Introduction
Lissorchiids are relatively small trematodes that inhabit the intestines of freshwater, less often brackish-water fishes, mainly cyprinids (Bray, Reference Bray, Jones, Gibson and Bray2008). According to Bray's (Reference Bray, Jones, Gibson and Bray2008) review, in Europe lissorchiids are represented by species of the genera Asymphylodora Looss, 1899 and Palaeorchis Szidat, Reference Szidat1943. The genus Asymphylodora is common in the Palaearctic region and is the second species-rich genus of fish trematodes after Phyllodistomum Braun, 1899, with the eight species recorded in the database of European fauna (Scholz et al., Reference Scholz, Besprozvannykh and Boutorina2016). However, intergeneric relations of species assigned to the genus Asymphylodora are not clarified enough. For the Asian species, Asymphylodora macrostoma Ozaki, 1925 and Asymphylodora indica Srivastava, 1936, Szidat (Reference Szidat1943) erected a new genus, Parasymphylodora. The validity of the genus Parasymphylodora Szidat, Reference Szidat1943 is still being questioned. Shimazu (Reference Shimazu1992) shares the view of Sobolev (Reference Sobolev and Skriabin1955) that the morphological features used by Szidat (Reference Szidat1943) to validate his new genus do not seem of generic importance and the genus Parasymphylodora should be suppressed as a synonym of the genus Asymphylodora. This point of view is also shared by Bray (Reference Bray, Jones, Gibson and Bray2008), who believes that validation of the Parasymphylodora requires stronger distinguishing features. However, some authors consider Parasymphylodora as a valid genus with three species, P. markewitschi (Kulakovskaja, 1947), Parasymphylodora parasquamosa Kulakova, Reference Kulakova1972 and Parasymphylodora progenetica (Serkova & Bychovsky, Reference Serkova and Bychovsky1940) representing European fauna (Bykhovskaya-Pavlovskaya & Kulakova, Reference Bykhovskaya-Pavlovskaya, Kulakova and Bauer1987; Niewiadomska, Reference Niewiadomska2003). The clarification of phylogenetic relationships of trematodes today undoubtedly depends on molecular markers. Unfortunately, a comprehensive molecular phylogenetic analysis of relationships between lissorchiids is lacking, as only a few species were available for previous studies (Besprozvannykh et al., Reference Besprozvannykh, Ermolenko and Atopkin2012; Sokolov & Gordeev, Reference Sokolov and Gordeev2019; Petkevičiūtė et al., Reference Petkevičiūtė, Stanevičiūtė and Stunžėnas2020).
Recently, molecular data have become essential for matching different stages of digenean life-cycles (Cribb, Reference Cribb2016; Faltýnková et al., Reference Faltýnková, Sures and Kostadinova2016). The cercariae of lissorchiid species develop within rediae in different gastropods (pulmonates and prosobranchs) and are of a non-oculate cercariaeum type, characterized by lack of tail (tailless), that usually encyst in gastropods. Analysis of the literature showed that a number of larval lissorchiid trematodes are associated with the faucet snail, Bithynia tentaculata (Linnaeus, 1758) in Europe, but most are known only under provisional names, as Cercaria paludinae impurae (Filippi, 1854), Cercariaeum bithyneae Khan, Reference Khan1962, Cercariaeum helveticum (Dubois, Reference Dubois1929) or Cercariaeum internale Khan, Reference Khan1962, and are not associated with any adult forms (Khan, Reference Khan1962; Morley et al., Reference Morley, Adam and Lewis2004; Cichy et al., Reference Cichy, Faltýnková and Żbikowska2011). In general, the information available on the life-cycles and specificity of European lissorchiids is very limited, based mainly on comparative morphological analysis and some experimental infections, which sometimes yielded contradictory results (Stunkard, Reference Stunkard1959; Van den Broek & Jong, Reference Van den Broek and de Jong1979; Našincová & Scholz, Reference Našincová and Scholz1994). Although molecular approaches provide a reliable elucidation of life-cycles, only one life-cycle of European lissorchiid, namely Palaeorchis incognitus Szidat, Reference Szidat1943, has been confirmed based on DNA sequence comparisons (Petkevičiūtė et al., Reference Petkevičiūtė, Stanevičiūtė and Stunžėnas2020).
Karyotypic data (number and morphology of chromosomes) is one of the fundamental characteristics of the species, which is useful for identifying the species of different organisms and solving phylogenetic and taxonomic problems. Trematodes, in general, are karyotypically conservative, with related species on generic and even family levels tending to have the same number and closely related chromosome morphology differing by a few chromosome rearrangements. The existing karyological information on lissorchiid trematodes, while quite scarce, is intriguing, as very different karyotypes were described, with diploid chromosome numbers varying from 2n = 14 to 2n = 22 (Baršienė, Reference Baršienė1993; Baršienė et al., Reference Baršienė, Roca, Tapia and Martin1995; Petkevičiūtė et al., Reference Petkevičiūtė, Stanevičiūtė and Stunžėnas2020).
In the present study, new samples of larval and adult Asymphylodora sensu lato (including those assigned to the genus Parasymphylodora) from freshwater ecosystems are used to examine phylogenetic affinities of these digeneans and to link their life-cycle stages. Here we report on the results of the comparative karyological analysis and respective molecular study of larval lisorchiid trematodes infecting the faucet snail, B. tentaculata, and some pulmonate snails, together with comparative studies of adults from fish hosts via molecular data. For this purpose, we used sequences of the nuclear ribosomal internal transcribed spacer 2 (ITS2) region and partial 28S rRNA gene. Molecular and karyological data obtained in this study will enable the future confident identification of all life-cycle stages and assessment of evolutionary pathways and intra-familial phylogenetic relations of lissorchiids.
