Introduction
Obesity is a significant health challenge facing modern society. The World Health Organisation has reported that prevalence has nearly tripled over the last 40 years. Reference Ng, Fleming and Robinson1 With an estimated 1.9 billion people considered overweight or obese globally, the social and financial burdens of this epidemic are staggering. Reference Ng, Fleming and Robinson1,2 In part, obesity has been attributed to the consumption of added sugars, with the greatest source from sugar-sweetened beverages (SSBs). Reference Bailey, Fulgoni, Cowan and Gaine3 Epidemiological studies have found that consumption of added sugars is associated with obesity, type 2 diabetes and cardio-metabolic disease. Reference Luger, Lafontan, Bes-Rastrollo, Winzer, Yumuk and Farpour-Lambert4-Reference Yang, Zhang, Gregg, Flanders, Merritt and Hu8 However, what is less well understood is the potential influence added sugars may have on the developing fetus during early life.
There is a growing recognition that the origins of obesity begin in utero; extensive research has consistently shown an increased risk of childhood obesity when the mother is overweight or obese Reference Whitaker9,Reference Catalano and Shankar10 or consumes a high-fat diet. Reference Parlee and MacDougald11-Reference Ribaroff, Wastnedge, Drake, Sharpe and Chambers13 This intergenerational link may be a factor in the amplification of the obesity epidemic. Reference Camilleri-Carter, Dowling, Robker and Piper14 Dysregulation of gene expression through DNA methylation, histone modification and mitochondrial dysfunction has been reported in obese offspring. Reference Desai, Beall and Ross15,Reference Fernandez-Twinn, Constância and Ozanne16 Whether excessive maternal sugar consumption is involved in these mechanistic links remains unclear.
Three recent epidemiological studies have found an association between maternal SSBs consumption during pregnancy and childhood adiposity. Reference Gillman, Rifas-Shiman, Fernandez-Barres, Kleinman, Taveras and Oken17-Reference Chen, Aris and Bernard19 Specifically, body mass index and fat mass were higher in children and infants under 7 years of age, independent of the child’s SSBs intake. This association was stronger when mothers consumed SSBs during the second trimester. Reference Gillman, Rifas-Shiman, Fernandez-Barres, Kleinman, Taveras and Oken17 However, such studies are inevitably subject to a range of confounding factors that limit their ability to identify causality. Because they can eliminate confounds, animal models have advanced our understanding of the biological mechanisms involved in maternal over-nutrition, Reference Tellechea, Mensegue and Pirola20,Reference Christians, Lennie, Wild and Garcha21 including high-sugar diets. Reference Vickers, Clayton, Yap and Sloboda22 Given SSB intake is a prime target for the prevention of excessive weight gain during pregnancy, it is important to validate the epidemiological findings Reference Gillman, Rifas-Shiman, Fernandez-Barres, Kleinman, Taveras and Oken17-Reference Chen, Aris and Bernard19 in animal models. Evidence from some rodent studies where dams were fed sucrose as part of a solid food diet during pregnancy has reported impaired metabolism in their offspring. These impairments have included increased body weight (BW), adiposity, hyperglycaemia, insulin resistance and altered hepatic lipid metabolism. Reference D’Alessandro, Oliva, Fortino and Chicco23-Reference D’Alessandro, Oliva, Ferreira, Selenscig, Lombardo and Chicco25 The translational value of these experiments is limited because the sucrose content (up to 65% of energy intake) was much higher than what humans normally consume. Thus, a more appropriate animal model for human SSB consumption during pregnancy would be the manipulation of the maternal diet with sucrose administered in solution.
The research included in the present review was confined to studies in which dams were provided with sucrose solutions at concentrations more comparable to those found in commercially available SSBs (8–20% w/v). We sought to determine whether sucrose consumption at this concentration alters offspring metabolism. The main aim was to identify possible relationships between maternal intakes of sucrose solutions and offspring outcomes for BW, body composition and glycaemic control. A secondary aim was to assess whether maternal sucrose consumption during pregnancy affects their offspring’s consumption patterns.
Materials and methods
This review was reported in accordance with the Preferred Reporting Items for Systematic Reviews and Meta-Analysis (PRISMA 2009) Guidelines (Supplementary Table S1). The protocol was developed with the SYstematic Review Centre for Laboratory animal Experimentation’s (SYRCLE) Protocol template, Version 2.0 Reference de Vries, Hooijmans and Langendam26 and registered on Prospero on the 18th March 2019 (Protocol number: 127115; available from https://www.crd.york.ac.uk/prospero/display_record.php?RecordID=127115) and Camarades NC-3R Systematic Review Facility (http://syrf.org.uk/protocols/).
Search strategy and study selection
The following databases were searched from inception to 15 January 2019: PubMed, EMBASE via OvidSP, Web of Science and Scopus. The extensive search strategy used keywords, medical subject headings (MeSH), EMTREE terms and related synonyms based on maternal sucrose feeding and fetal development. Previously published animal filters Reference Hooijmans, Tillema, Leenaars and Ritskes-Hoitinga27,Reference de Vries, Hooijmans, Tillema, Leenaars and Ritskes-Hoitinga28 were adapted to identify rodent (rat and mouse) papers and used in the final search sets for PubMed and EMBASE. No animal filters were used for Web of Science and Scopus as they are currently not available. The final search strategy for each database can be found in Supplementary Table S2. Extracted publications were combined and duplicates manually removed in Endnote reference management software (EndNote™ X8).
Papers were screened by two independent reviewers, according to pre-defined exclusion criteria. Based on title and abstract, papers were excluded if they were non-experimental, non-rodent, genetically bred or metabolically compromised rodent models, non-interventional during pregnancy or lactation or if they reported fetal but no offspring outcomes. Further exclusion criteria were applied to full-text screening. We then further excluded papers with no appropriate control, isocaloric pair-feeding models, high-fat diets (>15% total energy content), protein/calorie restricted diets, intragastric or intraperitoneal feeding models or if sucrose was administered as a solid component of the diet (i.e. chow component). Only papers providing dams with a sucrose solution with a concentration between 8 and 20 % w/v were included. No restriction was placed on publication date, but only English papers were included. Hand screening of references lists was performed to identify further publications.
Data extraction
We extracted bibliographical (author, year, title) and methodological information (experimental conditions, maternal and offspring sample size, unit of analysis) from eligible papers. Data on animal characteristics (species, strain, age, weight, offspring sex, litter standardisation), sucrose feeding (exposure timing: during pre-conception and/or pregnancy and/or lactation; concentration/s; compulsory or voluntary administration), maternal and offspring metabolic (bodyweight, body composition, glycaemic control) and behavioural outcomes (food/fluid intake and preference behaviours) were also extracted. In cross-fostering models, data were extracted for each experimental group. Outcome measures were collected as mean and standard error of mean (S.E.M.) or standard deviation (S.D.), as reported in the publications. If required, a digital ruler (Pixel Ruler version 3.1) was used for extracting graphical data. A second reviewer checked 5% of the extracted data for errors and discrepancies were corrected based on the original text. Attempts to contact the author were made if data were not available or further information was required.
