Introduction
Members of the genus Muntiacus Rafinesque, 1815, currently classified into 11 species with more than a dozen subspecies, are small-sized cervid species originally distributed in India, South-East Asia and southern China (Wildon & Reeder, Reference Wildon and Reeder2005; Groves & Grubb, Reference Groves and Grubb2011). Reeves's muntjac, Muntiacus reevesi (Ogilby, 1839), is indigenous to south-eastern China and Taiwan, but naturalized in Britain and Japan, at least, after escape from an animal exhibition facility or intentional release (Chapman et al., Reference Chapman, Harris and Stanford1994; Ohdachi et al., Reference Ohdachi, Ishibashi, Iwasa and Saitoh2010). In Japan, Reeves's muntjacs are expanding their population at two localities, i.e. the southern region of the Boso Peninsula, Chiba Prefecture and Izu-Oshima Island, Tokyo, where approximately 50,000 and 17,000 muntjacs, respectively, are currently estimated to be populating the natural environment after escape from zoological facilities (Chiba Prefectural Office, 2013, 2017; Tokyo Municipal Office, Reference Anisimova and Gascuel2017). The escape and naturalization of Reeves's muntjacs is thought to have happened between 1960 and 1980 on the southern Boso Peninsula, covering more than 1400 km2, while a typhoon in the autumn of 1970 afforded their escape from Tokyo Municipal Oshima Park Zoo on the 91.1 km2 island of Izu-Oshima. Control measures for this alien mammalian species were initiated in 2000 and 2007, respectively, and the total trapped animal numbers in 2014, 2015 and 2016 were 2160, 2187 and 2400 on the Boso Peninsula (Chiba Prefectural Office, 2017) and 1022, 1412 and 2191 on Izu-Oshima Island (Tokyo Municipal Office, 2017), respectively. However, these measures have been deemed to have had an insufficient impact on population control.
Despite the remarkable progress of the naturalization of alien Reeves's muntjacs in Japan, few parasitological investigations have been conducted. Earlier work from our laboratory reported a case of Gongylonema pulchrum parasitism in the oesophagus of a trapped Reeves's muntjac on Izu-Oshima Island (Makouloutou et al., Reference Makouloutou, Setsuda and Yokoyama2013; Setsuda et al., Reference Setsuda, Varcasia and Scale2018). In the present study, we examined the gastrointestinal helminths of feral alien Reeves's muntjacs trapped on Izu-Oshima Island to determine whether these animals maintain any gastrointestinal helminths brought from their original endemic area or have newly acquired ones following their introduction to Japan.
Materials and methods
Parasite collection and morphological examination
Viscera of feral alien Reeves's muntjacs were collected on 26 January, 2015 (seven animals), 25 and 26 July, 2015 (15 animals) and 29 October, 2016 (ten animals) with the permission of the Tokyo Metropolitan Oshima Island Branch Office. All muntjacs of either sex were mature adults with body weights of 6.6–10.0 kg. Referring to the growth curve data of Asada (Reference Asada2014) indicating that the average body weights of male and female muntjacs naturalized on the Boso Peninsula reach 9.6 kg and 8.8 kg, respectively, at 1.5 years of age then plateau, all examined muntjacs were older than six months. Frozen viscera were transported to the Laboratory of Veterinary Parasitology, Yamaguchi University, and helminth parasites were collected according to a standard procedure (Sultan et al., Reference Sultan, Omar, Makouloutou, Kaneshiro, Saita, Yokoyama, Suzuki, Hosoi and Sato2014). Briefly, organs were separated, placed in different vessels, opened longitudinally or cut into blocks and washed in tap water. Following repeated washings, the sediments were checked under a dissection microscope. Collected parasites were washed in physiological saline and preserved in either neutral-buffered 10% formalin or 70% alcohol. For scanning electron microscopy (SEM), formalin-fixed worms were processed as described previously (Tran et al., Reference Tran, Sato and Luc2015).
DNA extraction, polymerase chain reaction (PCR) and sequencing
Parasite DNA was individually extracted from worms according to Setsuda et al. (Reference Setsuda, Da, Hasegawa, Behnke, Rana, Dhakal and Sato2016). PCR amplification of overlapping fragments of the nuclear ribosomal RNA gene (rDNA) was performed in a 25 µl volume using different primer combinations as previously described (Makouloutou et al., Reference Makouloutou, Setsuda and Yokoyama2013). The mitochondrial DNA cytochrome c oxidase subunit 1 gene (cox-1) region of worms was amplified by primer pairs of BpCoxI-F1 (5′-TTTGGTCATCCTGAGGTTTATATT-3′) and BpCoxI-R1 (5′-ATGAAAATGTCTAACTACATAATAAGTATC-3′), or CapiCox1_F (5′-GTGGTTTTGGTAATTGAATGGTA-3′) and BpCoxI-R1, according to Sultan et al. (Reference Sultan, Omar, Makouloutou, Kaneshiro, Saita, Yokoyama, Suzuki, Hosoi and Sato2014). The purification of PCR products using a commercial kit and subsequent nucleotide sequencing, including that of rDNA fragments containing the internal transcribed spacer (ITS) regions, were conducted as described in Setsuda et al. (Reference Setsuda, Da, Hasegawa, Behnke, Rana, Dhakal and Sato2016). The obtained sequences were assembled manually with the aid of the CLUSTAL W multiple alignment program (Thompson et al., Reference Thompson, Higgins and Gibson1994).