Materials and methods
Collection and morphological identification of specimens
Adult specimens of Asymphylodora tincae were recovered from tench, Tinca tinca (L.). Adults of Parasymphylodora markewitschi and P. parasquamosa were found in common rudd, Scardinius erythrophthalmus (L.). Fishes were caught in different water bodies of Lithuania (table 1); T. tinca were caught in Ilmėdas Lake (55° 15′ 40″ N, 25° 33′ 46″ E); and S. erythropthalmus were obtained from Ilmėdas Lake and from Curonian Lagoon (55° 20′ 48″ N, 21° 11′ 42″ E). The gastrointestinal tract of fish was removed and examined for parasites using the gut wash method described by Cribb & Bray (Reference Cribb and Bray2010). Specimens found in the intestine were washed in saline solution, photographed with aid of a digital camera on the light microscope Olympus BX51 (Tokyo, Japan), and identified in vivo. The morphology of the adult worms agreed well with the morphological descriptions of these species provided in the relevant identification keys to fish trematodes (Bykhovskaya-Pavlovskaya & Kulakova, Reference Bykhovskaya-Pavlovskaya, Kulakova and Bauer1987; Niewiadomska, Reference Niewiadomska2003). After morphological identification, specimens were preserved in 96% ethanol for molecular analysis.
Table 1. Species subjected to karyological and/or molecular phylogenetic analysis with information for hosts, localities, karyotype and/or GenBank accession numbers.

Karyotypes and sequences generated in the present study are indicated in boldface type.
a Isogenophores and paragenophores were assigned isolate numbers and are stored in the helminthological collection of the Institute of Ecology of Nature Research Centre.
Molluscs were collected with strainers or hand-picked in water bodies of Lithuania (table 1). A. vortex and Stagnicola palustris, infected with A. tincae were collected in Balsys Lake (54° 47′ 7″ N, 25° 19′ 55″ E) and two ponds (54° 45′ 9″ N, 25° 17′ 31″ E and 54° 44′ 52″ N, 25° 16′ 38″ E) in Vilnius; and B. tentaculata infected with Parasymphylodora spp. were collected in Ilmėdas Lake (55° 15′ 40″ N, 25° 33′ 46″ E) and Stirniai Lake (55° 15′ 1″ N, 25° 36′ 11″ E). Molluscs were kept in the laboratory climate box at 18°C and examined within three days post-collection for the presence of naturally emerged cercariae. Cercariae were studied in the living condition and photographed under an Olympus BX51 microscope equipped with digital camera using both differential interference contrast and bright field microscopy. Some live specimens were stained with neutral red dye to better distinguish features. Representative infected snails were dissected in order to study the intramolluscan stages. Specimens of cercariae and rediae from one single infected snail host were pooled and fixed in 96% ethanol for molecular characterization. Lissorchiid metacercariae recovered from dissected snails also were collected and fixed in 96% ethanol. For molecular analysis, individual metacercariae were processed.
Larval stages were attributed to morphological groups Squamosum and Parasquamosum, and identified to the genus level using the appropriate morphological descriptions of Sobolev (Reference Sobolev and Skriabin1955), Bykhovskaya-Pavlovskaya & Kulakova (Reference Bykhovskaya-Pavlovskaya and Kulakova1971), and Kulakova (Reference Kulakova1972) and other relevant publications (e.g. Lambert, Reference Lambert1976; Chernogorenko, Reference Chernogorenko1983; Našincová & Scholz, Reference Našincová and Scholz1994; Besprozvannykh, Reference Besprozvannykh2005; Kudlai, Reference Kudlai2010). The consistently long tubular excretory vesicle reaching to the anterior margin of the testis primordium differentiates the cercariaeum of Parasquamosum group from Squamosum, in which the vesicle is small, pyriform or oval as in A. tincae (type species) and A. progenetica (fig. 1). Selected samples were subjected to further detailed karyological study and parallel molecular genetic analyses.

Fig. 1. Photomicrographs of live larval developmental stages: (A) cercariaeum of Parasymphylodora markewitschi; (B) cercariaeum of Parasymphylodora parasquamosa; (C) redia of Parasymphylodora parasquamosa; and (D) cercariaeum of Asymphylodora tincae. ExV = excretory vesicle. Scale bar = 100 mμ.
Metaphase chromosome preparations
For karyological analysis, living snails were incubated in 0.01% colchicine in well water for 3–5 h. Afterwards the snails were dissected and tissues containing trematode parthenitae were removed and treated in distilled water for 30–40 min at room temperature for hypotony. This material was fixed in a freshly prepared solution of ethanol and glacial acetic acid (3:1) with three changes, 20 min each. Chromosome spreads were prepared using an air-drying technique, as described previously (Petkevičiūtė et al., Reference Petkevičiūtė, Kudlai, Stunžėnas and Stanevičiūtė2015). Slide preparations were then treated with 1N hydrochloric acid for 10–15 min, rinsed three times in distilled water and stained with 4% Giemsa solution (pH 6.8) for 30–40 min. Chromosomes were examined with an Olympus BX51 microscope using 100× oil immersion objective. Measurements (absolute length in micrometres, relative length in percentage and centromeric indices) are given as mean values and standard deviations. Terminology relating to the centromere position follows that of Levan et al. (Reference Levan, Fredga and Sandberg1964). A chromosome was metacentric (m) if the centromeric index (CI) fell in the range 37.5–50.0, submetacentric (sm) in the range 25.0–37.5, subtelocentric (st) in the range 12.5–25.0 and acrocentric (a) if CI < 12.5. When a centromere position was on the borderline between two categories, two chromosome categories were listed. Data were analysed using Student's t test. Results were considered significant when P < 0.05.
