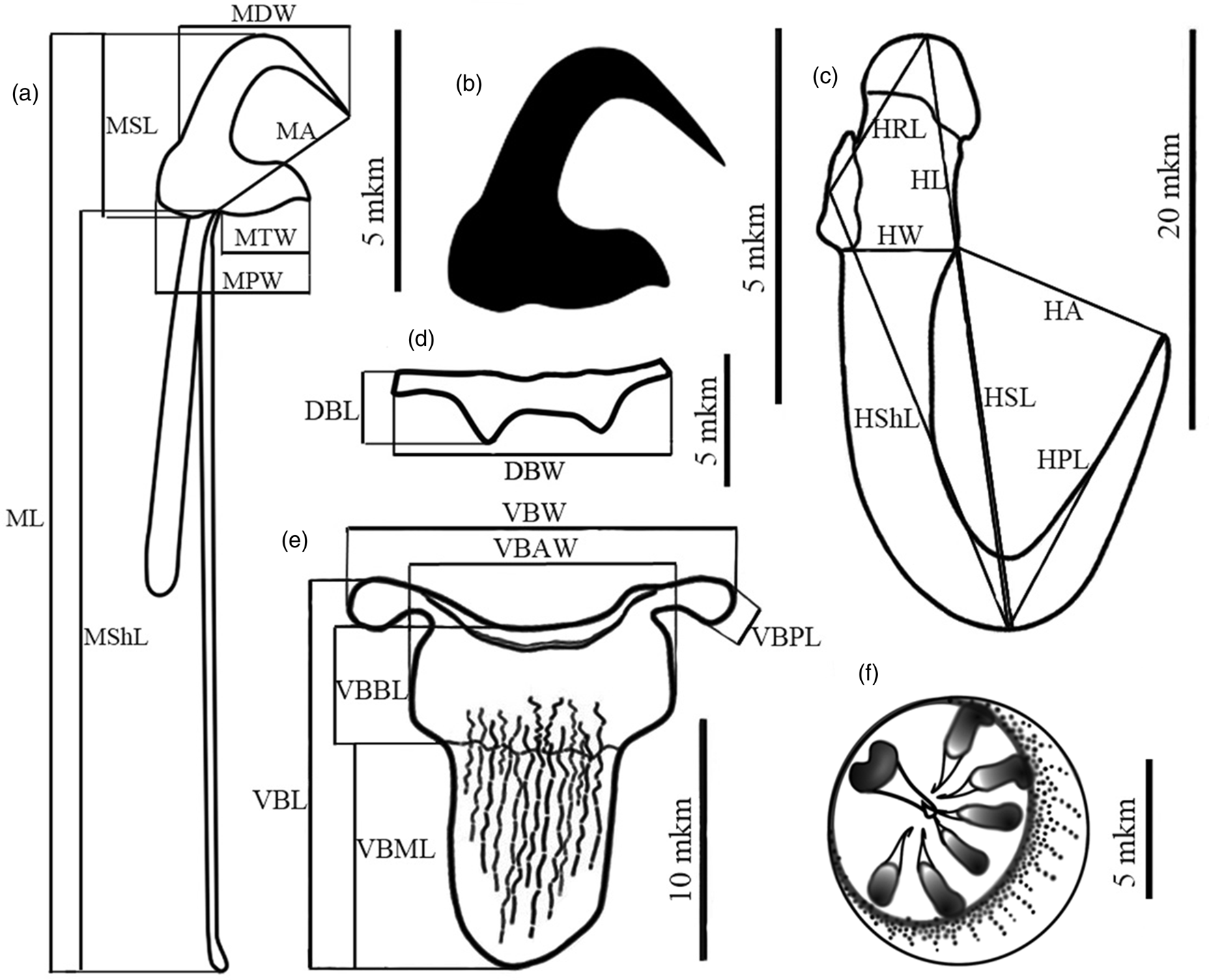
Fig. 2. Haptoral and male copulatory organ structures of Gyrodactylus gerasevi n. sp. with scheme of the measurements: (a) marginal hook; (b) marginal hook sickle; (c) hamulus; (d) dorsal bar; (e) ventral bar; (f) MCO. For abbreviations, see Materials and Methods.
All measurements are given in micrometres, as the range followed by the mean, standard deviation and number of measurements (in parentheses). Principal component analysis (PCA) was carried out based on the correlation matrix of the seven log-transformed measurements describing the main parameters of both hamuli and marginal hooks from 169 and 111 gyrodactylids, respectively. Descriptive statistics, univariate and multivariate analysis of variance (ANOVA, MANOVA), discriminant analysis and PCA were produced using the software package Statistica 6 for Windows (TIBCO Software Inc.,Tulsa, USA).
Molecular analysis
Molecular analyses were performed on a total of 81 samples coming from two sites in the western Mediterranean Sea (19 Aquarium of Cala Gonone; 18 Naracu Nieddu), and from four sites in the Black Sea (13 Gelendzhik; 6 Batiliman; 16 Sevastopol; 9 Karadag) (see table 1 for details).
DNA extraction
Genomic DNA of specimens was extracted using the kits Macherey-Nagel NucleoSpin Tissue (Macherey-Nagel GmbH & Co. KG, Dueren, Germany) and Dnk-Ehkstran Kit (Syntol, Moscow, Russia), following the supplier's instructions and the protocol provided by Cossu et al. (Reference Cossu, Dedola, Scarpa, Sanna, Lai, Maltagliati, Curini-Galletti and Casu2015). After extraction, DNA solution was stored at 4°C. Sample quality and DNA concentration were determined via spectrophotometry using a ND-8000 (NanoDrop Technologies, Thermo Fisher Scientific, Waltham, USA). The DNA mean concentration obtained for the samples was 20 ng/μL.
Polymerase chain reaction (PCR) amplification and sequencing
PCRs of a ITS2 rDNA 429-bp-long fragment for samples from the Mediterranean and Black seas were performed using the following primers, specifically designed for the present study by the authors: Gyro ITS2 F (5′-TGCAGCAAACTGTGTTA-3′) and Gyro ITS2 R (5′-CGTTACAAAGCGAACTAAG-3′). Furthermore, using the primers ITS1A (5′-GTAACAAGGTTTCCGTAGGTG-3′) and ITS2 (5′-TCCTCCGCTTAGTGATA-3′) designed by Matějusová et al. (Reference Matějusová, Gelnar, McBeath, Collins and Cunningham2001), PCRs were also performed for a 5.8S rDNA 157-bp-long fragment on a sub-set of 15 specimens from the Black Sea, and for the whole ITS1–5.8S–ITS2 region (977, 978 bp long) on a sub-set of two specimens from the Mediterranean and Black seas (see table 2 for details and GenBank accession numbers). The latter were sequenced with two additional primers: ITS1R (50-ATTTG CGTTC GAGAG ACC G-30) and ITS2F (TGGTG GATCA CTCGG CTC A-30) (Ziętara et al., Reference Ziętara, Lebedeva, Muñoz and Lumme2012). Reactions were carried out in a total volume of 25 μl containing 10 ng of total genomic DNA on average, 2.0 U of Taq DNA Polymerase (Sigma Aldrich, Merck KGaA, Darmstadt, Germany), 1× reaction buffer, 3 mm of magnesium chloride, 0.24 μM of each primer and 200 μM of each deoxynucleotide triphosphates (dNTP). PCRs were performed in an Applied Biosystems GeneAmp PCR System 9700 Thermal Cycler (Applied Biosystems, Waltham, USA), programmed as follows: 1 cycle of 4 min at 94°C, 35 cycles of 30 sec at 94°C, 30 sec at 50°C and 30 sec at 72°C. At the end, a post-treatment of 5 min at 72°C and a final cooling at 4°C were carried out. Both positive and negative controls were used to test the effectiveness of the PCR protocols, and the absence of possible contaminations. Electrophoresis was carried out on 2% agarose gels, prepared using 1× sodium boric acid buffer (pH 8.2) and stained with Gel Red Nucleic Acid Stain (Biotium, Fremont, USA). PCR products were purified by ExoSAP-IT (Thermo Fisher Scientific, Waltham, USA) and sequenced for both forward and reverse strands (by means of the same primers used for PCR), using an external sequencing core service (Macrogen, Amsterdam, Netherlands).
Table 2. List of the new Gyrodactylus samples included in the molecular analysis with GenBank accession numbers.

Phylogenetic and phylogeographic analysis
Seventy-nine and 15 newly generated ITS2 and 5.8S rDNA sequences, respectively, were aligned using the program CLUSTAL W (Thompson et al., Reference Thompson, Higgins and Gibson1994), as implemented in the BioEdit 7.0.5.2 software package (Hall, Reference Hall1999) and deposited in the GenBank (table 2).
