Introduction
The blue whiting (Micromesistius poutassou) (Risso, 1827) is a small oceanic and benthopelagic gadoid fish, broadly distributed in North Atlantic waters and the Mediterranean Sea. The species is of considerable economic importance with total catches reaching 1538 kt in 2017, of which Norway was the largest producer with close to 400 kt (FAO, 2020). The parasite fauna of blue whiting seems to have a core of parasite species present throughout its north-east (NE) Atlantic distribution. The dominating parasite species appear to be the anisakid nematode Anisakis simplex (s.s.) and the coccidian Goussia sp., with prevalence reaching 100% for both parasites in catches from the NE Atlantic Ocean and the North Sea (Berland, Reference Berland2006; Levsen et al., Reference Levsen, Svanevik, Cipriani, Mattiucci, Gay, Hastie and Pierce2018).
Many studies have focused on the adverse health effects of larval nematodes on marine fish species (reviewed by Buchmann, Reference Buchmann2012; Mackenzie & Hemmingsen, Reference Mackenzie and Hemmingsen2014; Horbowy et al., Reference Horbowy, Podolska and Nadolna-Altyn2016; Mladineo et al., Reference Mladineo, Popovic, Drmic-Hofman and Poljak2016; Debenedetti et al., Reference Debenedetti, Madrid, Trelis, Codes, Gil-Gómez, Sáez-Durán and Fuentes2019). Formation of granulomas on the surface of internal organs (Buchmann & Mehrdana, Reference Buchmann and Mehrdana2016; Dezfuli et al., Reference Dezfuli, Manera, DePasquale, Pironi and Giari2017) or in the skeletal musculature is a common response to larval nematodes in fish (Umberger et al., Reference Umberger, de Buron, Roumillat and Mcelroy2013; Cipriani et al., Reference Cipriani, Acerra, Bellisario, Sbaraglia, Cheleschi, Nascetti and Mattiucci2016). Granulomas are focal chronic inflammatory lesions that appear as nodules in tissues and organs of the host (Ferguson, Reference Ferguson2006; Dezfuli et al., Reference Dezfuli, Fernandes, Galindo, Castaldelli, Manera, DePasquale and Giari2016). Cellular immune responses in fish–parasite systems generally involve the following cell components: neutrophils (Jørgensen et al., Reference Jørgensen, Korbut, Jeberg, Kania and Buchmann2018; Furtado et al., Reference Furtado, Cardoso, Figueredo, Marchiori and Martins2019), mast cells (MCs) (Reite & Evensen, Reference Reite and Evensen2006; Buchmann, Reference Buchmann2012; Dezfuli et al., Reference Dezfuli, Giari, Lorenzoni, Carosi, Manera and Bosi2018), macrophage aggregates (MAs) (Agius & Roberts, Reference Agius and Roberts2003; Dezfuli et al., Reference Dezfuli, Manera, DePasquale, Pironi and Giari2017) and epithelioid cells (Ferguson, Reference Ferguson2006; Dezfuli et al., Reference Dezfuli, Manera, DePasquale, Pironi and Giari2017).
The present study aimed to perform a histochemical, immunohistochemical and ultrastructural analysis of different organs of blue whiting from the NE Atlantic Ocean parasitized by Anisakis simplex (s.s.) and Goussia sp. to document the immunohistopathological responses to the parasites. Our results are the first to provide data on the cellular component of the granuloma around Anisakis simplex (s.s.) larvae in the liver of a fish. Indeed, scanning electron microscopy on histological sections of fish liver with any helminth has not been reported previously.
Materials and methods
Fish sampling and epidemiological data
Samples of blue whiting (n = 150 + 30) were obtained from a commercial fishing catch in April 2018 off St Kilda (N58°04′ W09°40′) in the NE Atlantic Ocean (FAO 27 area, Division VI a), on board the fishery and research vessel MS Kings Bay (Institute of Marine Research, cruise no. ‘Kings Bay’ 2018843).
Tissue samples of n = 30 fish were collected and processed for immunohistochemistry analysis within 1 hour post-catch. Thus, the fish stomach, pyloric caeca, intestine, liver, gonads and skeletal muscles were inspected for the presence of Anisakis sp. larvae. Pieces of organ tissue (approx. 15 × 15 × 10 mm) containing Anisakis larvae were excised, photographed and then fixed in 10% neutral buffered formalin for 24 h. For negative control, tissue pieces of a single uninfected liver were processed the same way as infected organ samples. After 24 h, formalin was substituted with ethanol 70% and the samples were stored and prepared for transport to the laboratory.
Furthermore, 150 specimens were randomly selected from the same fishing catch and inspected for detection of anisakid nematodes and other parasites, to be used as a reference for overall infection data. Fish were weighed, measured and disposed for parasitological survey, conducted by the UV press method as previously described (Levsen et al., Reference Levsen, Svanevik, Cipriani, Mattiucci, Gay, Hastie and Pierce2018). The larvae localized in the fish host were counted and morphologically assigned to the genus level using bright-field microscopy following the diagnostic keys of Berland (Reference Berland1961). Subsequently, a subsample of 80 Anisakis spp. Larvae, randomly selected from morphologically confirmed Anisakis type I larvae, were washed in saline solution and stored at –70°C for further genetic identification.
Molecular identification
A total of n = 100 Anisakis type I larvae were identified to the species level based on the direct sequence analysis of mitochondrial (mtDNA cox2) and nuclear (elongation factor EF1 α-1 of nDNA) gene loci. Total DNA was extracted from a tissue portion (≈2 mg) of each Anisakis larva. The Quick-gDNA™ Miniprep Kit (ZYMO RESEARCH) was used as extraction method. DNA obtained was quantified using a NanoDrop®TC1-E20 spectrophotometer (BioTek Synergy HT).