Meta-analysis
The main outcome measures included for meta-analyses were maternal and offspring BW and fasting glucose levels (FGLs). Random effects meta-analyses were performed using R Studio Statistical Package, Version 3.5.1 (2018-07-02) ‘Feather Spray’ for standardised mean differences (SMD) and corresponding 95% confidence intervals. The metacont function from the meta package was used for standard analyses. The rma function from the metafor package was used for the meta-regression. Papers containing different interventional groups of animals, Reference Ozkan, Topsakal and Ozmen29 including those using cross-fostering models, Reference Toop, Muhlhausler, O’Dea and Gentili30,Reference Bocarsly, Barson and Hauca31 were included as separate data sets and thus effectively treated as independent experiments. For studies that compared more than one data set to the same control group, a correction was made using the following equation: N corrected control = N control/no. of experimental groups. Reference Vesterinen, Sena and Egan32 The I2 statistic was used to assess heterogeneity.
Data for maternal BW (~PND21) and data for maternal FGL (taken between pre-conception and PND21) were extracted, and separate meta-analyses were performed for these outcomes. All dams were exposed to a minimum of 4 weeks’ sucrose feeding. Offspring BW data were extracted in the pre-weaning period (PND15–28) and adulthood (PND56–504). Offspring analyses included rodents exposed to sucrose prior to mating and/or post mating (i.e. pregnancy or pregnancy and lactation); however, rodents exposed to sucrose only during the lactation period (through a cross-fostering model) were excluded to analyse in utero effects. Offspring provided direct access to sucrose solution post-weaning were also excluded. Sub-group analyses were conducted on maternal and offspring data for sucrose concentration, strain and on sex in offspring outcomes. In addition, a sub-group analysis, separated by pre and post mating sucrose exposure, was performed on offspring BW to eliminate confounding effects of the maternal metabolic state. Meta-regression analysis was also performed to analyse the effect of offspring age on BW during adulthood. All data are presented as forest plots drawn using the forest function from the metafor package. Four papers included in this review were excluded from the meta-analysis for the following reasons: (i) no sample size reported; (ii) insufficient statistical reporting and (iii) insufficient data for pre-weaning or adult time points.
Study quality assessment
A comprehensive assessment of methodological quality was performed using the SYRCLE’s Risk of Bias (RoB) Tool. Reference Hooijmans, Rovers, de Vries, Leenaars, Ritskes-Hoitinga and Langendam33 Adapted from the Cochrane RoB Tool, Reference Higgins, Altman and Gotzsche34 it has been specifically designed for use in systematic reviews of animal models to assess internal validity. Briefly, the checklist relates to five categories of bias: selection (items 1–3), performance (items 4–5), detection (items 6–7), attrition (item 8), reporting (item 9) and with the final item relating to litter as the unit of analysis (item 10). Due to multiparous births in rodents, using the individual pup as an experimental unit rather than litter increases the chance of Type 1 errors and as such may cause unjustly inflated precision. Items were judged as ‘low’ risk of bias, ‘high’ risk of bias or ‘unclear’ if we were unable to clearly assign risk of bias. Two independent reviewers (HLM and CHL or HLM and RA) assessed the included publications, with discrepancies resolved by discussion.
In addition, all papers were assessed for reporting quality by adherence to the Animal Research: Reporting of in Vivo Experiments (ARRIVE) Guidelines Checklist. Reference Kilkenny, Browne, Cuthill, Emerson and Altman35 Developed by the National Centre for the Replacement, Refinement and Reduction of Animals in Research (NC3Rs) to improve reporting standards in animal publications, our assessment aimed to identify specific areas of poor reporting to aid in evaluating the reliability of each paper. We modified and developed the ARRIVE Guidelines into evaluation descriptors and a reporting system (Supplementary Table S3). The 20-item checklist was extended to include 40 associated sub-items relevant to this review. Items and sub-items are described as fully reported (FR), partially reported (PR) or non-reported, similar to previously reported tools. 36,Reference Morahan, Leenaars, Boakes and Rooney37
Results
Study selection
Our initial database search identified 4678 papers for review. Following removal of duplicates (2219 papers), a total of 2459 papers were screened based on eligibility criteria applied to title and abstract (2181 papers excluded) and full text (263 papers excluded). Searching of reference lists did not yield any further inclusions. Fifteen papers (including 16 maternal groups and 19 offspring groups) of maternal sucrose exposure were identified for final inclusion in this systematic review (see Fig. 1). A full list of the 263 excluded papers in Phase 2 and reason for exclusion can be found in Supplementary Table S4.
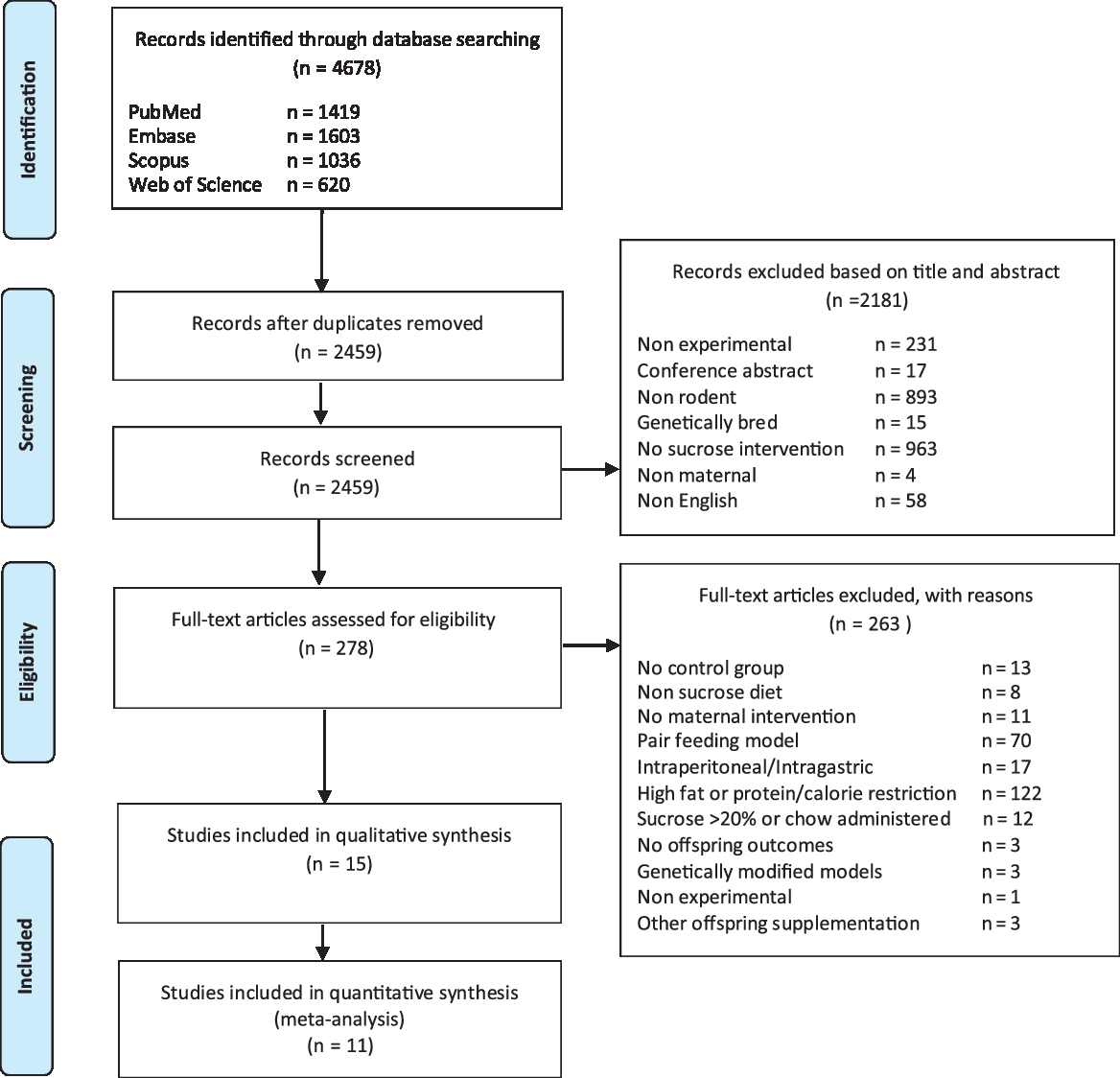
Fig. 1. PRISMA flow.