Molecular sequencing and phylogenetic analyses
Genomic DNA was isolated from ethanol-fixed specimens according to Stunžėnas’ protocol (Stunžėnas et al., Reference Stunžėnas, Petkevičiūtė and Stanevičiūtė2011; Petkevičiūtė et al., Reference Petkevičiūtė, Stunžėnas and Stanevičiūtė2014). Amplification of the two rDNA regions was performed following the protocol used in our previous studies (Petkevičiūtė et al., Reference Petkevičiūtė, Stunžėnas and Stanevičiūtė2014, Reference Petkevičiūtė, Stanevičiūtė and Stunžėnas2020).
The ITS2 was amplified using the forward primer 3S (5′-CGG TGG ATC ACT CGG CTC GTG-3′) (Bowles et al., Reference Bowles, Blair and McManus1995) and the reverse primer ITS2.2 (5′-CCT GGT TAG TTT CTT TTC CTC CGC-3′) (Cribb et al., Reference Cribb, Anderson, Adlard and Bray1998). Part of the internal transcribed spacer 1, the complete 5.8S rDNA and ITS2, also a small section at the 5′ end of the 28S gene were amplified using forward primer GoJe-F (5′-CTTGCAATTGTTCCCCGTGA-3′) and the reverse primer GoJe-R (5′-CTGTTCACTCGCCGTTACTG-3′) (Petkevičiūtė et al., Reference Petkevičiūtė, Stanevičiūtė and Stunžėnas2020). A fragment at the 5′ end of the 28S rRNA gene was amplified using the forward primers Digl2 (5′-AAG CAT ATC ACT AAG CGG-3′) or ZX-1 (5′-ACC CGC TGA ATT TAA GCA TAT-3′) (Scholz et al., Reference Scholz, De Chambrier, Kuchta, Littlewood and Waeschenbach2013) and the reverse primers L0 (5′-GCT ATC CTG AG (AG) GAA ACT TCG-3′) (Tkach et al., Reference Tkach, Grabda-Kazubska, Pawlowski and Swiderski1999) or 1500R (5′-GCT ATC CTG AGG GAA ACT TCG-3′) (Olson et al., Reference Olson, Cribb, Tkach, Bray and Littlewood2003; Tkach et al., Reference Tkach, Littlewood, Olson, Kinsella and Swiderski2003).
Polymerase chain reaction (PCR) products were purified and sequenced in both directions at BaseClear B.V. (Leiden, Netherlands) using the PCR primers. Contiguous sequences were assembled using Sequencher 4.10.1 software (Gene Codes Corporation, Ann Arbor, USA). Sequences generated in this study have been deposited in the GenBank database (table 1).
The newly generated sequences were aligned and compared with sequences of other lissorchiid taxa available on GenBank (table 1). Sequence data for the Monorchiidae, the sister family to the Lissorchiidae, were included in this dataset. Both the ITS2 and 28S datasets were aligned independently using ClustalW (Thompson et al., Reference Thompson, Higgins and Gibson1994) with an open gap penalty of 15 and gap extension penalty of 6.66. The best-fit model of sequence evolution for phylogenetic analysis was estimated using jModeltest v.0.1.1 software (Posada, Reference Posada2008). Maximum likelihood (ML) phylogenetic trees were obtained and analysed using MEGA v.11.0.11 (Tamura et al., Reference Tamura, Stecher and Kumar2021). Branch support was estimated by bootstrap analyses with 1000 pseudoreplicates. The ML trees were obtained using the general time reversible model with a gamma distribution rate and a proportion of invariant sites (GTR + G + I) for both the ITS2 and the 28S gene datasets. The value for gamma and the number of invariant sites were estimated from the data. Parsimony analysis based on subtree pruning and regrafting was used with default parsimony settings. If two or more sequences belonged to one species, they were collapsed into one branch, except those newly obtained in this study. Estimates of mean evolutionary divergence over sequence pairs within and between groups were calculated using the MEGA v.11.0.11 programme.
Results
Molecular data and phylogenetic inference
Comparative molecular analysis of newly obtained 28S and ITS2 rDNA sequences of larval and adult developmental stages provided a definitive identification of larval lissorchiid trematodes. Sequence data from the cercariaeum developing in pulmonate snail A. vortex was identical to adult A. tincae from tench, T. tinca.
Faucet snail, B. tentaculata, was infected with two different lissorchiid species. Sequences generated for larval stages from B. tentaculata collected in Stirniai Lake were identical to those from sexual adults of P. markewitschi infecting common rudd, S. erythrophthalmus.
Comparative sequence analysis reliably confirmed that the other cercariaeum found in B. tentaculata collected in Ilmėdas Lake is the larval form of P. parasquamosa from common rudd. Sequences of encysted metacercariae of the lissorchiid trematodes, found in the tissues of B. tentaculata from the same locality, were identical to those of adults and cercariae of P. parasquamosa.