The genetic variation was assessed by estimating the number of polymorphic sites (S), number of allelic variants (H), nucleotide diversity (π) and haplotype/allelic diversity (h), using the software package DnaSP6.12.03 (Librado & Rozas, Reference Librado and Rozas2009).
A median-joining network (Bandelt et al., Reference Bandelt, Forster and Rohl1999) was constructed using the software package Network 10.0.0.0 (www.fluxus-engineering.com) to infer the genetic relationships among sequences obtained in the present study and to detect the occurrence (if any) of traces of evolutionary forces acting on the Black Sea and Mediterranean populations. The transitions and transversions were equally weighted. Due to the lack of knowledge regarding the possible occurrence of retromutation events, the same weight (10) was assigned to all the observed polymorphisms.
To depict the relationships among species and compare the inter vs. intraspecies distances, phylogenetic analyses were performed on a dataset including the sequences of Gyrodactylus obtained in this study along with representatives of the ITS2 rDNA sequences available in GenBank for Gyrodactylus spp. from the Mediterranean Sea and Black Sea: G. arcuatus; G. branchialis; G. bubyri; G. corleonis; G. gondae; G. ginestrae; G. flesi; G. harengi; G. longipes; G. orecchiae; G. ostendicus; G. proterorhini; G. rarus; G. rugiensis; and G. rugiensoides; plus for further species closely related to G. sphinx: G. chileani Ziętara, Lebedeva, Muñoz & Lumme, 2012; Gyrodactylus sp. sensu Huyse et al. (Reference Huyse, Audenaert and Volckaert2003); G. scartichthi Lebedeva, Muñoz & Lumme, 2021; G. viridae Lebedeva, Muñoz & Lumme, 2021; and G. zietarae Lebedeva, Muñoz & Lumme, 2021. Moreover, three closely related species were included in the analyses: G. flavescensis Huyse, Malmberg & Volckaert, 2004; G. branchicus Malmberg, 1964; and G. robustus fig. 7. The software MrBayes 3.1.2 (Ronquist & Huelsenbeck, Reference Ronquist and Huelsenbeck2003) was used to perform a phylogenetic Bayesian rooted tree analysis on this dataset, setting G. bubyri, G. corleonis, G. ginestrae, G. rugiensis and G. rugiensoides as an outgroup cluster.
Two independent runs, each consisting of four Metropolis-coupled Markov chain Monte Carlo chains (one cold and three heated chains) were run simultaneously for 5,000,000 generations, sampling trees every 1000 generations. Parameters were set according to the results of Jmodeltest (Posada, Reference Posada2008). The first 25% of sampled trees were discarded as burn-in. Runs were carried out by means of the CIPRES Phylogenetic Portal (Miller et al., Reference Miller, Pfeiffer and Schwartz2010). Convergence of chains was verified checking the Average Standard Deviation of Split Frequencies (which should approach 0) (Ronquist et al., Reference Ronquist, Teslenko and van der Mark2012), and the Potential Scale Reduction Factor (which should be around 1) (Gelman & Rubin, Reference Gelman and Rubin1992) following Scarpa et al. (Reference Scarpa, Sanna, Cossu, Lai, Casu and Curini-Galletti2019). Phylogenetic trees were visualized and edited using FigTree 1.4.0 (available at http://tree.bio.ed.ac.uk/software/figtree/).
The uncorrected pairwise genetic distances (p-distance) between the taxa were estimated using the software MEGA 7 (Kumar et al., Reference Kumar, Stecher and Tamura2016).
Furthermore, the use of the Automatic Barcode Gap Discovery (ABGD) (Puillandre et al., Reference Puillandre, Lambert, Brouillet and Achaz2012) allowed to infer the occurrence of molecular taxonomic entities among the sequences obtained in the present study. The ABGD method was applied. Taxonomic entities were identified by the ABGD online tool (available at https://bioinfo.mnhn.fr/abi/public/abgd/) using the K2P pairwise genetic distance (Kimura, Reference Kimura1980) with a prior P ranging from 0.001 to 0.12. According to Puillandre et al. (Reference Puillandre, Lambert, Brouillet and Achaz2012), the correct species estimate was selected at the gene specific priors for maximum divergence of intraspecific diversity, corresponding to P = 0.001.
Results
Morphometric analysis
The comparison of the main characters for the species identification of the representatives of Gyrodactylus, between the specimens collected from the three host species in the different seas and regions, showed no evident differences in the shape of the hamuli, dorsal and ventral bars, and marginal hooks (fig. 3). Moreover, the ranges of the 22 characters of the haptoral structures and the diameter of the copulatory organ of the newly collected gyrodactylids from the Mediterranean and Black seas significantly overlapped with those of the type specimens of G. sphinx, as well as between specimens from different hosts and localities (table 3).
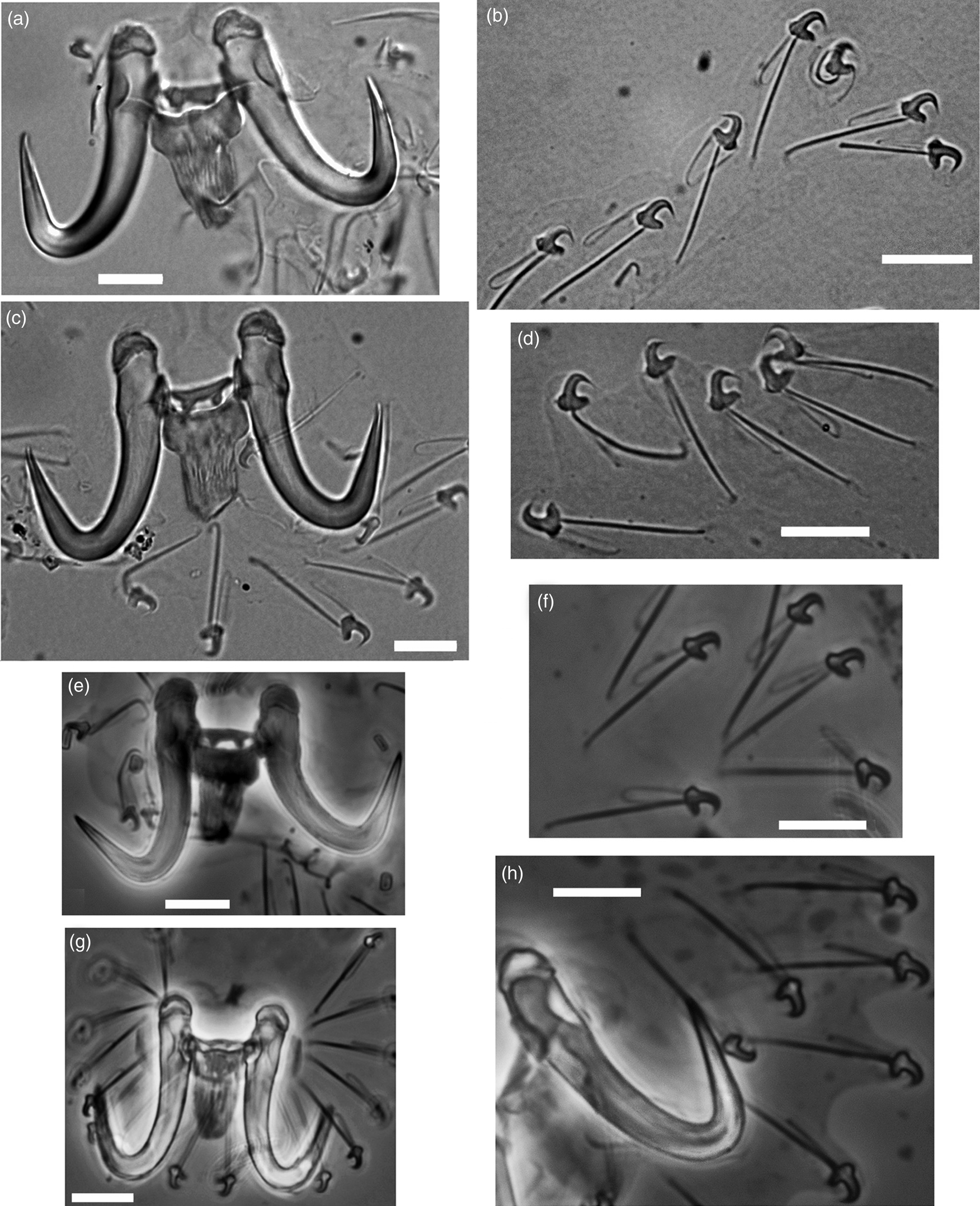
Fig. 3. Haptoral structures of Gyrodactylus spp.: Gyrodactylus gerasevi n. sp. ex Salaria pavo (a, b) and ex Salaria basilisca (c, d) from the Mediterranean Sea, off Sardinia; Gyrodactylus gerasevi n. sp. ex Aidablennius sphynx from the Black Sea, off Caucasus (e, f); Gyrodactylus sphinx ex Aidablennius sphynx from the Black Sea, off Sevastopol (g, h). Scale bars: 10 μm.