Study characteristics and design
Although several terms for mice were included in the database search, only rat studies were identified. Eleven of the included papers used Sprague-Dawley rats, Reference Bocarsly, Barson and Hauca31,Reference Kisioglu and Nergiz-Unal38-Reference Wu, Mao and Liu47 three used Wistar rats Reference Toop, Muhlhausler, O’Dea and Gentili30,Reference Kendig, Ekayanti, Stewart, Boakes and Rooney48,Reference Toop, Muhlhausler, O’Dea and Gentili49 and one did not report strain type. Reference Ozkan, Topsakal and Ozmen29 Sucrose was administered at either 10% w/v Reference Ozkan, Topsakal and Ozmen29-Reference Bocarsly, Barson and Hauca31,Reference Kendig, Ekayanti, Stewart, Boakes and Rooney48,Reference Toop, Muhlhausler, O’Dea and Gentili49 or 20% w/v Reference Ozkan, Topsakal and Ozmen29,Reference Kisioglu and Nergiz-Unal38-Reference Wu, Mao and Liu47 and maternal exposure ranged from 16 to 126 days, including periods during pre-conception, gestation and lactation. The majority Reference Ozkan, Topsakal and Ozmen29,Reference Toop, Muhlhausler, O’Dea and Gentili30,Reference Vesterinen, Sena and Egan32-Reference Wu, Mao and Liu47 employed a compulsory drinking paradigm with sucrose solution being the only source of drinking fluid. A voluntary consumption model was used in two papers, with sucrose offered in addition to drinking water. Reference Bocarsly, Barson and Hauca31,Reference Kendig, Ekayanti, Stewart, Boakes and Rooney48 In all papers, offspring were weaned on to chow and water. They remained on this standard diet until the end of experimental testing, except for one study. Reference Kendig, Ekayanti, Stewart, Boakes and Rooney48 Here male offspring aged ~13 weeks old were given direct access to chow and 10% sucrose solution for seven weeks. This data set was not included in offspring meta-analyses to avoid confounding the results. Study designs for maternal sucrose interventions can be found in Supplementary Table S5. A cross-fostering model was used in two papers, giving rise to three additional offspring groups. Reference Toop, Muhlhausler, O’Dea and Gentili30,Reference Bocarsly, Barson and Hauca31 Full details of extracted study characteristics can be found in the Supplementary Table S6.
For each study, the chow component of the diet was identical between control and treatment groups; therefore, the only difference was availability of sucrose in drinking fluid. Macronutrient composition of control diet was not always reported, Reference Ozkan, Topsakal and Ozmen29,Reference Gu, He and Fan41,Reference Wu, Shi and Zhu44,Reference Wu, Li and Bo46,Reference Wu, Mao and Liu47 and, for the papers that included this information, variations were noted in energy content for fat (range 4% to 13.5%), carbohydrate (39.1% to 60%) and protein (19.3% to 28.5%). Total energy content was similar between reported chows (~ 3 to 4 kcal/g). A full breakdown of reported chow composition can be found in the Supplementary Table S7.
Maternal outcomes
A summary of maternal outcomes is presented in Table 1. Ten of the included papers reported maternal results for bodyweight, body composition, glycaemic control and consumption. Due to variations in experimental design between studies, data were not available for all of the maternal outcomes listed in Table 1.
Table 1. Summary of maternal characteristics and outcome measures of included studies. Reported outcomes extracted include maternal bodyweight prior to mating, during pregnancy and lactation, fasting blood glucose and insulin levels and consumption data

DNP – data not provided; FBGL – fasting blood glucose levels; FPI – fasting plasma insulin; G – gestation; GD21 – gestational day 21 (last day of pregnancy); L – lactation; N – sample size; NR – not reported; PND – postnatal day; PC – pre-conception; PND21 – postnatal day 21 (last day of lactation); SD – Sprague-Dawley; WS – Wistar; % w/v – concentration percentage weight per volume; – outcome not measured; ↑increase; ↓decrease; † data measured at PND28; * graphical data provided but unable to determine statistical effect.
Maternal BW and body composition
Maternal BW data were extracted from seven experimental groups. Reference Ozkan, Topsakal and Ozmen29,Reference Bocarsly, Barson and Hauca31,Reference Yuruk and Nergiz-Unal43,Reference Kuang, Sun and Lv45,Reference Kendig, Ekayanti, Stewart, Boakes and Rooney48,Reference Toop, Muhlhausler, O’Dea and Gentili49 No significant change was reported for BW in dams measured during gestation (GD21), Reference Kuang, Sun and Lv45 lactation (PND21) Reference Bocarsly, Barson and Hauca31 or both time-points. Reference Yuruk and Nergiz-Unal43,Reference Kendig, Ekayanti, Stewart, Boakes and Rooney48,Reference Toop, Muhlhausler, O’Dea and Gentili49 Two data sets from one study observed a significant increase in BW at PND21 when dams were fed 10% or 20% w/v sucrose during pregnancy and lactation. Reference Ozkan, Topsakal and Ozmen29 A meta-analysis on six of the extracted data sets measured at PND21 did not show a significant effect on maternal BW from sucrose consumption (SMD = 0.76, 95% CI -0.21, 1.73, I 2 = 77%), as seen in Fig. 2. Body composition in dams was seldom reported, with fat mass described in only three papers. Fat mass was assessed either directly by the Soxhlet method for total body fat content Reference Kisioglu and Nergiz-Unal38 or by resection of fat pad deposits Reference Kendig, Ekayanti, Stewart, Boakes and Rooney48,Reference Toop, Muhlhausler, O’Dea and Gentili49 or, indirectly, by plasma leptin concentration. Reference Toop, Muhlhausler, O’Dea and Gentili49 Increased total body fat was observed in dams fed sucrose for 18 weeks from pre-conception to lactation. Reference Kisioglu and Nergiz-Unal38 Increased visceral and subcutaneous adipose tissue in dams fed from 4 weeks pre-conception to lactation was noted, although leptin concentration did not differ between sucrose fed dams and control dams. Reference Toop, Muhlhausler, O’Dea and Gentili49 Conversely, no significant difference was identified in retroperitoneal fat pad analysis during a shorter 10-week intervention, commencing four weeks pre-mating and ceasing at parturition. Reference Kendig, Ekayanti, Stewart, Boakes and Rooney48

Fig. 2. Maternal body weight.