For the sequencing of the mtDNA cox2 gene locus, amplification was performed using the primers 211 F (5′-TTT TCT AGT TAT ATA GAT TGR TTT YAT-3′) and 210R (5′-CAC CAA CTC TTA AAA TTA TC-3′) (Mattiucci et al., Reference Mattiucci, Cipriani, Webb, Paoletti, Marcer, Bellisario and Nascetti2014). Polymerase chain reaction (PCR) was carried out according to the procedures provided by Mattiucci et al. (Reference Mattiucci, Cipriani, Webb, Paoletti, Marcer, Bellisario and Nascetti2014). The sequences obtained were compared with those already deposited for the same gene in GenBank for other Anisakis species. Anisakis spp. larvae, identified by the mtDNA cox2 gene, were sequenced at the elongation factor (EF1 α-1 nDNA) nuclear gene. The EF1 α-1 nDNA was amplified using the primers EF-F (5′-TCCTCAAGCGTTGTTATCTGTT-3′) and EF-R (5′-AGTTTTGCCACTAGCGGTTCC-3′) (Mattiucci et al., Reference Mattiucci, Acerra, Paoletti, Cipriani, Levsen, Webb and Nascetti2016). The PCR conditions and procedures followed those previously reported (Mattiucci et al., Reference Mattiucci, Acerra, Paoletti, Cipriani, Levsen, Webb and Nascetti2016). The sequences obtained for the EF1 α-1 nDNA gene for the larval specimens were compared with those previously deposited in GenBank, at the diagnostic positions (i.e. 186 and 286) as previously detailed (Mattiucci et al., Reference Mattiucci, Acerra, Paoletti, Cipriani, Levsen, Webb and Nascetti2016).
Quantitative infection assessment focused on nematode prevalence and abundance values, separately for liver, rest of viscera and skeletal muscle of fish. The epidemiological parameters considered were prevalence (P, %), mean abundance (mA), and mean intensity (mI) with standard deviation (±SD) and range of infection (min–max).
Histopathology
For histological purpose, fixed pieces of visceral organs and skeletal muscles of 30 M. poutassou were sent to the University of Ferrara (Italy). Each organ sample was embedded in paraffin wax and cut with routine histological techniques. Multiple 5-μm sections were taken from each tissue block, stained with either Alcian Blue–haematoxylin and eosin (AB/HE), Alcian Blue 8 GX pH 2.5 and periodic acid Schiff's (AB/PAS) and Giemsa, examined and photographed using a Nikon Microscope ECLIPSE 80i (Nikon, Tokyo, Japan).
Histochemistry and immunohistochemistry
Immunohistochemistry tests were carried out on sections (4–6 μm) of liver, gonads, pyloric caeca and skeletal muscles using the antibodies and dilution indicated in table 1. The sections were rehydrated and treated for 20 min with 3% H2O2 in Tris-buffered saline (TBS) pH 7.6 (TBS: 0.05 M Tris-HCl, 0.15 M NaCl) to block endogenous peroxidase. Slides used for the detection of the rabbit polyclonal anti-tumour necrosis factor (TNF)-α and mouse monoclonal Mast cell tryptase antibodies were heated 2 × 5 min in microwave at 500 W in 0.01 M citrate buffer pH 6.0 for antigen retrieval. Sections were rinsed 2 × 5 min in TBS, then incubated for 30 min with 1:20 goat normal serum or with 1:20 rabbit normal serum to avoid tissue-unspecific reaction. The use of one or the other normal serum depends on the host species in which the used primary antibody was produced (see table 1). After a washing step of 2 × 5 min in TBS, sections were incubated at room temperature for 24 h with the primary antibody. The optimum dilution for each antibody was determined by several trials in our laboratories. Slides treated with polyclonal anti-rabbit primary antibodies were washed twice for 5 min in TBS and incubated for 1 h with 1:200 biotinylated anti-rabbit immunoglobulins (Vector Labs, Burlingame, CA, USA). The sections treated with monoclonal anti-mouse primary antibodies were incubated for 1 h with 1:200 biotinylated anti-mouse immunoglobulins (Vector Labs). Finally, slides were treated for 1 h at room temperature with the streptavidin-biotin/horseradish peroxidase complex (Vectastain® ABC kit, Vector Labs) and the reaction was developed with a freshly prepared solution of 4 mg of 3,3'-diaminobenzidine tetrahydrochloride (Sigma-Aldrich, St. Louis, MO, USA) in 10 ml of 0.05 M TBS containing 0.1 ml of 3% H2O2. After 15 min washing steps in running tap water, sections were quickly washed in TBS, counterstained for 15 min with Mayer's haematoxylin, de-hydrated and mounted with Eukitt.
Table 1. Antibodies used in the present study, their specificity, source and dilution.
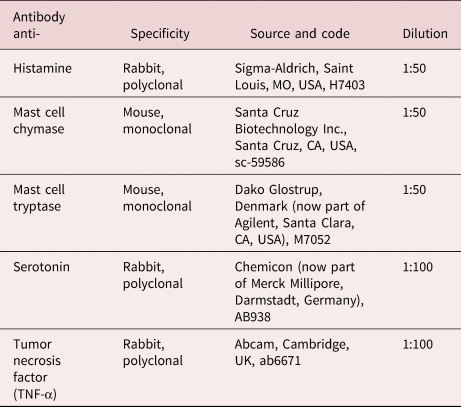
In liver, the immunohistochemical results could be invalidated by the presence of melanin in the MAs. A melanin bleaching protocol was used to remove melanin (Chung et al., Reference Chung, Choi and Sears2016), treating liver sections with 0.5% H2O2 in 10 mm Tris/HCl pH 10.0 at 80°C for 15 min prior of the primary antibody incubation. Further control protocols were performed by (1) incubation step with phosphate-buffered saline (PBS) pH 7.4 instead of the primary antibody; (2) treatment with PBS instead of the secondary antibody; (3) melanin bleaching treatment prior of the incubation step with PBS instead of the primary antibody; (4) melanin bleaching protocol prior of the treatment with PBS instead of the secondary antibody. All these procedures gave the expected results.