Table 3. Metrical data of Gyrodactylus spp. from the different Bleniidae fish and localities of the Mediterranean and Black seasa and univariate comparison (P-value of t-test) of the total samples of Gyrodactylus sphinx with Gyrodactylus gerasevi n. sp.
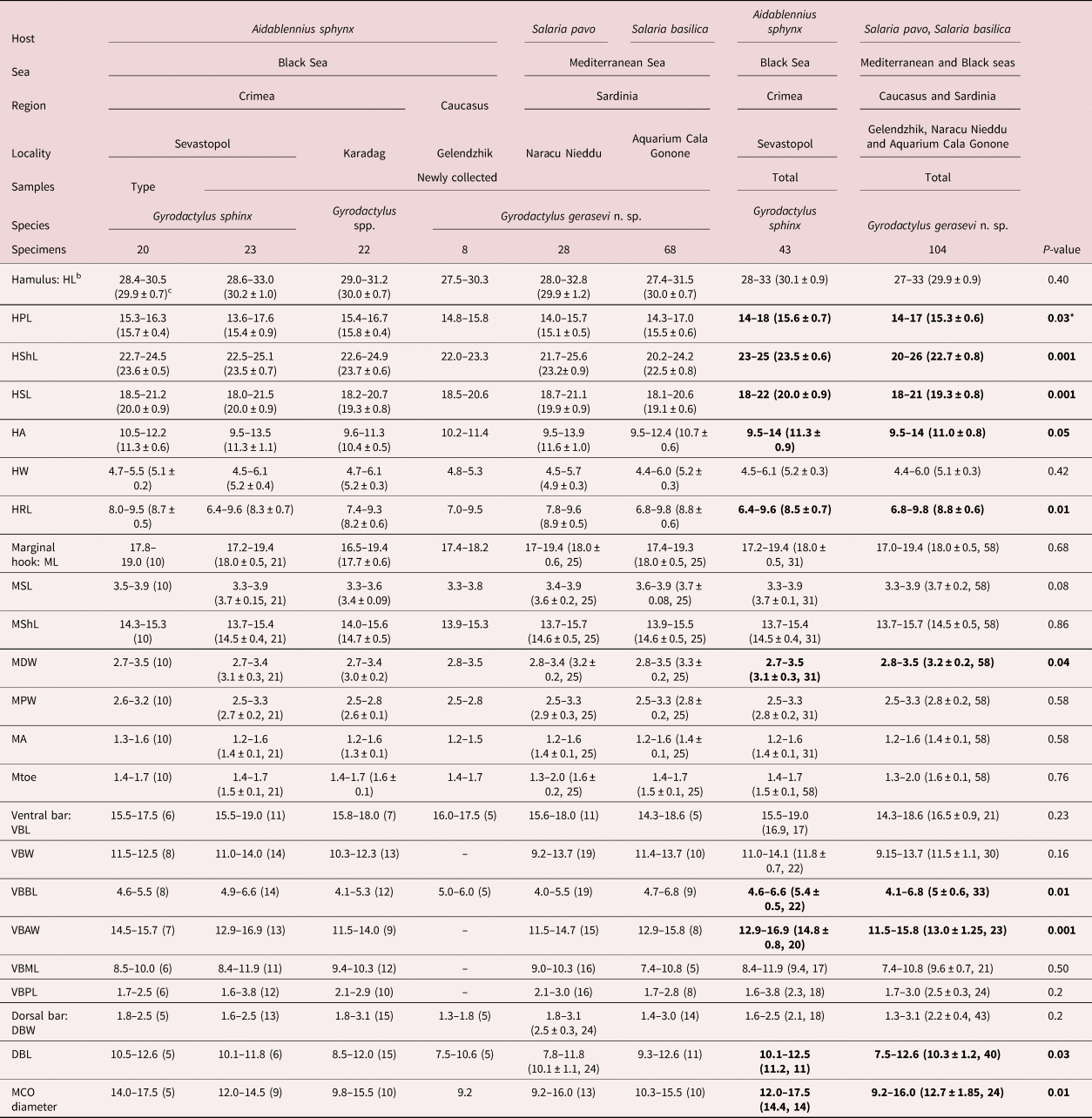
a Raw data are presented in additional file.
b For abbreviations, see Materials and Methods and fig. 2.
c Mean and standard deviation are presented if the number of measurements ≥20, and number of measurements is presented after comma if it differs from the total number of examined specimens.
* Significant value of differences, with P-value < 0.05, in bold.
The MANOVA revealed a significant influence of the host species (A. sphinx vs. S. pavo vs. S. basilisca), seas (Mediterranean vs. Black seas), regions (Sardinia vs. Crimea) and localities (Naracu Nieddu vs. Aquarium of Cala Gonone; Aquarium of Cala Gonone vs. Sevastopol vs. Karadag) on the variability of the morphometry of hamuli and marginal hooks of Gyrodactylus (table 4). Six of the seven variables of hamulus (HPL, HShL, HSL, HA, HW, HRL) and three of marginal hook (MSL, MPW, MDW) accounted for the variation among host species, and with greater significance (P < 0.001) the following: HShL (F = 31), HSL (F = 16), HA (F = 13), HRL (F = 10), HPL (F = 9.7) and MSL (F = 8). Four (HPL, HShL, HSL, HRL) and three (MSL, MPW, MDW) characters of hamulus and marginal hook, respectively, explained the differences between both seas and regions, and these that significantly contributed (P < 0.001) were: HShL (F = 41), HRL (F = 20) and MSL (F = 12) for seas and HShL (F = 51), HRL (F = 19), HSL (F = 12), HPL (F = 11) and MPW (F = 12) for regions. Six characters of hamulus (HPL, HShL, HSL, HA, HW, HRL) and four of marginal hook (MSL, MPW, MDW, MA) distinguished gyrodactylids corresponding to localities, and the most significant (P < 0.001) were the following: HShL (F = 25), HSL (F = 17), HA (F = 15), HPL (F = 9), HRL (F = 7) and MSL (F = 33), MPW (F = 8). In summary, six of seven measurements of the hamulus (mainly HShL) were significantly dependent on the studied factors, while only three dimensions of the marginal hook sickle were determined by host, sea, region or locality. However, in general, the variability of the latter was very low, comparable with the objective measurement error of 0.5 μm (table 3).
Table 4. Multivariate analysis of the variance of seven measurements of both the anchor and the marginal hook of Gyrodactylus spp. collected from the different localities and host species.a

a See table 1 for details of host species, locality and number of specimens.
b The sample from Caucasus (Gelendzhik) is not included in this analysis, because a small number of specimens.
According to the discriminant analysis based on the seven measurements of hamulus, the examined gyrodactylids were correctly assigned to the host species in 78%, to the sea in 80%, to the region in 83% and to the locality in 61% of cases. The same analysis of the seven measurements of the marginal hook resulted in the correct classification of the parasites in 61%, 72%, 70% and 61% of cases, respectively. Thus, despite the fact that MANOVA results revealed significant differences in the measurements of hamuli and marginal hooks between the gyrodactylids collected from different hosts, seas, regions and localities, 20 to 40% of the specimens were not correctly assigned to the corresponding sample according to the measured characters of these haptoral structures.
A lack of clear differentiation between samples was confirmed by the PCA. Thus, seven dimensions of each hamulus and marginal hook were reduced to three first principal components (i.e. factors) describing 67.6% of the overall variance of the hamulus (fig. 4) and 65.4% of the marginal hook (fig. 5). Confidence intervals of all groups of specimens, separated by host species (figs 4a, b and 5a, b), regions (figs 4c, d and 5c, d) and hosts and localities (figs 4e, f and 5e, f), overlapped significantly in the first two plans of PCA. Therefore, the variability of the morphometry of the haptoral structures did not allow to discriminate different groups of specimens among the examined gyrodactylids.

Fig. 4. Plots of 169 Gyrodactylus specimens based on their scores in the first (a, c, e) and second (b, d, f) planes of the PCA run on metrical data for seven characters of the hamulus (for scheme of measurements, see fig. 2). (a, b) Specimens grouped with the corresponding host species; (c, d) specimens grouped with the corresponding regions; (e, f) specimens grouped with the corresponding hosts and localities. Ellipsis show 95% confidence intervals. Note that in the samples from Sardinia and Caucasus only ITS2 haplotypes of Gyrodactylus gerasevi n. sp. ex Salaria pavo and S. basilisca are present, while in the samples from Crimea, ITS2 haplotypes of both species of Gyrodactylus ex Aidablennius sphynx are found.

