Introduction
Toxocara canis is the most common intestinal nematode in dogs. Mammals (including humans) and birds can be infected with eggs of the parasite, which deliver larvae that migrate through tissues without completely maturing. In humans, Toxocara larvae induce systemic manifestations known as larva migrans visceral syndrome. Larvae can be located in the eyes or the central nervous system, producing ocular larva migrans syndrome or eosinophilic meningoencephalitis syndrome, respectively (Kayes, Reference Kayes1997; Pawlowski, Reference Pawlowski2001; Despommier, Reference Despommier2003; Moreira-Silva et al., Reference Moreira-Silva, Rodrigues, Pimenta, Gomes, Freire and Pereira2004).
Studies of the migration of Toxocara canis larvae in paratenic hosts have been performed in mice (Burren, Reference Burren1968; Abo-Shehada & Herbert, Reference Abo-Shehada and Herbert1984; Abo-Shehada et al., Reference Abo-Shehada, Al-Zubaidy and Herbert1984; Parsons & Grieve, Reference Parsons and Grieve1990; Bardón et al., Reference Bardón, Cuéllar and Guillén1994; Epe et al., Reference Epe, Sabel, Schnieder and Stoye1994; Farjat et al., Reference Farjat, Minvielle, Pezzani and Niedfeld1995; Guardis et al., Reference Guardis, Radman, Burgos, Fonrouge and Archelli2002; Pecinali et al., Reference Pecinali, Gomes, Amendoeira, Bastos, Martins, Pegado, Bastos, Bozza and Castro-Faria-Neto2005; Camparoto et al., Reference Camparoto, Fulan, Colli, Paludo, Falavigna-Guilherme and Fernandez2008; Ollero et al., Reference Ollero, Fenoy, Cuéllar, Guillén and Del Aguila2008), rats (Lescano et al., Reference Lescano, Queiroz and Chieffi2004), non-human primates (Aljeboori et al., Reference Aljeboori, Stout and Ivey1970; Glickman & Summers, Reference Glickman and Summers1983), chickens (Galvin, Reference Galvin1964; Maruyama et al., Reference Maruyama, Nino, Yamamoto and Katsube1994; Taira et al., Reference Taira, Permin and Kapel2003a), pigs (Helwigh et al., Reference Helwigh, Lind and Nansen1999; Taira et al., Reference Taira, Saeed, Lind, Murrell and Kapel2003b; Sommerfelt et al., Reference Sommerfelt, Rosa, Duchene, Degregorio, López, Pisanú and De Torres2004) and sheep (Aldawek et al., Reference Aldawek, Levkut, Revajová, Kolodzieyski, Seveiková and Dubinský2002).
In the experimental models cited above, the authors described larvae migrating from the intestine to the liver. When eggs are introduced into the stomach, larval migration occurs mainly via a portal route within 12 h of infection by gavages. Over the next 72 h, larvae are detected in the liver and lungs. One week later, larvae are found in muscles and the central nervous system. Thereafter, larvae accumulate in nervous tissue, and their presence in other organs decreases. For this reason, some authors describe the first week of migration as visceral; thereafter, myotropic–neurotropic migration begins (Burren, Reference Burren1968; Abo-Shehada & Herbert, Reference Abo-Shehada and Herbert1984; Lescano et al., Reference Lescano, Queiroz and Chieffi2004; Cho et al., Reference Cho, Egami, Ohnuki, Saito, Chinone, Shichinohe, Suganuma and Akao2007; Alba-Hurtado et al., Reference Alba-Hurtado, Muñoz-Guzmán, Valdivia-Anda, Tórtora and Ortega-Pierres2009).
