Introduction
The increasing use of assisted reproductive technologies (ART) in humans has raised some concerns regarding its impact on the short- and long-term health of the offspring. Reference El Hajj and Haaf1,Reference Hargreave, Jensen, Hansen and Dehlendorff2 Several studies show that stressful conditions, derived from gametes and embryo manipulation during the first week of preimplantation development, are correlated with differences in the natural epigenetic reprogramming that occurs during this period. Reference Canovas, Ivanova and Romar3–Reference Menelaou, Prater and Tunster5 From the ovarian hyperstimulation treatments to any other ART like ovum pick-up (OPU), AI, or embryo transfer (ET), each step in ART represents a possible alteration in the epigenome with putative consequences in the transcriptome and physiology of the offspring. Reference Van Montfoort, Hanssen, De Sutter, Viville, Geraedts and De Boer6–Reference Hattori, Hiura and Kitamura8 A correlation has been observed between the use of these techniques for infertility treatments and the birth weight and growth parameters, which could trigger an increased risk of cardiovascular diseases, diabetes, and obesity in adulthood, Reference Feuer and Rinaudo9 when compared to naturally conceived offspring. Unfortunately, in humans, investigating the impact of ART on long-term phenotypical traits is still a challenge since the first ART-derived offspring generation is still in their middle forties. Reference Ferraretti, Nygren and Andersen10 Therefore, animal models with a shorter life expectancy can allow the evaluation of the isolated impact of one specific ART on their mid and long-term phenotypical traits, such as metabolism, cardiovascular health, reproductive capacity, as well as their possible genetic and epigenetic disorders.
The pig represents an excellent model for human studies Reference Van, Wydooghe, Heras and Vandaele11 due to its similar anatomical, physiological and genetic characteristics. Reference Bellinger, Merricks and Nichols12,Reference Lorenzen, Follmann, Jungersen and Agerholm13 Despite the anatomical differences between the sow and woman reproductive system, the pig is considered an adequate model for ART studies. Reference Lorenzen, Follmann, Jungersen and Agerholm13 On the one hand, the genetic variability can be avoided by using the highly selected male and female breeders, available in commercial farms and AI centers. On the other hand, the health and fertility of these animals have been already proven, which can avert the bias existing in humans derived from parental reproductive pathologies or infertility. Finally, pigs allow us to study and understand, in an acceptable time, the long-term effects derived from specific techniques, since the life expectancy of the swine species (from 12 to 18 years old) is much shorter than humans.
By contrast, some ART such as ET, Reference Cameron, Beebe, Blackshaw and Keates14 IVF, or embryo culture (EC) still show poor performance in the pig, making it difficult for the offspring obtaining. This fact implies that studies on live pigs assessing the potential impact of these techniques on the health, growth rate, productive indexes, or reproductive capacity of the offspring, compared to those naturally produced, are almost inexistent. One of the latest strategies accomplished to improve the results is the supplementation of the media, used during IVF and EC, with reproductive fluids (RF), which are reproductive secretions that are physiologically present in the female reproductive tract. The use of RF as additives includes hormones, growth factors, enzymes, as well as a large number of proteins, playing an important role not only during gamete maturation but also during fertilization and embryonic development. Reference Avilés, Gutiérrez-Adán and Coy15 Cánovas et al. Reference Canovas, Ivanova and Romar3 showed that embryos produced with these additives were more similar to those produced in vivo in terms of embryo development, gene expression, and epigenetic pattern. In addition, the feasibility of this in vitro embryo production system was proven in terms of percentages of pregnancies, farrowing rate, litter size, or birth weight, Reference París-Oller, Navarro-Serna and Soriano-Úbeda16 remaining the health of the offspring after birth to be assessed.
Physiological parameters, such as the hematological profile, are important indicators that provide information about the general health status of the animals. In newborn piglets and young pigs, a few studies have published reference intervals that can be used for consistent comparisons. Reference Casas-Díaz, Closa-Sebastià, Marco, Lavín, Bach-Raich and Cuenca17–Reference Ventrella, Dondi and Barone19
On the other hand, investigating the carbohydrates’ metabolism by performing the oral glucose tolerance test (OGTT) is a useful tool, widely established in young people, for the study of type 2 diabetes as well as its progress over time. Reference Chen, Aguirre and Hannon20 Moreover, Manel et al., Reference Manell, Hedenqvist, Svensson and Jensen-waern21 established a refined OGTT, using the pig as a model, comparable to that used in children and adolescents.
Therefore, using the pig as a model, this work was conducted to compare the growth, hematological profile, and metabolism (glucose tolerance) until month 6 of age in in vitro-derived (with or without RF added to culture media) and artificial insemination-derived piglets. Taking into consideration all the above-mentioned issues, we hypothesized that the inclusion of RF in the culture media during the in vitro production of embryos could have a beneficial impact on the phenotype of the offspring. If our hypothesis is confirmed, the use of RF could be a potential future additive to improve the health of ART-derived human offspring too.
Methods
Unless otherwise indicated, all chemicals and reagents were purchased from Sigma-Aldrich Chemical S.A. (Madrid, Spain). Porcine follicular fluid (FF), oviductal fluid (OF), and uterine fluid (UF) were provided by EmbryoCloud (NaturARTs®, Murcia, Spain).
Ethics
The experimental work performed in this study was submitted to evaluation by the CEEA (Comité Ético de experimentación Animal) from University of Murcia. After approval, authorization from “Dirección General de Agricultura, Ganadería, Pesca y Acuicultura” – Región de Murcia- nr A13170706 was given to perform the experiments with animals.
Animals
Experiments were performed in collaboration with a commercial farm (Cefu S.A., Murcia, Spain). Three groups of animals were differentiated according to the ART of origin. Each group consisted of four litters from crossbred sows (Landrace × Large White) with the same genetic line. The initial number of live piglets used in the study was 105 (58 females and 47 males):
AI group (n = 58): Piglets were obtained by AI. This was considered the control group.
C-IVP group (n = 29): Piglets were obtained from females that became pregnant after the transfer of embryos produced in vitro using culture media with bovine serum albumine (BSA) as the major protein source.
RF-IVP group (n = 18): Piglets were obtained from females that had become pregnant after the transfer of embryos produced in vitro using RF from the different phases of the estrous cycle as supplements in both IVF and EC media.
Briefly, once the embryos were produced, the ET procedure was performed surgically by a paralumbar laparo-endoscopy single site (LESS) assisted approach with a monoport device (GelPOINT® Advanced Access Platform, Applied Medical, Rancho Santa Margarita, CA, USA). Then, when the end of the uterine horn was located and exteriorized, it was punctured allowing, through a small opening, the introduction of an ET catheter (Emtrac Delphin catheter, Gynetics® Lommel, Belgium), and the embryos were deposited into the uterine lumen.