Fig. 5. Plots of 111 Gyrodactylus specimens based on their scores in the first (a, c, e) and second (b, d, f) planes of the PCA run on metrical data for seven characters of the marginal hooks (for scheme of measurements, see fig. 2). (a, b) Specimens grouped with the corresponding host species; (c, d) specimens grouped with the corresponding regions; (e, f) specimens grouped with the corresponding hosts and localities. Ellipsis show 95% confidence intervals. Note that in the samples from Sardinia and Caucasus only ITS2 haplotypes of Gyrodactylus gerasevi n. sp. ex Salaria pavo and S. basilisca are present, while in the samples from Crimea, ITS2 haplotypes of both species of Gyrodactylus ex Aidablennius sphynx are found.
Molecular analysis
On the whole ITS2 rDNA dataset of 79 sequences of gyrodactylids from the Mediterranean Sea and Black Sea analysed in this study (for the GenBank accession numbers see table 2), 11 polymorphic sites were found, resulting in a total of seven allelic variants (table 5). The most common variant was shared by 51 individuals from both Mediterranean and Black seas, while the second most common variant was exclusive to 22 individuals from two sites of the Black Sea (Sevastopol and Karadag). Four allelic variants were exclusive to single individuals from three sites in the Black Sea (Batiliman, Gelendzhik, Karadag) and one to the Mediterranean Sea (Naracu Nieddu), while one variant was found in two individuals from the Mediterranean site of Naracu Nieddu. Overall, the estimates of genetic divergence (table 5) showed low values at each sampling locality. In particular, a total lack of genetic variation was found in the Mediterranean Sea among the individuals from the Aquarium of Cala Gonone and in the Black Sea for the individuals from Sevastopol.
Table 5. Indices of genetic variation and frequencies of haplotype distribution at the ITS2 rRNA region.

a N, sample sizes; S, number of polymorphic sites; H, number of allelic variants; h, haplotype/allelic diversity; π, nucleotide diversity. bSee fig. 6.
The median-joining network analysis, carried out for the ITS2 rDNA region on Mediterranean and Black Sea samples (fig. 6), identified two main clusters of sequences (A and B), which diverged for six point mutations. The cluster A encompassed individuals from the Black Sea along with the whole sample of specimens from the Mediterranean Sea. In particular, within this cluster, 89% of the individuals shared the same central allelic variant, with no geographic structuring among Mediterranean and Black Sea; the remaining five surrounding allelic variants diverged for one/two point mutations in accordance with a star-like network configuration. Estimates of genetic divergence were also performed on the whole group of sequences included within the cluster A, thus evidencing five polymorphic sites, six allelic variants and low levels of genetic variation among samples. The cluster B was represented by a single allelic variant, which was exclusive to individuals from two sites of the Black Sea (Sevastopol and Karadag). Estimates of genetic divergence performed for this cluster of sequences showed the total lack of genetic variation among samples (table 5).
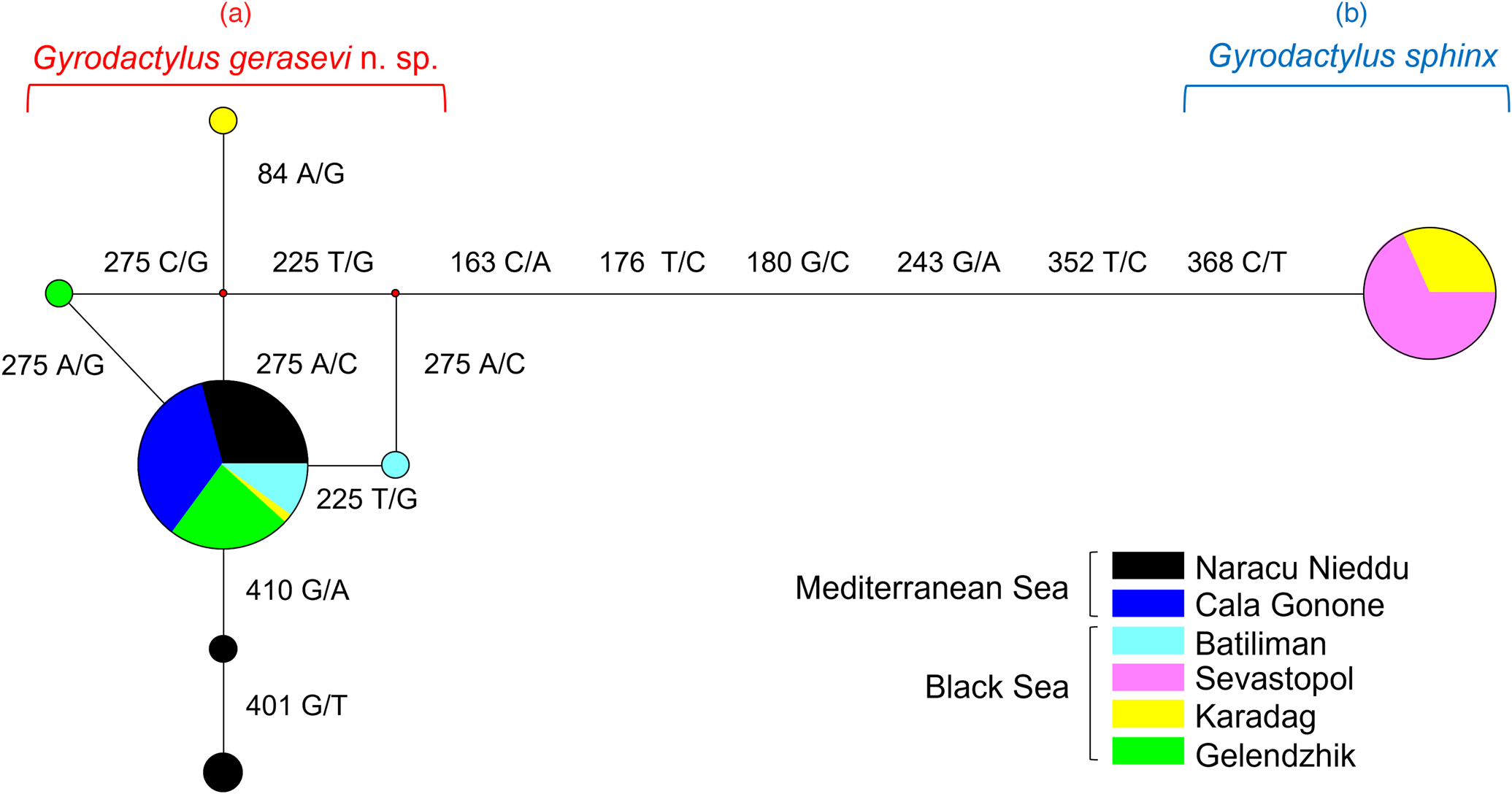
Fig. 6. Median-joining network performed on the ITS2 rDNA dataset with sequences coloured according to the sampling localities. The small red spots on the nodes show median vectors representing the hypothetical connecting sequences calculated with maximum parsimony method. The position of the polymorphic sites (with reference to the 429 bp ITS2 fragment analysed) separating two haplotypes (a – cluster A, Gyrodactylus gerasevi n. sp.; b – cluster B, Gyrodactylus sphinx) and the corresponding nucleotide changes are indicated on the branches.
For the Bayesian phylogenetic tree analysis (fig. 7), almost all the identical sequences obtained in the present study were removed from the dataset analysed, thus including only 12 sequences, which were representative of all (7) the allelic variants found among the samples. Six of these sequences belonged to cluster A of the median-joining network analysis and the other six to cluster B (fig. 6).

Fig. 7. Bayesian phylogenetic tree, performed on the ITS2 rDNA dataset, including all the allelic variants found among the Gyrodactylus specimens analysed in the present study along with the sequences of other Gyrodactylus spp. from GenBank. Values of node supports are expressed in posterior probabilities. Scale bar shows the number of substitutions per site.
The results showed a highly supported monophyletic cluster including all the sequences obtained in the present study. Within this cluster, an internal sub-structuring was evident with a sub-group of sequences exclusive to the Black Sea (with samples from Sevastopol and from Karadag). The whole cluster of gyrodactylids isolated on the three species of Blenniidae from the Mediterranean and Black seas in the present study grouped within a highly supported large clade also including, on a separate sister cluster, the species G. proterorhini, Gyrodactylus sp. sensu Huyse et al. (Reference Huyse, Audenaert and Volckaert2003), G. orecchiae, G. chileani, G. zietarae, G. scartichthys and G. viridae.
