Introduction
Epidemiological and animal studies have demonstrated that environmental and nutritional changes during pregnancy and lactation may initiate permanent changes in metabolism that lead to several diseases later in life.Reference Barker1–Reference Franzago, Fraticelli, Stuppia and Vitacolonna3 This phenomenon, known as metabolic programming, involves epigenetic changes during critical windows of development and plays an important role, for example, in the onset of obesity.Reference Agosti, Tandoi, Morlacchi and Bossi4
Breastfeeding is considered a critical period of development since milk is the first source of nutrition for the baby after birth. A child is considered exclusively breastfed only when breast milk is ingested with no intake of any other food or drink for the first 6 months of life.5 Introduction of any food before 6 months of age characterizes as early weaning.Reference Harder, Bergmann, Kallischnigg and Plagemann6 Studies have shown that alterations during the lactation period, even if transitory, are related to late-emerging metabolic disturbances.Reference de Albuquerque Maia, Lisboa and de Oliveira7–Reference Kikusui, Kanbara and Ozaki9 Our research group developed an animal model of early weaning and observed, in males, hyperphagia, overweight, increased visceral adiposity, and resistance to leptin in the adult offspring.Reference Lima Nda, de Moura and Passos10–Reference Lima Nda, Franco and Peixoto-Silva12 In addition, hepatic alterations were detected, with increased triglycerides and microsteatosis associated with increased oxidative stress.Reference Franco, Lisboa and Lima13 Early-weaned females also exhibited hyperphagia and higher adiposity, despite having normal body mass.Reference Pietrobon, Bertasso and Silva14 A sexual dimorphism seems to be involved in these phenotypes, since we have already shown that females differ from males in parameters such as hormonal profiles, hepatic lipid metabolism, brown adipose tissue function, and fat deposit distribution.Reference Souza, de Moura and Lisboa15
It is known that obesity is a multifactorial disease that may be associated with the development of several other metabolic diseases and is considered a major epidemic in the 21st century.Reference Williams, Mesidor, Winters, Dubbert and Wyatt16 Alterations in the endocannabinoid system (ECS) have been seen as one of the mechanisms capable of regulating aspects related to hyperphagia and greater storage of calories, playing a crucial role in the regulation of energy balance.Reference Quarta, Mazza, Obici, Pasquali and Pagotto17,Reference Watkins and Kim18 Likewise, the dopaminergic system can control the search for palatable food and rewardReference Alsio, Olszewski and Norback19,Reference Volkow, Wise and Baler20 ; the excess of fat and sugar in foods can activate central pathways that are similar to those triggered by drug addiction.Reference Volkow, Wise and Baler20 Therefore, alterations of the endocannabinoid and dopaminergic systems have been associated with the development of binge-eating and obesity.Reference Volkow, Wise and Baler20–Reference Kessler, Hutson, Herman and Potenza24
Type 1 (CB1r) and type 2 (CB2r) cannabinoid receptors are distributed throughout most of central nervous system (CNS) and in various peripheral organs, such as the white adipose tissue (WAT) and liver.Reference Hu and Mackie25–Reference Bazwinsky-Wutschke, Zipprich and Dehghani27 N-arachidonoyl ethanolamine (anandamide) and 2-arachidonoylglycerol (2-AG) are endogenous cannabinoids derived from arachidonic acid that control energy and lipid metabolism.Reference Di Marzo, De Petrocellis and Bisogno28,Reference Di Marzo29 Anandamide is produced by the enzyme N-arachidonyl-phosphatidylethanolamine phospholipase-D (NAPE-PLD), whereas 2-AG is generated by the action of diacylglycerol-lipase (DAGL). The anandamide is metabolized by fatty acid amide hydrolase (FAAH), whereas the 2-AG is hydrolyzed by monoacylglycerol lipase (MAGL).Reference Di Marzo29 In the lateral hypothalamus (LH), the ECS function is to integrate signals that transmit information about the energy state of the organism, causing increased food intake.Reference Sanchez-Fuentes, Marichal-Cancino and Mendez-Diaz30–Reference Jo, Chen, Chua, Talmage and Role32 In the WAT, the ECS stimulates lipogenesis and adipogenesisReference van Eenige, van der Stelt, Rensen and Kooijman33 and, in the liver, the ECS is involved in the development of acute and chronic hepatic diseases.Reference Siegmund and Schwabe34