Maternal FGLs, glycaemic control and triglycerides
Maternal intervention groups received a minimum of 4 weeks’ access to sucrose solutions and data were obtained for FGL in 10 experimental groups. Two data sets were taken prior to mating, Reference Toop, Muhlhausler, O’Dea and Gentili30,Reference Kendig, Ekayanti, Stewart, Boakes and Rooney48 five during pregnancy Reference Feng, Zhou and Zhang40-Reference He, Zhang and Yang42,Reference Kuang, Sun and Lv45,Reference Wu, Li and Bo46 and three at the end of lactation. Reference Ozkan, Topsakal and Ozmen29,Reference Yuruk and Nergiz-Unal43 Meta-analysis identified a significant increase in FGL in dams exposed to sucrose relative to control dams (SMD = 1.61; 95% CI = 0.78, 2.44; I 2 = 80%), as shown in Fig. 3a. When concentration was taken into consideration, no effect was evident in 10% w/v solutions (SMD = 0.43; 95% CI= -0.68, 1.53; I 2 = 76%), whereas 20% solutions resulted in a significant elevation in maternal FGL, with low heterogeneity (SMD = 2.02; 95% CI = 1.51, 2.52; I 2 = 0%), see Fig. 3b. As for strain, although Wistar rats showed no significant effect (SMD = -0.07; 95% CI= -0.85, 0.71; I 2 = 56%), FGL was significantly elevated in Sprague-Dawley rats (SMD = 2.05; 95% CI = 1.51, 2.59; I 2 = 4%); see Fig. 3c. Two data sets did not report strain type. Reference Ozkan, Topsakal and Ozmen29 It is worth noting that both studies using Wistar rats provided sucrose concentrations of 10% w/v, thus making it impossible to determine whether these failures to detect an effect of sucrose consumption on FGL were due to concentration or strain. Reference Kendig, Ekayanti, Stewart, Boakes and Rooney48,Reference Toop, Muhlhausler, O’Dea and Gentili49
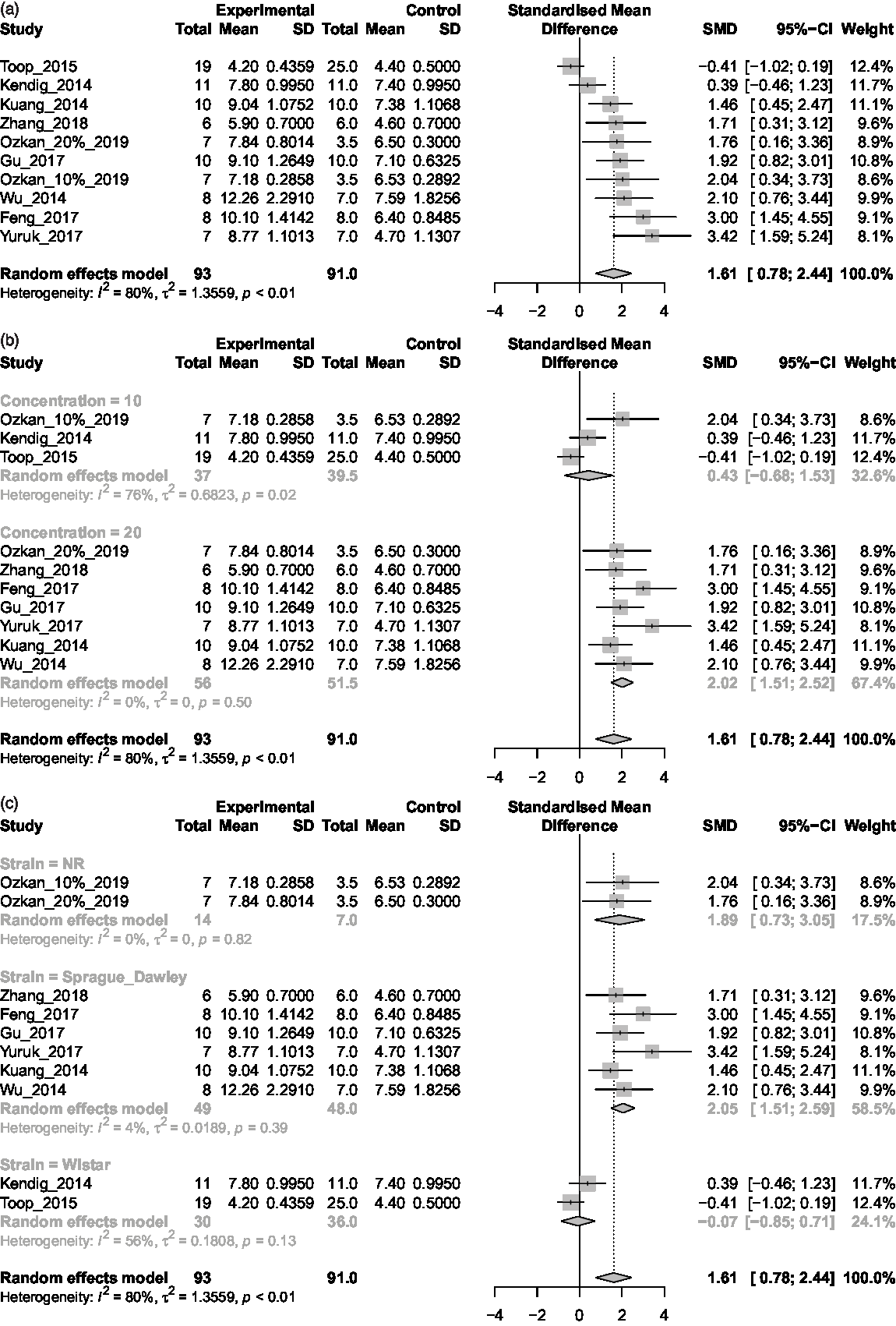
Fig. 3. (a) Maternal BGL. (b) Maternal BGL concentration. (c) Maternal BGL.