The entire process by which the embryos were produced and subsequently transferred through minimally invasive surgery, as well as the data collected at birth (farrowing rate, survival rate, litter size, and placental parameters), are described in more detail in a previously published article. Reference París-Oller, Navarro-Serna and Soriano-Úbeda16
The study was performed from day 0 to 180 post-birth. However, the final number of animals included in the study was 75 (42 females and 33 males) since a total of 13 were excluded from the AI group at birth to homogenize the number of piglets by group and 17 were due to the causalities suffered during the period of the study in all groups.
During this experiment, the animals had ad libitum access to food and water. Depending on their age, the animals were located in different units but the same facilities of the farm. From birth to day 28 the piglets remained in the parturition unit (until the weaning period). After weaning, the animals were relocated to the transition unit. On day 84 of age, the piglets were transferred to the fodder unit, remaining in this place until the end of the study.
Growth parameters
Bodyweight (BW, kg), average daily weight gain (ADWG, kg) and Crown-rump length (CRL, cm) were checked at different days of life: 0, 3, 9, 15, 30, 45, 60, 75, 90, 105, 120, 135, 150, 165 and 180.
Bodyweight (BW) and average daily weight gain (ADWG)
The BW was obtained with two different scales depending on the age. From day 0 to 45 of age, the piglets were weighed using a digital hanging scale tying a rope to the animal back end behind the hock. From day 60 onwards, all animals were weighed on a mobile scale (Livestock scale GE-4, Anapesing).
In addition, ADWG was calculated by the following formula:

Crown-rump length
An additional growth parameter named CRL was checked with a tape measure. The CRL parameter refers to the distance between the frontal region of the skull and the rump, at the birth of the tail.
Blood collection
Blood samples were collected by direct venipuncture of the jugular vein with a Vacutainer® system using a 20G × 25 mm needle and BD Vacutainer® lithium heparin tubes. Blood tubes were transported to the laboratory and hematological analysis was performed using a hematology analyzer (Siemens ADVIA® 120, USA). The parameters analysed were concentration of erythrocytes (RBC, ×106 cells/µL), hemoglobin (HB, g/dl), hematocrit (HCT, %), mean corpuscular volume (MCV, fL), mean corpuscular hemoglobin (MCH, g/dL), mean cell hemoglobin concentration (MCHC, g/dl), hemoglobin concentration distribution width (HDW, g/dl), cell hemoglobin concentration mean (CHCM, g/dl), Red blood cells distribution width (RDW, %), reticulocytes indices [percentage of reticulocytes (%), mean corpuscular volume of reticulocytes (MCVr, fL) and reticulocyte-hemoglobin content (CHr, pg)], concentration of white blood cells (WBC, ×103 cells/µL), neutrophils (×103 cells/µL), lymphocytes (×103 ells/µL), monocytes (×103 cells/µL), eosinophils (×103 cells/µL), basophils (×103 cells/µL), concentration of platelets (PLT, ×103 ells/µL) and platelets indices [platelecrit (PCT, %), mean platelet volume (MPV, fL), platelet distribution width (PDW, %), platelet component distribution width (PCDW, g/dl), mean platelet mass (MPM, pg), platelet mass distribution width (PMDW, pg) and large platelets (Large PLT, ×103 cells/µL)].
Oral glucose tolerance test (OGTT)
OGTT was performed at day 45 of age from four litters of relative animals per group. To do this, when possible, the two males and two females of highest and lowest weight per litter were selected for the study. A total of 14 animals (Males = 9; Females = 5) belonged to RF-IVP group, 15 (Males = 7; Females = 8) belonged to C-IVP group and 16 (Males = 8; Females = 8) belonged to AI group.
After an 18 h overnight fast, water was withdrawn and, 1 h later, all selected piglets ingested 1.75 g/kg BW of glucose solution (100% Glucose carbs, Myprotein). Then, blood samples were collected from the auricular lateral vein before (baseline level) and 5, 10, 15, 20, 30, 45, 60, 90, 120, and 150 min after glucose intake. Blood glucose concentration (BGC) was immediately measured by a glucometer (GlucoMenLX Plus+) using test strips (Image 1).
Statistical analysis
All growth parameters were analyzed using the R studio software version 3.4.4 (R core Team 2018). A robust mixed analysis of variance (ANOVA) was used to find differences for BW and ADWG data while a mixed ANOVA was used for CRL since the Mauchly’s test for sphericity was significant (p < 0.05), but the corrections Greenhouse-Geisser (GGe) and Huynh Feldt (HFe) were not valid for the first two.
BW and ADWG data were analyzed by sex and group following a robust mixed ANOVA since Mauchly’s test for sphericity was significant (p < 0.05) for both cases, but the GGe and HFe corrections were invalid.
The differences for CRL were also investigated through a robust mixed ANOVA in the case of males, while in the case of females it was used a mixed ANOVA with GGe correction.
In addition, to know if there was a correlation between the litter size and the BW of the individuals at days 0, 30, 75, 120, and 180, a two-way ANOVA was performed for these days in the case of males. In females, a two-way ANOVA was performed for days 0, 120, and 180, and a Welch–James test for days 30 and 75, since there was normality but no homoscedasticity. In all the parameters significant differences were considered those that obtained probability levels of p < 0.05. Growth parameters are presented as mean ± SD (standard deviation). Values of p < 0.05 were considered significant.
The litter size and all blood parameter data analyses were performed using GraphPad Software, version 7, La Jolla California USA. D´Agostino & Pearson normality test was performed to assess the normality of the data. One-way ANOVA was used when data were normally distributed and Kruskal–Wallis test when data did not follow a normal distribution. Dunn´s multiple comparison test was used to assess differences between groups. Hematological results are presented as mean ± SD. Values of p < 0.05 were considered significant.
To study the OGTT results, the software used was IBM SPSS Statistics (v22.0). One-way ANOVA was performed to analyze the differences between data. Tukey post-hoc tests were applied when ANOVA showed significant differences (p < 0.05). Then, the Pearson correlation coefficient was used to detect litter influence. The results derived from the OGTT are presented as mean ± SEM (standard error of the mean). Also, the area under the curve (AUC) analysis was used to identify differences (p < 0.05) between groups for OGTT.
Results
Growth parameters from day 0 to day 180 of age
The results of growth parameters at the different days and, split by sex, are shown in Supplementary Tables S1, S3, S5, for males and S2, S4, S6 for females.