The mesolimbic and mesocortical dopaminergic pathways influence appetite, motivating the search for food.Reference de Macedo, de Freitas and da Silva Torres35,Reference Bassareo and Di Chiara36 This dopaminergic system consists of neurons located in the ventral tegmental area (VTA), which release dopamine by activating the dopaminergic receptors (D1r and D2r) in regions of the brain such as the nucleus accumbens (NAc).Reference Hoebel, Hernandez, Schwartz, Mark and Hunter37,Reference Hanlon, Baldo, Sadeghian and Kelley38 On the other hand, in the arcuate nucleus (ARC) of the hypothalamus, dopamine signaling inhibits feeding.Reference Liang and Pan39 Endocannabinoids directly and indirectly modulate dopaminergic function.Reference Bloomfield, Ashok, Volkow and Howes40
Therefore, considering the hyperphagia and other metabolic dysfunctions associated with obesity previously detected in both sexes of an animal model of early weaning,Reference Lima Nda, de Moura and Passos10–Reference Lima Nda, Franco and Peixoto-Silva12,Reference Pietrobon, Bertasso and Silva14 we hypothesized that early-weaned animals could show a higher preference for palatable diets due to dysfunctions of both dopaminergic and ECSs in adult life and that such changes could be tissue- and sex-dependent. In the current study, early-weaned offspring of both sexes were challenged by offering a choice between a diet rich in fat and one rich in sucrose. Furthermore, the dopaminergic system was evaluated in the VTA, prefrontal cortex (PFC), NAc, dorsal striatum (DS), and ARC, and ECS parameters were assessed in the LH, adipose tissue, and liver.
Methods
Experimental model
The Ethics Committee for the Care and Use of Experimental Animals of the Institute of Biology Roberto Alcantara Gomes of UERJ approved this experiment (CEUA/035/2017). All efforts were made to minimize the number of animals used and to refine the procedures. Animals were kept in a room under controlled temperature (21 ± 2°C), humidity (55% ± 5%), and light–dark cycle (9:00 a.m./9:00 p.m.), with free access to water and food. At 90 days of age, nulliparous Wistar rats were mated with males (2 females/1 male) for 7 days. After mating, each female was placed in an individual cage with free access to water and food until delivery. After birth, the litters were adjusted to 6 pups (3 males and 3 females) per lactating rat dam and randomly assigned to one of the following two groups: the control group (CONT, n = 7), that is, dams whose pups had a standard lactation period (21 days), and the early-weaning group (EW, n = 7), in which dams were slightly anaesthetized and wrapped with a bandage to prevent pups from accessing teats during the last 3 days of lactation, as described in our previous study.Reference Lima, Moura and Franco11 It is important to highlight that a period of 21 days of lactation for rats corresponds to 6 months of lactation for an infant, and thus considering this information, our experimental model mimics a child weaned at the 5th month of age.Reference Quinn41 Both groups received a standard rodent chow diet (Nuvilab®, São Paulo, Brazil) and potable water ad libitum.
Food intake and body mass
During lactation, the body mass of the EW and CONT pups was monitored daily until postnatal day 21 (PN21). After PN21, body mass, and food intake were assessed every 3 days until PN180 in both groups. Animals were housed in groups of three or four same-sex siblings per cage throughout experiment. The amount of food intake (in grams) was estimated by calculating the difference between the quantity of chow initially placed in the cage and the final amount of chow left in the cage three days later and then dividing this result by the number of animals in the cage.
Evaluation of feeding behavior and diets composition
At PN175, the animals were submitted to the food challenge test during the night,Reference Pinheiro, Moura and Manhaes42,Reference Pinheiro, Moura and Manhaes43 which is the active period for rodents. During the food challenge period, animals were kept in the same cage in order to avoid the effects of social isolation.