Transmission electron microscopy
For transmission electron microscopy, several pieces mostly up to 7 mm in size of all ten infected livers and one single liver with no Anisakis sp. larva were fixed in 2.5% glutaraldehyde in 0.1 M sodium cacodylate buffer for 3 h at 4°C and embedded in Durcupan ACM resins. Stained ultrathin sections were examined in a Hitachi H-800 at an acceleration of 100 kV as detailed in Dezfuli et al. (Reference Dezfuli, Giari, Lorenzoni, Carosi, Manera and Bosi2018).
Scanning electron microscopy
For scanning electron microscopy (SEM), pieces of ten livers with visible encapsulated Anisakis sp. larvae were prepared with routine techniques for SEM. Furthermore, 7-μm histological sections from the above infected livers and sections from a single liver free of Anisakis sp. larvae were placed on coverslips, then dehydrated through a graded ethanol series and coated with gold in an Edward S 150 sputter coater. Samples were then examined with a Cambridge Stereoscan 360 (London, UK) at an acceleration voltage of 20 kV.
Results
Parasite molecular identification and epidemiological data
Out of the n = 150 M. poutassou specimens analysed, 69 were males and 81 females (total length, mean ± SD = 273.5 ± 27.4 mm; mean weight ± SD = 104.1 ± 38.4 g) (table 2).
Table 2. Anisakis simplex (s.s.) infection in Micromesistius potassou.

n, number of fish; TL, mean total length ± SD; TW, mean total weight ± SD; Infected, number of infected fish; P (%), prevalence; A, mean abundance; mI (±SD), mean intensity ± SD with range (min–max); NLTot (%), number of total larvae and percentage in the indicated site of infection.
A total of 5816 nematode larvae were recovered from the viscera and flesh of the examined fish. Based on basic diagnostic morphological characters, all the third-stage larvae (L3) nematodes were recognized as Anisakis spp. showing larval Type I characters (sensu Berland, Reference Berland1961).
According to the sequences of 629 bp in length from the mtDNA cox2 gene locus, all the Anisakis spp. larvae (n = 100) were assigned to the species A. simplex (s.s.). The sequences obtained matched 99–100%, with the sequences previously deposited in GenBank for the species. In addition, the identity of the specimens recognized by mtDNA cox2 sequences analysis as belonging to A. simplex (s.s.) was confirmed by sequence analysis of the locus EF1 α-1 nDNA, showing of the two diagnostic nucleotide positions characteristic for the species A. simplex (s.s.), as reported in Mattiucci et al. (Reference Mattiucci, Acerra, Paoletti, Cipriani, Levsen, Webb and Nascetti2016).
A total of ten mtDNA cox2 sequences were deposited in GenBank (accession numbers: from MT989556 to MT989565).
Anisakis simplex (s.s.) larvae were detected in the liver (fig. 1a, b, c), the viscera, muscle and gonad of the fish (fig. 2a, b), in the muscle the larvae were encapsulated in tissue (fig. 2a). Data of prevalence (P), mean abundance (mA), and mean intensity (mI) of A. simplex (s.s.) larvae at different sites of infection (liver, rest of viscera and muscle) are given in table 2.

Fig. 1. Photos after necropsy of blue whiting, Micromesistius poutassou. (a) numerous Anisakis simplex (s.s.) (L3) larvae (arrows) encapsulated on surface of liver are visible; (b) portion of infected liver with some A. simplex (s.s.) larvae (arrows); (c) heavily infected liver, all the surface of the organ is covered with encapsulated anisakid larvae.
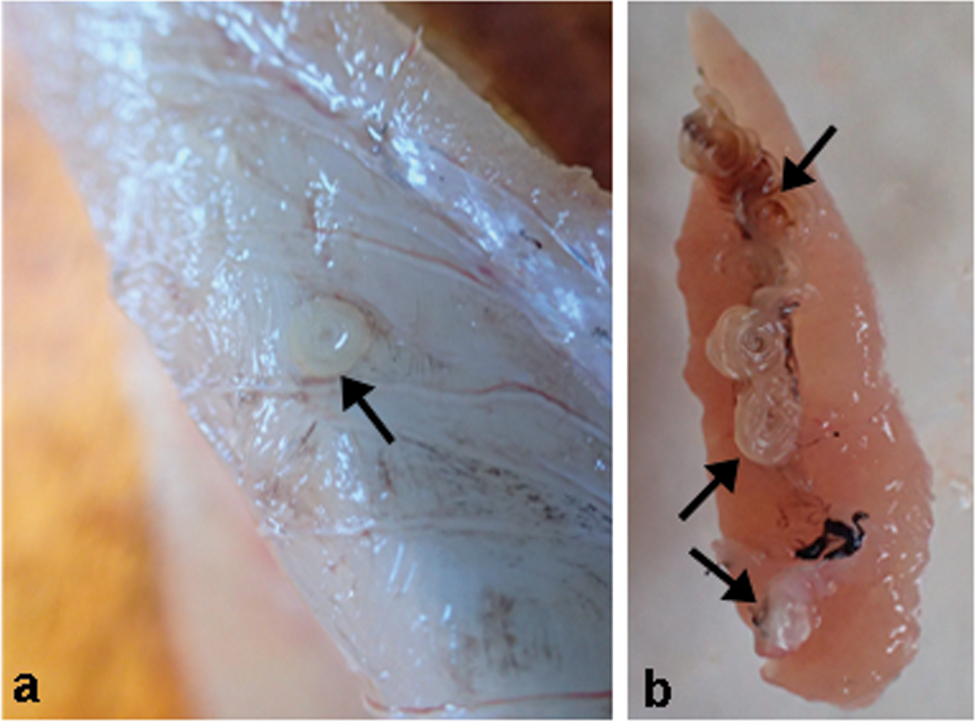
Fig. 2. Photos after necropsy of blue whiting, Micromesistius poutassou. (a) larva of Anisakis simplex (s.s.) (arrow) is encapsulated in host skeletal muscle; (b) ovary of M. poutassou with A. simplex (s.s.) larvae (arrows) encysted on organ surface.