Bodyweight (BW)
Males
When data were analyzed at each time point of animal age, it was observed (Fig. 1a and Supplementary Table S1) that piglets from the C-IVP group were heavier (p < 0.05) than their AI counterparts during the first days of life. C-IVP piglets were also heavier than RF-IVP ones at days 9, 15, and 45. In further estimations (from day 60 onwards), the two in vitro groups (C-IVP and RF-IVP) tended to be statistically similar between them and heavier (p < 0.05) than pigs from the AI group. Considering that litter size previously reported by our group (París-Oller et al., 2020) showed significant differences between the RF-IVP and AI groups (data from París-Oller et al., 2020: RF-IVP 4.50 ± 1.91a (n = 18); AI: 14.5 ± 6.40b (n = 58), p < 0.05), the correlation between litter size and weight was analyzed. No correlation was found between these two parameters (litter size and BW) on any of the days studied (0, 30, 75, 120, and 180).
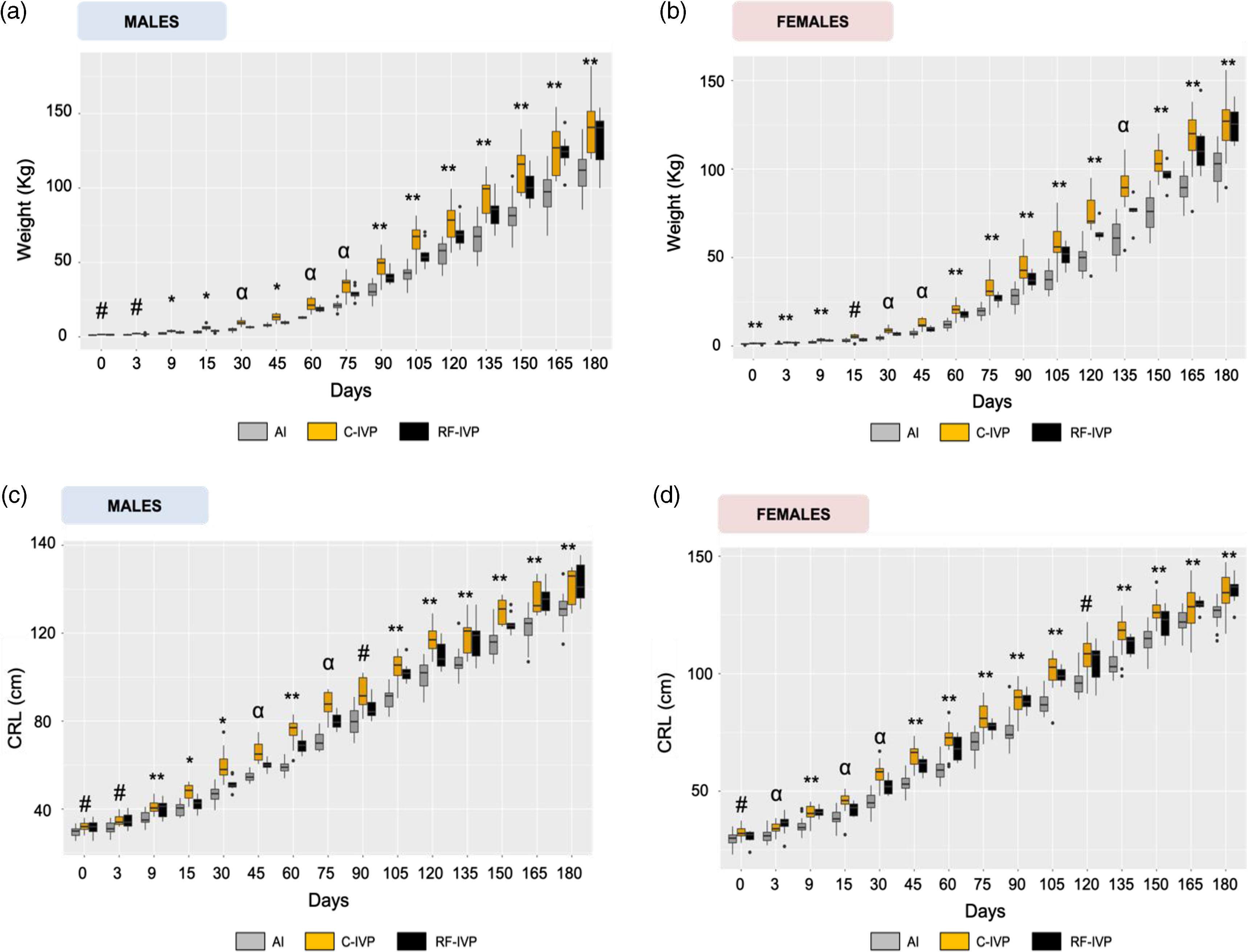
Fig. 1. Growth parameters from day 0 to day 180 of age.
Females
When data was analyzed in females at each time point of animal age (Fig. 1b and Supplementary Table S2), it can be observed that the heaviest group at birth (C-IVP) remained heavier for almost the whole experiment, except for day 180. On that day, the average weight of C-IVP animals was overtaken by the weight of the RF-IVP group. Meanwhile, the BW of the AI group always remained below the other two experimental groups.
The weight of females during the study followed the same pattern as males, where both IVP groups reached similar mean values by 180 days with AI pigs having a lower weight. Furthermore, no correlation was found between litter size and BW at days 0, 75, 120, and 180, while it was found on day 30.
Average daily weight gain (ADWG)
Males
Analyzing the data from each of the three groups by day of measurement, values of ADWG showed some differences (Supplementary Table S3). On day 3, ADWG was higher in C-IVP than in AI and RF-IVP animals (p < 0.05). These differences remained until day 15. On day 30, C-IVP was higher than AI and RF-IVP was similar to both C-IVP and AI. From that point on and through the 6 months of study, the general tendency was the same: C-IVP and AI groups showed differences between them (p < 0.05) at many of the time points apart from those mentioned (60, 105, and 135 days) showing C-IVP higher values of ADWG than AI. R-IVF tended to get values between C-IVP and AI and were statistically similar to them (p > 0.05).
Females
Similar to males, when ADWG was studied in females, some differences were evidenced in the analyses at specific time points (Supplementary Table S4). Animals from the AI group showed a lower ADWG than animals from the C-IVP and RF-IVP groups at day 3 (p < 0.05). On days 9, 15, 45, 75, and 90 these differences only were found between AI and C-IVP animals, being RF-IVP animals similar to AI and different from C-IVP (p < 0.05). However, from day 105 onwards, the three groups showed similar ADWG.
Crown-Rump Length (CRL)
Males
Results of CRL in males for all the groups and days are represented in Fig. 1c and Supplementary Table S5. Animals from the AI group showed the shortest CRL than those from the C-IVP and RF-IVP groups on days 9, 60, 120, 135, 150, 165, and 180 of life (p < 0.05). On day 3 and from day 90 to 105, the shortest CRL was found between the AI and C-IVP groups (p < 0.05), while on day 30, that difference was found between C-IVP and RF-IVP (p < 0.05). Finally, on days 45 and 75 the three experimental groups were different from each other (p < 0.05).