The genetic p-distances (see supplementary table S1) calculated among the sequences used to draw the Bayesian tree evidenced that the lowest p-distances for the outgroup cluster, which included G. bubyri, G. corleonis, G. ginestrae, G. rugiensis and G. rugiensoides, correspond to the comparison performed with all the gyrodactylid sequences obtained in the present study. Furthermore, all the sequences isolated in the present study showed the lowest p-distances when compared with G. proterorhini, Gyrodactylus sp. sensu Huyse et al. (Reference Huyse, Audenaert and Volckaert2003), G. orecchiae, G. chileani, G. zietarae, G. Scartichthys and G. viridae. These results are in accordance with the above reported phylogenetic tree analysis (fig. 7).
The ABGD species delimitation method carried out for the ITS2 rDNA region, identified 27 taxonomic entities when checked at the prior maximal distance (P = 0.001) and in 100% of the partitions. Consistently to the phylogenetic tree analysis, all the sequences of Gyrodactylus from the present study were included in a large unique taxonomic entity. The only exception was represented by all sequences from Sevastopol and seven sequences from Karadag, which were included in a different taxonomic unit. This latter taxonomic unit corresponded to the cluster B evidenced in the median-joining network analysis and to the sub-cluster internal to the group of gyrodactylids analysed in the present study that was found in the phylogenetic tree analysis.
The comparison of the 5.8S rDNA 157 bp-long sequences of 16 gyrodactylids ex A. sphynx from the Black Sea, off Sevastopol and one specimen ex S. basilisca from the Mediterranean Sea (see table 2 for details of these specimens), with those of other Gyrodactylus spp. already deposited in GenBank, revealed 20 identical matches including six species – namely, G. orecchiae, G. chileani, G. proterorhini, G. scartichthi, G. viridae, G. zietarae and Gyrodactylus sp. sensu Huyse et al. (Reference Huyse, Audenaert and Volckaert2003).
Overall, the molecular analyses performed here were consistent in evidencing the presence of two genetic groups among the Gyrodactylus samples analysed in the present study. One of them is widespread with a genetic homogeneity among distant geographic areas and is described below as a new species, the other is restricted to the Crimea region, and is herein redescribed as G. sphinx; these species have an identical 5.8S rDNA sequence with the G. orecchiae species group.
Gyrodactylidae van Beneden & Hess, 1863
Gyrodactylus von Nordmann, 1832
Gyrodactylus sphinx Dmitrieva & Gerasev, 2000
Taxonomic summary
-
Synonyms. Gyrodactylus sp. 2 sensu Dmitrieva & Gerasev (Reference Dmitrieva and Gerasev1997).
-
Type host. Aidablennius sphynx.
-
Other hosts. Salaria pavo.
-
Type locality. Black Sea, off Sevastopol (Crimea).
-
Other localities. Black Sea, off Karadag (Crimea).
-
Type site on host. Gills, skin and fins.
-
Type specimens. 23 specimens, n. 702.M.8 s.holo, 703.M.8 s. – 707.M.8 s. IBSS collection.
-
Voucher specimens. 23 specimens, n. 1308.M.8 s – 1330.M.8 s IBSS collection (http://marineparasites.org/taxa/?taxon=66).
-
DNA reference sequences. The 1003 bp sequence encoding partial 18S (8 bp), complete ITS1 (418 bp), 5.8S (157 bp), ITS2 (402 bp) and partial 28S (18 bp) is deposited in GenBank under accession number OL703638.
Redescription
Small worms, with elongate body 192–532 (378 ± 100, n = 24) long × 41–114 (77 ± 21, n = 23) wide. Two pronounced cephalic lobes, each containing a large head organ; paired cephalic glands, anterolateral and posterolateral to pharynx, the latter most massive, 15–20 (n = 3) wide.
Mouth ventrally subterminal, opens in pharynx. Anterior bulb of pharynx 28–32 (30, n = 7) long × 35–43 (38, n = 7) wide, posterior bulb 29–33 (31, n = 7) long × 31–44 (36, n = 7) wide. Oesophagus short, intestinal bifurcate, caeca simple and nonconfluent, extending slightly posterior to testis. Excretory bladders not discernible on mounted worms.
Testis oval 22–33 (28, n = 7) wide. Male copulatory organ, 12.0–17.5 (14.4, n = 14) in diameter, lying just anterior to intestinal bifurcation, round, armed with a large central spine and a row of 6–7 small spines. Uterus with up to two generations of embryos. Seminal receptacle not clearly distinguished from ovary; together, they form the egg-cell-forming region (EGCF; sensu Jones et al., Reference Jones, Ernst and Whittington1997), which lies between testis and uterus. Vitelline cells grouped in posterior part of body behind testis.
Haptor rounded, 43–80 (60, n = 10) long × 54–80 (65, n = 10) wide, with one pair of hamuli, two bars and 16 marginal hooks. Hamuli (fig. 3f) rather massive, ratio HW/HL 0.15–0.2 (0.2 ± 0.01, n = 43) (see table 3 for metrical data of the haptoral hard-parts), with wide root in 2–3 times shorter than shaft, ratio HRL/HShL 0.3–0.5 (0.4 ± 0.03, n = 43); point quite long, its tip almost reaches the anterior edge of the sickle, ratio HPL/HSL 0.7–1.0 (0.8 ± 0.05, n = 43), and arises at acute angle 27–43° (34° ± 3°, n = 43) to the latter (angle between HPL and HSL; fig. 2). Dorsal bar (fig. 3e) transversal elongated, with two posterior triangular projections on both sides of the middle. Ventral bar (fig. 3e) basal part almost thrice as wide as its length, ratio VBAW/VBBL 2.3–3.2 (2.7 ± 0.2, n = 20); with well-marked lateral processes, extending beyond the lateral edges of the basal part, ratio VBAW/VBW 1.2–1.4 (1.3 ± 0.05, n = 20); ventral bar membrane cone-shaped, significant longer than basal part, ratio VBML/VBBL 1.4–2.1 (1.8, n = 17). Marginal hooks (fig. 3f) with long shaft and very short sickle, ratio MSL/ML 0.17–0.22 (0.2 ± 0.01, n = 31); distal and proximal parts of sickle almost equal in width, ratio MDW/MPW 0.7–1.0 (0.9 ± 0.07, n = 31); proximal part with well-pronounced heel and trapezoidal toe, the latter over half of the proximal part width, ratio MTW/MPW 0.4–0.6 (0.5 ± 0.05, n = 31).
Remarks
The first description of Gyrodactylus sphinx was very brief and included a comparison with only one, not very similar, congener (Dmitrieva & Gerasev, Reference Dmitrieva and Gerasev1997). A more detailed comparative analysis is provided herein, since new data on the morphology and phylogeny of G. sphinx were obtained in the present study.
Of the 22 Gyrodactylus spp. recorded in the Mediterranean and Black seas, G. flesi most closely resembles G. sphinx in the shape of the marginal hook with a slim long point, rather massive hamulus with relatively short roots, as well as in the ventral bar with the distinctly lateral processes. However, G. sphinx differs from G. flesi (Malmberg, Reference Malmberg1970) in: (1) the half-length hamulus (28–33 vs. 59–61); (2) the cone-shaped ventral bar membrane, which is trapezoidal in G. flesi; and (3) the shorter length (3.3–3.9 vs. 5) and shape of the marginal hook sickle, having a trapezoidal heel compared to being more tapered towards the end in G. flesi.
It should be noted that the type host and locality of G. flesi are Platichthys flesus and the Baltic Sea, respectively, and the sequences of the ITS2 of specimens from this sea included in the phylogenetic tree analysis were rather divergent from those of G. sphinx (fig. 7). At the same time, in the Black Sea, G. flesi was found on the type host and seven other species (Dmitrieva & Gerasev, Reference Dmitrieva and Gerasev1997), and no DNA sequences of this gyrodactylid from these fish and sea are available.
On the other hand, among the species of the Mediterranean Sea, G. orecchiae grouped in the same cluster with G. sphinx in the phylogenetic tree based on ITS2 rDNA (fig. 7). These species share the following common characters: dimensions and general shape of the marginal hook, rather massive hamulus, ventral bar with well-developed membrane and lateral processes as well as armament of MCO. However, G. sphinx is distinguished from G. orecchiae (Paladini et al., Reference Paladini, Cable, Fioravanti, Faria, Di Cave and Shinn2009) by: (1) the marginal hook sickle with a straightened point (the point tip is turned up in the latter); (2) the straight root of the hamulus, which is inward-folded in G. orecchiae; (3) the cone-shaped ventral bar membrane, which is trapezoidal in G. orecchiae; and (4) the narrower ventral bar lateral processes (1.6–3.8 vs. 6). It is noteworthy that G. orecchiae was described from cage-reared S. aurata in the Adriatic Sea, and its natural host range is unknown.