Only one fish out of the 150 was not infected with A. simplex (s.s.). The overall prevalence recorded for A. simplex (s.s.) was P = 99.3% (149 infected out of 150), with mean intensity 39 (table 2). Prevalence and mean intensities in liver and rest viscera were P = 98.7%, mI = 24.6; and P = 97.3%, mI = 8.2, respectively (table 2). The prevalence in the skeletal muscle was P = 90.7%, with mI = 7.2. The relative proportion of A. simplex (s.s.) larvae by infection site was 62.7% in liver, 20.5% of larvae in the rest of viscera and 16.8% in the skeletal muscle (table 2). In a few M. poutassou, larvae of A. simplex were observed on the surface of the testes and ovaries (fig. 2b).
Histopathology
During dissection and then observations of histological sections of visceral organs and flesh, it was noted that liver, gonads, pyloric caeca and hypaxial flesh surrounding visceral cavity were the organs infected with encapsulated A. simplex (s.s.) larvae. Among them, liver was the most parasitized organ, with the presence of numerous larvae on its surface (fig. 3a) and, frequently, also inside the hepatic tissue. Infected liver showed varying degrees of alterations ranging from hydropic degeneration (cellular oedema) to acute cell swellings (fig. 3b, c and fig. 5a, e). Additionally, the liver always harboured oocysts of the coccidian Goussia sp. (100% prevalence was observed also in the 30 samples used for immunohistochemistry analyses) (figs. 3b, c and 4a, b, c). Moreover, single macrophages, and MAs, were found scattered in the parenchyma and, inside the MAs, engulfed Goussia sp. oocytes were often noticed (fig. 3c).

Fig. 3. Histological sections of infected organs of Micromesistius poutassou. (a) periphery of liver with encysted A. simplex (s.s.) larvae (arrows)(alcian blue and periodic acid Schiff's, AB/PAS stain), scale bar = 200 μm; (b) micrograph shows liver with co-occurrence of A. simplex (arrow) and coccidian Goussia sp. (curved arrows)(AB/PAS stain), scale bar = 20 μm; (c) high magnification of macrophage aggregates (MAs) in liver, several Goussia sp. (arrows) are engulfed by MAs, note acute swelling of hepatocytes (alcian blue and haematoxylin & Eosin, AB/H&E stain), scale bar = 20 μm; (d) longitudinal section of a pyloric caecum with A. simplex (s.s.) larva (arrow) externally encysted, lack of cellular response around nematode is evident (AB/H&E stain), scale bar = 200 μm.

Fig. 4. Micrographs of immunohistochemical reactions on infected liver of Micromesistius poutassou. (a) periphery of liver, co-occurrence of Goussia sp. (curved arrows) and A. simplex (s. s.) larva (asterisk) is evident, note positive immunoreactivity of macrophage aggregates (MAs) to anti-mast cell tryptase (arrows), scale bar = 100 μm; (b) high magnification of MAs positive to anti-mast cell tryptase, some Goussia sp. (arrows) are engulfed by MAs, several hepatocytes are infected with Goussia sp. (curved arrows), acute swelling of hepatocytes is visible, scale bar = 50 μm; (c) single macrophage (curved arrows) shows more intense reactivity to anti-tumour necrosis factor (TNF-α) in comparison to MAs (arrows), hepatocytes infected with Goussia sp. show acute swelling, scale bar = 50 μm.
The skeletal muscle of many fish was infected with A. simplex (s.s.), mostly in the hypaxial part of the flesh, but no host cellular reaction was encountered around the encapsulated larvae. Few blue whiting harboured nematode larvae on visceral peritoneum of the gonads, nevertheless, no immune cells encircled A. simplex (s.s.) larvae. In several blue whiting, A. simplex (s.s.) larvae were encapsulated on the external surface of the pyloric caeca, observations of the histological sections did not reveal appreciable host cellular reactions (fig. 3d).
Histochemistry and immunohistochemistry
In the liver of blue whiting, macrophages and MAs were immunoreactive to the antibody anti-mast cell tryptase, mainly in peripheral zone of the organ and near the nematode encapsulated larva (fig. 4a). Indeed, positive MAs engulfed coccidian Goussia sp. (fig. 4b). MAs also showed a weak reactivity to the antibody anti-TNF-α (fig. 4c). A more intense reactivity to anti-TNF-α was observed in single macrophage scattered in the liver parenchyma (fig. 4c). No results were obtained with the antibodies anti-histamine, -mast cell chymase and -serotonin. In sections of infected gonads, muscle and pyloric caeca no immunoreactivity to antibody anti-mast cell tryptase and anti-TNF-α was recorded.