New nucleotide sequences reported in the present study are available from the DDBJ/EMBL/GenBank databases under the accession numbers LC415111–LC415114.
Phylogenetic analysis
Fragments of the newly obtained rDNA sequences and partial cox-1 sequences were submitted to the Basic Local Alignment Search Tool (BLAST) on the National Center for Biotechnology Information website to identify highly similar nucleotide sequences. Phylogenetic trees were constructed for the newly obtained sequences in the present study and those retrieved from the DDBJ/EMBL/GenBank databases. The accession numbers of the sequences analysed in the present study are given in the figures showing phylogenetic trees. Maximum likelihood (ML) analysis was performed with the program PhyML (Guindon & Gascuel, Reference Guindon and Gascuel2003; Dereeper et al., Reference Dereeper, Guignon and Blanc2008) provided on the ‘phylogeny.fr’ website (http://www.phylogeny.fr/), using 670 characters, of which 211 were variable, for trichostrongylid nematodes, or 679 characters, of which 200 were variable, for chabertiid nematodes. The probability of inferred branches was assessed by the approximate likelihood ratio test, an alternative to the non-parametric bootstrap estimation of branch support (Anisimova & Gascuel, Reference Anisimova and Gascuel2006).
Results
Prevalence of worms
In addition to G. pulchrum in the oesophageal mucosa of 11 (34.4%) Reeves's muntjacs, trichostrongylid and chabertiid nematodes were collected from the small and large intestines, respectively. Although the prevalence and intensity were rather varied at different collection times (table 1), 28.1% and 46.9% of a total of 32 examined muntjacs were parasitized with these nematodes, with an intensity of 1–47 (average 14.2) and 1–148 (average 41.5) worms, respectively.
Table 1. Worm recovery from the intestine of feral alien Reeves's muntjacs on Izu-Oshima Island, Tokyo.

a Worm recovery/animal, expressed as range and average in parentheses.
Chabaudstrongylus ninhae in the small intestine
Trichostrongylid nematodes collected from the small intestine had an oval cephalic vesicle, caudal bursa with a long dorsal ray deeply divided, didelphic uterus and synlophes, of which the lateral ridges were smaller than the median ridges. These morphological characters were consistent with the definition of the subfamily Cooperiinae (Skrjabin et Schultz, 1937) of the family Trichostrongylidae (Leiper, 1908), according to Lichtenfels (Reference Lichtenfels1980). Although the present specimens had a simple cephalic vesicle, other characters such as a short ovejector, less than 0.50 mm, and a long dorsal ray divided in the posterior third matched exactly with the definition of the genus Chabaudstrongylus Durette-Desset et Denké, 1978. In this genus, C. ninhae (Dróżdż, 1967) from an Indian muntjac (Muntiacus muntjak) in northern Vietnam and C. dubosti (Durette-Desset et Chabaud, 1974) Durette-Desset et Denké, 1978, from a water chevrotain (Hyemoschus aquaticus) in Gabon have been described (Dróżdż, Reference Dróżdż1967; Durette-Desset & Chabaud, Reference Durette-Desset and Chabaud1974; Durette-Desset & Denké, Reference Durette-Desset and Denké1978), and almost all the morphological characters of the present specimens were identical to those of the former species (table 2). A brief description of the present C. ninhae specimens is as follows.
Table 2. Comparison of measurements of Chabaudstrongylus spp. from muntjacs and chevrotains (in mm).a

a Values are expressed in range and average in parentheses, if available. ‘—’ denotes no data.
b Synonymized by Durette-Desset & Denké (Reference Durette-Desset and Denké1978).
c From the anterior end.
d From the posterior end.
Small-sized worms, 5–8 mm in length, with slight sexual dimorphism. Anterior end of both sexes with simple cephalic vesicle (fig. 1a). Synlophe well developed over entire worm length, with its pattern identical to that of C. dubosti (see Fig. 1E of Durette-Desset & Chabaud, Reference Durette-Desset and Chabaud1974 for C. dubosti). Copulatory bursa developed symmetrically, with the dorsal ray branched in the posterior third (fig. 1b). Externodorsal ray originated from the dorsal ray as illustrated in Fig. 1G of Durette-Desset & Chabaud (Reference Durette-Desset and Chabaud1974) for C. dubosti (fig. 1c). Peripheral ends of all rays extending to the edge of the bursa. Genital cone was characteristic structure, as shown in fig. 1d, which showed close resemblance to that of Chabaudstrongylus sp. illustrated by Gibbons and Khalil (Reference Gibbons, Khalil, Stone, Platt and Khalil1983). Two alate spicules golden brown in colour and equal in size and shape with ventral and dorsal processes at the peripheral end (fig. 1e). Vulva situated near the posterior fourth of female worms, transverse slit, connected directly to the ovejector (fig. 1f). Except for the bifurcation of internal peripheral branches of dorsal rays reported in the original description, the present specimens were identical in morphology to C. ninhae described by Dróżdż (Reference Dróżdż1967).

