Introduction
Among the parasites that constrain the survival and productivity of sheep and goats, the gastrointestinal nematodes rank highest, with Haemonchus contortus being of paramount importance (Perry et al., Reference Perry2002). Haemonchus contortus causes notable morbidity and mortality as well as production losses in the animals due to their blood-feeding behaviour. Both adult and fourth-stage larvae are haematophagous and thus cause severe anaemia, losses in weight, milk, meat and wool production, and often death, particularly in young animals (Rashid and Irshadullah, Reference Rashid and Irshadullah2014). It has been estimated that each worm is responsible for about 0.05 ml of blood loss per day, either by ingestion or seepage from lesions (Urquhart et al., Reference Urquhart2000).
Haemonchus contortus is one of the most important parasites of sheep and goats and is quite prevalent throughout the world (Burke et al., Reference Burke2016; Rashid and Irshadullah, Reference Rashid and Irshadullah2018). During the establishment of parasites in their microhabitat, chains of dynamic interactions occur at the host–parasite interface. The host reacts against parasites in a number of ways, including the production of reactive oxygen species (ROS) that are generated by the host during normal aerobic metabolism, as well as by activated leukocytes via oxidative burst (Callahan et al., Reference Callahan, Crouch and James1988; Chiumiento and Bruschi, Reference Chiumiento and Bruschi2009). The production of ROS by immune effector cells considerably increases due to parasitic infections and is thought to play a role in killing or expulsion of parasites from their host, thereby preventing the establishment of infection (Callahan et al., Reference Callahan, Crouch and James1988; Smith and Bryant, Reference Smith and Bryant1989; Batra et al., Reference Batra1993; Ben-Smith et al., Reference Ben-Smith, Lammas and Behnke2002). Oxidative stress develops due to an imbalance between the generation and removal of ROS within the organism, which causes oxidative damage to all major classes of biomolecules, leading to protein oxidation, lipid peroxidation, DNA modification/strand breakage and depolymerization of polysaccharides (Southern and Powis, Reference Southern and Powis1988; Chiumiento and Bruschi, Reference Chiumiento and Bruschi2009). Biological effects of ROS on these intracellular targets depend upon the concentration of ROS (Weydert and Cullen, Reference Weydert and Cullen2010) and the damage may be due to depletion of antioxidant defence, an increase in ROS production, or both (Halliwell and Gutteridge, Reference Halliwell and Gutteridge2007).
Oxidative damage has been attributed to many helminth parasites: Fasciola hepatica (Kolodziejczyk et al., Reference Kolodziejczyk, Siemieniuk and Skrzydlewska2005; Siemieniuk et al., Reference Siemieniuk, Kolodziejczyk and Skrzydlewska2008), Dicrocoelium dendriticum (Deger et al., Reference Deger2008), Trichinella spiralis (Othman et al., Reference Othman2016); Taenia saginata (Łuszczak et al., Reference Łuszczak, Ziaja-Sołtys and Rzymowska2011), Echinococcus granulosus (Heidarpour et al., Reference Heidarpour2012, Reference Heidarpour2013a, Reference Heidarpourb) and Schistosoma mansoni (de Oliveira et al., Reference De Oliveira2013). To our knowledge, there is no report on the status of oxidative stress and antioxidant defence in goats infected with H. contortus. Most of the above-mentioned studies have been carried out on experimental animals, but it is debatable whether experimental model animals can be used for pathological studies, given the difference in responses of the host. Based upon the pathological responses in sheep and rabbits infected with F. hepatica, Le Bars and Banting (Reference Le Bars, Banting and EJL1976) suggested that rabbits cannot be used as a model for the study of pathological effects. Thus in the present study we investigated various biochemical and oxidative stress parameters in goats naturally infected with H. contortus. This study may provide information on the oxidative response of infected animals and the pathogenesis of the disease.
Materials and methods
Collection and preparation of samples
Gastrointestinal tracts (GI) were collected from goats (Capra aegagrus hircus) slaughtered at the local abattoir (Aligarh, India), labelled individually, and brought to the laboratory. The GI tracts were opened and H. contortus were collected from each sample. The worms were counted manually. Based upon the number of worms present in the individual host, the host's infection status was categorized as low (< 100 worms), mild (100–500 worms) or heavily infected (> 500 worms).