The group of G. sphinх (fig. 7), besides G. orecchiae, includes six other species – one, G. proterorhini on Proterorhinus semilunaris (Heckel, 1837), from the Danube River, Black Sea Basin (Kvach et al., Reference Kvach, Ondračková, Seifertová and Hulak2019), and five from distant localities, namely G. scartichthi, G. viridae, G. zietarae on Scartichthys viridis Valenciennes, 1836 (Lebedeva et al., Reference Lebedeva, Muñoz and Lumme2021) and G. chileani on Helcogrammoides chilensis (Cancino, 1960) of the Pacific Ocean, off Chile (Ziętara et al., Reference Ziętara, Lebedeva, Muñoz and Lumme2012), and an undescribed Gyrodactylus sp. on Gobius niger Linnaeus, 1758 of the North Sea (Huyse et al., Reference Huyse, Audenaert and Volckaert2003). The first four of the abovementioned species are very different morphologically, mainly in the folded roots of the hamuli or the presence of additional pieces near them (Ergens, Reference Ergens and Bauer1985; Lebedeva et al., Reference Lebedeva, Muñoz and Lumme2021), which are absent in G. sphinх. Whereas, the latter resembles G. chileani (Ziętara et al., Reference Ziętara, Lebedeva, Muñoz and Lumme2012) in the shape of the ventral bar, the dorsal bar with narrowed lateral parts, rather robust hamuli and the marginal hooks with the long point of the sickle, differing in (1) the less pronounced heel and trapezoidal toe of the marginal hook, which is triangular in G. chileani, and (2) the straight root of hamulus compared with inward folded those in the latter. The remaining species of Gyrodactylus of the North Sea differ significantly from G. sphinx in morphology of both hooks and bars.
Gyrodactylus gerasevi n. sp.
Taxonomic summary
-
Type host. Salaria pavo.
-
Other hosts. Salaria basilica, A. sphynx.
-
Type locality. Mediterranean Sea, Naracu Nieddu (North Sardinia).
-
Other localities. Black Sea, off Batiliman (Crimea) and Gelendzhik (Caucasus); Mediterranean Sea, Aquarium of Cala Gonone (West Sardinia).
-
Type site on host. Gills, skin and fins.
-
Type specimens. Holotype n. IBSS collection 713.M.30.holo and 28 paratypes, n. IBSS collection 714.M.30–726.M.30, 729.M.30–739.M.30.
-
Voucher specimens. 76 specimens, n. IBSS collection 727.M.30–728.M.30, 1302.M.30–1307.M.30 (http://marineparasites.org/taxa/?taxon = 785).
-
Etymology. The species is named after the Russian researcher of monogeneans Pavel Gerasev.
-
ZooBank number for species. urn:lsid:zoobank.org:act:236950ED-62EC-476C-BB27-FA6A49383130.
-
DNA reference sequences. The 1002 bp sequence encoding partial 18S (17 bp), complete ITS1 (419 bp), 5.8S (157 bp), ITS2 (402 bp) and partial 28S (7 bp) is deposited in GenBank under accession number OL709356.
Description
Small worms, with elongate body 206–516 (371, n = 15) long × 49–102 (73, n = 15) wide. Cephalic region bilobed, each lobe bears a large head organ; two ducts lead posteriolateral to paired groups of cephalic glands, the most massive posterior bulb 18 (n = 2) wide (fig. 8a).

Fig. 8. Photomicrographs of Gyrodactylus gerasevi n. sp.: (a) anterior part of the body; (b) haptor; (c) male copulatory organ. Abbreviations: C, caeca; CG, cephalic glands; EGCF, egg-cell-forming region; P, pharynx; T, testis; U, uterus.
Mouth ventrally subterminal, directly opens in pharynx comprising two bulbs, anterior 25–32 (29, n = 10) long × 33–44 (39, n = 10) wide and posterior 25–33 (29, n = 10) long × 25–32 (29, n = 10) wide. Intestine bifurcate; caeca simple, terminate blindly behind of posterior margin of testis (fig. 8a).
Testis oval, rather small, 25–30 (28, n = 5) wide. Male copulatory organ lying just anterior to intestinal bifurcation, usually medial, ovate, armed with one large spine and 6–7 small spines in a single row (fig. 8c). Uterus with 1–2, or without, embryos occupying the middle part of body. The EGCF lies between the testis and uterus. Vitelline cells grouped in posterior part of body behind testis.
Haptor rounded, 46–79 (59, n = 13) long × 46–79 (59, n = 13) wide, armed with a pair of hamuli, two bars and 16 marginal hooks (table 3). Hamuli (figs 2 and 3a) rather massive, ratio HW/HL 0.15–0.2 (0.2 ± 0.01, n = 104), with wide root in approximately two times shorter than shaft, ratio HRL/HShL 0.4–0.5 (0.5 ± 0.03, n = 104); point quite long, its tip almost reaches the anterior edge of the sickle, ratio HPL/HSL 0.7–0.9 (0.8 ± 0.04, n = 104), and arises at acute angle 28–42° (34° ± 3°, n = 104) to the latter. Dorsal bar (figs 2 and 3a) transversal elongated, with two posterior triangular projections on both sides of the middle. Ventral bar (figs 2 and 3a) basal part almost thrice as wide as its length, ratio VBAW/VBBL 2.2–3.2 (2.7 ± 0.3, n = 23); with well-marked lateral processes, extending beyond the lateral edges of the basal part, ratio VBAW/VBW 1.1–1.3 (1.2 ± 0.05, n = 22); ventral bar membrane cone-shaped, significantly longer than basal part, ratio VBML/VBBL 1.4–2.4 (2.0 ± 0.3, n = 21). Marginal hooks (figs 2 and 3b) with long shaft and very short sickle, ratio MSL/ML 0.19–0.22 (0.2 ± 0.01, n = 58); distal and proximal parts of sickle almost equal in width, ratio MDW/MPW 0.7–0.9 (0.8 ± 0.06, n = 58); proximal part with well-pronounced heel and trapezoidal toe, the latter over half of the proximal part width, ratio MTW/MPW 0.4–0.6 (0.5 ± 0.05, n = 58).
Remarks
According to the studied anatomical and morphological characters, G. gerasevi n. sp. strongly resembles G. sphinx. Comparison of the measurements of these species by the Student's t-test (table 3) revealed significant differences between the mean values of nine dimensions of haptoral structures, and MCO size; however, the ranges of all these characters overlap significantly, so it is not practically useful for the discrimination of the species. The multivariate discriminant analysis allowed us to correctly divide no more than 80% of the samples of the different species by hamuli measurements, and 70% by those of marginal hook.
Thus, the clear division of G. gerasevi n. sp. from G. sphinx was possible based solely on differences in the sequences of the ITS region – namely, the p-distance between species in the whole ITS2 sequence was 0.0224, in the whole ITS1 sequence was 0.0096 and in the complete ITS1–5.8S–ITS2 sequence was 0.0133.
Among the gyrodactylids of the Black Sea, besides the cryptic G. sphinx, G. gerasevi n. sp. most closely resembles G. flesi in the shape of the marginal hook, hamulus and ventral bar. However, G. gerasevi differs from G. flesi in: (1) the half-length hamulus (27–33 vs. 59–61); (2) the cone-shaped ventral bar membrane, which is trapezoidal in G. flesi; and (3) the shorter length (3.3–3.9 vs. 5) and shape of the marginal hook sickle, having a trapezoidal heel, which is triangular in G. flesi (Malmberg, Reference Malmberg1970).
Among the species of the Mediterranean Sea, G. gerasevi n. sp. and G. orecchiae share the following characters: dimensions and general shape of the marginal hook, rather massive hamulus, ventral bar with well-developed membrane and lateral processes as well as armament of MCO. However, G. gerasevi is distinguished from G. orecchiae by: (1) the marginal hook sickle with a straightened point (the end of the tip is turned up in the latter); (2) the straight root of the hamulus, which is folded inwards in G. orecchiae; (3) the cone-shaped ventral bar membrane, which is trapezoidal in G. orecchiae; and (4) the narrower ventral bar lateral processes (1.7–3.0 vs. 6.0) (Paladini et al., Reference Paladini, Cable, Fioravanti, Faria, Di Cave and Shinn2009).
The group of species most phylogenetically related to G. gerasevi n. sp. (fig. 7), besides G. sphinх and G. orecchiae, includes six other species: G. proterorhini, G. scartichthi, G. viridae, G. zietarae, G. chileani and Gyrodactylus sp. from G. niger. Among these species, G. gerasevi n. sp. most closely resembles G. chileani in the shape of the ventral bar, the dorsal bar with narrowed lateral parts, the rather robust hamuli and the marginal hooks with the long point of the sickle, but differs in: (1) the less pronounced heel and trapezoidal toe of the marginal hook (the latter is triangular in G. chileani); and (2) the straight root of the hamulus, which is folded inward in G. chileani. The remaining five species of Gyrodactylus significantly differ from G. sphinx in the morphology of both hooks and bars (Huyse et al., Reference Huyse, Audenaert and Volckaert2003; Ziętara et al., Reference Ziętara, Lebedeva, Muñoz and Lumme2012; Kvach et al., Reference Kvach, Ondračková, Seifertová and Hulak2019; Lebedeva et al., Reference Lebedeva, Muñoz and Lumme2021).