Females
Results of CRL in females are represented in Fig. 1d and Supplementary Table S6. On days 3, 15, and 30 of life, the three groups were different from each other. However, on day 9 and from day 45 to 180, animals from the AI group showed shorter CRL than those from C-IVP and RF-IVP. In this last-mentioned interval (day 45–180), the CRL of RF-IVP remained, on most of the evaluated periods, in intermediate values between AI and C-IVP group. However, in the last two measurements (days 165 and 180) a greater length for RF-IVP than for C-IVP animals was evidenced.
Hematological parameters
The blood hematological parameters were analyzed along the 180 days of age in all the animals together, including both sexes (Supplementary Tables S7a–c). When significant differences were found in a blood parameter, males and females were separately studied (Supplementary Tables S8a–c and S9a–c respectively). Only those differences maintained for a certain time are described below.
Red blood cells parameters
Red blood cells count (RBC)
RBC in males showed significant differences on days 30, 60, and 90 of life (Fig. 2a) where C-IVP showed higher RBC than the other two groups, except on day 60.
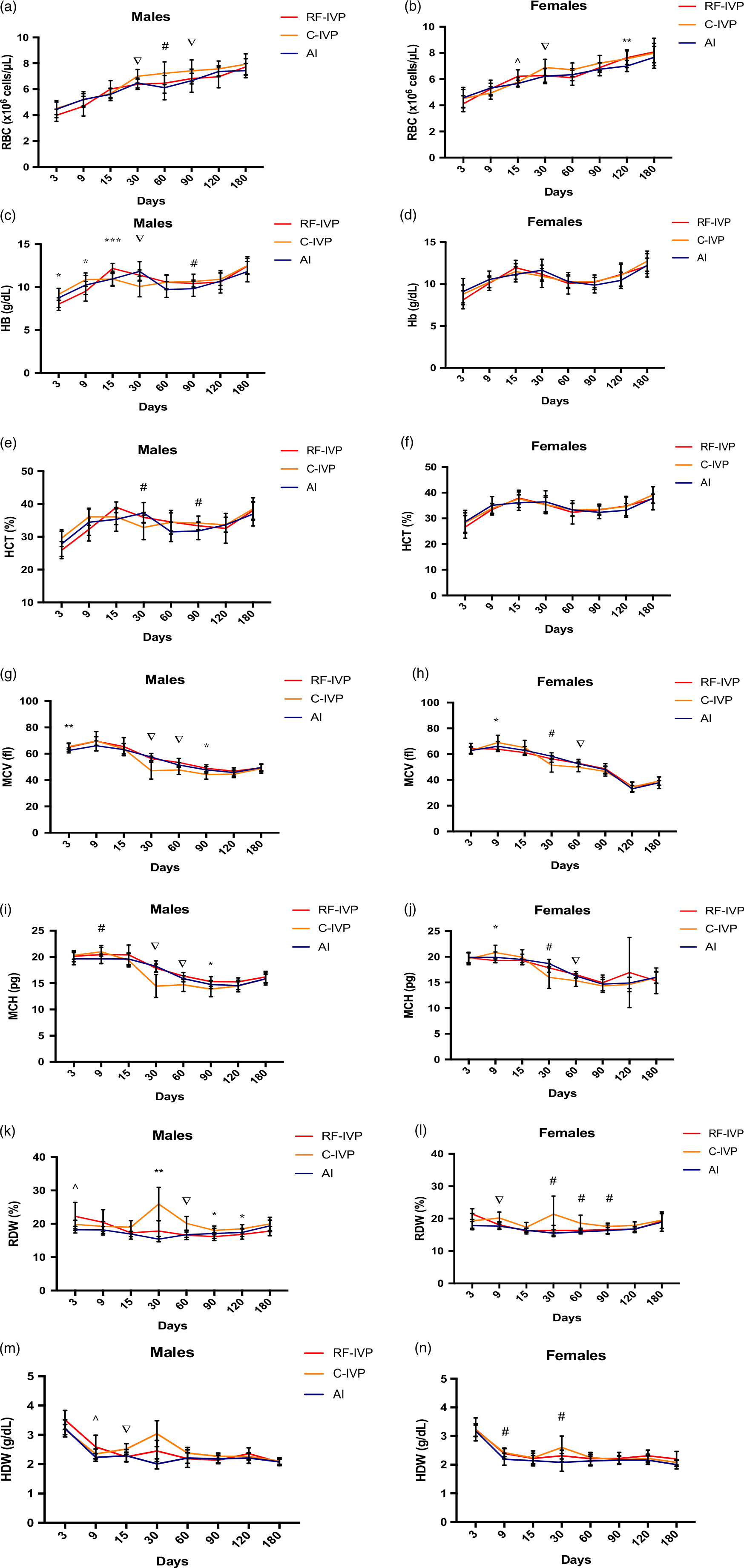
Fig. 2. Red blood cells parameters.
Females, instead, showed higher values of RBC for RF-IVP than for AI at day 15 (Fig. 2b and Supplementary Table S9a). In addition, as described for males, on day 30, higher RBC values were found in C-IVP than in the other two groups. Subsequently, only on day 120, significant differences were observed between the AI group and the other two groups.
Hemoglobin (Hb)
As it can be observed in Fig. 2c, the Hb concentration in males was significantly higher in the C-IVP group on days 3 and 9 than in RF-IVP. It was also observed a Hb peak at day 15 in RF-IVP, which showed higher Hb values compared to C-IVP and AI. On day 30, another Hb peak was observed in the C-IVP group, which showed the highest Hb value. On day 90, the males from C-IVP showed a higher Hb value than males from AI. A similar pattern in Hb concentration along the time was observed when females were analyzed (Fig. 2d), although no significant differences for any group or day of analysis were found
Hematocrit (HCT)
Figure 2e shows the results of HTC in males. RF-IVP showed a peak in males at day 15, with higher values than AI males. From that point, HCT values seemed to decrease, being higher in C-IVP at day 30. On the other hand, on day 90, the HCT for C-IVP males was lower than for AI males.
No differences in HCT values were found throughout the study for females (Fig. 2f).
Mean corpuscular volume (MCV)
MCV in males was lower for the AI group on day 3 regarding the other two experimental groups (Fig. 2g). However, on days 30 and 60, values in the C-IVP group were lower compared with the other two groups. On day 90, these differences were maintained but only between the C-IVP and RF-IVP groups.
In C-IVP females, MCV showed higher values on day 9, compared with RF-IVP animals (Fig. 2h). Similar to males, at day 30, females from the C-IVP group showed lower MCV values, but only regarding AI. However, at day 60, the same differences found in males between C-IVP, and the other two groups were also observed when females were analyzed.