Small pieces of infected and non-infected abomasa were washed several times with Hanks’ balanced salt solution (HBSS), premaintained at 37 ± 2°C to remove debris, blotted on filter paper and homogenized separately in a Potter Elvehjem homogenizer, in 0.1m ice-cold phosphate buffer (pH 7.4). The homogenates were sonicated (Ultrasonic processor, 5 mm probe) in an ice bath for 3 × 1 minute at 30 s intervals and then centrifuged at 9000 g for 15 minutes at 4°C. After centrifugation, supernatants were collected and stored at −20°C in aliquots and were used for the estimation of antioxidant and oxidant parameters and other biochemical variables. In the present study, only tissues from those hosts that had only H. contortus infection were analysed. The samples of those animals that had concurrent infections of other helminths were discarded. A total of nine infected goats were used, with three in each category of infection.
Biochemical analysis
Analysis of antioxidant enzymes
The level of antioxidant enzymes, i.e. catalase (CAT), glutathione S-transferase (GST), glutathione reductase (GR) and glutathione peroxidase (GPx), was estimated by standard spectrophotometric methods as described by Aebi (Reference Aebi and Bergmeyer1974), Habig et al. (Reference Habig, Pabst and Jakoby1974), Carlberg and Mannervik (Reference Carlberg and Mannervik1985) and Paglia and Valentine (Reference Paglia and Valentine1967), respectively.
Analysis of marker enzymes
Aspartate (AST) and alanine (ALT) aminotransferases were analysed using a Span diagnostic enzyme kit (Surat, India). The assay principle of these enzymes is based on the method of Reitman and Frankel (Reference Reitman and Frankel1957). The activity of acid (ACP) and alkaline (ALP) phosphatases was determined by the method of Bergmeyer et al. (Reference Bergmeyer, Gawehn, Grassl and Bergmeyer1974).
Analysis of oxidant parameters
The concentrations of superoxide anion (O2−), protein carbonyl (PC), malondialdehyde (MDA) and reduced glutathione (GSH) were estimated by the methods of Green and Hill (Reference Green and Hill1984), Levine et al. (Reference Levine1990), Ohkawa et al. (Reference Ohkawa, Ohishi and Yagi1979) and Jollow et al. (Reference Jollow1974), respectively. Protein concentration in the samples was determined by the dye-binding method of Spector (Reference Spector1978).
Statistical analysis
Statistical analysis was performed using one-way ANOVA followed by post-hoc Tukey's HSD multiple comparison tests using the statistical software R v. 2.15.1(R Development Core Team, 2012). The confidence level was held at 95% and P ≤ 0.05 was considered significant.
Results
Antioxidant enzymes
The activity of CAT, GST and GR was found to increase whereas GPx activity declined in the infected tissues as compared to non-infected tissues (fig. 1). A significant increase in the activities of CAT and GST was recorded in mild and heavily infected animals, whereas there were no differences in the values of these enzymes in animals with low or no infection. The activity of GR and GPx was significant in all the groups compared with the non-infected animals.
