Introduction
The musk deer (Moschus berezovskii), which is unique to Asia, is both rare and medicinally useful. It is categorized as endangered in the IUCN Red List (Wang & Harris, Reference Wang and Harris2008) and is a Class I nationally protected wildlife species (Smith et al., Reference Smith, Xie, Hoffmann, Lunde, MacKinnon, Wilson and Wozencraft2010). It is also an economically important species due to its musk, harvested from the scent gland of mature males (Green, Reference Green1986), which is used in perfumes as well as in around 300 traditional Chinese medicine prescriptions (Sheng & Liu, Reference Sheng and Liu2007). Due to the high value of musk, a programme of artificial rearing began in China in the late 1950s. Techniques for obtaining musk without harming the animals were developed to ensure conservation of the deer and the sustainable use of musk. To date, more than 10,000 musk deer have been raised in various provinces, including Sichuan and Shaanxi, and these two provinces account for the majority of musk deer (Wang et al., Reference Wang, Hao and Liu2010a; Li & Jiang, Reference Li and Jiang2014; Cai et al., Reference Cai, Lin, Cheng, Wang, Fu, Zhu, Wu and Yang2016).
Cestodes of the genus Moniezia in the family Anoplocephalidae are an important parasite of herbivorous ruminant animals, including musk deer, causing monieziasis in domestic and wild animals, especially juveniles. These parasites have a worldwide distribution and are present in 30 provinces in China, including Inner Mongolia, Xinjiang, Qinghai, Guizhou, Sichuan and Tibet (Lin & He, Reference Lin and He1975; Shen, Reference Shen2005; Yang & Zhang, Reference Yang and Zhang2013). Monieziasis poses a great threat to artificial breeding programmes by causing high mortality of young deer (Yang et al., Reference Yang, Cai, Sha, Chen and Chen2002). In 1982, a tapeworm found in wild musk deer in Barkam, Sichuan Province, was defined as the new species Moniezia (S.) sichuanensis sp. nov. in a new subgenus (Sinicamoniezia subgen. nov.) of Moniezia, based on the unusual sawtooth-shaped interproglottidal glands situated at the anterior part of each proglottid, the thick vagina and the absence of a cirrus spine (Wu, Reference Wu1982). Subsequent research has focused on the morphological characteristics, biology and epidemiology of M. sichuanensis, as well as controlling monieziasis induced by this species in captive musk deer (Yang et al., Reference Yang, Chen, Cai, Sha and Chen2001a, b). However, genetic information for the genus Moniezia is of limited availability, making it difficult to explore the molecular characteristics of this species. Genetic information has been reported recently for M. expansa, M. benedeni and M. monardi (Ohtori et al., Reference Ohtori, Mikiko and Itagaki2015), as well as two undefined Moniezia species (Wickström et al., Reference Wickström, Haukisalmi, Varis, Hantula and Henttonen2005), making it possible to study M. sichuanensis.
In this study, 12 M. sichuanensis specimens were collected from musk deer in five distinct areas. To better understand the molecular characteristics of M. sichuanensis, the internal transcribed spacer 1 (ITS1) and 5.8S rRNA gene (ITS1–5.8S) of ribosomal DNA were amplified and analysed.
Materials and methods
Tapeworm collection and staining
Twelve tapeworms were isolated from newly retrieved faeces of 12 musk deer after conventional deworming using praziquantel tablets (25 mg/kg body weight, single oral administration). Tapeworms were placed immediately in disposable plastic bags, labelled and shipped to Sichuan Agricultural University. A single M. expansa tapeworm from a goat was provided by the Department of Parasitology, Sichuan Agricultural University. Specific information about the tapeworm samples is shown in table 1 and fig. 1. Each individual cestode was washed in saline and divided into two parts (mature and immature). Mature segments were fixed in 70% alcohol and stained with carmine for morphological examination of the interproglottid region and reproductive organs (Wu, Reference Wu1982; Yang et al., Reference Yang, Sha, Cai, Chen and Chen2001b; Yang & Zhang, Reference Yang and Zhang2013). Immature segments were frozen and stored at −70°C for DNA extraction.

Fig. 1. Sampling sites from which the 12 M. sichuanensis specimens were collected from musk deer raised in Sichuan and Shaanxi provinces, China.
Table 1. Species information and GenBank accession numbers of the ITS1–5.8S sequences for Moniezia spp.