Alignments of the ITS2 and partial 28S data sets yielded 514 and 1267 characters for analysis, respectively; a couple sequence of the Lepocreadioidea and the Gorgoderidea were used as the outgroup. Both (ML and maximum parsimony) analyses of these datasets produced almost identical tree topologies (figs 2, 3); there all lissorchiid sequences clustered in one strongly supported main clade. Sequences representing the five genera of lissorchiids, so far molecularly analysed, form individual branches or clades. The phylogenetic analysis indicates two strongly supported clades (fig. 2), one of the largest containing four species of the genus Asymphylodora and the other – two Parasymphylodora species; also in addition, three species of the genus Lissorchis formed a strongly supported clade in the ITS2 tree (fig. 3). A branch of Asaccotrema vietnamiense formed a clade with Parasymphylodora species (fig. 2), but this relationship is not so well supported. Palaeorchis incognitus and Lisorchis kritskyi clustered as independent branches. Palaeorchis incognitus was closest to the main clade node in the both trees.
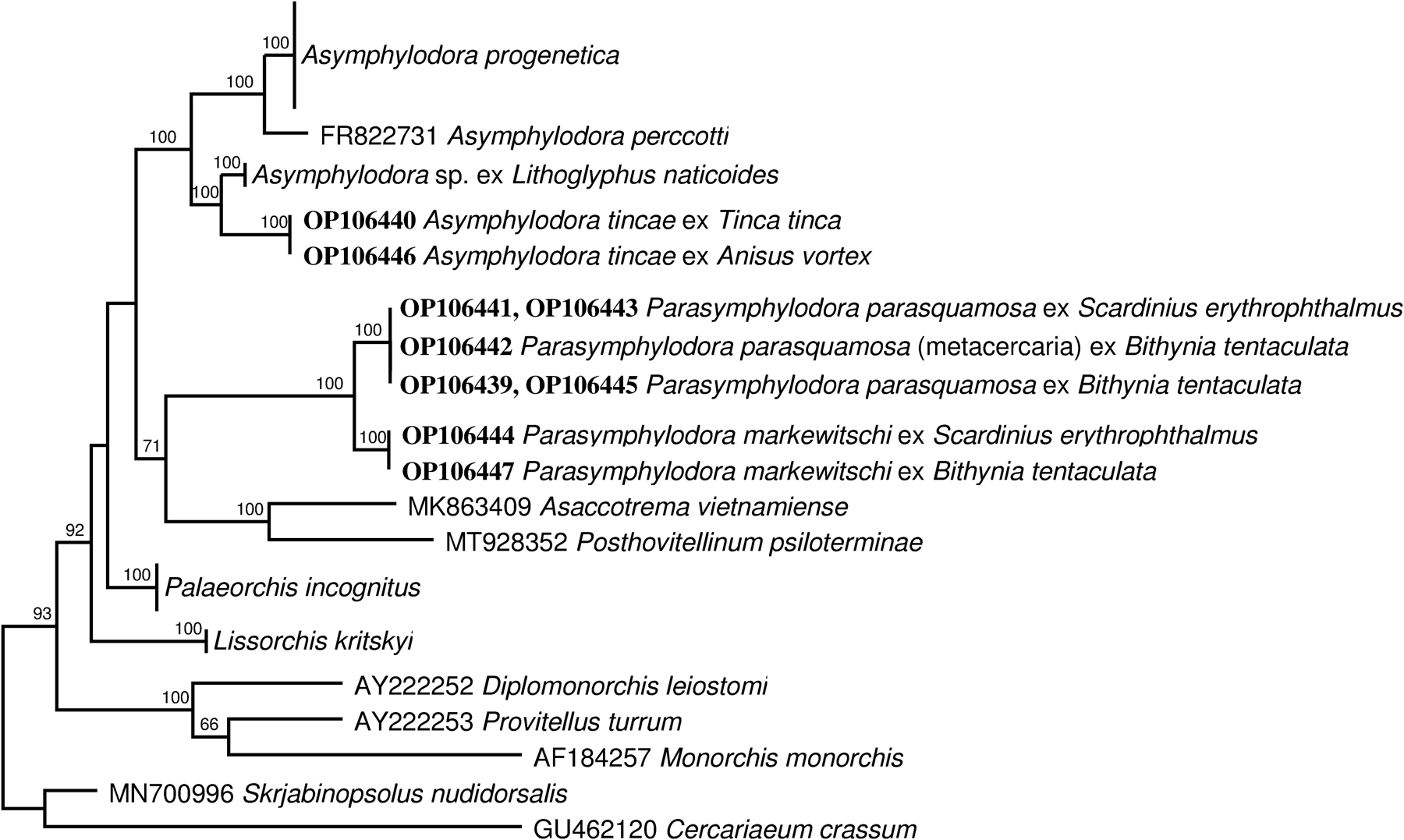
Fig. 2. Phylogenetic tree based on maximum likelihood analysis of partial sequences of the 28S nuclear rDNA gene. Bootstrap support values lower than 70% are not shown. The GenBank numbers of sequences generated in this study are indicated in boldface type.

Fig. 3. Phylogenetic tree based on maximum likelihood analysis of the internal transcribed spacer nuclear rDNA region. The GenBank numbers of sequences generated in this study are indicated in boldface type.
Intergeneric divergence among lissorchiid genera in the 28S fragment was 3.3–11.6% and 4.8–16% for the ITS2 fragment. The 28S sequence of A. vietnamiense had the greatest divergence from other lissorchiid: 10.9%–12%. Surprisingly, the studied sequences of Parasymphylodora species have one of the greatest divergences from other lissorchiid genera; even from phylogenetically closest Asymphylodora species, their ITS2 and 28S fragment reached 16% and 11.3% divergence, respectively. Interspecific ITS2 and 28S fragment divergence of Asymphylodora species varied from 4.8%–11.5% and 3.3%–7%, respectively. ITS2 and 28S fragment divergence of the Parasymphylodora species was 3.5% and 2.5%, respectively.