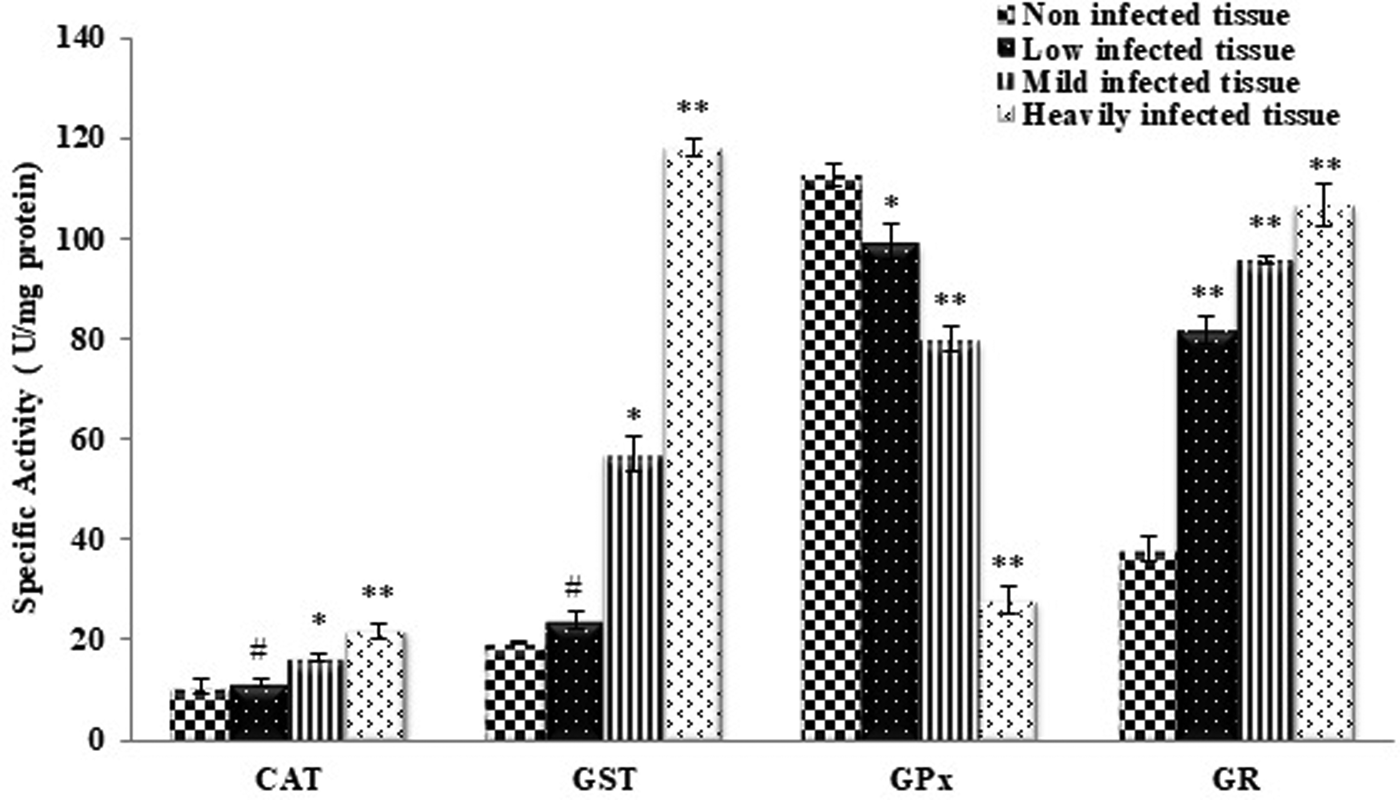
Fig. 1. Activities of antioxidant enzymes in non-infected host tissue and host tissue infected with Haemonchus contortus. CAT, catalase; GST, glutathione S-transferase; GR, glutathione reductase; GPx, glutathione peroxidase. All values are the mean ± S.E. of three replicates. Statistical analysis was performed by one-way ANOVA followed by the post-hoc Tukey's test; #, non-significant; *, significant at P ≤ 0.05; **, significant at P ≤ 0.001.
Marker enzymes
The levels of AST, ALT and ALP were found to be significantly higher in the low, mild and heavily infected tissues compared to the controls (fig. 2). The level of ACP was also found to be significantly higher in the mild and heavily infected groups compared to the control, but the differences were insignificant in cases of low infection.