Fig. 1. Morphology of Chabaudstrongylus ninhae from feral alien Reeves's muntjacs. (a) Anterior end of male worm with a simple cephalic vesicle; (b, c) Ventral and dorsal views, respectively, of caudal end of male worm with a branched dorsal ray (DR) in the symmetrical copulatory bursa. Arrows indicate base of externodorsal ray; (d) Genital cone of male worm; (e) Alate, trifurcated spicules of male worm; (f) Ovejector between sphincters. Vu, vulva. Magnifications of photographs (b), (c) and (f) are identical and scale bar is shown in (c).
The present specimens and C. dubosti differed morphologically regarding the shape of the cephalic vesicle (simple vs. elongated), position of the nerve ring (at mid-oesophagus vs. almost at posterior fourth of oesophagus) and shape and size of spicules. However, these two species appeared to be closely related, particularly with regard to an almost identical arrangement and size of rays in the copulatory bursa.
Oesophagostomum muntiacum in the large intestine
Chabertiid nematodes collected from the caecum and colon had a small and shallow buccal capsule, transverse cervical groove at the level of the excretory pore and externodorsal rays arising from a common stem with the dorsal ray. These morphological characters were consistent with the definition of the subfamily Oesophagostominae (Railliet, 1916) of the family Chabertiidae (Popova, 1952), according to Durette-Desset (Reference Durette-Desset1983). Morphological characters of the present specimens such as an incomplete transverse cervical groove at the level of the excretory pore, external corona radiata with ten elements, internal corona radiata with 20 elements and short spicules less than 0.5 mm matched exactly with the definition of the genus Oesophagostomum Railliet et Henry, 1913. In this genus, Oesophagostomum (Hysteracrum) sp. (Yen, Reference Yen1973) from northern red muntjacs (Muntiacus vaginalis) in southern China, O. labiatum (Dróżdż, Reference Dróżdż1973) from Indian muntjacs in northern Vietnam and O. muntiacum Jian, 1989 from Reeves's muntjacs in central China have been described (Dróżdż, Reference Dróżdż1973; Yen, Reference Yen1973; Jian, Reference Jian1989), and mostly the morphological characters of the present specimens were identical to those of these species. Furthermore, Neorhabditostomum yeni Gibbons et Kan, 1987, resembling the three aforementioned species in morphology, was described from Indian muntjacs in Malaysia (Gibbons & Kan, Reference Gibbons and Kan1987). The genus Neorhabditostomum Gibbons et Kan, 1987, of the subfamily Oesophagostominae, was proposed based on a cephalic extremity with a single leaf crown of ten elements, and six internal labial papillae, transverse cervical groove completely encircling the body and unsegmented, and elongate oesophageal funnel (Gibbons & Kan, Reference Gibbons and Kan1987). Morphologically, the present specimens were almost identical to O. muntiacum, different from O. labiatum and N. yeni. A morphometric comparison of the present specimens with hitherto known Oesophagostomum spp. from muntjacs is shown in table 3. A brief description of the present specimens, O. muntiacum, is as follows.
Table 3. Comparison of measurements of Oesophagostomum spp. from muntjacs (in mm).a

a Values are expressed in range and average in parentheses, if available. ‘—’ denotes no available data.
b From the anterior end.
c From the posterior end.
d Values reported in the original article (0.245–0.262 mm from the posterior end) are apparently a mistake, and these must be the distances between the vulva and anus. By adding distance values between the anus and posterior end, estimated values are provided here.
Small-sized worms, 3–5 mm in length, with sexual dimorphism. Anterior end of both sexes with cephalic vesicle, delineated by cervical groove, particularly on the ventral side (fig. 2a–c). Excretory pores at the level of the cervical groove and cervical papillae at the level just posterior to the end of the oesophagus. Oesophageal funnel conspicuous and nerve ring at the border between the oesophageal corpus and bulb. Head delineated from cephalic vesicle, with four sub-median papillae and two amphids (fig. 3a–c). Mouth opening surrounded by external and internal coronae radiatae. External corona radiata with ten petal-like elements and two slender elements of each internal corona radiata at the base of each external corona radiata element (fig. 3d). Copulatory bursa developed symmetrically, as illustrated in Fig. 10 of Gibbons & Kan (Reference Gibbons and Kan1987), with externodorsal rays arising from a common stem with the dorsal ray (figs 2d, e and 3e). Spicules equal and slender, with a gubernaculum. Caudal portion of female worms tapering gradually with long slender tails, measuring 0.287–0.335 mm (fig. 2f). Vulval lips at 0.176–0.231 mm anterior to the anus. Intrauterine eggs oval, embryonated, with thin shells.