Species diversity of larval stages
Pulmonate snails A. vortex, and S. palustris, and prosobranch snail B. tentaculata from different localities in Lithuania (table 1) were found to serve as first intermediate hosts for different morphotypes of cercariaeum. These intramolluscan infections broadly corresponded morphologically to those larvae of known lissorchiids in that the cercariaeum develops in rediae without collar or locomotory appendages and have a spinous tegument, well-developed oral and ventral suckers, muscular pharynx, one large testis and tubular or oval excretory vesicle. The morphology of the present cercariae is in good agreement with characteristics of the cercariaeum assigned to the morphological groups Squamosum (larvae developing in rediae; testis single, the excretory vesicle small, pyriform or rounded) and Parasquamosum (differs from the first group in that the excretory vesicle is tubular and thick-walled) and corresponding to the genera Asymphylodora and Parasymphylodora, respectively.
Asymphylodora tincae (Modeer, 1790).
Morphological group of cercariaeum: Squamosum.
First intermediate host: A. vortex (Linnaeus, 1758) (Gastropoda: Planorbidae), S. palustris (O.F. Müller, 1774) (Gastropoda: Lymnaeidae).
Localities: Verkiai pond, Vilnius, Lithuania; Balsys Lake, Lithuania.
Representative sequences: OP106446 (28S) and OP106426 (5.8S-ITS2-28S).
Karyotype structure: 2n = 20 = 6 m + 10sm + 4st.
Remarks: All our lisorchiid cercariae from the pulmonate snails have small thin-walled pyriform excretory vesicle (fig. 1D) and develop in rediae containing cercariae of the same state of maturation. General morphology of cercariae corresponds well to cercariaeum of A. tincae described by Našincová & Scholz (Reference Našincová and Scholz1994): the body tailless, covered with tegumental spines; the oral sucker subterminal, slightly smaller than ventral sucker; the prepharynx conspicuous, the pharynx oval, muscular; the oesophagus bifurcated immediately anterior to the ventral sucker; the excretory vesicle thin-walled and small; the testes single and anterior to the excretory vesicle; and the cirrus sac primordium and metraterm lie posterolateral to the ventral sucker.
Parasymphylodora markewitschi (Kulakovskaja, 1947).
Morphological group of cercariaeum: Parasquamosum.
First intermediate host: B. tentaculata (Linnaeus, 1758) (Gastropoda: Bithyniidae).
Locality: Stirniai Lake, Lithuania.
Representative sequences: OP106447 (28S) and OP106430 (5.8S-ITS2-28S).
Karyotype structure: 2n = 14 = 8sm + 2 m + 2st + 2a/st.
Remarks: Emerged specimens were attached to the tentacles of the host snail. The morphology of these larval stages is in good agreement with the morphology of larval stages of P. markewitschi from B. tentaculata described by Lambert (Reference Lambert1976) and Kulakova (Reference Kulakova1972). Key features are tailless body, the tegument covered by small spines; the suckers more or less the same size; pharynx well developed, oval; intestinal bifurcation slightly in front of ventral sucker; and single large testis, excretory vesicle long, tubular, surrounded by a dense row of cells (fig. 1A).
Parasymphylodora parasquamosa Kulakova, Reference Kulakova1972.
Morphological group of cercariaeum: Parasquamosum.
First and second intermediate host: B. tentaculata (Linnaeus, 1758) (Gastropoda: Bithyniidae).
Locality: Ilmėdas Lake, Lithuania.
Representative sequences: OP106439, OP106442, OP106445 (28S) and OP106425, OP106429 (5.8S-ITS2-28S).
Remarks: We found a single pre-patent infection with lissorchiid rediae and cercariae (fig. 1B, C). The rediae are consistent with previously reported lissorchiid rediae from this host in being cylindrical sacs without locomotory appendages, with spherical pharynx and short caecum, containing cercariae of varying developmental stages (fig. 1C). Morphologically cercariae conformed to the descriptions of Parasymphylodora spp. cercariae published by Kulakova (Reference Kulakova1972). However, the two species, P. markewitschi and P. parasquamosa, are very similar and differ mainly in the location of vitelline follicles, but in the intramolluscan cercariaeum from our material vitellaria were not yet visible. Encysted metacercariae of P. parasquamosa were found in the same snail species from the same locality.
Chromosome set structure
The karyotypes of intramolluscan stages of three species were studied.
The chromosomes of 36 mitotic cells of larval A. tincae ex A. vortex and S. palustris were analysed. Most cells had 20 chromosomes in the diploid complements. Figure 4 shows them arranged in order of decreasing size. Two aneuploid cells (5.6%) displaying a chromosome number lower than the mode (2n = 19) were encountered. The chromosomes are middle sized. Measurements of absolute length give mean values from 1.76 to 7.59 μm. The mean total chromosome length (TCL) of the haploid complement is 35.79 μm. Two first pairs of metacentric elements are distinctly larger than the remaining chromosomes and contributed 33.75% to the TCL (table 2). Most chromosomes are biarmed with medially or sub-medially localized centromeres; chromosome pairs 4 and 7 have sub-terminal centromeres and represent a subtelocentric type of structure. The homologues of pairs 8 and 9 could not be distinguished clearly. There are no statistically significant differences in their length and centromeric indices.

Fig. 4. Mitotic chromosomes of Asymphylodora spp.: (A) A′, mitotic metaphase and karyotype of Asymphylodora tincae; (B) B′, Asymphylodora progenetica; and (C) C′, Asymphylodora markewitschi. Scale bar = 10 mμ.