Discussion
Gyrodactylid fauna in the Mediterranean Sea and Black Sea
The finding of G. gerasevi n. sp. in the present study leads to ten species of Gyrodactylus present in the Mediterranean Sea, to 15 species in the Black Sea and to four species shared between the two basins. However, two of the latter (G. anguillae and G. bubyri) were reported from rivers flowing into the Mediterranean Sea (Vanhove et al., Reference Vanhove, Economou, Zogaris, Giakoumi, Zanella, Volckaert and Huyse2013), and only G. arcuatus was previously found in the coastal ecosystems of the Mediterranean Sea itself (Huyse et al., Reference Huyse, Pampoulie, Audenaert and Volckaert2006). Thus, G. gerasevi can be considered as the second species found in the marine habitats of both seas.
Six of the 15 species of Gyrodactylus recorded in the Black Sea are not currently found in other seas. All of them infect marine fish that are also widespread in the Mediterranean Sea – that is, G. sphinx on Blenniidae; G. crenilabri on Labridae; G. mulli on Mullus barbatus Linnaeus, 1758; G. atherinae and G. ginestrae on Atherina boyeri Risso, 1810; and G. alviga on 13 fish species, with the primary host Merlangius merlangus (Linnaeus, 1758) (Dmitrieva & Gerasev, Reference Dmitrieva and Gerasev1997; Gerasev & Dmitrieva, Reference Gerasev and Dmitrieva2004, Reference Gerasev and Dmitrieva2005; Kvach et al., Reference Kvach, Ondračková, Seifertová and Hulak2019). This fact may be explained by either considering they have not yet been found in the Mediterranean Basin, or by assuming that some of them may have survived in refugia during the regressions and isolation of the ancient Ponto–Caspian Basin, and are consequently present in the modern Black Sea, but not surviving in the neighbouring Mediterranean Basin. This latter explanation has been suggested previously for the three species of Gyrodactylus occurring only in the Black Sea populations of A. boyeri (Kvach et al., Reference Kvach, Ondračková, Seifertová and Hulak2019). Moreover, the following two species of Gyrodactylus were reported for the Black Sea (Dmitrieva & Gerasev, Reference Dmitrieva and Gerasev1997) but not for the Mediterranean Sea: G. flesi, a parasite of Platichthys spp. in the Baltic Sea, also found off the northeast coast of Asia (Zhukov, Reference Zhukov1960) and in the Sea of Okhotsk (Sokolov, Reference Sokolov2010); G. harengi on Clupea harengus Linnaeus, 1758 in the Baltic and White seas and in the North Pacific Ocean (Malmberg, Reference Malmberg1970). Another species which was not found in the Mediterranean Sea is G. mugili, a parasite of Planiliza haematocheila (Temminck & Schlegel, 1845) in the Sea of Japan, on the same host and on Mugil cephalus Linnaeus, 1758 in the Black Sea and Sea of Azov (Maltsev & Miroshnichenko, Reference Maltsev and Miroshnichenko1998), and recently reported in two other mullet species from the Iraqi rivers flowing into the Arabian Gulf (Kritsky et al., Reference Kritsky, Ali and Khamees2013). It is assumed that this species appeared in the Azov–Black seas region together with P. haematocheila introduced from the Sea of Japan, and then switched to the aboriginal M. cephalus (Maltsev & Miroshnichenko, Reference Maltsev and Miroshnichenko1998). However, the finding of G. mugili in Iraq shows that its natural distribution and host range can be greatly underestimated. Lastly, the Ponto–Caspian relic G. proterorhini has been successfully co-introduced with its goby host into many European river basins (Danube, Rhine, Scheldt and Vistula) and has invaded the coastal and estuarine waters of the Baltic and North seas (Ondračková, Reference Ondračková2016). Thus, it is likely that G. gerasevi n. sp. will not be the last finding of gyrodactylids reported in the Black Sea and later in the Mediterranean Sea.
Moreover, until recently, G. sphinx was the only species of Gyrodactylus known for the 387 species of Blenniidae (Hsiu-Chin & Hastings, Reference Hsiu-Chin and Hastings2013). However, three new species – namely, G. scartichthi, G. viridae and G. zietarae – have recently been described on the combtooth blenny S. viridis (Lebedeva et al., Reference Lebedeva, Muñoz and Lumme2021). Therefore G. gerasevi n. sp. represents the fifth species reported for this fish family, and S. pavo and S. basilisca are found as new hosts for gyrodactylids.
Inter- and intraspecific morphometric variability
Some influence of host species, sea and locality was found on the multidimensional morphological characters of the haptoral attachment structures of the investigated Gyrodactylus spp. Moreover, univariate analysis revealed statistically significant differences between the mean measurements of some morphometric characters of G. sphinx and G. gerasevi n. sp. However, about one third of all investigated gyrodactylids were not correctly identified by the discriminant analysis based on these measurements as belonging to host species, seas or regions. Similarly, the two species were not clearly separated on the basis of their morphology.
The morphometric plasticity of the haptoral structures in gyrodactylids is well known, and it is affected by various host and environmental parameters (Malmberg, Reference Malmberg1970; Harris, Reference Harris1998; Geets et al., Reference Geets, Appleby and Ollevier1999; Dmitrieva & Dimitrov, Reference Dmitrieva and Dimitrov2002; Huyse & Volckaert, Reference Huyse and Volckaert2002; Bueno-Silva et al., Reference Bueno-Silva, Boeger and Pie2011). The most important of them is considered to be water temperature (Mo, Reference Mo1991a, Reference Mob; Olstad et al., Reference Olstad, Bachmann and Bakke2009). Perhaps the rather low intraspecific and interspecific variability of the haptoral hard parts found in the studied gyrodactylids could be due to the fact that they have always been sampled in the summer season, with comparable temperature ranges in the different years and localities.
The impact of primary and secondary hosts on the haptoral sclerite size was also found in some gyrodactylid species, reporting trends to increase (Mo, Reference Mo1991a, Reference Mob; Olstad et al., Reference Olstad, Bachmann and Bakke2009) or decrease (Geets et al., Reference Geets, Appleby and Ollevier1999; Dmitrieva & Dimitrov, Reference Dmitrieva and Dimitrov2002) the lengths of hamulus and marginal hook in less suitable hosts. However, no common mode of changes of the measurements of these structures in dependence on fish species was revealed in the examined specimens of G. gerasevi n. sp. found in three host species. Thus, the natural host range and specificity of both G. sphinx and G. gerasevi n. sp. need further study.
The geographical variability in the sizes of the haptoral structures in gyrodactylids is well documented for many species (Malmberg, Reference Malmberg1970; Huyse et al., Reference Huyse, Pampoulie, Audenaert and Volckaert2006; Stoyanov et al., Reference Stoyanov, Huyse, Pankov and Georgiev2016). Spatial isolation is a general, and apparently a primary factor of the geographical variability of the haptoral structures for both the freshwater and marine species of Gyrodactylus, but water salinity can also influence their sizes. For example, the significant variability in the sizes of the haptoral hard parts was revealed for the samples of G. alviga and G. flesi from Black Sea localities with different water salinity (off the Crimean coast and the Bay of Bourgas, off Bulgaria) (Dmitrieva & Dimitrov, Reference Dmitrieva and Dimitrov2002). Indeed, the maximum range of most measurements of G. gerasevi n. sp. were larger in the Mediterranean Sea (table 3: Caucasus vs. Sardinia), where the salinity is higher than in the Black Sea. However, in general, the morphological variability of the 104 specimens of G. gerasevi n. sp. studied from the different hosts and regions of the Mediterranean and Black seas was comparable and even lower than the intraspecific variability reported for other species of Gyrodactylus (Shinn et al., Reference Shinn, Gibson and Sommerville2001).
Multivariate approaches (PCA, Linear discriminant analysis, etc.) to analyse the morphometry of haptoral structures have been successfully applied do discriminate species or different phylogenetic lineages of Gyrodactylus that are morphologically indistinguishable (Huyse & Volckaert, Reference Huyse and Volckaert2002; Shinn et al., Reference Shinn, Hansen, Olstad, Bachmann and Bakke2004; Hahn et al., Reference Hahn, Bakke, Bachmann, Weiss and Harris2011; Xavier et al., Reference Xavier, Faria, Paladini, van Oosterhout, Johnson and Cable2015; Razo-Mendivil et al., Reference Razo-Mendivil, García-Vásquez and Rubio-Godoy2016). However, the investigated gyrodactylids were not discriminated by PCA according to their belonging to the samples from different hosts, seas and regions as well as from different species.