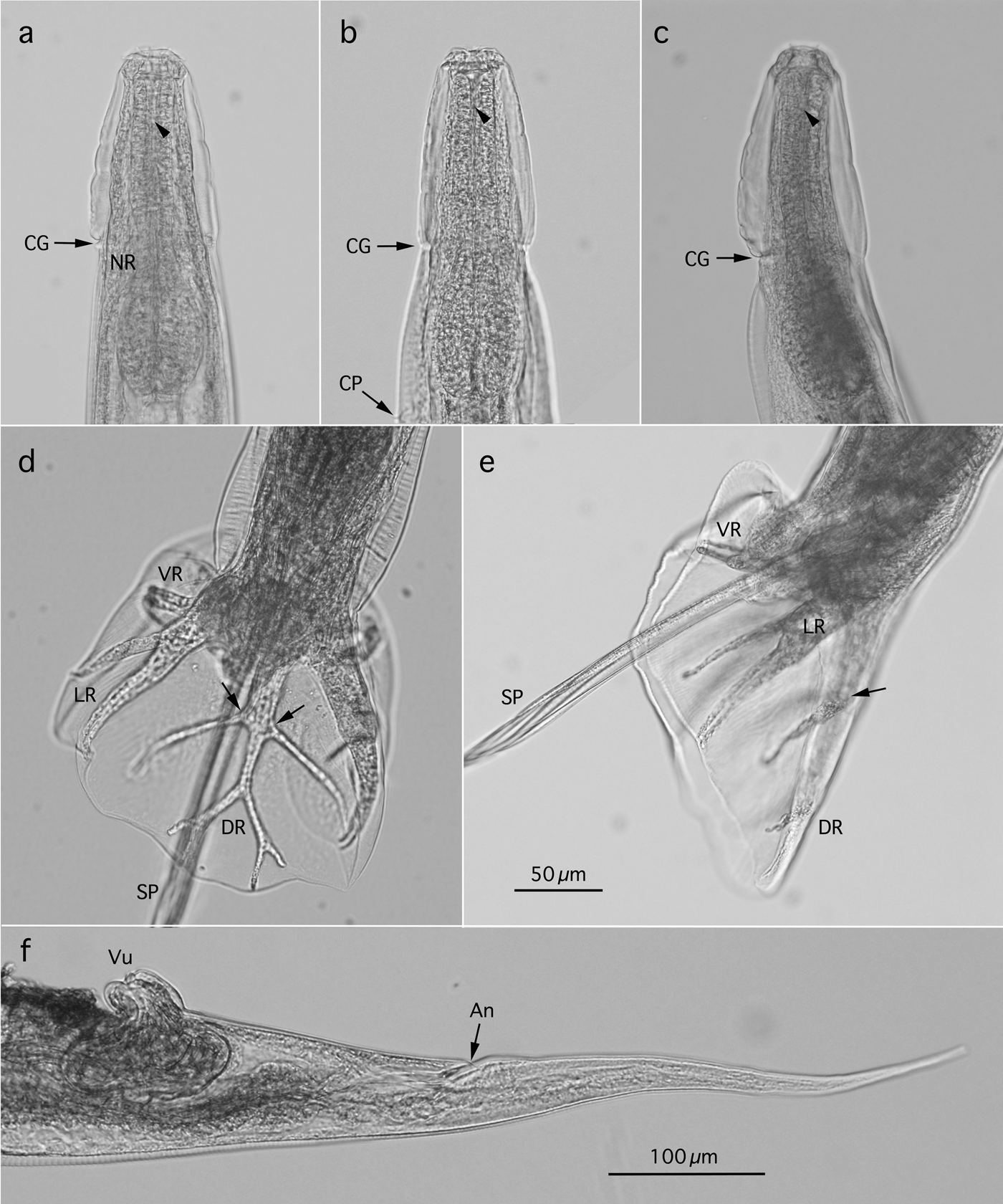
Fig. 2. Morphology of Oesophagostomum muntiacum from feral alien Reeves's muntjacs. (a, b) Lateral and ventral views, respectively, of anterior end of male worm with a cephalic vesicle delineated by the cervical groove (CG). Arrowhead indicates bottom of oesophageal funnel; (c) Lateral view of anterior end of female worm; (d) Dorsal view of caudal end of male worm with a branched dorsal ray (DR) in the symmetrical copulatory bursa. Arrows indicate base of externodorsal ray; (e) Lateral view of caudal end of male worm with a developed copulatory bursa supported by ventral, lateral and dorsal rays. Arrow indicates base of externodorsal ray; (f) Lateral view of caudal end of female worm. CP, cervical papilla; NR, nerve ring; LR, lateral ray; SP, spicule; VR, ventral ray; An, anus; Vu, vulva. Magnifications of photographs (a–e) are identical and scale bar is shown in (e).