First, the animals were fasted for 12 h, from 9 a.m. until 9 p.m., after which they could select between a high-fat diet (20% higher content of saturated fat compared to the standard chow) and a high-sugar diet (38% higher content of sucrose compared to the standard chow). Food intake was first recorded at 9:30 p.m.; thus, voracity was determined by calculating the amount of chow that was consumed during the initial 30-min period. Voracity was used as a measure of excessive appetite, a behavior that shows similarities to drug craving.Reference Avena, Long and Hoebel44–Reference Avena, Rada and Hoebel47 On the next day (9 a.m.), food intake was recorded by calculating the amount of chow that was consumed during the previous 12 h. After the food challenge, all animals received standard chow.
The palatable diets were prepared in our laboratory using a standard commercial diet for rodents (Nuvilab CR-1, Nuvital Nutrientes S/A – Quimtia, PR, Brasil). The standard chow was grinded and the resulting bran was mixed with butter or sugar by using an industrial food mixer. To prepare 100 g, we added 20 g of butter to 62 g of standard chow for the preparation of the high-fat diet and 38 g sucrose to 62 g of standard chow for the preparation of the high-sugar diet. We also added 18 g of sucrose in the high-fat diet. When the mix achieved a good consistence to be pelletized, it was placed in an industrial heater for 12 h to dry. The chows were kept at room temperature. The diets were placed in the housing cage cover in a proper space for the food. To separate the two diets, we used a bottle with drinking filtered water. The texture and shape of the chow pellets of the palatable diets were similar to those of the standard diet. The compositions of the palatable diets are shown in Table 1.
Table 1. Composition of the standard and palatable diets

Values observed in 100 g of rat chow.
Euthanasia and tissue collection
Prior to euthanasia, animals were fasted overnight. At PN180, immediately before euthanasia, the animals’ body masses were measured. At 8 a.m., a lethal dose of thiopental was injected, and blood was obtained by cardiac puncture. The blood was collected in the heparin tube and centrifuged (663 × g, 25 min, 4°C). The plasma was stored at −20°C. The brain (for extraction of hypothalamus (LH, ARC), VTA, PFC, NAc, and DS), visceral adipose tissue (VAT), and liver were collected and stored at −80°C for further procedures. Coronal brain sections were cut using a cryostat (Hyrax C25, Zeiss, Germany) and punches of the LH (−2.10 to −3.6 mm), DS (Bregma 2.16–0.96 mm), nucleus accumbens (NAc, Bregma 2.16–0.96 mm), ARC of hypothalamus (ARC, Bregma −2.1 to −3.6 mm), PFC (Bregma 4.20–2.52 mm), and VTA (Bregma −5.64 to −6.36 mm) were extracted from the brains.Reference Paxinos, Watson and Emson48
Western blot analysis
In the LH, VAT, and liver, we measured the following biomarkers of ECS: NAPE-PLD, FAAH, DAGL, MAGL, CB1r, and CB2r. Dopaminergic system biomarkers were evaluated in the following areas: 1) VTA (TH); 2) PFC, DS, and NAc (TH, DAT, D1r, and D2r); 3) ARC (D2r).