Thus, the examined samples of G. sphinx and G. gerasevi n. sp. from three host species in three regions of the Mediterranean and Black seas are morphologically indistinguishable, and these Gyrodactylus species are apparently cryptic.
Molecular intraspecific variability
The morphological characters used to distinguish the species of Gyrodactylus are related almost exclusively to the shape, size and proportions of several structures of the haptor. Given that perhaps over 20,000 species are tentatively estimated in this genus (Bakke et al., Reference Bakke, Harris and Cable2002), a large number of related species can be expected with non-significant differences in these characters. Thus, the determination of Gyrodactylus species based only on the morphology seems to be unreliable. As a consequence, the separation of cryptic species of Gyrodactylus is often the result of molecular studies (Huyse & Volckaert, Reference Huyse and Volckaert2002; Ziętara & Lumme, Reference Ziętara and Lumme2003; Razo-Mendivil et al., Reference Razo-Mendivil, García-Vásquez and Rubio-Godoy2016; Lumme et al., Reference Lumme, Ziętara and Lebedeva2017). In the context of the present study, where ITS was used to discriminate entities, a genetic distance above 1% between ITS sequences has been suggested as an argument for species separation within the genus Gyrodactylus (Ziętara & Lumme, Reference Ziętara and Lumme2003; Huyse et al., Reference Huyse, Malmberg and Volckaert2004).
The analysis of ITS2 sequences revealed the presence of seven allelic variants among the Gyrodactylus specimens analysed in the present study. The most frequent variant was found in both Mediterranean and Black seas. However, 28% of specimens from the Black Sea, off Crimea, showed a highly divergent allelic variant, resulting in a well-supported separate genetic group. This result suggests the occurrence of a separate taxonomic unit within the Black Sea Gyrodactylus specimens examined, despite no morphological differences being found between the samples.
Among the Gyrodactylus spp., in the case of closely related species, sibling species or even morphologically variable but conspecific taxa, the morphological and molecular data are not always fully concordant. Often, the morphological variability is less than the genetic one (Mo, Reference Mo1991a, Reference Mob; Huyse & Volckaert, Reference Huyse and Volckaert2002; Ziętara & Lumme, Reference Ziętara and Lumme2003; Razo-Mendivil et al., Reference Razo-Mendivil, García-Vásquez and Rubio-Godoy2016; Lumme et al., Reference Lumme, Ziętara and Lebedeva2017), which may be explained by the unification of the morphological adaptations to the same or related hosts, similar environmental conditions, under restriction of the gene flow enhanced by viviparity and progenesis. The sample of morphologically indistinguishable gyrodactylids from A. sphynx in Karadag included three ITS2 allelic variants, genetically distanced in 2%, and an identical level of genetic divergence was observed between specimens from the same host species in Sevastopol and Batiliman, two localities close to each other. Although these populations are sympatric (Karadag) or geographically close enough (Sevastopol and Batiliman), the genotypic differences in eight nucleotides suggested their reproductive isolation.
In such a context, the same, or even less distance, in ITS2 has been observed for G. flesi and G. robustus, having a similar morphology and infecting the same populations of host, and also the morphologically similar G. branchicus and G. rarus, whose taxonomic status has been evaluated (Ziętara & Lumme, Reference Ziętara and Lumme2003), as well as between G. branchialis and G. gondae, and G. rugiensis and G. rugeinsoides, while the conspecific samples from different hosts and regions – namely, G. arcuatus, G. branchicus, G. rarus, G. gondae and G. ostendicus – differed by less than 1%.
Thus, according to the comparison of intra- and interspecific levels of divergences in ITS2 in the aforementioned species and the genetic variability of the gyrodactylids analysed here, as well as the sympatric occurrence of the most distant allelic variants, the existence of two evolutionary entities is assumed.
Taking into account that G. sphinx was described from A. sphynx in the Black Sea, off Sevastopol, the allelic variant of ITS2, which was the only one reported from this fish and locality, was considered to belong to this species. The most widespread ITS2 variant, as well as five other allelic variants, were recognized as belonging to the new species G. gerasevi n. sp. Considering that the degree of genetic isolation among gyrodactylids can be overestimated when specimens are completely asexual, parthenogenetic clones (Ziętara & Lumme, Reference Ziętara and Lumme2003), further studies on this species complex using other regions of the genome (e.g. cytochrome c oxidase I mitochondrial DNA) will be useful to confirm the validity of the species.
The occurrence of the two different species – G. sphinx only in the Black Sea off Crimea and G. gerasevi n. sp. – on the same hosts both in the Mediterranean and Black seas may be explained as a possible consequence of both the geographical distance between localities and the non-migratory habits of the hosts, which resulted in parapatric divergence. It may be also assumed that gyrodactylids have crossed the Bosporus Strait together with their hosts re-migrating into the Black Sea, moving according to the dominant counter-clockwise current along the Anatolian coast (Korotaev, Reference Korotaev2003). Moreover, the lower salinity in the north-western region of the Black Sea, which likely represents a geographic barrier to the dispersal of gyrodactylids, may have further prompted their diffusion along the South (Anatolia) and then north-eastern (Сaucasus) coasts. Therefore, Crimea may have been the last Black Sea region reached by gyrodactylids infecting blenniids. This event could explain the reduced gene flow between ‘Crimean’ specimens and other gyrodactylids, and thus the genetic divergence of G. sphinx, which is exclusive to the Black Sea, off Crimea.
Phylogenetic relationships
The results obtained by the Bayesian phylogenetic tree analysis showed the monophyletic cluster of G. sphinx and G. gerasevi n. sp. included in a large genetic clade with seven other species – namely, G. chileani, G. orecchiae, G. proterorhini, G. scartichthi, G. viridae, G. zietarae and Gyrodactylus sp. sensu Huyse et al. (Reference Huyse, Audenaert and Volckaert2003) – which was previously recognized as a new group of marine species that cannot be placed in any of the subgenera sensu Malmberg (Reference Malmberg1970), and whose ancestors likely crossed the equator northward (Ziętara et al., Reference Ziętara, Lebedeva, Muñoz and Lumme2012).
Despite the geographical distance among the members of this species group, three of the Southeast Pacific species, as well as G. sphinx and G. gerasevi n. sp., infect the same host taxon – that is, blenniids. This suggests a possible relatedness, despite the very wide distribution area of these species. It is noteworthy that the subgenus-specific 5.8S rDNA (Ziętara et al., Reference Ziętara, Huyse, Lumme and Volckaert2002) of G. sphinx and G. gerasevi n. sp. was identical in all the above-mentioned species, confirming the belonging of both species from the Black and Mediterranean seas to this lineage of cross-ocean distributed marine species. The naturalness of this subgenus, its origin and the morphological characters that distinguish its members still require further study.
In conclusion, the variability of morphometry observed in gyrodactylids from blenniids of the Black and Mediterranean seas did not allow us to discriminate distinct species. However, molecular data showed the presence of two separate taxonomic entities – one of them, G gerasevi n. sp., with an overall genetic homogeneity between distant geographical areas of the western Mediterranean Sea and the Black Sea, the other, G. sphinx, grouping only individuals from two Black Sea localities. Both species are cryptic, and they are new members of the cross-ocean distributed G. orecchiae species group.
Supplementary material
To view supplementary material for this article, please visit https://doi.org/10.1017/S0022149X21000778
Acknowledgements
Many thanks to F. Gagliardi and all the Staff of the Aquarium of Cala Gonone, who collaborated and made available the infected specimens of Salaria basilisca.
Financial support
E.D., E.V. and D.P. were supported by the federal budget of the Russian Academy of Sciences (project number 121030100028-0), and by RFBR and Government of Sevastopol (project number 20-44-920004); E.D. was supported by a grant from the Visiting Professor/Scientist Programme of the University of Sassari 2014.
Conflicts of interest
None.
Ethical standards
All applicable institutional, national and international guidelines for the care and use of animals were followed. All studied fishes are listed as a ‘Least Concern’ species by the UICN.
Author contributions
E.D. and D.S. contributed equally to this work, conducted the data analysis and wrote the first draft of the manuscript. D.S. supervised the molecular analysis, E.D. supervised morphometry study. P.M. conceived the study design and provided intellectual comments to the manuscript. E.V. and D.P. collected material from the Black Sea and carried out molecular and morphometry analysis of these samples. P.M., C.B. and M.C.P. collected material from the Mediterranean Sea and participate in their analysis. G.G. and M.C. provided research resources and commented on the project design. All authors read and approved the final manuscript.