Gerbils have recently been used as experimental animals for toxocariasis, especially in studies of ocular lesions and lesions in the central nervous system (Takayanagi et al., Reference Takayanagi, Akao, Suzuki, Tomoda, Tsukidate and Fujita1999; Alba-Hurtado et al., Reference Alba-Hurtado, Tórtora, Tsutsumi and Ortega-Pierres2000; Akao et al., Reference Akao, Tomoda, Hayashi, Suzuki, Shimizu-Suganuma, Shichinohe and Fujita2003; Zanandréa et al., Reference Zanandréa, Oliveira, Abreu and Pereira2008). Little information is available concerning the migration of Toxocara larvae in gerbils (Cho et al., Reference Cho, Egami, Ohnuki, Saito, Chinone, Shichinohe, Suganuma and Akao2007; Alba-Hurtado et al., Reference Alba-Hurtado, Muñoz-Guzmán, Valdivia-Anda, Tórtora and Ortega-Pierres2009), especially during the first hours after infection. There are no reports concerning larval migration after re-infection with the parasite in gerbils. For these reasons, we investigated the migration of Toxocara larvae in gerbils after primary infection and re-infection. We used immunohistochemistry to identify larval antigens in tissue lesions.
Materials and methods
Recovery of Toxocara eggs
Toxocara canis eggs were obtained from adult females eliminated by puppies treated with piperazine (125–180 g/kg body weight). The eggs were maintained in a glass vial containing 0.1 n sulphuric acid. The glass vial was covered with gauze, protected from light and left at room temperature for 6 weeks. After the development of the third larval stage, which usually occurs 6 weeks after collection, the eggs were washed. The concentration of the suspension was adjusted to prepare inocula of 1500 eggs per animal. Inoculations were performed by gavage with appropriate metallic needles.
Experimental infections and larval recovery
Up to 35 gerbils received primary infections and three individuals per group were euthanized with pentobarbital anaesthesia or in a carbon dioxide chamber after 4, 8, 12, 24, 48, 72 and 96 h, and also 7, 15, 30 and 60 days post-infection (primary infected group). Another group of 24 gerbils was re-infected 4 weeks after primary infections and three individuals per group were euthanized 4, 8, 48, 72 h and 7, 15, 30, 60 days after re-infection (re-infected group).
Larval counting in the intestine was performed by taking segments of the organ, which were opened, washed and flattened between two 7.5 × 5 cm glass slides. The slides were sealed with tape and observed under an optical microscope. The liver was weighed, and fragments from different lobes were weighed and flattened between two 7.5 × 5 cm glass slides for larval counting (squashed fragments represented 30–40% of the total organ weight). The total number of larvae in the liver was obtained by correcting the number of larvae counted in the fragments according to the total weight of the organ. The remaining fragments were immediately fixed in neutral formalin. The encephalon was removed and separated into two hemispheres. One hemisphere was immediately fixed in buffered formalin. The other hemisphere was flattened between 7.5 × 5 cm slides for larval counting, which was performed as described for the liver. Because lung fragments were difficult to flatten, the left lung was digested in an acid pepsin solution with a pH of 1.1–1.2 (2.6 g pepsin 1:10,000 with biological activity, 5.0 g NaCl and 7.0 ml HCl in 493 ml of distilled water). After centrifugation (at 1000 rpm for 5 min), the larvae in the sediment were counted. The number of larvae in each organ was expressed as a percentage of the total number of larvae counted in each animal.
Histopathology and immunohistochemistry
Fragments of the liver, intestine, lung, heart, skeletal muscles, encephalon and eyes were fixed in 4% buffered formalin. After eyeball fixation, the lenses were removed. Each globe was sectioned in the frontal plane, and the two halves were examined under a stereoscopic microscope to search for larvae and lesions. All tissues were paraffin embedded. Sections were stained with haematoxylin and eosin and used for immunohistochemistry staining.
Immunohistochemistry for the detection of parasite antigens was performed in sections mounted on silanized slides using a polyclonal anti-Toxocara serum. The latter was obtained from rabbits after four inoculations with 5000 T. canis eggs at 2-week intervals (Smith et al., Reference Smith, Quinn, Bruce and Girdwood1982). One week after the last inoculation, blood was collected by cardiac puncture. The serum immunoglobulin was precipitated with ammonium sulphate. The precipitate was dialysed for 12 h, with three changes of phosphate-buffereds saline (PBS). Immunohistochemistry was performed using avidin–biotin–peroxidase (DAKO, Carpinteria, California, USA) according to the manufacturer's instructions. The primary antibody was used at a dilution of 1:300. No previous adsorption with antigens from other nematodes was performed using the rabbit serum. For this reason, cross-reactivity with other nematode larvae could not be ruled out.