Maternal fasting insulin was reported in four data sets, with three observing a significant increase relative to control Reference Ozkan, Topsakal and Ozmen29,Reference Yuruk and Nergiz-Unal43 , whereas one found no change. Reference Toop, Muhlhausler, O’Dea and Gentili49 Two papers reported dynamic assessment of maternal glycaemic control through glucose tolerance tests (GTT). Reference Kendig, Ekayanti, Stewart, Boakes and Rooney48,Reference Toop, Muhlhausler, O’Dea and Gentili49 Reduced glucose disposal was observed following either oral Reference Kendig, Ekayanti, Stewart, Boakes and Rooney48 or intraperitoneal Reference Toop, Muhlhausler, O’Dea and Gentili49 administration of a glucose load, thus suggesting glucose intolerance. In both cases, this was measured just prior to conception, following 4 weeks of sucrose exposure. However, subsequent intraperitoneal GTT, performed at the end of lactation, observed an amelioration of this effect. Reference Toop, Muhlhausler, O’Dea and Gentili49
Two data sets provided plasma triglyceride (TG) concentrations. Reference Kendig, Ekayanti, Stewart, Boakes and Rooney48,Reference Toop, Muhlhausler, O’Dea and Gentili49 One identified a significant increase at the pre-conception time point Reference Kendig, Ekayanti, Stewart, Boakes and Rooney48 and one observed no change at the end of lactation. Reference Toop, Muhlhausler, O’Dea and Gentili49
Maternal food and fluid intake
Significant variation in consumption was evident in eight data sets reporting maternal food and fluid intakes during the intervention period. Reference Ozkan, Topsakal and Ozmen29,Reference Feng, Zhou and Zhang40,Reference Kuang, Sun and Lv45,Reference Kendig, Ekayanti, Stewart, Boakes and Rooney48,Reference Toop, Muhlhausler, O’Dea and Gentili49 Sustained increases in sucrose solution intake compared to water were found in two groups, with a compensatory reduction of chow intake Reference Kendig, Ekayanti, Stewart, Boakes and Rooney48,Reference Toop, Muhlhausler, O’Dea and Gentili49 . Other studies reported either reduced fluid intake, Reference Ozkan, Topsakal and Ozmen29 an increase in food intake with no change in fluid Reference Kisioglu and Nergiz-Unal38 or no change in food or fluid intake relative to control dams. Reference Feng, Zhou and Zhang40,Reference Kuang, Sun and Lv45
Relationship between maternal sucrose consumption and offspring BW and body composition
The relationship between maternal sucrose consumption and BW in pre-weaning and adult rats was not uniform across papers (Table 2). Substantial increases in offspring BW during the pre-weaning period, defined as PND1–28, were found in some studies, Reference Ozkan, Topsakal and Ozmen29,Reference Zhang, Zhu and Zhang39,Reference Kuang, Sun and Lv45 whereas no effect was found in others Reference Toop, Muhlhausler, O’Dea and Gentili30,Reference Kendig, Ekayanti, Stewart, Boakes and Rooney48 or even a significant reduction. Reference Bocarsly, Barson and Hauca31 Overall, the meta-analysis on 13 experimental data sets from seven papers identified a minor elevation in pre-weaning BW (SMD = 0.81; 95% CI= 0.06, 1.57; I2 = 81%), although significant heterogeneity was present (see Fig. 4). Further exploration by subgroup analysis showed an increase in pre-weaning BW of animals when the males and females were analysed together, compared to males and females analysed separately. An increase was also seen in rats of unknown strain compared to Sprague-Dawley and Wistar rats. It should be noted, the two data sets that did not report strain were extracted from the same publication. Reference Ozkan, Topsakal and Ozmen29 No effect was detected on pre-weaning offspring BW for concentration. See Supplementary Fig. S8 for sub-group analysis.
Table 2. Summary of offspring anthropometric outcome measures. Reported outcomes extracted include offspring bodyweight at birth, the pre-weaning period and adulthood. Also included were body composition outcomes

GD – gestational day 21; N – sample size; NR – not reported; PND – postnatal day; PC – pre-conception; PND – postnatal day; SD – Sprague-Dawley; WS – Wistar; % w/v – concentration percentage weight per volume; – outcome not measured; ↑ – increase; ↓ – decrease; ♂ - male; ♀ - female.
a In female interventional group exposed to sucrose during gestation period only (cross-fostering model).
b In female interventional group exposed to sucrose during lactation period only (cross-fostering model).
c In female interventional group exposed to sucrose during gestation and lactation periods (cross-fostering model).
d In male interventional group exposed to sucrose during gestation period only (cross-fostering model).
e In male interventional group exposed to sucrose during lactation period only (cross-fostering model).
f In male interventional group exposed to sucrose during gestation and lactation (cross-fostering model).
∞ Cross-fostering model.

Fig. 4. Offspring body weight weaning.
BW data were available for 323 adult offspring. When maternal sucrose consumption and the impact on BW in adult offspring rats were analysed, a significant increase in BW (SMD = 0.47; 95% CI= 0.13, 0.81; I2 = 36%) (Fig. 5a) was found with low heterogeneity. Further exploration by subgroup analysis did not show a clear effect of sex or concentration, but the increase in BW may be specific to Sprague-Dawley rats or when dams were exposed to sucrose post conception rather than prior to mating (Fig. 5b-e). Variability in the timing of BW measurement ranged from 56 to 504 days of age and, according to meta-regression modelling, the effect on BW did not depend on age, p = 0.5944.

Fig. 5. (a) Offspring body weight adult. (b) Offspring body weight adult sex. (c) Offspring body weight adult concentration. (d) Offspring body weight adult strain. (e) Offspring body weight adult exposure.