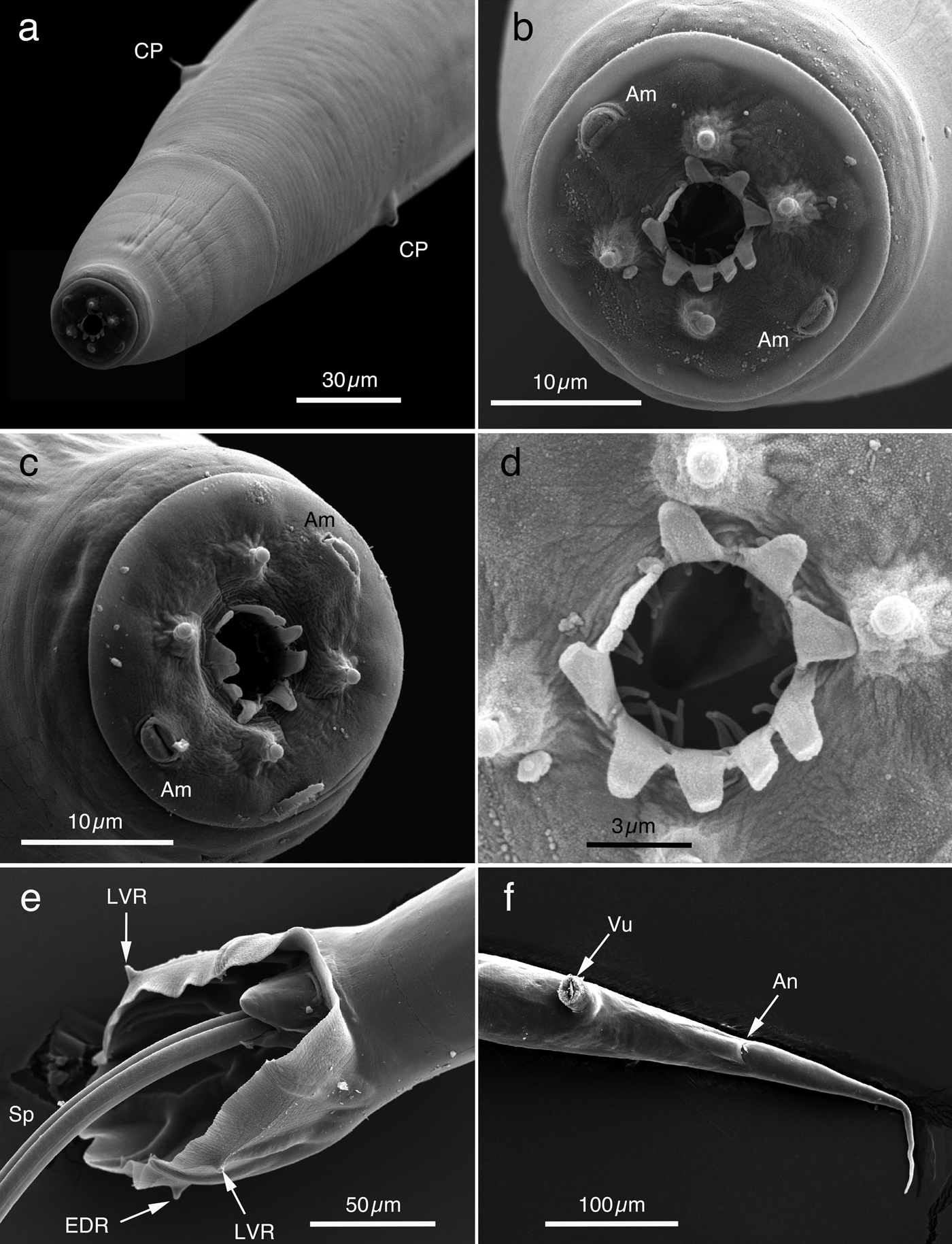
Fig. 3. SEM of Oesophagostomum muntiacum from feral alien Reeves's muntjacs. (a) Anterior end of male worm with cervical papillae (CP); (b) En face view of head of male worm shown in (a), delineated from cephalic vesicle, with four sub-median papillae and two amphids (Am). Mouth opening is surrounded by external and internal coronae radiatae. External corona radiata with ten petal-like elements and two slender elements of internal corona radiata at the base of each external corona radiata element; (c) Head of female worm, delineated from cephalic vesicle, with four sub-median papillae and two amphids (Am). Mouth opening is surrounded by ten petal-like elements of external coronae radiata; (d) Mouth opening of female worm shown in (b), surrounded by external and internal coronae radiatae; (e) Ventral view of copulatory bursa of male worm with protruded spicules (Sp). Some rays supporting the copulatory bursa do not reach the edge of the membrane (their tips are visible); (f) Ventral view of caudal portion of female worm. EDR, externodorsal ray; LVR, lateroventral ray; Vu, vulva; An, anus.