Results
Primary infections
No gross changes were observed in the intestine. In liver and lungs, small, whitish nodules were observed in the organ surface during the second week after infection. Haemorrhagic areas were frequently seen in the lungs up to the seventh day after infection. There were no apparent macroscopic changes in the encephalon.
The number of larvae was greater in the intestine for the first 24 h, then larvae began to be frequent in the liver. After 72 h, larvae were frequently observed in the lungs and began to be found in the encephalon. After 1 week, the number of larvae increased in the encephalon and decreased in liver; however, larvae reappeared in the liver 1 week later. After 30 and 60 days, larvae were more frequently observed in the encephalon. Larvae could also be found in the liver at these time points (fig. 1).

Fig. 1 The proportion (%) of Toxocara canis larvae recovered from primary infections in gerbils up to (A) 96 h and (B) 60 days post-infection; jejunum (closed circle), ileum (open circle), caecum (closed triangle), liver (open triangle), lung (closed diamond) and encephalon (closed square).
In the flattened liver and encephalon fragments, mobile larvae were easily observed under the microscope. Larvae frequently appeared without surrounding inflammatory cells in the intestinal wall, crypts and lymphatic vessels, the liver, skeletal muscles and in the encephalon until the second week after infection (fig. 2a). The focal necrosis of neurons, central chromatolysis and foci of glial proliferation were observed in the cerebellum at 7 days post-infection. Focal eosinophilic infiltrates were progressively more frequent 7 days post-infection in the liver, lungs, kidneys and heart (fig. 2b). Granulomatous reactions appeared after the first week, initially as loose macrophage and eosinophil accumulations correlating with larval antigens or larval fragments (fig. 2c). At approximately 30 days post-infection, the granulomata were more organized; peripheral fibrosis was observed in the liver, lungs, skeletal muscles and kidneys (fig. 2d). Focal areas of haemorrhaging were frequent in the lungs. Immunohistochemistry showed the presence of parasite antigens in areas of inflammatory infiltrates, in the vicinity of larvae and within macrophages making up granulomas in all organs.
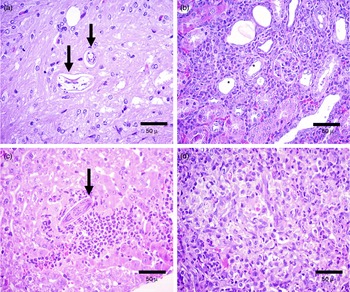
Fig. 2 Histopathological changes to the internal tissues of gerbils on day 15 post-infection with Toxocara canis, to show (a) the brain with intact larvae (arrows) without any inflammatory reaction; (b) the kidney with eosinophilic infiltrates between the tubules (*); (c) the liver with granulomata and eosinophils and macrophages adjacent to a larva (arrow) and (d) on day 60 post-infection, lung tissue with granulomata and large numbers of eosinophils.
Between 7 and 30 days post-infection, focal areas of haemorrhaging were observed in the plexiform layer of the retina and vitreous humour of the eyes. Limited eosinophil infiltration at the base of the optic nerve, and focal areas of scleritis and episcleritis with eosinophils, were frequently observed. Focal areas of myositis with eosinophils were observed in eye muscles. These lesions were infrequent at 30 days post-infection.
Secondary infections
Manifestations of injury in motor areas, such as walking in a circle, paresis and paralysis of hind limbs, were commonly observed in re-infected animals at 30 days after re-infection.