Only two papers reported data on adiposity of offspring, assessed directly by resection of fat pads Reference Toop, Muhlhausler, O’Dea and Gentili30 or total body fat Reference Kisioglu and Nergiz-Unal38, and their results differed. Toop et al. Reference Toop, Muhlhausler, O’Dea and Gentili30 reported decreases in female retroperitoneal fat mass and male total fat mass in 12-week-old rats exposed to maternal sucrose consumption during a 4-week preconception period, pregnancy or lactation. In contrast, Kisioglu et al. Reference Kisioglu and Nergiz-Unal38 observed an increase in offspring total body fat at the end of weaning. Although in this study, maternal sucrose exposure was significantly longer, starting 12 weeks prior to conception and finishing at the end of lactation. Furthermore, the data were not separated by sex. Reference Kisioglu and Nergiz-Unal38
Relationship between maternal sucrose consumption and offspring FGLs, glycaemic control and TGs
Twelve papers provided data for fasting blood or plasma glucose concentration in offspring measured between the pre-weaning and adult period (Table 3). Thirteen experimental data sets were included in the general meta-analysis in adult offspring measured at PND84–616, with no effect observed (SMD = 0.32; 95% CI= −0.18, 0.82; I2 = 55%); see Fig. 6. Seven of these papers provided additional data on glucose control, as indicated by static assessment of fasting plasma insulin levels, Reference Ozkan, Topsakal and Ozmen29,Reference Toop, Muhlhausler, O’Dea and Gentili30,Reference Zhang, Zhu and Zhang39,Reference Yuruk and Nergiz-Unal43,Reference Toop, Muhlhausler, O’Dea and Gentili49 Homeostatic Model of Assessment of Insulin Resistance (HOMA-IR) Reference Zhang, Zhu and Zhang39,Reference He, Zhang and Yang42,Reference Yuruk and Nergiz-Unal43 and QUICKI estimates Reference Yuruk and Nergiz-Unal43,Reference Kendig, Ekayanti, Stewart, Boakes and Rooney48 or by dynamic assessment of whole body glucose tolerance through intraperitoneal Reference Toop, Muhlhausler, O’Dea and Gentili30,Reference Zhang, Zhu and Zhang39,Reference He, Zhang and Yang42 or oral Reference Kendig, Ekayanti, Stewart, Boakes and Rooney48 GTT. There was no significant effect on glucose disposal in offspring exposed to maternal sucrose consumption compared to control offspring aged 3 weeks, Reference Toop, Muhlhausler, O’Dea and Gentili30,Reference Yuruk and Nergiz-Unal43 12 weeks Reference Toop, Muhlhausler, O’Dea and Gentili30 and approximately 13 weeks old. Reference Kendig, Ekayanti, Stewart, Boakes and Rooney48 In one study where male offspring who presented with a normal oral GTT at 13 weeks and were subsequently were offered sucrose for the following seven weeks, their QUICKI Index was elevated compared to controls. Reference Kendig, Ekayanti, Stewart, Boakes and Rooney48 Further evidence for metabolic programming in male offspring was provided by Zhang et al. Reference Zhang, Zhu and Zhang39 , who reported hyperglycaemia at the 30-min time point during an intraperitoneal GTT in 4-month-old rats; however, HOMA-IR levels were unchanged. When intraperitoneal GTT was retested at 6 months of age, there was no longer any effect. Furthermore, He et al. Reference He, Zhang and Yang42 observed hyperglycaemia after 30-min during an intraperitoneal GTT, hyperinsulinaemia and elevated HOMA-IR levels for aged offspring (22 months).
Table 3. Summary of offspring glycaemic outcome measures. Reported outcomes extracted include offspring fasting plasma or blood glucose levels, fasting plasma insulin levels and other assessments for glycaemic control

FGL – fasting glucose level; FPI – fasting plasma insulin; G – gestation; IPGTT – intraperitoneal glucose tolerance test; N – sample size; NR – not reported; OGTT – oral glucose tolerance test; PND – postnatal day; PC – pre-conception; PND – postnatal day; SD – Sprague-Dawley; WS – Wistar; % w/v – concentration percentage weight per volume; – outcome not measured; ↑ – increase; ↓ – decrease; ♂ - male; ♀ - female.
a In female interventional group exposed to sucrose during gestation period only (cross-fostering model).
b In female interventional group exposed to sucrose during lactation period only (cross-fostering model).
c In female interventional group exposed to sucrose during gestation and lactation periods (cross-fostering model).
d In male interventional group exposed to sucrose during gestation period only (cross-fostering model).
e In male interventional group exposed to sucrose during lactation period only (cross-fostering model).
f In male interventional group exposed to sucrose during gestation and lactation (cross-fostering model).
† In male interventional group when sucrose was added to their diet from PND91 to 142 compared to control offspring.
∞ Cross-fostering model.

Fig. 6. Offspring BGL adult.
Only four papers reported data on liver and/or plasma TG concentrations. Reference Toop, Muhlhausler, O’Dea and Gentili30,Reference Bocarsly, Barson and Hauca31,Reference Zhang, Zhu and Zhang39,Reference Yuruk and Nergiz-Unal43 Due to limited data, a meta-analysis could not be conducted. No effect was observed in plasma and/or liver TGs in rats aged PND21 to PND180. Reference Toop, Muhlhausler, O’Dea and Gentili30,Reference Bocarsly, Barson and Hauca31,Reference Zhang, Zhu and Zhang39 In contrast, Yuruk et al. Reference Yuruk and Nergiz-Unal43 reported significantly higher levels for blood and liver TG concentrations measured at weaning. A full summary of offspring glycaemic outcomes is shown in Table 3.
Relationship between maternal sucrose consumption and offspring food and fluid intake
Food and fluid intake was seldom reported in offspring outcomes. Four papers reported no significant change in chow consumption for offspring exposed compared to control offspring. Reference Toop, Muhlhausler, O’Dea and Gentili30,Reference Zhang, Zhu and Zhang39,Reference He, Zhang and Yang42,Reference Kendig, Ekayanti, Stewart, Boakes and Rooney48 Similarly, water consumption did not differ between groups, as reported in two papers Reference Zhang, Zhu and Zhang39,Reference He, Zhang and Yang42 and a third observed no significant difference in sucrose consumption when 13-week-old males were given ad libitum access to 10% w/v sucrose for 7 weeks. Reference Kendig, Ekayanti, Stewart, Boakes and Rooney48
Study quality assessment
Risk of bias
Results from the assessment of risk of bias are presented in Table 4. The results are presented for individual papers, and each item was assigned either low risk, high risk or unclear risk. In summary, risk of bias for the majority of papers was judged unclear. Questions 1–3 related to selection bias attributed to a lack of randomisation, baseline characteristics or concealment. Twelve of the included papers reported randomising animals to groups, yet failed to adequately describe the methods (e.g. random number generator) and therefore were judged unclear. Three papers failed to describe randomisation at all. Reference Ozkan, Topsakal and Ozmen29,Reference Bocarsly, Barson and Hauca31,Reference Yuruk and Nergiz-Unal43 Dam age and weight at the commencement of the study were considered necessary to determine whether groups were comparable, yet only six papers reported both (Q2). Concealment of the investigator to group allocation (Q3) was not reported by any papers (0/15). Similarly, randomisation of housing (0/15 papers) and blinding of caregivers (1/15 papers) were poorly performed. For detection bias, two papers reported randomly selecting animals for outcomes assessment (Q6) and blinding the assessor during outcome measurements (Q7). Data exclusion due to adverse events was addressed in three papers (Q8). The majority of studies (13/15) were assessed as free of selective outcome reporting by comparing methods and results within the paper (Question 9). Litter as a unit of analysis was considered if either (a) one animal was randomly selected to represent the litter including situations where one animal of each sex was used, (b) litter mean was used for analysis or (c) if litter was a random factor in mixed models analysis. Only five papers were judged free of using incorrect unit of analysis causing unjustly inflated precision (Q10).