Mean corpuscular hemoglobin (MCH)
Males from the C-IVP group showed higher MCH values than those from the AI group on day 9. At days 30 and 60, values for the C-IVP group decreased significantly compared to the other two groups (Fig. 2i). However, on day 90 these differences only remained between C-IVP and R-IVP groups. On the other hand, females showed differences for MCH between C-IVP and RF-IVP at day 9, while on day 30, differences were observed between C-IVP and AI (Fig. 2j). Similar to males, on day 60 differences were also observed between C-IVP and the other two groups.
Red blood cell distribution width (RDW)
Different fluctuations were observed in both, males (Fig. 2k) and females (Fig. 2l). The global picture indicates higher values for C-IVP piglets from day 9 onwards (females) or day 15 onwards (males) compared to AI piglets. RDW values in RF-IVP remained between the other two groups. The most remarkable difference was observed on day 30, in which an RDW peak was observed for both males and females in the C-IVP group.
Hemoglobin concentration distribution width (HDW)
In males, higher values for HDW were found in the RF-IVP group compared to AI at day 9 (Fig. 2m). On day 15, animals from the C-IVP group showed the highest HDW compared to the other two groups, having AI the lowest values and remaining the RF-IVP value in between. On day 30, a peak was observed for the C-IVP group, and differences were observed between all groups.
Similarly, females showed the same HDW pattern through the analysis (Fig. 2n), and differences were found between C-IVP and AI. HDW values of RF-IVP remained in between C-IVP and AI.
Reticulocyte parameters
The most frequent differences found in the percentages of reticulocytes, mean cell volume of reticulocytes (MCVr), and reticulocyte hemoglobin content (CHr), were those between C-IVP animals and the other two groups, with RF-IVP animals occasionally showing similar values to one or the other group depending on the time point (Fig. 3a–f). While these differences in the C-IVP group were upward for the reticulocyte percentage, they were downward for the CHr.
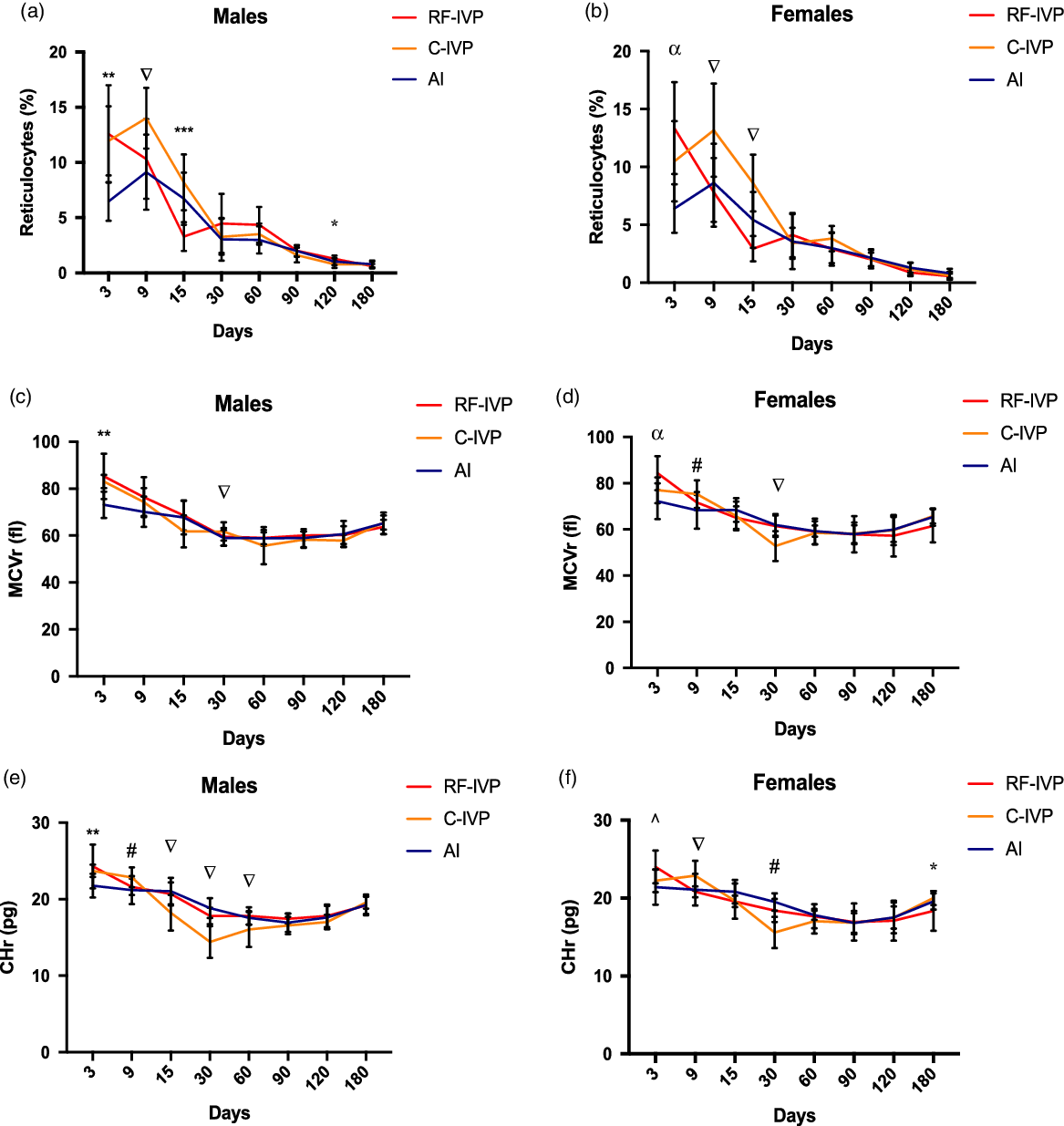
Fig. 3. Reticulocyte parameters.
White blood cells parameters
The white blood cell parameters were analyzed along the 180 days of age (including both, males and females: Supplementary Tables S10a–c). When significant differences were found between some of them, males and females were separately studied (Supplementary Tables S11a–c and S12a–c respectively).
The concentration of white blood cells (WBC) was similar for animals in the three experimental groups. Statistical differences were found in males at days 3 and 9 of life (Fig. 4a) but not in females (Fig. 4b).

Fig. 4. White blood cells parameters.
As for monocytes, eosinophils, basophils, and lymphocyte concentration, differences were found, occasionally, at different time points for males and females (Supplementary Tables 11a–c and 12a–c).
Platelets
Platelets were also analyzed along the 180 days of age (including both, males and females: Supplementary Tables S13a–c). When significant differences were found between some blood parameters, males and females were separately studied (Supplementary Tables S14a–c and S15a–c respectively). Only those differences maintained for a certain time are described below.