Small nodules on the surface of the liver and lungs were more frequent and appeared earlier after re-infection than in primary infected animals. Larval distribution in the re-infected group was similar to that observed in the primary infected group. However, the number of larvae increased in the liver and was reduced in the brain at 15 days after re-infection (fig. 3). Microscopic examination of liver fragments mounted between slides revealed the presence of organized granulomata around larvae. These larvae were mobile and sometimes observed leaving the granulomata.

Fig. 3 The proportion (%) of Toxocara canis larvae recovered in gerbils re-infected 30 days following a primary infection up to (A) 72 h and (B) 60 days post-infection; jejunum (closed circle), ileum (open circle), caecum (closed triangle), liver (open triangle) and encephalon (closed square).
The inflammatory reaction was more severe, with higher number of eosinophils, in all organs compared to those in the primary infected group. Necrosis of Purkinje cells and foci of demyelization with microglia aggregates were observed in the cerebellum. Larvae were observed in the vicinity of the lesions. Focal eosinophilic meningitis was observed in all animals at different times after re-infection. Granulomata were observed in the brain and cerebellum at 15 days after re-infection, some with areas of central necrosis and calcification (fig. 4a). Although loose granulomas were frequently found during the first week after re-infection, all of the granulomata observed after the second week were typical epithelioid granulomas with frequent peripheral fibrosis. Larvae or larval fragments were observed within some granulomata (fig. 4b, c). In the eyes, lesions were similar to those observed in the primary infected group. Immunohistochemistry confirmed the presence of parasite antigens in areas of eosinophilic inflammatory infiltrates, in granulomata and in the vicinity of intact larvae (fig. 4d).

Fig. 4 Histopathological changes to the internal tissues of gerbils re-infected at 30 days following a primary infection with Toxocara canis, to show (a) the encephalon 15 days after re-infection, with microglial granuloma (arrow) and central necrosis; typical epitheliod granuloma with peripheral fibrosis and larva on day 30 after re-infection in (b) liver and (c) kidney; and (d) immunohistochemistry in encephalon at 30 days after re-infection, with larval antigens within microglia.
Discussion
The results in the primary infected group demonstrated that, in gerbils, penetration of the wall of the small intestine by Toxocara larvae was completed in 24 h, confirming reports from other studies (Burren, Reference Burren1968; Abo-Shehada et al., Reference Abo-Shehada, Al-Zubaidy and Herbert1984; Parsons & Grieve, Reference Parsons and Grieve1990; Cho et al., Reference Cho, Egami, Ohnuki, Saito, Chinone, Shichinohe, Suganuma and Akao2007; Alba-Hurtado et al., Reference Alba-Hurtado, Muñoz-Guzmán, Valdivia-Anda, Tórtora and Ortega-Pierres2009).
The presence of larvae within lymphatic vessels in intestinal mucosa at 4 h post-infection is an indirect indication that this route can be used to reach the systemic circulation, as proposed in mice by Abo-Shehada et al. (Reference Abo-Shehada, Al-Zubaidy and Herbert1984). It is interesting to note that there were essentially no inflammatory lesions around larvae in the intestinal walls of infected animals.
Larvae were quickly observed in the liver, where they were numerous, for up to 96 h post-infection. The number of larvae decreased over subsequent days and they were rarely observed in the livers of animals sacrificed at 7 days post-infection. Interestingly, the number of larvae in the liver increased 1 week later and progressively decreased until 60 days post-infection. A reduction in the larval load in the liver starting from the fifth day of infection was previously reported in gerbils (Parsons & Grieve, Reference Parsons and Grieve1990; Cho et al., Reference Cho, Egami, Ohnuki, Saito, Chinone, Shichinohe, Suganuma and Akao2007; Alba-Hurtado et al., Reference Alba-Hurtado, Muñoz-Guzmán, Valdivia-Anda, Tórtora and Ortega-Pierres2009); however, a subsequent increase in the larval load was not mentioned in any of these reports. This fluctuation in larval load has been reported in mice; a reduction was observed after 48 h, and an increase in larval load was observed at 21 days post-infection (Farjat et al., Reference Farjat, Minvielle, Pezzani and Niedfeld1995). This fluctuation in larval load in the liver may be due to a long migration via the lymphatic system, as larvae would have to go through the lymph nodes before reaching the circulation.