Table 2. Measurements (means ± standard deviation) and classification of chromosomes of Asymphylodora sensu lato.

RL = relative length; CI = centromeric indices; C = classification (m = metacentric; sm = submetacentric; st = subtelocentric; a = acrocentric chromosomes).
A total of 47 mitotic cells of A. progenetica ex B. tentaculata was examined. The modal number of the chromosomes, 2n = 20, was found in 44 (93.6%) of the cells – 3 (6.4%) aneuploidy. In general, the structure of this karyotype is very similar to that of A. tincae, both with respect to the relative length and the centromeric indices of corresponding chromosomes (fig. 4; table 2). The mean absolute length of the chromosomes ranges from 1.92 to 8.34 μm, that is, they are somewhat larger than those of A. tincae. The TCL of the haploid complement is 39.73 μm. Differences in the absolute length could be partially accounted for by the different degree of condensation of the chromosomes on the preparations. Statistically significant interspecific difference was revealed in the relative length value of chromosome pair 1; they are slightly larger than the corresponding chromosomes in the karyotype of A. tincae and comprise 21.0% vs. 17.62% of the TCL. Comparing centromeric indices of individual chromosomes showed small but statistically significant differences in pairs 3 and 7. The centromeres of these chromosomes in A. progenetica are located more medially and the values of the centromeric indices are higher.
Examination of 52 mitotic metaphase plates of P. markewitschi ex B. tentaculata revealed the modal diploid number 2n = 14 (fig. 4). Five spreads (9.6%) displaying values lower than modal, represent aneuploidies or (more likely) loss of chromosomes during the slide preparation. The largest chromosome measured 7.82 μm, while the smallest were 2.55 μm long (table 2). The mean TCL of the haploid complement is 36.16 μm. According to the centromere position, chromosome pairs 1, 2, 3 and 5 are considered metacentric, pairs 4 and 7 are subtelocentric or acrocentric/subtelocentric, respectively, and pair 6 is metacentric (table 2).
Discussion
Identification of larval stages of lissorchiid trematodes has a long and complicated history and there are still many controversies in the data on their life cycles (for a review see Stunkard, Reference Stunkard1959; Našincová & Scholz, Reference Našincová and Scholz1994). In order to systemize existing data Dubois (Reference Dubois1929) established five groups for the tailless larvae, the cercariaeum. Szidat (Reference Szidat1943) declared that the larvae of Asymphylodora certainly belong in the ‘Squamosum’ group of Dubois. The larvae belonging to this group develop in rediae and have a single testis; their excretory vesicle is small, pyriform or rounded. While the ‘Helveticum’ group, characterized by the presence of two testes and tubular excretory vesicle, contains the developmental stages of Palaeorchis. Cercariae of a new genus, Parasymphylodora, erected by Szidat (Reference Szidat1943), possessing a single testis and tubular thick-walled excretory vesicle, were assigned to the ‘Parasquamosum’ group.
Our morphological observations of lissorchiid cercariaeum developing in various gastropod snails have led to the discovery of larvae corresponding to the Squamosum and Parasquamosum groups. In addition to our previous finding of A. progenetica ex B. tentaculata (Petkevičiūtė et al., Reference Petkevičiūtė, Stanevičiūtė and Stunžėnas2020), subsequent molecular analysis in this study resulted in the discovery of three other distinct species-level lineages: larval and adult A. tincae ex A. vortex and Tinca tinca; P. markewitschi ex B. tentaculata and Scardinius erythrophthalmus; and P. parasquamosa ex B. tentaculata and S. erythrophthalmus.
Molecular analyses demonstrate unequivocally that the six sequenced species of Asymphylodora sensu lato represent two distinct clades. The position of the type species, A. tincae, provides the phylogenetic position of Asymphylodora sensu stricto, with which A. perccotti, A. progenetica and Asymphylodora sp. ex Lithoglyphus naticoides forms a well-supported clade (fig. 3). Parasymphylodora markewitschi and P. parasquamosa are grouped into a separate strongly supported clade. Considering their morphological and karyological features, as well as the molecular phylogenetic data, it can be said that the validity of the genus Parasymphylodora should be confirmed. However, in order to definitively establish the taxonomic status of Parasymphylodora, the inclusion of DNA sequences from Asian species of Parasymhylodora, that is, A. macrostoma and A. indica, in phylogenetic analysis is highly desirable.
The development of A. tincae was elucidated experimentally by Našincová & Scholz (Reference Našincová and Scholz1994) and it has been shown that the definitive host, the tench, T. tinca, became directly infected after the ingestion of pulmonate snails harbouring rediae with mature cercariae. However, Zietse et al. (Reference Zietse, Van Den Broek and Erwteman-Ooms1981) infected tench, T. tinca, with metacercariae designated as A. tincae, but they used larval stages from the snail B. tentaculata. Van den Broek & de Jong (Reference Van den Broek and de Jong1979) in their study also argue that B. tentaculata acts as first intermediate host of A. tincae and encysted as well as free progenetic metacercariae can be found within one snail. The taxonomic status of the species developing in Bithynia and erroneously designated as A. tincae in these studies is not clear. Confusion in the identification of larval stages from pulmonate snails and the genus Bithynia, hitherto identified as A. tincae by other authors, were briefly discussed by Našincová & Scholz (Reference Našincová and Scholz1994). However, B. tentaculata is still referred to as the intermediate host of A. tincae in some faunistic studies (Morley et al., Reference Morley, Adam and Lewis2004; Serbina, Reference Serbina and Be′er2010, Reference Serbina2014). Molecular results based on ITS2 and 28S rDNA sequences confirm that the type-species of the genus Asymphylodora, A. tincae, use pulmonate snails as first intermediate host, and tench, T. tinca, as the definitive host; cercariaeum belongs in the Squamosum group; the metacercarial stage was not detected.