The VAT was homogenized in an extract buffer (T-PER tissue protein extraction). The liver was homogenized in ice-cold RIPA buffer (50 mM Tris-HCl [pH 7.4], 1% NP-40, 150 mM NaCl, 1 mM EDTA, 1 mM PMSF, 1 mM Na3VO4, 1 mM NaF) with a protease inhibitor cocktail (F. Hoffmann-La Roche Ltda., Basel, CH). Samples were then centrifuged at 12839.9 × g for 30 min (VAT) or 19339.5 × g for 25 min (liver). All brain nuclei were sonicated twice in an ultrasonic processor for 10 s (15 s interval, 40% amplitude) with RIPA buffer (50 mM Tris-HCl [pH 7.4], 1% NP-40, 150 mM NaCl, 1 mM EDTA, 1 mM PMSF, 1 mM Na3VO4, 1 mM NaF) with a protease inhibitor (F. Hoffmann-La Roche Ltda. Basel, CH). Protein concentrations of all tissues were determined using the Pierce BCA Protein Assay Kit (Thermo Scientific, CA, USA). Homogenates were analyzed by SDS-PAGE using 10–30 µg total protein. Proteins were then transferred from the gel to a polyvinylidene difluoride membrane (Hybond ECL; Amersham Pharmacia Biotech, London, UK) and incubated with Tris-buffered saline (TBS) containing 5% albumin for 45 min. Following this step, membranes were washed with Tween-tris-buffered saline (0.1%) and incubated overnight at 4°C with a specific primary antibody: NAPE-PLD (1:200 – ab95397, Abcam), FAAH (1:500 – ab54615, Abcam), DAGL (1:500 – ab81984, Abcam), MAGL (1:400 – sc398942, Santa Cruz Biotechnology), CB1r (1:1000 – ab23703 Abcam), CB2r (1:500 – ab3561, Abcam), TH (1:1000 – T2928, Sigma-Aldrich), DAT (1:500 – ab1591P, Millipore), D1r (1:500 – ab81296, Abcam), D2r (1:500 – ab5084P, Millipore), GAPDH (1:1000 – D16H11, Cell Signaling), and β-Actin (1:1000 – A2228, Sigma-Aldrich). Membranes were then washed three times with Tween TBS (0.1%) and incubated for 1 h with an appropriate secondary antibody conjugated with biotin (1:7000, 1:10000, or 1:20000; anti-rabbit, anti-mouse [Sigma-Aldrich MO, USA], or anti-goat [Invitrogen Corporation, CA, USA]) at room temperature. Then, the membranes were washed again three times with Tween-TBS (0.1%) and incubated with streptavidin-conjugated horseradish peroxidase (GE Healthcare, Buckingham, Shire, UK). The protein bands were visualized by a chemiluminescence kit (ClarityTM Western ECL Substrate, Bio-Rad Laboratories Inc., USA) followed by exposure to Image Quant LAS (GE Healthcare, Buckinghamshire, UK). The area and density of the bands were quantified using ImageJ software (Wayne Rasband, National Institute of Health, MD, USA) and were normalized to β-actin (Sigma-Aldrich MO, USA) or glyceraldehyde-3-phosphate dehydrogenase (GAPDH) (Cell Signaling Technology MA, USA). The results were expressed as percentages (%) of the control group.
Statistical analysis
Statistical analyses were carried out using GraphPad Prism Software version 6 for Windows (GraphPad Software, San Diego, CA, USA). The data are shown as means ± SEM. One rat of each sex per litter was randomly used for the analyses (total of EW: 7 litters; CONT: 7 litters). Results were analyzed using two-way ANOVAs with programming and sex as between-subject factors followed by the Tukey’s multiple comparisons test ( Supplementary Material ). Differences were considered significant when P < 0.05. In order to qualify the magnitude of the obtained differences, the effect size was calculated. Effect size data are provided as Eta-squared (η 2: small > 0.1, medium > 0.3, large > 0.5).
Results
Early weaning increased food intake and body mass in both sexes. EW males had higher food intake (+15%, P < 0.05, η2 = 0.73), higher body mass (+17%, P < 0.05, η2 = 0.46), as well as higher visceral adiposity (+54%, P < 0.05, η2 = 0.59) when compared to CONT males, as depicted in Table 2. These parameters also showed the same alterations in EW females when compared to CONT ones: food consumption (+5%, P < 0.05, η2 = 0.35), body mass (+17%, P < 0.05, η2 = 0.35), and visceral fat mass (+51%, P < 0.05, η2 = 0.45). These data are in accordance with the findings of our previous studies.Reference Franco, Lisboa and Lima13,Reference Pietrobon, Bertasso and Silva14
Table 2. Body and adipose tissue masses in adult rats that were early-weaned

Total body mass gain was determined between PN30 and PN180. Visceral fat was collected at PN180. Groups: CONT, control rats, weaning at PN21; EW, early-weaning rats, weaning at PN18. Values expressed as mean ± SEM; n = 7 rats/group (one per sex per litter). Two-way ANOVAs followed by the Tukey’s test.
* P < 0.05 vs. same-sex CONT group.
As shown in Fig. 1a, EW males were hyperphagic from PN63 onward. When compared with CONT males, they showed increased food intake of standard chow (+16%, P < 0.0001; Fig. 1b). EW females ate more standard chow during the entire experimental period (Fig. 1c); the area under the curve of food intake was higher (+5%, P = 0.0002, Fig. 1d) in comparison with CONT females.