Neorhabditostomum yeni and O. muntiacum were independently described by Gibbons & Kan (Reference Gibbons and Kan1987) and Jian (Reference Jian1989), respectively, to identify the specimens of Yen (Reference Yen1973), Oesophagostomum (Hysteracrum) sp. Due to this reason, these three species resemble one another well morphologically, although some characters were characterized differently by the different authors. The Oesophagostomum specimens collected in the present study appeared to be identical to O. muntiacum, differentiated from N. yeni by its specific and/or generic definitions such as a cephalic extremity with a single leaf crown of ten elements without internal corona radiata, and six internal labial papillae, transverse cervical groove completely encircling the body, and longer spicules (0.454–0.557 mm vs. 0.379–0.447 mm when the body lengths of both species were almost identical) according to Gibbons & Kan (Reference Gibbons and Kan1987). Although O. labiatum recorded from Indian muntjacs in northern Vietnam (Dróżdż, Reference Dróżdż1973) had the same arrangement of bursal rays as O. muntiacum, it differed from O. muntiacum with respect to external corona radiata with six elements, internal corona radiata with 12 elements, cervical papillae located slightly behind the cephalic vesicle and worm dimensions (table 3).
Phylogenetic analysis
The rDNA nucleotide sequences were obtained for C. ninhae and O. muntiacum (5236-bp and 5165-bp long, respectively). The former sequence contained 1739-bp-long partial 18S rDNA, 414-bp-long ITS1, 144-bp-long 5.8S rDNA, 242-bp-long ITS2 and 2697-bp-long partial 28S rDNA, and the latter 1737-bp-long partial 18S rDNA, 372-bp-long ITS1, 144-bp-long 5.8S rDNA, 216-bp-long ITS2 and 2696-bp-long partial 28S rDNA. Submission of the newly obtained sequences of 18S or 28S rDNA to BLAST resulted in the identification of genetically related species in the same families, Trichostrongylidae and Chabertiidae. However, the resolution of these analyses was extremely low due to the paucity of deposited nucleotide sequences and extremely high identities of different species within the same family. For example, in the case of O. muntiacum (Chabertiidae: Oesophagostominae Railliet, 1916), Cyclodontostomum purvisi (Chabertiidae: Chabertiinae Popova, 1952) was identified as having the closest nucleotide sequences (DDBJ/EMBL/GenBank accession nos AJ920340 and AM039732). These two species showed 99.94% identity (1733/1734; 18S rDNA) with two insertion/deletion (indel) sites and 99.59% identity (2685/2696; 28S rDNA). The phylogenetic analyses of genera and/or species of Trichostrongylidae and Chabertiidae nematodes based on the ITS region of rDNA, however, showed a higher resolution (figs 4 and 5) and supported the morphological classification of the present specimens, C. ninhae and O. muntiacum, in the subfamilies Cooperiinae and Oesophagostominae, respectively.

Fig. 4. ML phylogenetic tree based on the ITS regions of rDNA sequence. Representatives of different species of Trichostrongylidae are shown and they are depicted by their DDBJ/EMBL/GenBank accession numbers. The original analyses were conducted with the following additional sequences: Cooperia oncophora (AB238687, AB238689, AB245027, AB245028, AB245030–AB245033 and AB245035–AB245045); Teladorsagia circumcincta (HQ389230, JF680984, JQ889795, JQ889797, KC295419 and KX929991–KX929993); Marshallagia marshalli (JQ839283, KC295417 and KC295418); Spiculopteragia houdemeri (AB367797, AB682690–AB682694, AB682696, AB682697, AB682699, AB682701 and AB682702); Libyostrongylus douglassi (HQ713426–HQ713430); Trichostrongylus colubriformis (AB908959, AB908960, HQ389229–HQ389231, KR002110 and KR002111); Trichostrongylus vitrinus (KR020011); and Trichostrongylus probolurus (JQ925867). Arrow indicates the newly obtained rDNA nucleotide sequence of Chabaudstrongylus ninhae in the present study.