Transmission electron microscopy
Ultrastructurally, in the infected liver, especially hepatocytes adjacent to each nematode larva had amorphous cytoplasmic vacuolation or rarefaction, liver cell with high level of lipid tended to possess numerous osmiophilic droplets (fig. 5a). Hepatocytes nuclei were enlarged and rich in euchromatin, with a thin rim of heterochromatin in the periphery of the nucleus attached to the nuclear membrane (fig. 5b). Each A. simplex (s.s.) larva was surrounded by a granuloma in which two distinct layers were recognized. An inner electron-dense epithelioid cells layer (fig. 5c) was formed of flattened cells with slight vacuolated cytoplasm. Ultrastructurally, the epithelioid cells were similar to macrophages and appeared to be compressed and cytoplasmic borders were indistinct (fig. 5c, d). The outer layer was less electron dense than the inner one and formed by epithelioid cells and collagen bundles scattered among them. In the outer layer, the epithelioid cells had elongated nuclei (fig. 5c) and presented numerous desmosomes (fig. 5d).
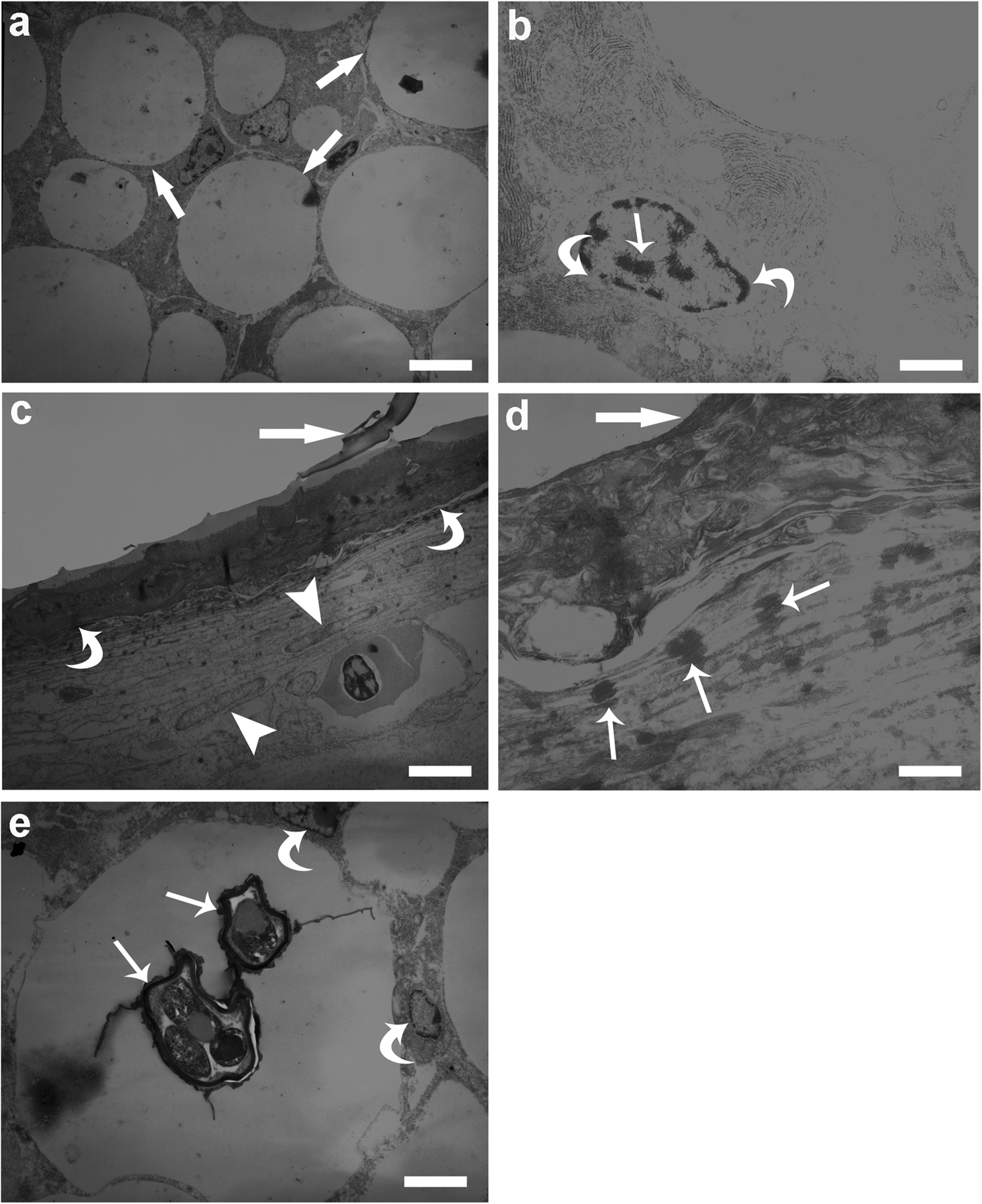
Fig. 5. Transmission electron microscopy of infected liver of Micromesistius poutassou. (a) Micrograph shows lipid droplets (arrows) within the cytoplasm of hepatocytes, bad condition of tissue, namely swollen of hepatocytes and their cytoplasmic vacuolation are notable, scale bar = 3.3 μm; (b) high magnification of hepatocyte nucleus rich in euchromatin (arrow), thin rim of heterochromatin (curved arrows) is also evident, scale bar = 0.8 μm; (c) interface region between A. simplex (s. s.) larva (arrow) and hepatic tissue, granuloma around each nematode larva is formed by two distinct layers, an inner layer of electron-dense epithelioid cells (curved arrows) and an outer layer formed by epithelioid cells with elongated nuclei (arrow heads), scale bar = 2.5 μm; (d) higher magnification of interface region, thick arrow shows A. simplex (s. s.) larva, occurrence of several desmosomes (arrows) between epithelioid cells are evident, scale bar = 0.4 μm; (e) two Goussia sp. (arrows) inside the cytoplasm of a hepatocyte, lipid droplet within the cytoplasm is visible, occurrence of coccidians induced displacement of the hepatocyte nuclei (curved arrows) to a marginal position, scale bar = 2.5 μm.