The kinetics for platelet concentration (PLT) through the study is shown in Fig. 5a and 5b. This parameter peaked at day 9 for both males and females in the three groups and again at day 30 but, on this occasion, only in C-IVP animals, with significant differences compared to RF-IVP and AI males and females.

Fig. 5. Platelets.
A similar pattern was found in the plateletcrit (PCT) both in males and females (Fig. 5c and 5d).
As for the mean platelet volume (MPV), mean platelet mass (MPM), and platelet dry mass distribution width (PMDW) parameters, the AI group tended to show higher values than the other two groups, particularly in females from day 30 onwards, as observed in Fig. 5f, 5h, 5j. Such differences were just occasionally observed in males on day 90 (Fig. 5e, 5g, 5i).
PLT values in males (Fig. 5k) were significantly higher in C-IVP at day 15 and significantly lower at day 90 than in R-IVP and AI. In females (Fig. 5l), PLT was significantly lower at day 3 in AI than in R-IVP and C-IVP, and C-IVP was significantly higher at day 15 than RF-IVP and AI.
Oral glucose tolerance test (OGTT)
A monophasic BGC curve was observed in the three groups (Fig. 6a). BGC steadily increased, reaching a maximum at 45 min after glucose intake, and it decreased to basal values (range 74.13–78.67 mg/dl) from that point to the end of the analysis. RF-IVP showed higher BGC values than AI at 15, 20, and 30 min. Moreover, a positive correlation between BGC value and BW was found.
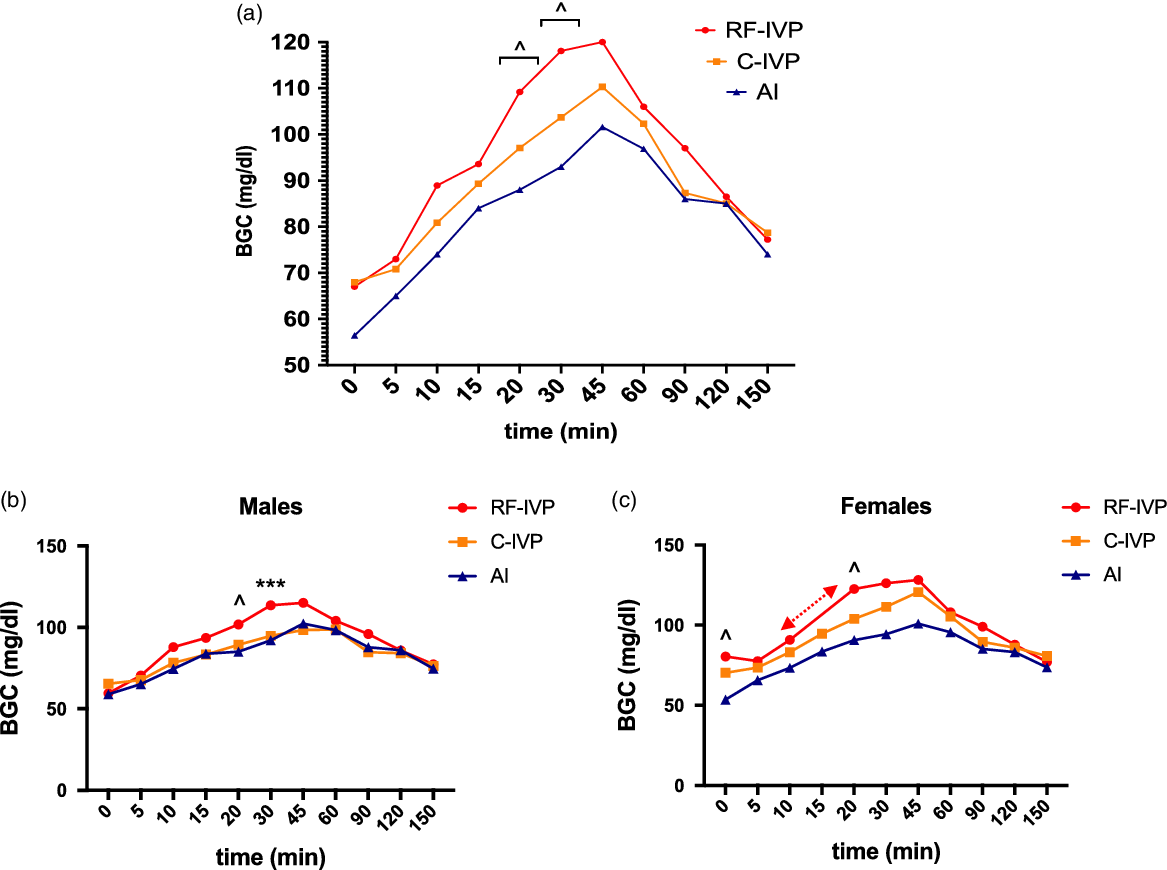
Fig. 6. Oral glucose tolerance test (OGTT).
When the analyses were repeated splitting the animals by sex, both males and females showed a monophasic curve (Fig. 6b and 6c) with similar basal levels, obtaining the highest blood glucose concentration at 45 min in all groups (Males: range 98.43–115.0 mg/dl; Females: range 100.88–128.20 mg/dl).
In males, differences between the AI and RF-IVP groups were present at 20 min and differences between the RF-IVP vs. AI and C-IVP groups at 30 min. In contrast, females showed differences between the AI and RF-IVP groups before glucose intake and at 20 min. Using the AUC analysis, differences between the AI and RF-IVP groups were identified in females (p = 0,03) until 90 min.
Discussion
The main objective of the present study was to find out if the addition of RFs during the in vitro production of pig embryos could affect the phenotype of the offspring from birth to 6 months of age. Previous studies had shown their beneficial effects on embryo development Reference Avilés, Gutiérrez-Adán and Coy15 but no data about the offspring growth and hematological traits have been published until now.
Growth differences between in vitro-derived and AI conceived piglets are modulated by RFs
The overall results of the present study, regarding birth weight and growth of the in vitro-derived offspring, show that the addition of RF to the culture media modulates the effects of the in vitro culture, mainly in males, resulting in animals phenotypically closer to those obtained by AI.
In the porcine species, the composition and physicochemical characteristics of the culture media for IVF have been shown to directly affect the development of blastocysts and the methylation and expression pattern of their genes. Reference Canovas, Ivanova and Romar3 Furthermore, our results show that the culture media used can have long-term consequences on pig growth.
In this study, males in the AI group showed a mean birth weight similar to that previously reported for this breed Reference Kyriazakis and Whittemore22 but lower when compared to the experimental groups (RF-IVP and C-IVP). These differences in bodyweight were maintained throughout the study period, where the C-IVP group was always the heaviest. Although the first plausible explanation for these differences could be attributed to the lower litter sizes in the two in vitro groups, the statistical analysis did not confirm such a hypothesis, since no correlation was found between litter size and weight at days 0, 30, 75, 120, and 180. Besides, litter sizes in the RF-IVP group were lower than in the C-IVP group, Reference Avilés, Gutiérrez-Adán and Coy15 which, again, would not explain the higher weight of the C-IVP animals.