Bithynia tentaculata acts as both first and second intermediate host (and final for A. progenetica) for different lissorchiid digeneans. During our recent study on the trematodes developing in B. tentaculata we found intramoluscan stages of three different species. Cercariaeum of two species definitely corresponds to the group Parasquamosum. One of these morphotypes was genetically consistent with the adult P. markewitschi, while the other was conspecific with the adult P. parasquamosa. The adults of both species are recovered from common rudd, S. erythrophthalmus. The results obtained in our study confirm previous experimental data on life cycles. The life-cycle of Asymphylodora (= Parasymphylodora) markewitschi was studied and developmental stages described by Lambert (Reference Lambert1976). The following life-cycle was established: the molluscan first intermediate host is B. tentaculata; the second intermediate host is another aquatic mollusc, most often Ampullaceana balthica (syn. Lymnaea limosa); and the definitive host is the fish Leuciscus cephalus. The cercariae migrate to the mollusc's tentacles, where they form a living coat. The life-cycle of this species in Primorye land, Far-East Russia, was studied by Besprozvannykh (Reference Besprozvannykh2005); cercariae were detected in Boreoelona (= Bithynia) ussuriensis and Boreoelona contortrix and adults – in cyprinid fish. Larval and adult stages of P. parasquamosa were described by Kulakova (Reference Kulakova1972). Cercariae of this species develop in rediae in B. tentaculata. In the possession of a single large oval testis, relatively small cirrus sac, tubular and flexible thick-walled excretory bladder, cercariaeum of P. parasquamosa closely resembles cercariaeum of P. markewitschi and clearly belongs to the Parasquamosum group.
Asymphylodora progenetica was described from B. tentaculata in Russia (Serkova & Bychovsky, Reference Serkova and Bychovsky1940). The life cycle of A. progenetica has been shortened to a single host. In this life cycle the cercariae do not leave mollusc but reach their sexual maturity in the same host by means of progenetic development throughout the stage of metacercaria up to the adult trematode that produces the eggs. Kulakova (Reference Kulakova1982) found and described gravid specimens of this species from the ide, Leuciscus idus (L.), and confirmed the opinion of the previous authors that this species can mature in fish as well as in mollusc host. Based on examination of specimens from fish and snail host Kulakova (Reference Kulakova1982) transferred the species to the genus Parasymphylodora. However, the subsequent morphological study (Kudlai, Reference Kudlai2010) of cercariaeum of A. progenetica showed that it does not conform to the concept of the group Parasquamosum (it is characterized with small and thin walled excretory bladder instead of tubular thick walled) and, consequently, its attribution to the genus Parasymphylodora is unfounded. The DNA sequences of intramolluscan stages of A. progenetica were obtained in a previous study (Petkevičiūtė et al., Reference Petkevičiūtė, Stanevičiūtė and Stunžėnas2020). The present phylogenetic analysis showed that this species does not indeed cluster with the other two representatives of the genus Parasymhylodora. Asymphylodora progenetica is closely related to A. tincae and form a highly supported clade together with A. perccotti and unidentified larval Asymphylodora sp. from L. naticoides.
Palaeorchis is represented in the analysis by only one species, P. incognitus, with intramolluscan stages from the gravel snail, L. naticoides, which form a distinct clade. This genus is included in the subfamily Lissorchiinae Magath, 1917 characterized by double testis. Noticeably, Lissorchis kritskyi Barnhart & Powell, 1979, representative of the type-genus Lissorchis, in the present molecular analyses do not form a common branch and show to be distant from Palaeorchis. However, the resolution of the phylogeny of Lissorchiinae with the available data is poor. In general, molecular phylogenetic analysis does not confirm the legitimacy of dividing lissorchiid species into two different subfamilies. Such a morphological feature as the number of testes, on which two subfamilies are based, does not seem phylogenetically significant. It should be noted that although species of Asymphylodorinae Szidat, Reference Szidat1943 are characterized by single testis, some species have two sperm ducts, indicating the dual nature of the testis (Bray, Reference Bray, Jones, Gibson and Bray2008; Shimazu, Reference Shimazu2016). Similarly, the species of the gorgoderid genera Gorgodera and Gorgoderina, differentiated on the basis of number of testes, nested as closely related taxons in the clade containing also species of Phyllodistomum sensu stricto in the phylogenetic trees from 28S and ITS2 data sets (Cutmore et al., Reference Cutmore, Miller, Curran, Bennett and Cribb2013; Petkevičiūtė et al., Reference Petkevičiūtė, Stanevičiūtė and Stunžėnas2020). Therefore, the existing molecular phylogenies of the lissorchiid and gorgoderid trematodes indicate that the number of testes is a homoplasious trait.