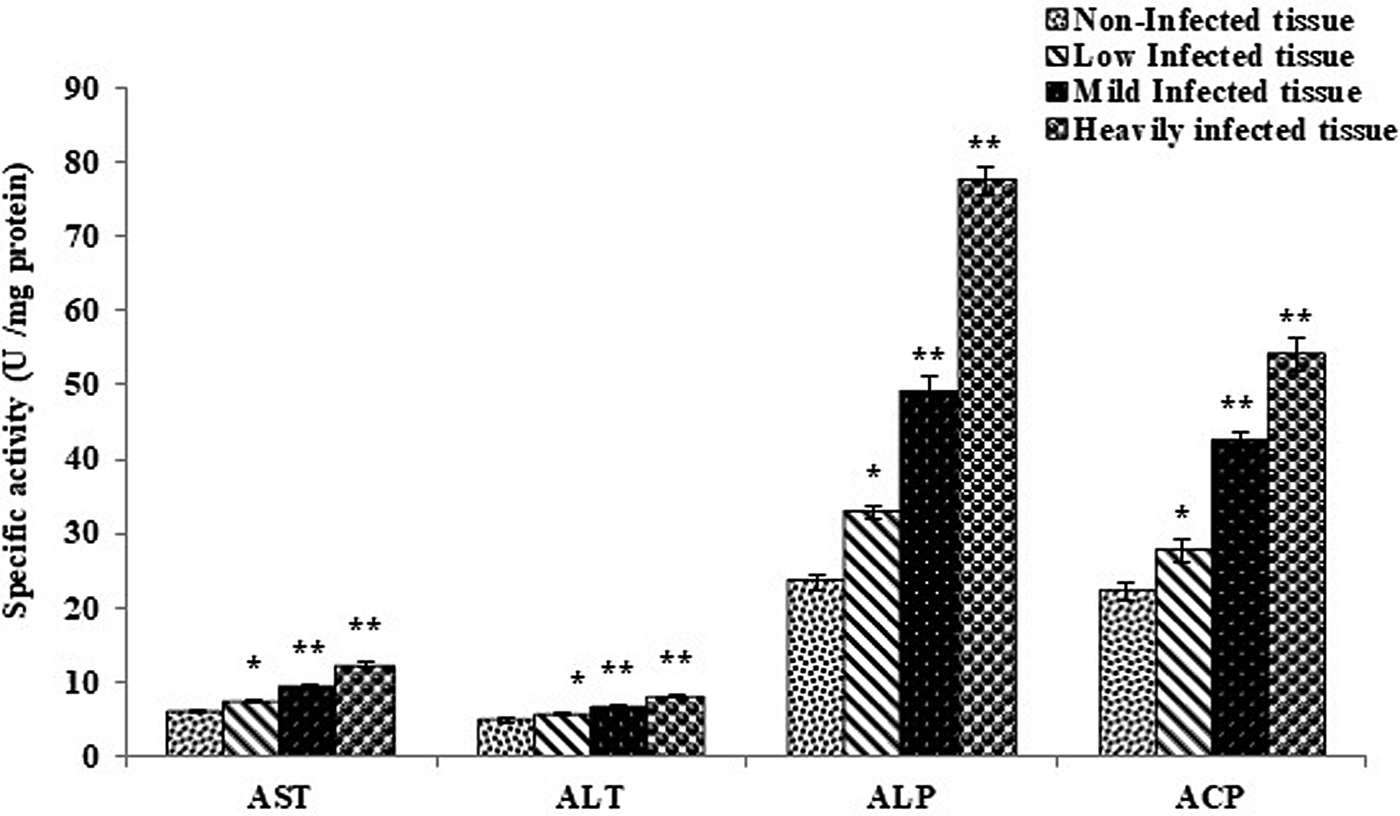
Fig. 2. Marker enzymes of non-infected host tissue and host tissue infected with Haemonchus contortus. AST, aspartate aminotransferase; ALT, alanine aminotransferase; ALP, alkaline phosphatase; ACP, acid phosphatase. All values are the mean ± S.E. of three replicates. Statistical analysis was performed by one-way ANOVA followed by the post-hoc Tukey's test; #, non-significant; *, significant at P ≤ 0.05; **, significant at P ≤ 0.001.
Oxidant parameters in the tissue
The results for oxidative stress markers such as superoxide anion (O2−) concentration, lipid peroxidation, protein oxidation and glutathione level are shown in fig. 3. A rise in the level of O2− was observed in the infected tissue, which was directly related to the number of parasites in the individual infected host (fig. 3a). The increase in O2− was significant in cases of mild (P < 0.05) and heavy infection (P < 0.001), but insignificant in cases of low infection. A significant rise in MDA level, an index of lipid peroxidation, was seen in all the groups (fig. 3b). An increase in the protein carbonyl level, a parameter of oxidative damage to proteins, was also found to be elevated in the infected tissue (fig. 3c). Reduced glutathione levels were also altered; a drastic decline was seen in all the groups analysed when compared with the non-infected tissue (fig. 3d).