In the case of females, a similar scenario was found, except for day 180, when the weight of group RF-IVP was higher than the weight of group C-IVP. In both cases, throughout the study, the RF-IVP group showed intermediate weight values, compared to C-IVP and AI groups. In the study of García-Vázquez et al., Reference García-Vázquez, Ruiz and Matás23 who transferred zygotes obtained by intracytoplasmic injection into the oviductal lumen, the weight at birth of the piglets was similar to that of our AI group but lower than that of our IVP groups. This may be due to the different procedures used, since, in our case, the embryos were produced in vitro and transferred at the blastocyst stage to the uterine lumen, increasing the time of exposition to a stressful environment outside the maternal body and hypothetically, leading to higher birth weights, as it occurs in calves. Reference Jacobsen, Schmidt, Helm, Sangild, Greve and Callesen24,Reference Van Wagtendonk-de Leeuw, Mullaart and De Roos25
Furthermore, in this study, we observed different effects of RF addition depending on sex. In males, differences in BW appeared between the C-IVP and the other two groups at the initial (day 0) and middle (day 90) time points. By contrast, during the second half of the experiment (from day 90 onwards), differences appeared between the AI and the two experimental groups. This result could suggest that the beneficial effect of the RF during the embryo and fetus development, and after birth, are in some way vanished from month 3 of postnatal life onwards, as the growth of the piglet is progressing. In females, the differences between the AI group and the experimental groups occurred at all the time points analyzed. These differences are corroborated by the report of Feuer et al. Reference Feuer, Donjacour and Simbulan26 in mice, where, although exposure of the embryos to a stressful environment caused lower bodyweights instead of higher, the effect differed depending on sex. In sum, our data indicate that the addition of RF to IVF and EC media could modulate the effects of embryonic stress in the case of males, providing the offspring with a phenotype similar to those born through AI, although these differences were compensated during the second half of the study (from day 90); by contrast, the addition of RF in the females seem not to affect their weight.
Even so, the ADWG was higher in C-IVP piglets for both sexes, at almost every time point followed by group RF-IVP, with group AI being the one that usually gained the least weight. These data suggest, again, that the addition of RF benefits the growth of both males and females in some way. Indeed, by comparing the ADWG values from our study during the first 75 days of age with those reported in the literature for this breed Reference Andersson, Haley and Ellegren27 we found that our results in both males and females from the RF-IVP group are the closest to those reported by Andersson et al., Reference Andersson, Haley and Ellegren27 being instead greater for the C-IVP group and lesser for the AI group. Besides, our data do coincide with those reported by Kyriazakis and Whittemore Reference Kyriazakis and Whittemore22 since, in all cases, the ADWG exceeded 0.750 Kg/day from day 120 onwards, when the animals had mostly exceeded 70 kg of weight.
As for the CRL, at most time points and for both sexes, the C-IVP group had the longest CRL, followed by group RF-IVP and being AI finally the shortest. Studies in humans have shown that during the first two years of age, the type of culture medium used in ART can affect the height of children. Reference Kleijkers, Van Montfoort and Smits28 These results coincide with those observed in the present study where not only differences depending on the culture media were noticed, but also differences between in vitro-derived and naturally conceived animals. Furthermore, observing both the weight and length data, we found that piglets born through ART show faster growth, coinciding with the data reported by Ceelen et al. Reference Ceelen, Van Weissenbruch and Prein29 On the other side, Gu et al., Reference Gu, Shi and Luo30 provided recently some parameters for cloned pigs of up to 72 weeks of age, being their results for growing animals similar to those obtained in our study.
However, although piglets in group RF-IVP also grew faster than those from group AI, they maintained intermediate CRL measurements between the other two groups. Finally, at the end of the experiment, and in both sexes, group RF-IVP outperformed CRL in groups C-IVP and AI. This seems to support the idea that the effect of adding fluids has consequences on growth parameters only during the first months of age, although longer studies would be necessary to confirm such a statement.
In summary, our work offers the first comparative data of the growth of animals produced through three assisted reproductive techniques (artificial insemination, transfer of embryos produced in vitro in culture media supplemented with RFs, and transfer of embryos produced in vitro in media culture supplemented exclusively with BSA), demonstrating differences between the groups for both males and females. Overall, there is a tendency to have a larger size at birth and faster growth in animals derived from in vitro fertilization and embryo culture, although this trend is diminished by the addition of RFs to the culture media.
Hematological differences between groups do not seem to provide clinical significance
Another overall observation of the present study was that hematological parameters were more similar between piglets born by AI and piglets derived from embryos produced with RF than those without RF. As previously described, there are very few references in the literature for hematological parameters in growing piglets. Thus, making firm comparisons using a reference interval is a challenging task. However, in a study by Casas-Díaz et al., Reference Casas-Díaz, Closa-Sebastià, Marco, Lavín, Bach-Raich and Cuenca17 reference intervals for wild boar were established for different age ranges being those described by Ventrella et al. Reference Ventrella, Dondi and Barone19 for piglets between 5 and 30 days old.
To compare the hematological parameters obtained with those provided by Casas-Díaz et al., Reference Casas-Díaz, Closa-Sebastià, Marco, Lavín, Bach-Raich and Cuenca17 we based our comparisons on the bodyweight of the animals since, according to their study, juvenile wild boars, for example, presented a weight of around 30 kg, which is the approximate weight of our piglets between days 75 and 90 postnatally.
Analyzing the erythrocyte indices, despite some exceptions, similar patterns were observed throughout the study in both males and females, with the C-IVP group being particularly striking compared with the other two groups. Interestingly, both AI and RF-IVP had a peak in Hb on days 15 and 30 of age, respectively, and both days also coincided with a drop in the percentage of reticulocytes. By contrast, the C-IVP group presented a first rise in Hb and HTC on day 9 of age, reaching the lowest levels of reticulocytes on day 30 of age.
Besides, according to Ekert et al., Reference Ekert Kabalin, Balenović, Valpotić, Pavičić and Valpotić31 an increase in RBC was accompanied by a decrease in reticulocyte parameters. However, a drop in the concentrations of HB, HTC, MCV, and MCH was also observed on day 30 for the C-IVP group, which was associated with an increase in RDW and HDW. It should be noted that this finding coincides with the weaning of the piglets, although previously, in all groups, and 7–8 days before weaning, a solid feed was introduced to the diet in addition to the sow’s milk. According to Ventrella et al., Reference Ventrella, Dondi and Barone19 even though all the parameters were within the established range, this change in diet, along with a maturation of the gastrointestinal system, could explain the differences observed, showing that the AI and RF-IVP groups had a similar pattern of changes. Interestingly, the HB peaks in males were reached at different time points in each group (day 9 for C-IVP, day 15 for RF-IVP, and day 28 for AI), which matches with the periods when males exceded 3.5 kg of weight. This coincidence could also indicate differences in the maturation phases of the animals, although further studies are necessary to confirm this observation.