Data on chromosome set structure are expected to yield relevant information usable for the reconstruction of the evolution of the group and for better understanding of phylogeny of related taxa. Unfortunately, the chromosomal analysis is randomly explored in taxonomic and phylogenetic studies of parasitic flatworms. Chromosome numbers and karyotypes have been previously described for six species of the family Lissorchiidae (Baršienė, Reference Baršienė1993; Baršienė et al., Reference Baršienė, Roca, Tapia and Martin1995; Petkevičiūtė et al., Reference Petkevičiūtė, Stanevičiūtė and Stunžėnas2020). Four of them have a diploid number of 20. Of these, karyotypes of two unidentified larval Asymphylodora sp. collected in Lithuania and Poland, and larval lissorchiid (regarded as Monorchiidae in the former taxonomy) from Spain were characterized by both the presence of two large metacentric pairs and the predominance of metacentric and submetacentric chromosomes (Baršienė, Reference Baršienė1993; Baršienė et al., Reference Baršienė, Roca, Tapia and Martin1995). Another larval Asymphylodora sp. from Lithuanian freshwaters showed 2n = 22 with one pair of large metacentric elements (Baršienė, Reference Baršienė1993). Apparently, all these species form a karyotypically closely related group; the difference in diploid number, 2n = 20 or 2n = 22, was most likely due to Robertsonian translocation. Cytogenetic theory leads to the prediction that Robertsonian translocations (centromeric fusions or fissions) would be among the most common types of chromosomal rearrangement incorporated in evolution (White, Reference White1978; Baker & Bickham, Reference Baker and Bickham1986). Comparative analysis indicates that changes in the number of chromosomes in the related groups of trematodes resulted from Robertsonian translocations rather than elimination of chromosomes (Grossman et al., Reference Grossman, Short and Cain1981; Petkevičiūtė et al., Reference Petkevičiūtė, Kudlai, Stunžėnas and Stanevičiūtė2015). Palaeorchis incognitus also presents 2n = 20, however, the karyotype of this species consists mainly of chromosomes with subterminally located centromeres, with karyotype formula of 8st + 4st-sm + 8sm and should be regarded as more ‘primitive’ in comparison with lissorchiid karyotypes composed of predominantly biarmed elements (Petkevičiūtė et al., Reference Petkevičiūtė, Stanevičiūtė and Stunžėnas2020).
In the current study, we provide new chromosomal data in three lissorchiid species. This survey revealed remarkable interspecific differences in chromosome set structure and confirmed the results observed in the previous studies. The diploid chromosome number, 2n = 20, and the karyotypes of A. tincae and A. progenetica are in concordance with those reported for the other representatives of the genus Asymphylodora. The noted high degree of similarity between the karyotypes of these two species corresponds to their close position in molecular phylogeny (fig. 3). However, neither the diploid chromosome number, 2n = 14, nor the karyotype of P. markewitschi resemble any of those reported for Asymphylodora spp. It is difficult to elucidate the possible pathways of formation of such different chromosome sets in related species and this is a very unusual case of karyotypic divergence among trematodes. The diploid set with 2n = 14 and identical morphology of corresponding chromosomes was reported for larval stages of Palaeorchis sp. from B. tentaculata (Baršienė, Reference Baršienė1993). It is likely that this cercaria has been misdiagnosed, and we actually have studied the same species. The complement of the mean total length of haploid chromosome of A. markewitschi, despite low chromosome number, reached 36.16 μm, that is, it is not significantly different from that of A. tincae or A. progenetica with 2n = 20 reaching 35.79 and 39.73 μm, respectively. There is a low correlation between nuclear DNA content and chromosome number (Méndez et al., Reference Méndez, Insua, López-Piñón and Méndez2001); however, in some animal groups positive correlations between the total length of the chromosome complement (TCL) and the total DNA content of the chromosomes have been shown (Rao & Rai, Reference Rao and Rai1987; Nur, Reference Nur and Rosen1989). It can be assumed that the 2n = 14 karyotype should be considered as a derivative state that arose as a result of centric fusion events, and the decrease in the number of chromosomes is not associated with the loss of chromosomal material. However, the sparsity of cytogenetic information in Lissorchiidae and the unknown situation in the other Parasymphylodora spp. does not allow to identify the point where the transformation from 2n = 20 to 2n = 14 took place. Furthermore, the revealed degree of molecular and karyotypic divergence of P. markewitschi falls within the range of intergeneric differences and confirms the rightful assignment of this species to the separate genus.
It should be noted that the chromosome sets composed of 22 or 20 elements are characteristic for the great majority of trematode species. Diploid number 2n = 14 is less common between digeneans but it was revealed in species of some unrelated families such as Paramphistomidae Fischoeder, 1901, Bucephalidae Poche, 1907, Echinochasmidae Odhner, 1910, Allocreadiidae Looss, 1900 or Opisthorchiidae Looss, 1899 (Baršienė, Reference Baršienė1993; Petkevičiūtė & Stanevičiūtė, Reference Petkevičiūtė and Stanevičiūtė2008; Petkevičiūtė et al., Reference Petkevičiūtė, Stunžėnas and Stanevičiūtė2014). From the new data on the karyotypes of lissorchiids, it is obvious that representatives of some families of trematodes can demonstrate varying degrees of chromosomal diversification, sharing conservative and highly derived karyotypes. In this perspective, the present data have added entirely new cytogenetic information in Trematoda. This finding is in accordance with the opinion of Baker & Bickham (Reference Baker and Bickham1980) that the rates of karyotypic evolution do not behave in a clock-like fashion and that there are periods of relatively rapid and extensive change in some lineages.
Overall, this study provides updated information on the phylogeny, diversity and life cycles of lissorchiid trematodes and can help in studying the ecological characteristics of freshwater ecosystems.
Financial support
This research was carried out under the long-term institutional research and experimental development (IIMTEP) program Biodiversity based at the Nature Research Centre (Lithuania), but received no specific grant from any funding agency, commercial or not-for-profit sectors.
Conflicts of interest
None.
Ethical standards
All applicable institutional, national and international guidelines for the care and use of animals were followed. All procedures performed in studies involving animals were in accordance with the ethical standards of the institution or practice at which the studies were conducted.