Fig. 1. Food intake evolution and area under curve (AUC) of food intake of adult male (a and b) and female rats (c and d). Values expressed as means ± SEM; n = 9 rats/group (one per sex per litter); statistical difference was considered significant when P < 0.05. *P < 0.05, ***P = 0.0002; ****P < 0.0001.
Eating behavior
After 30 min of the palatable diets being offered, EW males did not consume the high-sugar diet (Fig. 2a); on the other hand, they consumed more of the high-fat diet than the CONT group (+19%, P < 0.05, η2 = 0.10; Fig. 2a). After 12 h, we observed an increase in high-sugar diet consumption in EW males (+78% vs. CONT males, P < 0.05, η2 = 0.17; Fig. 2b). Concerning the high-fat diet, EW males had less fat intake when compared with CONT animals (−9%, P < 0.05, η2 = 0.17; Fig. 2b).

Fig. 2. Eating behavior of adult male and female rats. Control Group (CONT, black bar: males; gray bar: females); early weaning (EW, white bar: males; red bar: females). Food intake (in grams) in each of the groups at PN175 during a 30-min (a and c) or a 12-h (b and d) interval of a food challenge test. Food preference was measured based on the consumption of high-sugar and high-fat diets by both CONT and EW animals. A comparison between male and female food preference is shown (e and f, high-sugar diet after 30 min and 12 h, respectively; g and h, high-fat diet after 30 min and 12 h, respectively; CONT: blue bar; EW: purple bar). Values expressed as means ± SEM; n = 5–7 rats/group (one per sex per litter); statistical difference was considered significant when P < 0.05. *P < 0.05, **P < 0.01; ***P < 0.001 ****P < 0.0001.
The EW females did not show significant differences in the preference for either the high-sugar or high-fat diets when compared to CONT ones during the food challenge test (Fig. 2c, 2d).
When we compared the consumption between diets (high-sugar vs. high-fat diets), both CONT and EW groups, regardless the sex, consumed more of the high-fat diet than of the high-sugar one (Fig. 2a–2d). When we compared sex-specific effects (Fig. 2e–2f), during both the 30-min and 12-h periods, CONT and EW males ate more of the high-fat diet than CONT and EW females. Females consumed more of the high-sugar diet than males during both the 30-min and 12-h periods.
Biomarkers of dopaminergic system
We did not observe significant differences in the parameters of the dopaminergic system in the VTA (Fig. 3a), PFC (Fig. 3c), and ARC (Fig. 4b) in EW males. We found a decrease in D2r in the NAc (−29%, P < 0.05, η2 = 0.26; Fig. 3b) and DS (−25%, P < 0.05, η2 = 0.19; Fig. 4a) in EW males when compared to CONT males.

Fig. 3. Dopaminergic system I in male rats. Control Group (CONT: black bar); early weaning (EW: white bar). Ventral tegmental area (a), nucleus accumbens (b), and prefrontal cortex (c). Tyrosine hydroxylase (TH), dopamine transporter (DAT), and dopamine receptors (D1r and D2r). Representative Western blotting bands are shown. Values expressed as means ± SEM; n = 5–7 rats/group (one per sex per litter); statistical difference was considered significant when *P < 0.05.

Fig. 4. Dopaminergic system II in male rats. Control Group (CONT: black bar); early weaning (EW: white bar). Dorsal striatum (a) and arcuate nucleus (b). Tyrosine hydroxylase (TH), dopamine transporter (DAT) and dopamine receptors (D1r and D2r). Representative Western blotting bands are shown. Values expressed as means ± SEM; n = 5–7 rats/group (one per sex per litter); statistical difference was considered significant when *P < 0.05.
Regarding females, the EW group had lower TH in the VTA (−47%, P < 0.05, η2 = 0.25; Fig. 5a) and NAc (−43%, P < 0.05, η2 = 0.17; Fig. 5b), as well as lower D1r in the NAc (−66%, P < 0.05, η2 = 0.46; Fig. 5b) and lower D2r in the PFC (−39%, P < 0.05, η2 = 0.37; Fig. 5c). EW females had no changes in dopaminergic parameters in the DS (Fig. 6a) and ARC (Fig. 6b) when compared to CONT females.