DNA extraction, PCR amplification and amplicon sequencing
Total DNA was extracted from immature segments of the 13 samples (12 from musk deer and one from goat) using a QIAamp DNA Stool Mini Kit (Qiagen, Hilden, Germany) according to the manufacturer's protocol. Primers ITS1-F (5′-GCTGCTACCCGCATGATGTT-3′) and ITS1-R (5′-GGCAAGCCTATAGCCGCAAT-3′) (Nguyen et al., Reference Nguyen, Le, Huynh, Nguyen, Nguyen and Vu-Khac2012) were used to amplify the ITS1–5.8S region of ribosomal DNA. Polymerase chain reaction (PCR) was performed in a 25-μl reaction volume containing 12.5 μl of Taq PCR Master Mix (Tiangen Biochemical Technology Co., Ltd., Beijing, China), 8.5 μl of double-distilled water (ddH2O), 2 μl of DNA template and 1 μl of each forward and reverse primer (working concentration = 10 pmol/l). Reaction cycles consisted of an initial denaturation step at 94°C for 5 min; followed by 30 cycles of 94°C for 45 s, 56°C for 30 s and 72°C for 45 s; with a final extension of 72°C for 10 min. PCR products were visualized by 1% agarose gel electrophoresis using GoldenView staining. Amplicons of expected size were purified and cloned into the pMD19-T vector and introduced into Escherichia coli (DH5α). To ensure maximum accuracy, three positive colonies of each sample were sent for commercial sequencing (Invitrogen, Shanghai, China). Consensus sequences were utilized for subsequent analyses.
Phylogenetic analysis
The BLAST program (http://www.ncbi.nlm.nih.gov/BLAST) was used to compare the obtained nucleotide sequences with published gene sequences from other Moniezia spp. deposited in the National Center for Biotechnology Information (NCBI). The corresponding sequences are listed in table 1. Multiple sequence alignment was performed using DNAMAN software package, 2005 (Lynnon Corporation, San Ramon, California, USA) and the genetic similarities between species were also determined using this software. Phylogenetic trees of relevant species were constructed by the neighbour-joining method using MEGA 5.0 (http://www.megasoftware.net) based on genetic distances calculated by the Kimura 2-parameter model. The robustness of the tree was tested by bootstrapping with 1000 replicates. Phylogenetic analysis was performed using sequences generated from PCR analysis of the ~760 bp ITS1–5.8S fragment along with sequences obtained from NCBI (table 1). Dipylidium caninum (GenBank accession number: AM_491338) was used as the outgroup.
Results
The interproglottidal glands from all 12 specimens from musk deer had a clear sawtooth shape, the cirrus spine was absent and the vagina was thick. Based on previously published work (Wu, Reference Wu1982) (fig. 2), all 12 specimens from the five areas were identified as M. sichuanensis via morphological analysis. A 761–764 bp ITS1–5.8S fragment and a 754 bp ITS1–5.8S fragment were successfully amplified. All sequence information has been deposited in GenBank under accession numbers KX377878–KX377890.
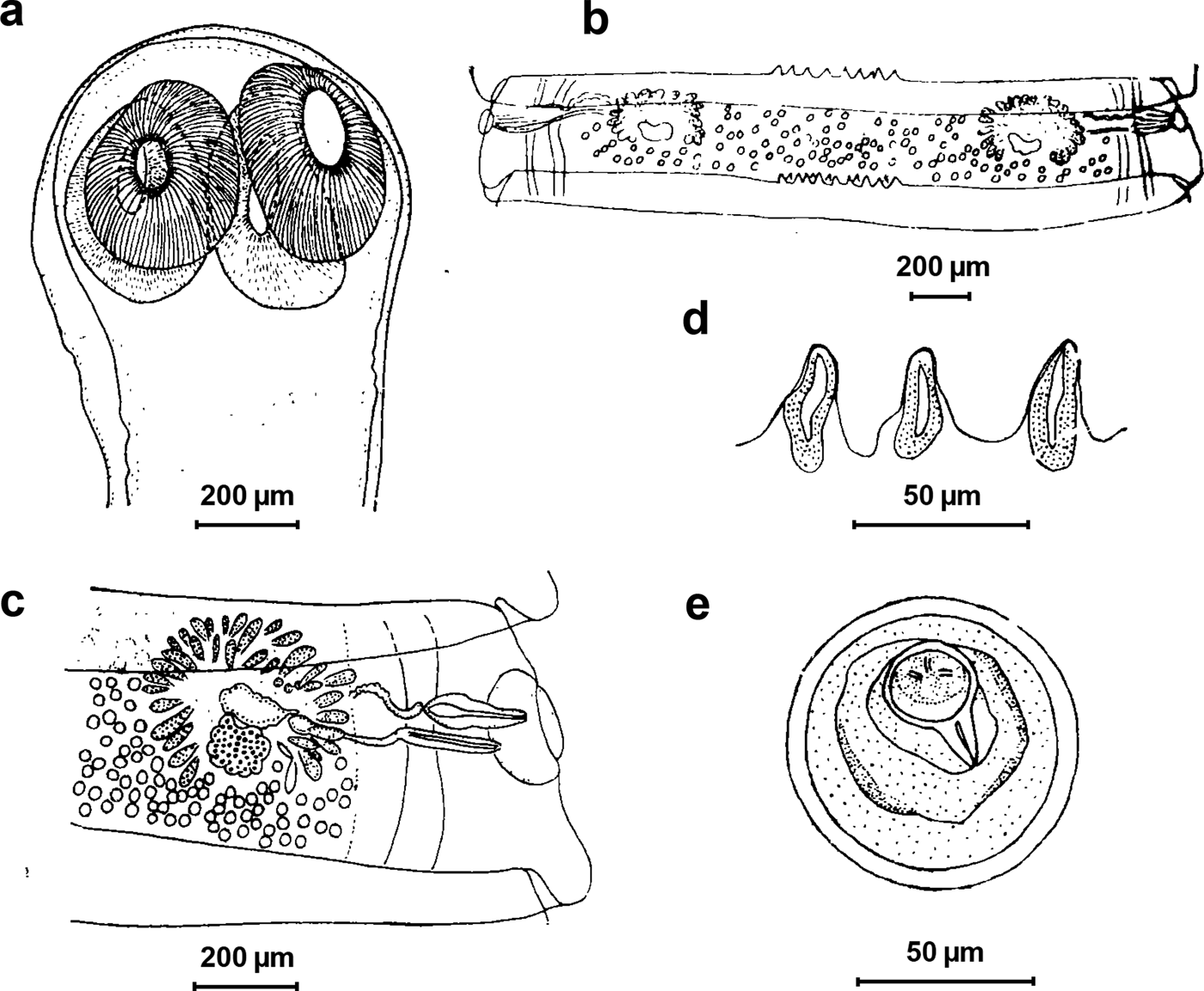
Fig. 2. Line drawings of Moniezia (S.) sichuanensis sp. nov.: (a) scolex; (b) mature proglottid; (c) mature proglottid (reproductive organs magnified); (d) interproglottidal glands; and (e) eggs. Drawings and interpretation are based on previously published work (Wu, Reference Wu1982).