The pattern of larval distribution after infection observed in the lungs and brain was similar to that described by other authors in gerbils (Cho et al., Reference Cho, Egami, Ohnuki, Saito, Chinone, Shichinohe, Suganuma and Akao2007; Alba-Hurtado et al., Reference Alba-Hurtado, Muñoz-Guzmán, Valdivia-Anda, Tórtora and Ortega-Pierres2009) and in mice (Burren, Reference Burren1968; Abo-Shehada & Herbert, Reference Abo-Shehada and Herbert1984; Guardis et al., Reference Guardis, Radman, Burgos, Fonrouge and Archelli2002; Pecinali et al., Reference Pecinali, Gomes, Amendoeira, Bastos, Martins, Pegado, Bastos, Bozza and Castro-Faria-Neto2005). The observed pulmonary lesions, especially the haemorrhagic lesions, were similar to those described in mice (Mossalam et al., Reference Mossalam, Atallah and Hosney1972; Zyngier, Reference Zyngier1974). In the encephalon, larvae were found starting at 72 h post-infection, and were more abundant after 1 week, confirming previous reports in gerbils (Cho et al., Reference Cho, Egami, Ohnuki, Saito, Chinone, Shichinohe, Suganuma and Akao2007; Alba-Hurtado et al., Reference Alba-Hurtado, Muñoz-Guzmán, Valdivia-Anda, Tórtora and Ortega-Pierres2009) and mice (Burren, Reference Burren1968; Abo-Shehada & Herbert, Reference Abo-Shehada and Herbert1984; Guardis et al., Reference Guardis, Radman, Burgos, Fonrouge and Archelli2002).
The histological picture observed in the liver was similar to that observed in mice examined at the same time of infection (Zyngier, Reference Zyngier1974). Focal inflammatory lesions and poorly organized granulomata were observed in the liver. These were frequently not associated with larvae, which suggested that the larvae move rapidly and that inflammatory reactions occur in larval tracks. In fact, the granulomata observed in sections of liver mounted between microscope slides showed no larvae or had a larva moving into them. It was sometimes possible to observe larvae leaving the granulomata. Serpiginous granulomata were observed in the liver, suggesting that inflammatory reactions occur in larval tracks in response to antigens being liberated by migrating larva. The presence of loose granulomata is an indirect indication that the cellular immune response to larval antigens is slower and weaker in gerbils. Typical epithelioid granulomata around larvae have been described more frequently in the livers of rats, chickens and guinea pigs at 10 days post-infection (Mossalam et al., Reference Mossalam, Atallah and Hosney1972); these findings likely reflect more effective adaptive immune responses against larval antigens in these species.
Regarding histological changes observed in the brain, inflammatory reactions were small or absent around the larvae during primary infection; these findings support those previously described in mice and gerbils (Mossalam et al., Reference Mossalam, Atallah and Hosney1972; Akao et al., Reference Akao, Tomoda, Hayashi, Suzuki, Shimizu-Suganuma, Shichinohe and Fujita2003). Focal necrosis of neurons in the cerebellum was especially frequent. Cerebellar lesions without inflammatory reactions were also reported in gerbils by Akao et al. (Reference Akao, Tomoda, Hayashi, Suzuki, Shimizu-Suganuma, Shichinohe and Fujita2003). However, such lesions have not been reported in other rodents used as models of T. canis infection.
The lesions observed in kidneys and skeletal muscles were similar to those described in mice (Mossalam et al., Reference Mossalam, Atallah and Hosney1972; Kayes & Oaks, Reference Kayes and Oaks1978).