Fig. 5. Dopaminergic system I in female rats. Control Group (CONT: gray bar); early weaning (EW: red bar). Ventral tegmental area (a), nucleus accumbens (b) and prefrontal cortex (c). Tyrosine hydroxylase (TH), dopamine transporter (DAT) and dopamine receptors (D1r and D2r). Representative Western blotting bands are shown. Values expressed as means ± SEM; n = 5–7 rats/group (one per sex per litter); statistical difference was considered significant when *P < 0.05.

Fig. 6. Dopaminergic system II in female rats. Control Group (CONT: gray bar); early weaning (EW: red bar). Dorsal striatum (a) and arcuate nucleus (b). Tyrosine hydroxylase (TH), dopamine transporter (DAT) and dopamine receptors (D1r and D2r). Representative Western blotting bands are shown. Values expressed as means ± SEM; n = 5–7 rats/group (one per sex per litter); statistical difference was considered significant when *P < 0.05.
Biomarkers of ECS in the lateral hypothalamus (LH)
In the LH of males (Fig. 7a), FAAH and MAGL protein contents were lower in the EW group (vs. CONT males; respectively −68%, P < 0.05, η2 = 0.29 and −50%, P < 0.05, η2 = 0.36).

Fig. 7. Endocannabinoid system in male rats. Control Group (CONT: black bar); early weaning (EW: white bar). Lateral hypothalamus (a), Visceral adipose tissue (b), and liver (c). N-arachidonoyl-phosphatidylethanolamine phospholipase-D (NAPE-PLD); fatty acid amide hydrolase (FAAH); diacylglycerol lipase (DAGL), monoacylglycerol lipase (MAGL), cannabinoid receptor (CB1r and CB2r). Representative Western blotting bands are shown. Values expressed as means ± SEM; n = 5–7 rats/group (one per sex per litter); statistical difference was considered significant when *P < 0.05.
Concerning the EW females (Fig. 8a), we observed decreased NAPE-PLD content (−55% vs. CONT females, P < 0.05, η2 = 0.21) and increased FAAH content (+84% vs. CONT females, P < 0.05, η2 = 0.38) in the LH. We did not detect any change in DAGL, CB1r, or CB2r between the female groups.

Fig. 8. Endocannabinoid system in female rats. Control Group (CONT: gray bar); early weaning (EW: red bar). Lateral hypothalamus (a), Visceral adipose tissue (b), and liver (c). N-arachidonoyl-phosphatidylethanolamine phospholipase-D (NAPE-PLD); fatty acid amide hydrolase (FAAH); diacylglycerol lipase (DAGL), monoacylglycerol lipase (MAGL), cannabinoid receptor (CB1r and CB2r). Representative Western blotting bands are shown. Values expressed as means ± SEM; n = 5–7 rats/group (one per sex per litter); statistical difference was considered significant when *P < 0.05.
Biomarkers of ECS in white visceral adipose tissue (VAT)
In the VAT, the EW offspring of either sex did not show any significant difference in protein contents of NAPE-PLD, FAAH, DAGL, MAGL, CB1r, and CB2r when compared to their control groups (males, Fig. 7b; females, Fig. 8b).
Biomarkers of ECS in the liver
In the liver, the EW offspring of both sexes showed a decrease in CB2r when compared to their respective controls (males, Fig. 7c: −42%, P < 0.05, η2 = 0.32; females, Fig. 8c: −63%, P < 0.05, η2 = 0.31). The other parameters of the ECS did not show significant differences between groups (males, Fig. 7c; females, Fig. 8c).