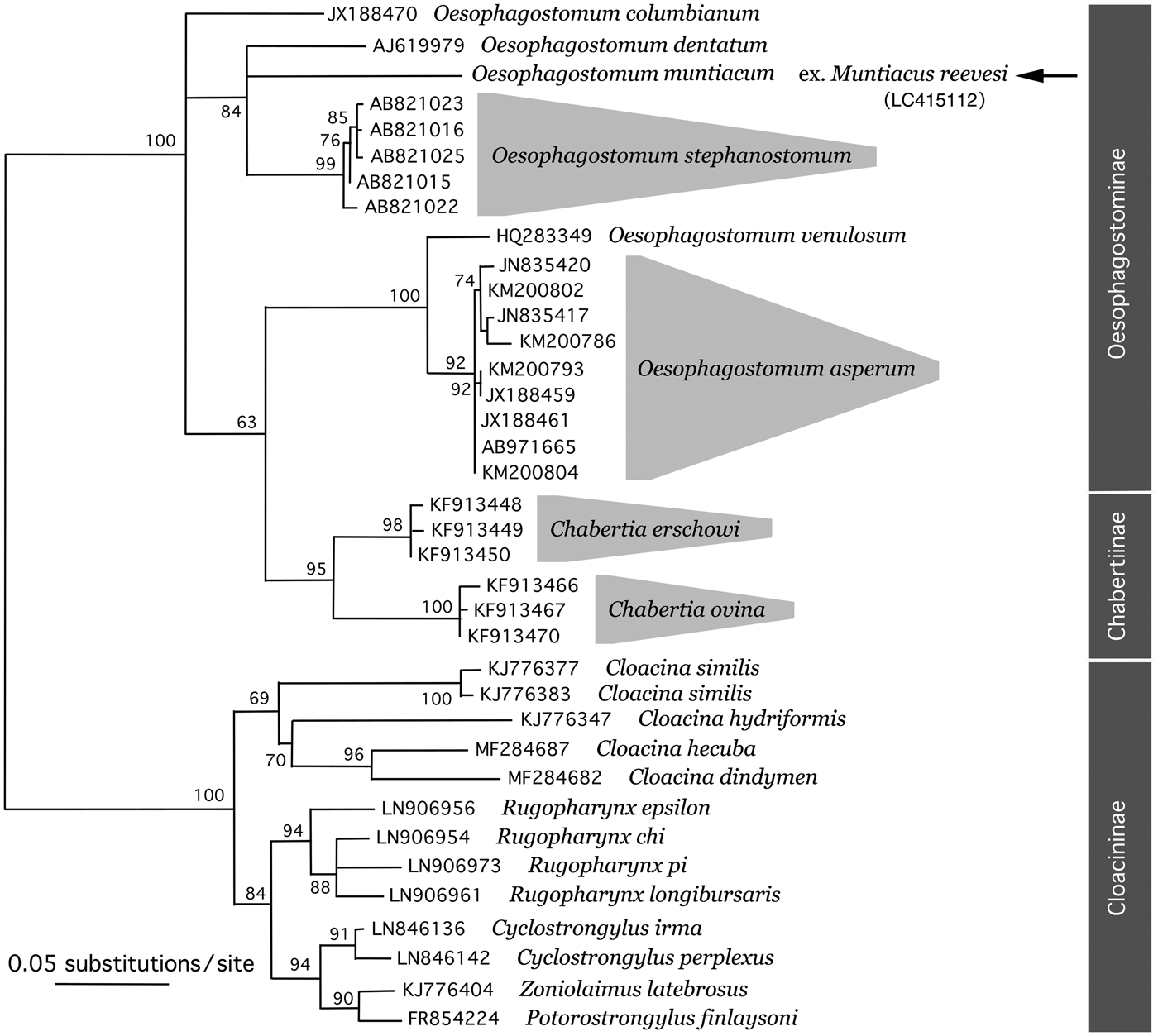
Fig. 5. ML phylogenetic tree based on the ITS regions of rDNA sequence. Representatives of different species of Chabertiidae are shown and they are depicted by their DDBJ/EMBL/GenBank accession numbers. The original analyses were conducted with the following additional sequences: Oesophagostomum columbianum (AB908964, AB908965, HQ844232 and JX188471); Oesophagostomum stephanostomum (AB821013, AB821014, AB821017–AB821021, AB821024 and AB821026–AB821031); Oesophagostomum asperum (JN835418, JN835419, JX188457, JX188458, JX188460, JX188462–JX188467, KM200785, KM200787–KM200792, KM200794–KM200801, KM200803, KM200805 and KM200806); Chabertia erschowi (KF913451–KF913456); Chabertia ovina (JF680981, KF913468, KF913469 and KF913471); Cloacina similis (KJ776380); Rugopharynx spp. (LN906955, LN906957–LN906960, LN906962–LN906972 and LN906974); and Cyclostrongylus spp. (LN846137–LN846141, LN846143 and LN846144). Arrow indicates the newly obtained rDNA nucleotide sequence of Oesophagostomum muntiacum in the present study.
As a result of different reactivities to the primer pairs used in the reactions, 375-bp- and 852-bp-long cox-1 nucleotide sequences were obtained for C. ninhae and O. muntiacum, respectively. Due to the paucity of deposited cox-1 nucleotide sequences, which showed high identities to the newly obtained ones, no phylogenetic analyses were conducted.