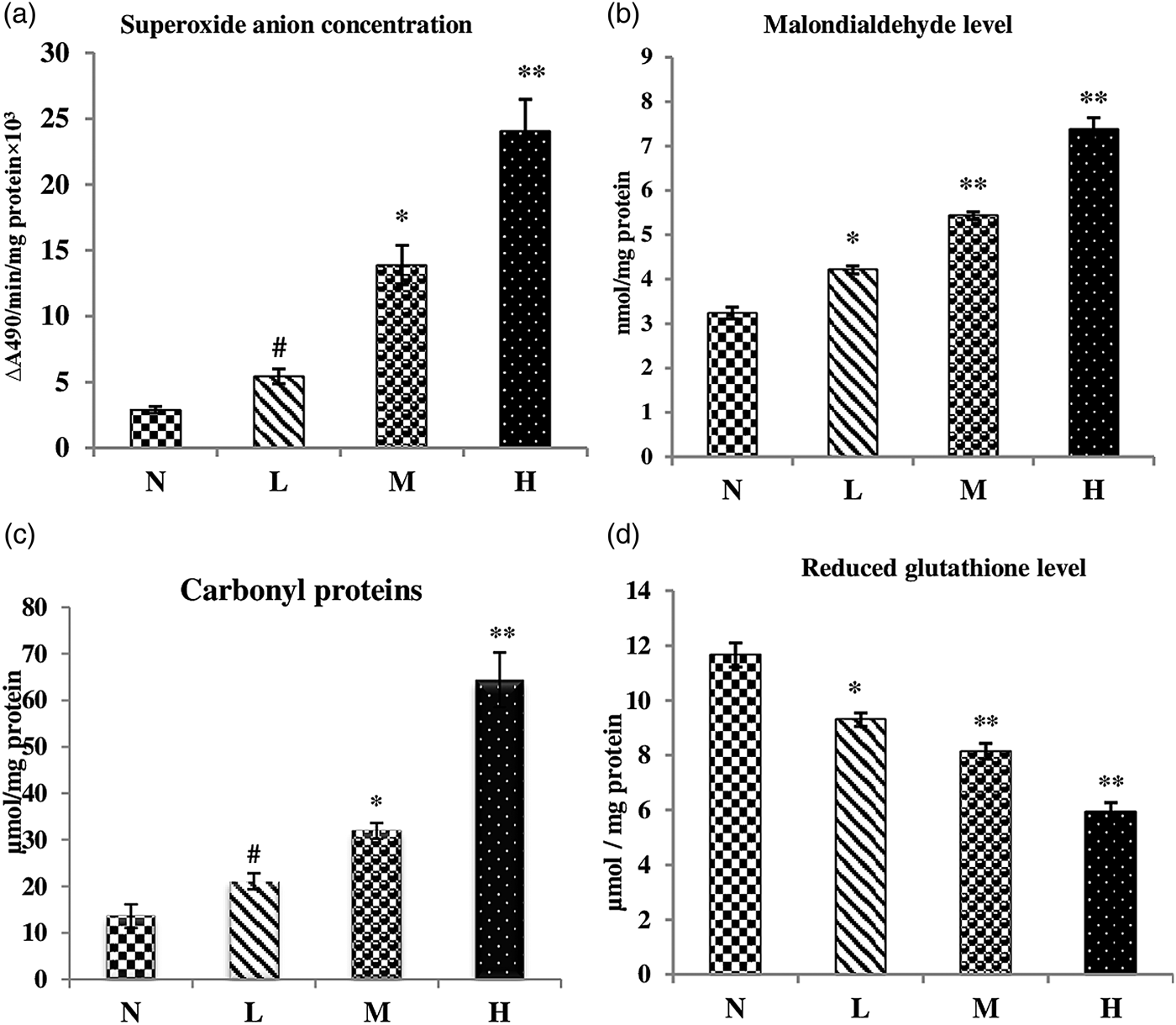
Fig. 3. Alteration in the level of the oxidant parameters in non-infected host tissue and host tissue infected with Haemonchus contortus. N, non-infected host tissue; L, low infection of host tissue; M, mildly infected host tissue; H, heavily infected host tissue. All values are the mean ± S.E. of three replicates. Statistical analysis was performed by one-way ANOVA followed by the post-hoc Tukey's test; #, non-significant; *, significant at P ≤ 0.05; **, significant at P ≤ 0.001.