As mentioned earlier, the prevalence of Goussia sp. in the liver was 100%. Additionally, the hepatocyte cytoplasm frequently hosted more than one coccidian, consequently displacing the nucleus into a marginal position and narrowing the cytoplasm (fig. 5e).
Scanning electron microscopy
SEM on pieces of liver with encapsulated A. simplex (s.s.) larvae revealed that the larvae were often covered with amorphous material (fig. 6a). SEM of histological sections of infected livers showed also that co-infections with A. simplex (s.s.) and the coccidian Goussia sp. commonly occurred (fig. 6b, c). All livers of blue whiting were infected with Goussia sp., and, frequently, more than one Goussia sp. oocyte infected single hepatocytes (fig. 6c).

Fig. 6. Scanning electron microscopy (SEM) of infected liver of Micromesistius poutassou. (a) Anisakis simplex (s.s.) larva (arrow) encapsulated on liver surface and covered with mucus-like material, scale bar = 400 μm; (b) SEM of liver histological section, typical appearance of preventricular portion of the oesophagus of A. simplex (s.s.) larva (arrow) encapsulated in hepatic tissue is evident, scale bar = 100 μm; (c) high magnification reveals co-occurrence of A. simplex (s.s.) larva (arrow) and Goussia sp. (curved arrows) inside the hepatocytes, note acute swelling and degeneration of liver cells, scale bar = 20 μm.
Discussion
Anisakis simplex (s.s.) seems to be able to utilize several pelagic and demersal teleost fish species as vectors to the definitive cetacean host level. In this respect, blue whiting appears to be one of the most important transport hosts in NE Atlantic oceanic waters (Levsen et al., Reference Levsen, Svanevik, Cipriani, Mattiucci, Gay, Hastie and Pierce2018). On the other hand, the fish species represents a suitable host in the life cycle of the species of the A. simplex (s.s.), being found hosting also the sibling species A. pegreffii in the Mediterranean Sea waters (Levsen et al., Reference Levsen, Svanevik, Cipriani, Mattiucci, Gay, Hastie and Pierce2018; Mattiucci et al., Reference Mattiucci, Cipriani, Levsen, Paoletti and Nascetti2018). Fishes acquire larval stages of the Anisakis spp. by ingesting infected planktonic or semi-planktonic crustacean or prey fish. Once in the new fish host, the larva penetrates the intestinal wall of fish and enters the visceral cavity, crawling over organs, encysting on their surface or migrating into the host muscle (Mattiucci et al., Reference Mattiucci, Cipriani, Levsen, Paoletti and Nascetti2018). Migration of larvae in fish musculature can occur when fish is still alive, intra vitam (Larsen et al., Reference Larsen, Bresciani and Buchmann2002), or after host death, post mortem (Hauck, Reference Hauck1977; Cipriani et al., Reference Cipriani, Acerra, Bellisario, Sbaraglia, Cheleschi, Nascetti and Mattiucci2016). The success of helminth infection largely depends on their capacity to manipulate and or evade the general efficiency of hosts immune system (Franke et al., Reference Franke, Rahn, Dittmar, Erin, Rieger, Haase and Scharsack2014).
According to the mtDNA cox2 and EF1 α-1 nDNA sequences analyses, all the Anisakis spp. larvae (n = 100) were identified as Anisakis simplex (s.s.). This finding is congruent with previous recordings on M. poutassou from that fishing ground of the NE Atlantic Ocean (Levsen et al., Reference Levsen, Svanevik, Cipriani, Mattiucci, Gay, Hastie and Pierce2018), and also with the geographic distribution of this parasite species (Mattiucci et al., Reference Mattiucci, Cipriani, Levsen, Paoletti and Nascetti2018). The reference sample of 150 specimens of M. poutassou inspected for detection of anisakid nematodes showed high infection levels, with 149 fish out of 150 infected by A. simplex (s.s.), and a mean intensity Im = 39. Similar infection levels were already reported, from the same area, in previous studies (Karasev, Reference Karasev and Monstad1990; Fernández et al., Reference Fernández, Aznar, Montero and Raga2005; Berland, Reference Berland2006; Levsen et al., Reference Levsen, Svanevik, Cipriani, Mattiucci, Gay, Hastie and Pierce2018), demonstrating that this gadoid species seems to tolerate well the high intensity of A. simplex (s.s.) larvae.