Multiple alignment showed that ITS1–5.8S sequences amplified from the 12 M. sichuanensis specimens shared between 98.7 and 100% similarity, and 67.8–92.4% similarity with other Moniezia spp. Similarity among the seven samples from Sichuan province was between 99.2 and 100%, and similarity was 99.6–100% among the five samples from Shaanxi province. As shown in table 2, nucleotide indels and substitutions were observed in the ITS1–5.8S sequences of the 12 M. sichuanensis specimens. According to the phylogenetic tree based on ITS1–5.8S sequences (fig. 3), the 12 M. sichuanensis samples clustered together (98.7–100% similarity), and also with M. monardi (92% similarity), M. expansa (77% similarity), M. benedeni (81% similarity), and two other unnamed Moniezia cestodes (77% and 74% similarity) in another branch. Based on the morphological observations and phylogenetic analyses, M. sichuanensis was confirmed as a separate species distinct from known species.

Fig. 3. Phylogenetic relationships between cestodes isolated from musk deer and other species of the genus Moniezia. Phylogeny was inferred based on the amplified ITS1–5.8S fragment using the neighbour-joining method. Numbers on nodes indicate bootstrap values resulting from phylogenetic analysis, and values less than 50% are not shown.
Table 2. Multiple sequence alignment of ITS1–5.8S fragment amplified from the 12 M. sichuanensis specimens.

Specimen ID and nucleotide positions of point mutations are shown. 5.8S starts from nucleotide 633 in MEK01. Only variable positions are shown: ’.’ indicates that the nucleotide position corresponds to the MEK01 reference isolate, and ‘–’ represents an alignment gap.
Discussion
Defining species within the genus Moniezia has proved controversial, and in an attempt to simplify the systematics of this genus, it was divided into three subgenera based on the presence or absence and shape of interproglottidal glands: (1) M. baeriezia (interproglottidal glands absent), including M. mettami, M. rugosa and M. baeri; (2) M. moniezia (rosette-shaped interproglottidal glands) including M. expansa and M. monardi; (3) Moniezia blanchariezia (line-shaped interproglottidal glands) including M. benedeni and M. pallida (Skrjabin & Schulz, Reference Skrjabin and Schulz1937). In his revision of the Anoplocephalidae tapeworms, Spassky (Reference Spassky1951) approved this division and concluded that the above-named species were valid. Although at least 12 Moniezia species have been reported in domestic and wild ruminants, it is difficult to distinguish them at the species level based on morphology alone. Among these, M. expans a and M. benedeni are the most widespread and harmful species (Schmidt, Reference Schmidt1986). Currently, morphological classification of Moniezia is mainly based on the size of adult worms and the morphology of the scolex, interproglottidal glands, reproductive organs and eggs (Machida et al., Reference Machida, Matsumura and Watanabe1974; Liu et al., Reference Liu, Wang and Bo2008; Wang et al., Reference Wang, Liu and Bo2010b).