Discussion
Parasitic infection is responsible for a variety of biochemical changes, depending upon the species of the parasites, the invasion sites and the worm burden (Esmaeilnejad et al., Reference Esmaeilnejad2012; de Oliveira et al., Reference De Oliveira2013; Ortolani et al., Reference Ortolani2013). The present study of the alteration in antioxidant enzymes and oxidative stress of goats infected with H. contortus provides reliable biochemical evidence for the generation of circulating oxidative stress. Increased free radical generation (O2−), enhanced lipid peroxidation and increased oxidation products of proteins, coupled with changes in the activity of the enzymatic (CAT, GST, GR and GPx) and non-enzymatic (GSH) antioxidant defences, were detected in naturally infected goats. However, the present study cannot be compared with previous reports, as no published report is available for goats infected with H. contortus.
Cells contain a variety of antioxidant mechanisms that play a central role in protection against ROS (Halliwell, Reference Halliwell1991). The increase in the activity of CAT, GR and GST in infected animals may be explained by the fact that oxidants may activate gene expression through antioxidant responsive elements (Rushmore et al., Reference Rushmore, Morton and Pickett1991). A significant increase in catalase activity was found in the animals infected with H. contortus, as also reported in hamsters infected with D. dendriticum (Sanchez-Campos et al., Reference Sanchez-Campos1999), in rat liver infected with F. hepatica (Kolodziejczyk et al., Reference Kolodziejczyk, Siemieniuk and Skrzydlewska2005) and in mice liver infected with S. mansoni (de Oliveira et al., Reference De Oliveira2013). The up-regulation in CAT activity is expected due to excessive peroxide production by leukocytes, as also suggested by de Oliveira et al. (Reference De Oliveira2013). We observed significantly increased levels of GR activity in H. contortus infected tissues, whereas Kolodziejczyk et al. (Reference Kolodziejczyk, Siemieniuk and Skrzydlewska2005) reported a significantly reduced level of GR activity in liver infected with F. hepatica. An increased level of GST in the infected animal may be due to toxic secretions by the parasites and the host's inflammatory response, as this enzyme is responsible for the neutralization of toxins (Brophy and Pritchard, Reference Brophy and Pritchard1994). Similarly, an increase in GST activity has been reported in hamsters infected with D. dendriticum (Sanchez-Campos et al., Reference Sanchez-Campos1999), in mice infected with Ancylostoma caninum (Gollapudi and Vardhani, Reference Gollapudi and Vardhani2013) and in the muscles of mice infected with T. spiralis (Derda et al., Reference Derda, Wandurska-Nowak and Hadas2004). GPx activity was found to be lower in the infected animals compared to the non-infected group, which is similar to the findings of Heidarpour et al. (Reference Heidarpour2012) and Kolodziejczyk et al. (Reference Kolodziejczyk, Siemieniuk and Skrzydlewska2005) for camels infected with E. granulosus and rats infected with F. hepatica, respectively. In contrast, an increase in the activity of GPx was reported in cattle infected with E. granulosus (Heidarpour et al., Reference Heidarpour2013a), in hamsters infected with D. dendriticum (Sanchez-Campos et al., Reference Sanchez-Campos1999), in sheep infected with F. hepatica (Benzer and Temizer–Ozan, Reference Benzer and Temizer-Ozan2003), in skeletal muscle of cattle infected with T. saginata (Łuszczak et al., Reference Łuszczak, Ziaja-Sołtys and Rzymowska2011) and in the liver of sheep infected with D. dendriticum and F. hepatica (Deger et al., Reference Deger2008). The superoxide dismutase (SOD) activity has also been found to be significantly reduced in abomasal tissue infected with H. contortus (Rashid and Irshadullah, Reference Rashid and Irshadullah2014).