Table 4. Summary of risk of bias assessment according to SYRCLE’s tool (Hooijmans et al., 2014). Results are presented for individual papers with questions judged as ‘low’ risk of bias, ‘high’ risk of bias or ‘unclear’ if unable to clearly assign risk, according to pre-defined signalling questions (hoojimans). Five types of bias and assessment of litter as a unit of analysis are included for assessment

Reporting adherence to ARRIVE guidelines checklist
The percentage of studies fully, partially or not reporting the 20-item checklist can be seen in Supplementary Fig. S9. In general, we observed low to moderate adherence to the guidelines for the included papers. Four items were assessed as 100% FR, including title, ethical statement, defining experimental outcomes in methods and reporting of each analysis with a measurement precision. Reporting of the abstract and background were judged as PR if the descriptor was not fully met (FR 47%; PR 53%). This was commonly observed when papers did not report species, strain or background summary in the abstract and human relevance in the introduction. Study design was not well reported (100% PR) due to an absence of blinding and reporting of litter or individual animal as unit of analysis. All papers PR detail on housing and husbandry (100% PR), with cage, bedding and housing type frequently omitted. Only two papers fully described sample size by inclusion of calculation or power analysis (13% FR; 73% PR; 13% NR). The majority of papers did not report adequate randomisation techniques and allocation to treatment groups (13% PR; 87% NR). Many failed to include testing for normality in statistical analyses (33% FR; 60% PR; 7% NR). Baseline data required for this review were maternal age and weight, with 5 out of 15 papers reporting both. Study limitations were described occasionally; however, no paper discussed scientific implications of their findings for the 3R’s in animal research (100% PR), and translation to human populations was considered in just over half the included papers (53% FR; 47% NR). Individual study assessment for adherence to ARRIVE guidelines can be found in Supplementary Table S10.
Discussion
The purpose of this systematic review and meta-analysis was to identify relationships between maternal sucrose consumption and the metabolic health in offspring. Importantly, for high translational relevance, we included maternal dietary interventions where sucrose concentration closely resembled that of SSBs consumed by humans. In total, we included 15 studies for review and quantitatively synthesised data from 184 dams and 323 offspring in rat models.
Results indicate maternal sucrose consumption prior to conception and during prenatal periods did not affect maternal BW. However, a significant increase in the BW of the adult offspring was identified. A sex effect was not evident in sub-group analyses, although this conclusion was limited by a paucity of female data. Sex differences have been reported in developmental programming, although they are not commonly explored. Reference Dearden, Bouret and Ozanne50,Reference Aiken and Ozanne51 Literature suggests that male offspring may be more susceptible to programming of adiposity and BW than females, with the protective actions of oestrogen, maternal and paternal epigenetics and sex disparities in placental function possible mechanisms. Reference Dearden, Bouret and Ozanne50-Reference Li, Tsuprykov, Yang and Hocher53 It is clear future studies must include female and male offspring to elucidate if sexual dimorphism exist.
A more accurate assessment of offspring adiposity can be obtained from changes in body composition rather than simple BW measurements. The latter may not indicate the contribution of prenatal food manipulation to abdominal fat. Reference Eleftheriades, Vafaei and Dontas54 Toop et al. Reference Toop, Muhlhausler, O’Dea and Gentili30 reported lower retroperitoneal adipose tissue in female adult offspring exposed to sucrose during pregnancy and lower relative total fat and visceral fat mass in adult males exposed during lactation. This was despite having similar BW to control offspring. This decrease in fat mass contrasts to the findings of Kisoglu et al. Reference Kisioglu and Nergiz-Unal38 who reported higher total body fat assessed by Soxhlet extraction, along with increased BW in 3-week-old pups, although sex was not considered in these data. In general, differing results limit our ability to interpret the potential influences prenatal sucrose exposure may have on offspring adiposity.
Hyperglycaemia and reduced glucose tolerance were evident in dams, particularly at the higher concentration of 20% w/v; however, meta-analyses did not reveal a change in the FGLs of adult offspring. When analysing outcomes for glycaemic control, we identified a number of different measures utilised, including GTT and indices from static assessment of glucose and insulin levels, such as QUICKI and HOMA-IR estimates. These were measured at varied ages in offspring. In the pre-weaning period and early adulthood, no effect was shown in male or females during OGTT Reference Kendig, Ekayanti, Stewart, Boakes and Rooney48 or intraperitoneal GTT Reference Toop, Muhlhausler, O’Dea and Gentili30,Reference Feng, Zhou and Zhang40 or in a study with combined sexes using HOMA-IR and QUICKI estimates. Reference Yuruk and Nergiz-Unal43 It was only when assessing older male rats, from 4 to 18 months in age, that reduced glucose tolerance was observed with Reference He, Zhang and Yang42 and without Reference Zhang, Zhu and Zhang39 hyperinsulinaemia. Of note, Zhang et al. Reference Zhang, Zhu and Zhang39 found this effect was normalised when the 4-month-old offspring were re-assessed at 6 months and their data show the transient nature of glycaemic homoeostasis. Overall, results suggest that prenatal sucrose exposure did not impact the FGLs or glycaemic control in younger offspring, and it was not until rats aged that poor glucose disposal became evident. However, in many cases, prenatal exposure was confounded with an altered maternal metabolic state at the outset of pregnancy.
At the outset of this review, we included secondary behavioural outcomes for food and fluid intakes and sweet taste preferences to determine if maternal sucrose consumption elicits hyperphagia or modulate taste preferences in offspring. While consumption was not frequently reported, studies that did report such data found no effect on offspring intakes. Reference Toop, Muhlhausler, O’Dea and Gentili30,Reference Zhang, Zhu and Zhang39,Reference He, Zhang and Yang42,Reference Kendig, Ekayanti, Stewart, Boakes and Rooney48 No study investigating sweet preference in offspring at relevant sucrose concentrations was identified.
Cross-fostering is a valuable research tool for providing insights into the effects of sucrose exposure during crucial time-periods, i.e. lactation or pregnancy. Reference Dickinson, Moss and Gatford55 Two studies utilised this approach and both found that offspring effects were dependent on the exposure window. Reference Toop, Muhlhausler, O’Dea and Gentili30,Reference Bocarsly, Barson and Hauca31 Some over-nutrition models suggest lactation to be a critical time-point, displaying developmental programming of obesity. Reference Ribaroff, Wastnedge, Drake, Sharpe and Chambers13,Reference Lagisz, Blair and Kenyon56,Reference Alfaradhi and Ozanne57 Bocarsly et al. results support this suggestion, finding adult males were heavier in the lactation group compared to control and pregnancy groups. Reference Bocarsly, Barson and Hauca31 Although Toop et al. observed no change to BW, exposure during the lactation period had a greater impact on adipose tissue deposition. When animals were exposed during pregnancy and lactation, poorer metabolic fatty acid profiles were also evident. Reference Toop, Muhlhausler, O’Dea and Gentili30 Notwithstanding differences between the development of human and rodent models, these results suggest health recommendations should highlight the importance of reducing SSB consumption not only during pregnancy but also throughout the lactation period.