It is well known that extraintestinal helminths frequently become encapsulated, as an evolved strategy to reduce parasite immune reactivity (Dezfuli et al., Reference Dezfuli, Manera, DePasquale, Pironi and Giari2017) and, thus, the extent of collateral damage to the infected host tissue. The granuloma is a chronic inflammatory focal response, most often organized in concentric cellular layers in/on the host tissue (Ferguson, Reference Ferguson2006). Encapsulation is considered a mutual adaptation between parasite and the host's immune response, representing a trade-off to ensure viability of both parasite and host (Bruschi & Chiumiento, Reference Bruschi and Chiumiento2011; Dezfuli et al., Reference Dezfuli, Manera, DePasquale, Pironi and Giari2017). In infected gonads, pyloric caeca and fillets of blue whiting, no appreciable host cellular response was noticed around A. simplex (s.s.) larvae. In a similar study, no cellular host reaction was seen in the muscles of Paralichthys lethostigma Jordan & Gilbert, 1884, parasitized by two species of philometrid nematodes (de Buron & Roumillat, Reference de Buron and Roumillat2010). Conversely, in skeletal muscle of Perca fluviatilis Linnaeus, 1758 infected with larvae of the nematode Eustrongylides sp. Jägerskiöld, 1909, numerous MAs and MCs were seen throughout the thick fibro-connectival layer of the capsule enclosing the larvae (Dezfuli et al., Reference Dezfuli, Manera, Lorenzoni, Pironi, Shinn and Giari2015).
The reasons for the lack or presence of immune cells in granulomas in visceral organs and skeletal muscle of fish is unknown and open to conjecture. It has been also hypothesized that this concomitant immunity may represent a trade-off between greater pathology that may be caused by the presence of dead larvae in host organs compared to encapsulation and isolation of live anisakids (reviewed by Buchmann, Reference Buchmann2012). Immune responses in fish against parasites depend on the eliciting agents and host species (Gauthier et al., Reference Gauthier, Vogelbein and Ottinger2004) since different parasites may differentially affect the various parts of the host physiology and immunological pathways (Ferguson, Reference Ferguson2006).
In M. poutassou, the liver was the most heavily infected organ, showing 100% prevalence of Goussia sp. and 99.3% of A. simplex (s.s.) larvae. In liver, recruitment of epithelioid cells, macrophages and macrophages aggregates were noticed. One of the well-conserved innate defence mechanisms is phagocytosis (Rieger et al., Reference Rieger, Konowalchuk, Grayfer, Katzenback, Havixbeck, Kiemele and Barreda2012; Grayfer et al., Reference Grayfer, Hodgkinson and Belosevic2014). In fish, generally, two populations of professional phagocytes were described, mononuclear phagocytes (circulating monocytes and tissue macrophages) (Secombes & Ellis, Reference Secombes, Ellis and Roberts2012; Esteban et al., Reference Esteban, Cuesta, Chaves-Pozo and Meseguer2015) and neutrophils (Secombes & Ellis, Reference Secombes, Ellis and Roberts2012). In infected liver of M. poutassou very few neutrophils were noticed. With regard to macrophages, in most fish species they exist in clusters (MAs) in the posterior kidney, spleen and less frequently in intestine and liver (Agius & Roberts, Reference Agius and Roberts2003) and contain pigments like hemosiderin, chromolipoids and melanin (Agius & Roberts, Reference Agius and Roberts2003; Secombes & Ellis, Reference Secombes, Ellis and Roberts2012; Dezfuli et al., Reference Dezfuli, Manera, DePasquale, Pironi and Giari2017).
The size and distribution of MAs might be an indicator of the general health status in fish (Latoszek et al., Reference Latoszek, Kamaszewski, Milczarek, Puppel, Szudrowicz, Adamski and Ostaszewska2019), and a biomarker of their immune capacity and activation (Stosik et al., Reference Stosik, Tokarz-Deptuła and Deptuła2019). Indeed, in fish liver, MAs may develop in association with chronic inflammatory lesions (Agius & Roberts, Reference Agius and Roberts2003; Estensoro et al., Reference Estensoro, Mulero, Redondo, Alvarez-Pellitero, Mulero and Sitjà-Bobdella2014; Dezfuli et al., Reference Dezfuli, Manera, DePasquale, Pironi and Giari2017). In the liver of blue whiting, single macrophages as well as numerous MAs were encountered in close proximity to A. simplex (s.s.) larvae and Goussia sp. oocysts. Data on involvement of macrophages and MAs against nematode infections appeared in (Dezfuli et al., Reference Dezfuli, Fernandes, Galindo, Castaldelli, Manera, DePasquale and Giari2016; Ventura et al., Reference Ventura, Ishikawa, de Araujo Gabriel, Silbiger, Cavichiolo and Takemoto2016) and relationship between MAs and protozoans was reviewed by Sitjà-Bobadilla et al. (Reference Sitjà-Bobadilla, Estensoro and Pérez-Sánchez2016). Indeed, those authors provided an image of carp macrophages which ingested merozoites of Goussia carpelli. It was reported that an increase in number and size of MAs in the spleen is the onset of the inflammatory response (Agius & Roberts, Reference Agius and Roberts2003) and in some instances it is also due to occurrence of engulfed parasites in MAs (Estensoro et al., Reference Estensoro, Mulero, Redondo, Alvarez-Pellitero, Mulero and Sitjà-Bobdella2014; Sitjà-Bobadilla et al., Reference Sitjà-Bobadilla, Estensoro and Pérez-Sánchez2016). Livers of all 150 blue whiting harboured high numbers of coccidians, and MAs engulfed variable numbers of Goussia sp. Thus, our assumption is most likely there could be a direct relationship between number of coccidian and that of MAs of blue whiting. Detoxification, recycling of exogenous and endogenous materials, and focal destruction have been attributed to MAs (Agius & Roberts, Reference Agius and Roberts2003). Frequently, numerous single macrophages, and MAs, were noticed in close proximity to the A. simplex (s.s.) larvae, but we failed to find where MAs had engulfed portions of the nematode, presumably because of large size of parasite larva.