It is known that Hb is an indicator of iron status, Reference Miller32 therefore, a decrease in blood Hb concentration could be related to an iron deficiency. However, taking into account the age of the piglets, the immaturity of iron metabolism could be another of the possible causes for the observed decrease. Reference Ventrella, Dondi and Barone19 Furthermore, this phenomenon was accompanied by a decrease in MCV and MCHC, with both parameters being lower in the C-IVP group compared to the AI group.
Similarly, a reduction in RBC, Hb, HTC, MCHC, and MCV, as well as an increase in RDW, was also reported by Yeom et al., Reference Yeom, Cho, Park and Lee33 in miniature young piglets, being compatible with iron deficiency anemia. However, taking into account that all the parameters were within the established range, according to Ventrella et al., Reference Ventrella, Dondi and Barone19 this finding in the first month of age seems to be a physiological adaptation phenomenon of the newborn during the first days of life.
As for the variations found for the lymphocyte concentrations in the total white cell count, they could be a possible consequence derived from stress due to manipulation during the blood collection, as previously suggested. Reference Grindem34,Reference Dubreuil and Lapierre35
When platelets were analyzed, both PLT and PCT showed higher values on day 30 for C-IVP piglets. An increase in the number of platelets is related to rapid growth. Reference Stockham and Scott36 Since those piglets in the C-IVP group showed a higher bodyweight and ADWG than the RF-IVP and AI groups, this could explain the increase in the platelet count. On the other hand, this finding has also been related to an iron deficiency, Reference Evstatiev, Bukaty and Jimenez37 which could also be explained due to the decrease in the Hb concentration above mentioned. However, most of the platelet indices are still under investigation Reference Kim, Park and Seo38 and thus a clear clinical significance cannot be attributed to the differences found.
To sum up, and despite the statistical differences found, we must notice that all the hematological parameters analyzed were within the normal range for all experimental groups, sex, and times of analysis and a definitive clinical meaning cannot be established.
Glucose tolerance in growing piglets is affected by embryonic origin and sex
In several species, like the mouse or human, subtle, yet significant changes in glucose metabolism in ART-derived offspring have been shown Reference Vrooman and Bartolomei39–Reference Chen, Wu, Zhao, Wu, Davies and Wittert41 . Both IVF and EC take place during a critical stage of embryonic development and entail stressful conditions which could modulate embryonic programming leading to impaired glucose tolerance in adult life. Moreover, suboptimal in vitro culture conditions have revealed a significant impact on glucose metabolism in mice, whereas optimized culture conditions restored normal glucose levels. Reference Donjacour, Liu, Lin, Simbulan and Rinaudo42 On the other side, it has been shown that the oral ingestion of glucose triggers a more physiological and greater insulin release since gastric inhibitory polypeptide and glucagon-like peptide-1 (GLP-1) are also released. Reference Manell, Hedenqvist, Svensson and Jensen-waern21
In our study, oral glucose tolerance was evaluated in piglets from in vitro- (C-IVP and RF-IVP) and in vivo-(AI)-derived embryos at day 45 of age, as part of the phenotypical characterization of the offspring, to evaluate the impact of in vitro culture using two different media.
In vivo and in vitro groups displayed a similar tendency after glucose intake, even though some differences were observed between the AI and RF-IVP groups during the ascendant phase. Nonetheless, the area under the curve (AUC), which is an index of whole glucose excursion and provides more information about glucose tolerance than glucose levels at a single time point Reference Sakaguchi, Takeda and Maeda43 did not show differences between the groups after 90 min. Two hours is the analysis period in humans for glucose intolerance in OGTT, but this period is extended up to 180 or 240 min in pigs. Reference Manell, Hedenqvist, Svensson and Jensen-waern21,Reference Pluschke, Simmons and Keates44 Our study stopped blood sample collection at 150 min because glycemic values were similar in all groups at this time. Considering that all the basal and peak values were in the physiological range, the fact of having selected the two piglets with the highest and lowest weight per litter would lead us to conclude that both extremes could be an example of representation of the entire group since we would be evaluating the weakest as well as the strongest. However, we cannot discard differences among groups that could emerge later into adulthood.
Conclusions
The results of this study demonstrate some differences, particularly in growth parameters, and less evident in hematological indices and glucose tolerance, between animals derived from AI and those derived from IVP, with a sex-dependent effect. When RF were added to the culture media, these differences tended to fade. Based on these findings, we conclude that the addition of RF to IVF and EC media could contribute to minimizing the phenotypical differences between the in vitro-derived offspring and those born through AI, particularly in males. Future experiments will be needed to delve into whether differences in glucose tolerance could be observed during adulthood.
Acknowledgments
The authors are thankful to Sergio Navarro Serna, Salvador Ruiz, Carmen Matás, Rafael Latorre, Octavio López, Ernesto Rodríguez Tobón, Ondřej Šimoník and Guillermo Ramis for their valuable assistance. We also appreciate the support of Sección de Apoyo Estadístico (SAE), Área Científica y de Investigación (ACTI), Universidad de Murcia (www.um.es/web/acti).
Financial support
European Union, Horizon 2020 Marie Skłodowska-Curie Action (Rep-Biotech 675526); Spanish Ministry of Economy and Competitiveness (MINECO) & European Regional Development Fund (FEDER), (AGL2015-66341-R); Fundación Séneca, Agencia de Ciencia y Tecnología de la Región de Murcia (20,040/GERM/16). I+D+i PID2020-113366RB-I00, funded by MCIN/AEI/10.13039/501100011033/ and “FEDER Una manera de hacer Europa”.
Conflicts of interest
The authors declare no conflicts of interest.
Ethical standards
The experimental work performed in this study was submitted to evaluation by the CEEA (Comité Ético de experimentación Animal) from University of Murcia. After approval, authorization from “Dirección General de Agricultura, Ganadería, Pesca y Acuicultura” – Región de Murcia – nr A13170706 was given to perform the experiments with animals.
Author’s contributions
PC conceived the idea. PC, RR, and SC designed the study. PC, SC, and EP-O wrote the first manuscript draft. EP-O, R B-P, and CS-U analyzed the data.
All the authors contributed to data collection and the final editing of the manuscript. All authors have approved the final version of the manuscript.
Supplementary material
To view supplementary material for this article, please visit https://doi.org/10.1017/S2040174421000702