Reactive oxygen species (ROS) are produced by phagocytes with the aim of destroying the parasite; however, the host's innate defence mechanism is not specific, so the destruction of its own tissues is possible. This reaction may lead to pathological processes. The level of O2− was found to be higher in infected animals and is related to the worm burden. Similarly, an increased level of O2− has been reported in animals infected with F. hepatica (Abo-Shousha et al., Reference Abo-Shousha, Khalil and Rashwan1999). Lipid peroxidation was significantly enhanced in the tissue infected with H. contortus. Lipid peroxidation results in the disarrangement and, ultimately, disruption of cell membranes, which leads to cell death. During helminth infections, lipid peroxidation has been found to increase (Kolodziejczyk et al., Reference Kolodziejczyk, Siemieniuk and Skrzydlewska2005; Heidarpour et al., Reference Heidarpour2012, Reference Heidarpour2013b; de Oliveira et al., Reference De Oliveira2013). Protein carbonyls are not only good markers for oxidative damage but are also oxidizing agents, which enhance oxidative damage to a membrane protein, principally in the form of disulphide skeleton protein adducts, causing denaturation and instability of the cytoskeleton (McMillan et al., Reference McMillan2005; Dalle-Donne et al., Reference Dalle-Donne2006). The increased carbonyl protein in the infected tissue confirms enhanced release of free radicals in H. contortus-infected animals. Siemieniuk et al. (Reference Siemieniuk, Kolodziejczyk and Skrzydlewska2008) studied the oxidative modifications of rat liver cells due to F. hepatica infection and reported a significant increase in protein oxidation and lipid peroxidation. De Oliveira et al. (Reference De Oliveira2013) reported an elevated level of protein carbonyls in the tissue of mice infected with S. mansoni. The increase in carbonyl proteins in the present study is directly related to the worm burden, as also reported by Saleha et al. (Reference Saleha, Mahranb and Al-Salahy2011). The glutathione level was significantly reduced; it may be connected with the enhanced oxidation of GSH into glutathione disulphide catalysed by free radicals. As glutathione is the most important cellular antioxidant and plays a major role in protecting the cells against oxidative stress (Shan et al., Reference Shan, Aw and Jones1990), it has been postulated that loss of GSH may compromise cellular antioxidant defences and lead to the accumulation of reactive oxygen species (Halprin and Ohkawara, Reference Halprin and Ohkawara1967). Furthermore, it was shown that administering GSH to experimentally infected rats greatly reduced the damage to membrane lipids of the liver tissue (Maffei Facino et al., Reference Maffei Facino1993). Glutathione peroxidase and GSH represent a major pathway for metabolizing hydrogen peroxide and lipid peroxides. Therefore, depletion of GSH and suppression of glutathione peroxidase activity, as we have observed in the present study, may result in the accumulation of peroxides to toxic levels. Similarly, Kolodziejczyk et al. (Reference Kolodziejczyk, Siemieniuk and Skrzydlewska2006) reported a decline in the level of GSH and GPx activity in the serum of rats infected with F. hepatica.
The elevated level of marker enzymes (AST, ALT, ACP and ALP) in infected tissues indicated some damage or changes in membrane permeability. Similar results have been reported by Khan et al. (Reference Khan, Saifullah and Abidi2013) and Kolodziejczyk et al. (Reference Kolodziejczyk, Siemieniuk and Skrzydlewska2005) in fish liver infected with Clinostomum complanatum and rat liver infected with F. hepatica, respectively. Deger et al. (Reference Deger2008) also reported a higher level of ALT and AST in the liver of sheep naturally infected with D. dendriticum and F. hepatica as compared to the control.
Observations in the present study on biochemical changes caused by haemonchosis in goats carry great importance as they may indicate the extent of damage to the abomasal mucosa, and thereby help in better understanding the host–parasite interactions. During H. contortus infection, it was observed that there were significant alterations in oxidative markers, indicators of cell lesion of the abomasum, which was confirmed by an increase in the marker enzymes, and consequently, there were changes in levels of antioxidants with the purpose of cell protection. It can be concluded that H. contortus plays a critical role as an oxidative stressor in goats.
Acknowledgements
The authors are grateful to the chairman, Department of Zoology, Aligarh Muslim University, Aligarh for providing laboratory facilities. Special thanks to Professor Athar Ali Khan, Department of Statistics and Operations Research, for his guidance and assistance in statistical analysis.
Financial support
The financial assistance by University Grants Commission, New Delhi (F.40-3(M/S)/2009(SA-III/MANF) is gratefully acknowledged.
Conflict of interest
None.