Using pathogens to detect monieziasis relies on the identification of eggs and/or a gravid proglottid. However, the shape of eggs is similar among closely related Moniezia species. In addition, excreted eggs are sometimes deformed, preventing morphological differentiation at the species level (Taylor, Reference Taylor1967; Chilton et al., Reference Chilton, O'Callaghan, Beveridge and Andrews2007). Identification via the proglottids is difficult because some individuals lack interproglottidal glands, and the proglottids excreted in the faeces sometimes lack interproglottidal glands.
In recent years, molecular methods have been introduced that help the classification and determination of Moniezia spp., with cytochrome c oxidase subunit 1 (COX1) and ITS genes being the most frequently used markers (Nguyen et al., Reference Nguyen, Le, Huynh, Nguyen, Nguyen and Vu-Khac2012; Diop et al., Reference Diop, Yanagida, Hailemariam, Menkir, Nakao, Sako, Ba and Ito2015; Ohtori et al., Reference Ohtori, Mikiko and Itagaki2015). ITS could serve as a good genetic marker because it can differentiate M. expansa and M. benedeni recovered from cattle, goats and sheep (Nguyen et al., Reference Nguyen, Le, Huynh, Nguyen, Nguyen and Vu-Khac2012). In addition, ITS can discriminate M. monardi from Japanese serows, M. benedeni from cattle and M. expansa from sheep (Ohtori et al., Reference Ohtori, Mikiko and Itagaki2015). Due to sequence variation caused by the rapid evolution of ITS genes, this gene could contribute to our understanding of the intra- and interspecific phylogenetic relationships among Moniezia spp., as well as the identification of a new species at the molecular level (Bowles et al., Reference Bowles, Blair and McManus1995; Fazaeli et al., Reference Fazaeli, Carter and Pennington2000).
In the present study, the ITS1–5.8S sequences obtained from the 12 M. sichuanensis specimens showed some nucleotide variation. However, the similarity ranged from 98.7 to 100%, and between 67.8 and 92.4% when compared with other Moniezia spp. The phylogenetic trees showed that all 12 samples were closely related to each other and clustered together in the same strain. Moniezia monardi, sharing a similarity of 92.4%, was the species most closely related to M. sichuanensis. However, the original description of this species was based on material found in a reedbuck in Angola (the original paper is not available), while the genetic information was reported from Japanese serow (Capricornis crispus) (Machida et al., Reference Machida, Matsumura and Watanabe1974; Ohtori et al., Reference Ohtori, Mikiko and Itagaki2015). It is worth noting that the main feature that distinguishes Moniezia spp. is the interproglottidal glands. The interproglottidal glands of M. expansa and M. monardi are grouped around depressions that open to the surface of the segment (rosette type) (Machida et al., Reference Machida, Matsumura and Watanabe1974; Liu et al., Reference Liu, Wang and Bo2008; Wang et al., Reference Wang, Liu and Bo2010b; Ohtori et al., Reference Ohtori, Mikiko and Itagaki2015). However, genetic analysis showed that these two species are in different branches (75% identity), indicating a distant relationship (fig. 3). This is consistent with the report of Ohtori et al. (Reference Ohtori, Mikiko and Itagaki2015). Although a relatively high sequence similarity was observed between M. monardi and M. sichuanensis (92.4%), this variation exceeded the intraspecies variation (98.7–100%) within the M. sichuanensis group. Additionally, due to the shape of the interproglottidal glands (rosette-shaped vs. sawtooth-shaped), M. monardi and M. sichuanensis were thought to be different species belonging to different subgenera of the genus Moniezia.
In summary, this study reports the first molecular information on M. sichuanensis, thereby improving our understanding of the taxonomy and phylogenetic relationships of species in the genus Moniezia. Moreover, the combined morphological and molecular information on M. sichuanensis could facilitate the future identification of Moniezia spp. and the control of monieziasis.
Acknowledgements
We would like to thank Baojian Wang for his help in data analysis and Keling Jian for kindly providing the cestode specimen from a goat.
Financial support
This work was supported by the Sichuan Science and Technology Support Program (grant numbers 2014|SZ0132, 2014Zc2733).
Conflict of interest
None.
Ethical standards
All procedures were reviewed and approved by the Animal Welfare Committee of China. During faecal collection, animal welfare was ensured throughout the sampling process.
Authors’ contributions
J.X. and H.L. participated in the design of the study, performed all experiments, collected and analysed the data and wrote the manuscript; J.C., W.F., Y.C. and H.W. helped in collecting the faecal samples; X.G., W.L. and X.P. participated in the processing of specimens; G.Y. devised the study, participated in its design and coordination, helped to interpret the results and edited the manuscript. All authors read and approved the final manuscript.