Contrary to what has been reported previously in gerbils, we did not observe severe lesions in the eyes after primary infection; larvae were rarely observed. We do not have a complete explanation for this observed discrepancy. It is likely due to the age of the gerbils, which were 45 and 60 days old, or the amount of inoculum used; inocula with a low number of larvae would be less effective in inducing immune responses in young animals, favouring the migration of larvae (Glickman & Schantz, Reference Glickman and Schantz1981; Zanandréa et al., Reference Zanandréa, Oliveira, Abreu and Pereira2008).
Immunohistochemistry with polyclonal anti-Toxocara serum demonstrated that the inflammatory infiltrates and granulomata observed in all organs were correlated with larvae or larval antigens. In infected humans, Toxocara granulomata were strongly stained with a polyclonal anti-Toxocara serum; stained Toxocara antigen was observed within macrophages in these granulomas (Musso et al., Reference Musso, Castelo, Tsanaclis and Pereira2007). The inflammatory cells of experimentally infected sheep, especially macrophages in the intestine, liver and lungs, were stained with anti-Toxocara serum raised in mice (Aldawek et al., Reference Aldawek, Levkut, Revajová, Kolodzieyski, Seveiková and Dubinský2002)
In re-infected animals, a dramatic change in larval migration was observed after the first 72 h. This difference was observed in the redistribution of larvae, which remained numerous in the liver 60 days after secondary infection, with a peak in abundance at 48 h. This observation was similar to that reported in re-infected mice (Zyngier, Reference Zyngier1974; Parsons & Grieve, Reference Parsons and Grieve1990; Wnukowska & Dzbenski, Reference Wnukowska and Dzbenski2008). Our findings demonstrate that larvae are retained in the liver for a longer time than in primary infected animals. Histological studies in mice also showed that epithelioid granulomata are larger after re-infection and surrounded by fibrous capsules (Zyngier, Reference Zyngier1974; Wnukowska & Dzbenski, Reference Wnukowska and Dzbenski2008). Upon re-infection, granulomata were present in liver fragments flattened between two slides. Larvae were observed moving inside these granulomata, suggesting that the larvae leave the granulomata and continue their migration. In fact, dead larvae were infrequent in the granulomas. The granulomatous reaction around larvae in the liver would explain their retention, as it would delay larval migration to other sites.
Granulomas with larvae, which were sometimes dead, were found more frequently in the encephalon and cerebellum of the secondary infected group than in those of the primary infected group. This is similar to what was observed in the liver. These granulomata are responsible for the more severe neurological symptoms observed in re-infected animals.
In all tissues, the granulomata around larvae or larval antigens were larger than those observed in the primary infected group. Although an evaluation of the immune response to Toxocara antigens has not been performed, the presence of large epithelioid granulomata in re-infected animals suggests a strong adaptive immune response against Toxocara antigens in this group. In fact, athymic nude mice infected with T. canis develop fewer and looser granulomata than control mice infected with the same larval load. This finding confirms the role of the cell-mediated immune response in the development of granulomas around Toxocara larvae or larval antigens (Sugane & Oshima, Reference Sugane and Oshima1983; Wnukowska & Dzbenski, Reference Wnukowska and Dzbenski2008).
In conclusion, our results demonstrated that larval migration during primary infection in gerbils is similar to that observed in mice. This migration has a visceral initial phase, in which larvae predominate in the liver and lungs. During the later neurotropic phase, larvae concentrate in the central nervous system. After re-infection, the visceral phase is amplified, and a great number of larvae are retained in the liver. Inflammatory lesions, especially granulomata, are more intense after re-infection. This finding suggests that the cellular immune response against larval antigens was greater than that observed during primary infection. Despite this intense granulomatous inflammatory reaction, the destruction of larvae within these granulomata was not frequently observed.
Financial support
This research was supported by intramural funding from the Infectious Diseases Unit at the Health Sciences Center, Federal University of Espirito Santo.
Conflict of interest
None.
Ethical standards
The authors assert that all procedures contributing to this work comply with the ethical standards of the relevant national and institutional guides for the care and use of laboratory animals. Experiments were approved by the Ethics Committee for the Use of Animals at the Federal University of Espirito Santo (n. 007/2008).