The current review allows for exploration of mechanisms between maternal sucrose consumption and developmental programming of offspring. Some studies suggest the dysregulation of insulin signalling pathways and lipogenesis may play a role in programming offspring phenotypes. Reference Ozkan, Topsakal and Ozmen29,Reference Toop, Muhlhausler, O’Dea and Gentili30,Reference Zhang, Zhu and Zhang39,Reference Yuruk and Nergiz-Unal43 Zhang et al. reported a downregulation of mRNA expression of key hepatic insulin signalling molecules IRS-1, Akt and GSK-3β, although protein expression remained unchanged. Reference Zhang, Zhu and Zhang39 Adult offspring also displayed reduced glucose tolerance and enlarged pancreatic islets. Supporting these results, Ozkan et al. observed damage to offspring pancreatic tissue, with pups showing decreased insulin secretion and insulin receptor expression, worsening with higher sucrose concentrations. Reference Yuruk and Nergiz-Unal43 Altered adipocyte programming was suggested for changes to adipose deposits in offspring with concomitant elevation of plasma free fatty acids (FFA) concentration and hepatic lipid content. Reference Toop, Muhlhausler, O’Dea and Gentili30 Yuruk et al. also found maternal sucrose consumption had a significant effect on increasing hepatic and liver TG and circulating NEFA levels at weaning. Other mechanisms in programming of obesity were explored, including altered appetite regulation Reference Kisioglu and Nergiz-Unal38 and sensitisation of reward pathways. Reference Bocarsly, Barson and Hauca31 Kisogulu et al. found elevated offspring BWs were associated with dysregulation of satiety peptides leptin and ghrelin, although free fructose appeared to have a more significant effect than sucrose. Reference Kisioglu and Nergiz-Unal38 Of note, this study did not report offspring food intake. From the limited data on offspring food or fluid intake, no effect from maternal sucrose consumption was seen. Reference Toop, Muhlhausler, O’Dea and Gentili30,Reference Zhang, Zhu and Zhang39,Reference He, Zhang and Yang42,Reference Kendig, Ekayanti, Stewart, Boakes and Rooney48 Taken together, a possible interplay of programming in metabolic pathways and appetite regulation may have detrimental effects on offspring phenotypes in rat models. Whether these mechanisms differ between rat and human systems remain unclear.
A fundamental reason for performing animal studies is to translate the outcomes to human health. There are calls amongst the academic community that rodent experimentation should more closely mimic the dietary and feeding behaviours of humans, Reference Speakman58 although some studies do not adhere to this principle. In our recent systematic review investigating metabolic and behavioural effects of prenatal exposure to non-nutritive sweeteners (NNS), we found many studies provided animals with NNS dosages that were not physiologically relevant to human consumption, hampering translational capacity. Reference Morahan, Leenaars, Boakes and Rooney37 The current review builds upon this research by only including sucrose concentrations found in commercially available SSBs. Nevertheless, it is important to acknowledge other factors can limit rodent-to-human translation when investigating maternal high-sugar diets. For example, overdrinking of sucrose solution with a compensatory reduction in chow intake was commonly observed in dams. Reference Kendig, Ekayanti, Stewart, Boakes and Rooney48,Reference Toop, Muhlhausler, O’Dea and Gentili49 In this case, it is possible that the observed offspring effects may be impacted by maternal protein restriction rather than, or in addition, to the excess sugar. Protein undernutrition appears to be important in the programming of metabolic disorders. Reference Fernandez-Twinn, Wayman and Ekizoglou59 Given the majority of pregnant women exceed the minimum recommendations for protein intake, 60 one must question whether this rodent model adequately reflects human consumption patterns.
While the effects identified in this review are not as pronounced as those reported for offspring exposed during prenatal life to high-fat/high-sugar diets, Reference Ainge, Thompson, Ozanne and Rooney12,Reference Ribaroff, Wastnedge, Drake, Sharpe and Chambers13,Reference Tellechea, Mensegue and Pirola20 it is apparent the isolated effects of sucrose may contribute to metabolic developmental programming. Thus, recommendations to reduce intake of SSBs prior to conception and during pregnancy remain valid.
Strengths and limitations
Common practice in research of developmental programming is to target outcomes in males only. Reference Ainge, Thompson, Ozanne and Rooney12,Reference Desai, Beall and Ross15,Reference Vesterinen, Sena and Egan32 This is evident in our review; over two-thirds of the offspring investigated were male. A number of studies reported results for both sexes combined together. Reference Ozkan, Topsakal and Ozmen29,Reference Yuruk and Nergiz-Unal43 Sexual dimorphism has frequently been observed for both human and animal metabolic programming; Reference Ravelli, van der Meulen, Osmond, Barker and Bleker61,Reference Fuente-Martín, Granado and Garcia-Caceres62 however, limited data meant that no firm conclusions could be reached on sex-specific differences. Future studies should include both males and females in their study design, with analyses exploring sex effect and sex by treatment interactions.
A comprehensive assessment of the study and reporting quality identified a high risk of bias and low or moderate adherence of reporting guidelines for many of the included studies. Generally, studies lacked appropriate reporting on randomisation and blinding techniques. This drawback has the potential to affect the internal bias of a study and may promote overestimation of the effects of the intervention. Reference Macleod, van der Worp, Sena, Howells, Dirnagl and Donnan63 A further concern, relevant to rodents having multiparous births, is the effect of litter on statistical analysis. For conclusions to be valid, each dam and its litter should be considered one experimental unit. If individual offspring are counted as its own experimental unit, this gives rise to an increase in Type 1 errors. Reference Ribaroff, Wastnedge, Drake, Sharpe and Chambers13,Reference Lazic and Essioux64 In over two-thirds of the studies, the experimental unit was not made clear. As such, we could not determine if statistical analyses in these studies used inflated sample sizes, which may lead to false-positive results.
The strengths of this review include detailed assessment of the quality of the studies it covers and meta-analyses, with sub-group analyses to explore heterogeneity in study design. Importantly, this review offers insights for translational relevance by including sucrose concentrations that mimic aspects of the human diet when consuming SSBs.
Conclusion
Sucrose consumption prior to conception or during pregnancy, at concentrations similar to that of SSBs, elicits hyperglycaemia and adiposity with no change to bodyweight in dams. A paucity of female offspring data limited our capacity to identify potential sexually dimorphic responses in body composition and glycaemic control; therefore, future studies must include both sexes. We also revealed study design and reporting weaknesses, predisposing many of the papers to bias. Although our data did not mirror results from epidemiological studies for significant risks of childhood obesity, our review identified a risk of obesity and poor glucose control in adult offspring. These effects were not as pronounced as those obtained from research using maternal high-fat/high-sugar diets. Current recommendations to reduce consumption of SSBs during pregnancy and lactation remain valid, not only for the metabolic health of mothers but also for that of future generations.
Supplementary mterial
For supplementary material for this article, please visit https://doi.org/10.1017/S2040174420000823
Acknowledgements
The authors wish to acknowledge Monica Cooper (Academic Liaison Librarian, University of Sydney) for her assistance with formulating search strategies and online database searches. The authors thank Rachel Adams (RA) for her assistance with Risk of Bias assessment.
Financial support
This research received no specific grant from any funding agency, commercial or not-for-profit sectors.
Conflicts of interest
None.
Ethical standards
The authors assert this research was conducted and reported in accordance with the Preferred Reporting Items for Systematic Review and Meta-Analyses (PRISMA) Statement.