In the infected liver of blue whiting, the layers of the capsule surrounding each larva were made by epithelioid cells. Epithelioid cells show morphological similarity to epithelial cells, and it is generally accepted that they are transformed macrophages that form upon persistent inflammatory stimulation (Gauthier et al., Reference Gauthier, Vogelbein and Ottinger2004). The origin of epithelioid cells from macrophages was documented from in vitro investigation (Secombes, Reference Secombes, Iwama and Nakanishi1996). It is interesting to note that the liver of fish is able to produce granuloma/capsule formed by metabolically active cells like macrophages and less active cells like epithelioid cells. Similar granuloma/capsules were encountered in the liver of another fish species naturally infected with the nematode Brevimulticaecum sp. Shikhobalova and Mozgovoi, 1952 (Dezfuli et al., Reference Dezfuli, Fernandes, Galindo, Castaldelli, Manera, DePasquale and Giari2016).
With regard to Anisakis spp. larvae in fish, it was mentioned that host cellular reactions are likely one of the stimuli inducing larval coiling and production of a defence wall made by an inner layer of dead host cells, and outer layer of leucocytes (Larsen et al., Reference Larsen, Bresciani and Buchmann2002). Even at the high prevalence and intensity of infection of A. simplex (s.s.) in blue whiting liver, it seems unlikely that the parasite is able to induce severe damage in the fish, with a consequent host population decline. Most probably a balance between nematode damage and fish defence response occurs in liver thanks to potential liver compensatory properties which is well known (Jia et al., Reference Jia, Qin, Yuan, Liao, Huang, Wang, Sun and Li2019). In terms of fish host - Anisakis interaction, recent studies have shown that a significant up-regulation of the gene coding for a Kunitz-type trypsin inhibitor protein occurs in A. simplex (s.s.) larvae infecting the liver of blue whiting (Palomba et al., Reference Palomba, Cipriani, Giulietti, Levsen, Nascetti and Mattiucci2020). The Kunitz-type trypsin inhibitor protein of Anisakis seems to have a role in the inhibition of the host's tryptases, as previously observed in other nematode species (Bernard & Peanasky, Reference Bernard and Peanasky1993). It could be thus hypothesized that an invading larva of A. simplex (s.s) increases transcripts of an inhibitor protein against the fish tryptases, abundant in the liver tissue, as a possible result of the parasite's mechanism of evasion of the host immune response (Palomba et al., Reference Palomba, Cipriani, Giulietti, Levsen, Nascetti and Mattiucci2020).
In fish, TNF-α is a cytokine involved in modulation of different immune cells in response to the presence of pathogens and tissue inflammation (Seno et al., Reference Seno, Sawada, Fukuzawa, Morita-Fujisawa, Takaishi, Hiai and Chiba2002; Hong et al., Reference Hong, Li, Xu, Secombes and Wang2013; Williams et al., Reference Williams, Duckworth, Burkitt, Watson, Campbell and Pritchard2015). TNF-α was mainly produced by monocytes and macrophages (Hehlgans & Pfeffer, Reference Hehlgans and Pfeffer2005), and was observed in cells of several tissues and organs of the turbot (Ronza et al., Reference Ronza, Losada, Villamarín, Bermúdez and Quiroga2015).
The presence of immunoreactive macrophages to the antibody anti-TNF-α in the liver of the blue whiting is clearly associated with the hepatic degeneration due to the A. simplex (s.s.) larvae and Goussia sp. In the liver of rats, an increase of TNF-α level was observed after the degeneration induced by a traumatic event (Gao et al., Reference Gao, Xie, Gu, Li, Zhang and Yu2014).
Tryptase is a serine protease produced and stored in mast cell granules (McNeil et al., Reference McNeil, Adachi and Stevens2007). Tryptase has been reported in gill mast cells of zebrafish (Dobson et al., Reference Dobson, Seibert, The, Da'as, Fraser, Paw and Berman2008), and in mucosal mast cells of the intestine of the fat snook, Centropomus parallelus Poey, 1860 (da Silva et al., Reference da Silva, Simões, Gutierre, Egami, Santos, Antoniazzi and Ranzani-Paiva2017), suggesting that the occurrence of tryptase is supportive in host tissue repair processes (Duchesne et al., Reference Duchesne, Tremblay and Côté2011). In the present parasitized liver samples of blue whiting, numerous macrophages and MAs were noticed, and they were positive to the antibody anti-mast cell tryptase. The presence of tryptase may suggest that liver tissue repairing processes were activated and employed as a response to the parasite infections.
In conclusion, the liver of blue whiting was the most infected organ in the present study. However, A. simplex (s.s.) larvae were efficiently sequestered inside the granulomas, since the surrounding parenchyma did not show any signs of fibrosis. Most likely, this focal encapsulation of the nematode permits uninfected portions of the liver to maintain their functions and provide adequate fish health condition and, thus, proper recruitment of the NE Atlantic blue whiting stock. Above assumption is based also on overall acceptable health condition of fish during inspection, indeed, it seems that Goussia sp. has a low virulence on fish liver with 100% prevalence in this organ and several hepatocytes with coccidians inside.
Acknowledgements
We would like to G. Servadei, C. Maestri for technical helps in laboratory and P. Boldrini from Centre of Electron Microscopy of University of Ferrara for technical assistance.
Financial support
This study was supported by grants from the University of Ferrara to B.S.D (FAR, year 2019). S. Mattiucci has contributed to the study with a grant from ‘Istituto Pasteur Italia-Fondazione Cenci Bolognetti’.
Conflict of interest
None.
Ethical standards
The authors assert that all procedures contributing to this study comply with the ethical standards of the relevant national and institutional guides.