Discussion
Alien species are organisms introduced intentionally or non-intentionally to non-endemic areas of the planet. Invasive species, successfully adapted to their new environments, are of current concern due to their impact on the ecological system, including biodiversity and landscapes, economy and health of related organisms (Atkinson, Reference Atkinson2001; Courchamp et al., Reference Courchamp, Chapuis and Pascal2003; Robertson et al., Reference Robertson, Adriaens, Lambin, Mill, Roy, Shuttleworth and Sutton-Croft2017). Representatives of invasive alien mammalian species in Japan include the common raccoon (Procyon lotor), Taiwan squirrel (Callosciurus erythraeus), Siberian chipmunk (Tamias sibiricus), hedgehog (Erinaceus spp.), brown rat (Rattus norvegicus), muskrat (Ondatra zibethicus), coypu (Myocastor coypus), American mink (Mustela vison), Taiwan macaque (Macaca cyclopis), etc. (Ohdachi et al., Reference Ohdachi, Ishibashi, Iwasa and Saitoh2010), most of which are invasive in Europe too (Robertson et al., Reference Robertson, Adriaens, Lambin, Mill, Roy, Shuttleworth and Sutton-Croft2017). All-year trapping of invasive alien mammals provides a better opportunity to find and characterize any parasite(s) with an unknown seasonal fluctuation in its prevalence (Sato & Suzuki, Reference Sato and Suzuki2006; Sato et al., Reference Sato, Suzuki, Osanai, Kamiya and Furuoka2006, Reference Sato, Torii, Une and Ooi2007).
Feral alien Reeves's muntjacs, with a recently expanding population on Izu-Oshima Island, Tokyo, were examined in the present study and three nematode species were detected in their alimentary tracts. In the oesophageal mucosa of the same host individuals, G. pulchrum was found in 11 (34.4%) of 32 examined animals. Details about this species are reported in a separate work (Setsuda et al., Reference Setsuda, Varcasia and Scale2018). Although little is known about the natural parasite fauna of Reeves's muntjacs or, indeed, muntjacs in general in their endemic areas, several valuable taxonomic works have been conducted on trichostrongylid or chabertiid nematodes (Dróżdż, Reference Dróżdż1967, Reference Dróżdż1973; Durette-Desset & Chabaud, Reference Durette-Desset and Chabaud1974; Gibbons & Kan, Reference Gibbons and Kan1987; Jian, Reference Jian1989). The trichostrongylid and chabertiid species from the feral alien Reeves's muntjacs on Izu-Oshima Island, Tokyo, were identified as C. ninhae and O. muntiacum, respectively. Some Oesophagostomum spp. (Oesophagostominae) and Chabertia spp. (Chabertiinae), both subfamilies classified in the family Chabertiidae, were not separated in distinct clades in our phylogenetic tree based on the ITS regions (fig. 5). In accordance, Newton et al. (Reference Newton, Chilton, Beveridge and Gasser1998) also noticed the same phylogenetic relationships among Oesophagostomum spp. and Chabertia ovina. This discrepancy between morphological taxonomy and phylogeny has not yet been explained.
Recent mammalogical studies have demonstrated a remarkable diverse speciation of muntjacs due to the recurrent fragmentation of endemic forests in South-East Asia and southern China (Schaller & Vrba, Reference Schaller and Vrba1996; Giao et al., Reference Giao, Tuoc, Dung, Wikramanayake, Amato, Arctander and MacKinnon1998; Wang & Lan, Reference Wang and Lan2000; Groves & Grubb, Reference Groves and Grubb2011; Li et al., Reference Li, Zhang, Swa, Maung and Quan2017). At present, we have no knowledge of the helminth fauna of the different Muntiacus spp.; however, they may harbour the trichostrongylid and chabertiid nematodes observed in the present study. Furthermore, it is possible they have additional species such as Spiculopteragia spp. (Trichostrongylidae: Ostertagiinae), Ashworthius tuyenquangi (Trichostrongylidae: Haemonchinae) and Trichuris muntjaci n. comb. (syn. Trichocephalus muntjaci Dróżdż, 1970) detected in M. muntjak in Vietnam (Dróżdż, Reference Dróżdż1965, Reference Dróżdż1970), or related species such as Hyostrongylus gabonensis (Trichostrongylidae: Graphidiinae), Oesophagostomum hyemoschi and Toxocara warreni (Ascarididae: Toxocarinae) identified in H. aquaticus in Gabon (Chabaud & Durette-Desset, Reference Chabaud and Durette-Desset1973; Durette-Desset & Chabaud, Reference Durette-Desset and Chabaud1974). Moreover, Ogmocotyle pygargi has also been reported from M. muntjak in Vietnam (Dróżdż, Reference Dróżdż1973). The rDNA and cox-1 nucleotide sequences of muntjac helminths determined here for the first time could help to clarify the relationships of parasite specimens with morphological variations and parasite populations from different Muntiacus spp. or host populations with different geographical distributions by allowing us to speculate on the relationships of the newly obtained specimens with archived ones and the possibility of co-evolution of these helminth species with their hosts.
Author ORCIDs
H. Sato, 0000-0002-5230-4677.
Acknowledgements
We are indebted to the Tokyo Metropolitan Oshima Island Branch Office for providing alien Reeves's muntjac samples and granting permission for them to be used for parasitological study. We also thank Professor Shuhei Tanaka, Faculty of Agriculture, Yamaguchi University, for his kind help with the SEM.
Financial support
This research received no specific grant from any funding agency, commercial or not-for-profit sectors.
Conflict of interest
None.
Ethical standards
The authors assert that all procedures contributing to this work comply with the ethical standards of the relevant national and institutional guides on the care and use of animals.