Discussion
In the present study, we aimed to understand male and female hyperphagia in an animal model of early weaning, evaluating the dopaminergic and ECSs in adult life. In the palatable food preference test, all animals from both groups showed preference for the high-fat diet. Sex-specific effects were also observed: males consumed more of the high-fat diet than did females, which consumed more of the high-sugar diet than males. EW males showed greater voracity for the high-fat diet than CONT ones, although this profile was later altered, since EW animals consumed more of the high-sugar than of the hight-fat one by the end of the food preference test when compared to CONT ones. EW males showed changes in the dopaminergic system that help explain these findings, such as lower D2r in NAc and DS. Regarding the ECS, we observed a reduction of FAAH and MAGL in the LH of EW males, suggesting lower degradation of anandamide and 2-AG, respectively, which is consistent with the detected hyperphagia. Interestingly, although EW females did not show differences in preference for palatable diets, they showed alterations in the markers of the dopaminergic and ECSs, which suggest reduced production of both dopamine and anandamide. The main findings related to the dopaminergic and ECSs in brain nuclei of both EW males and females are shown in Fig. 9.
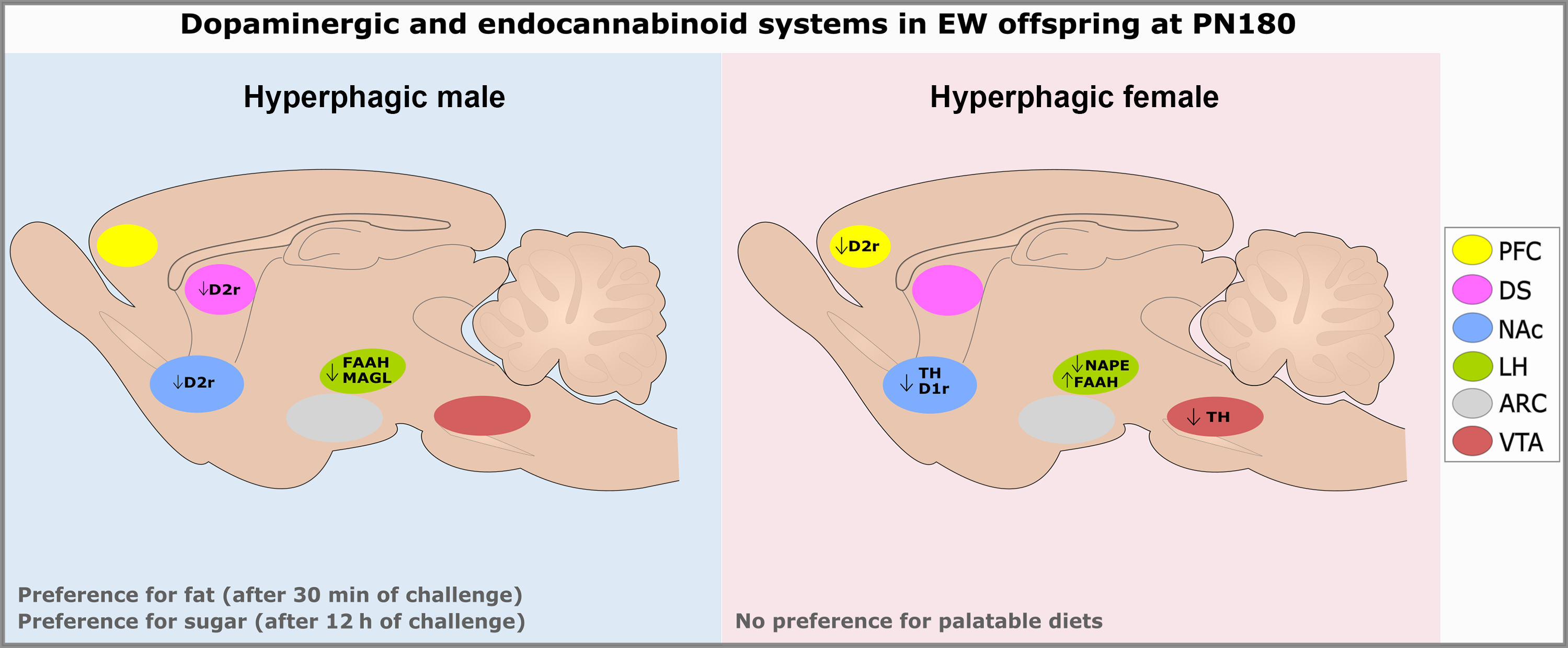
Fig. 9. Schematic overview of the main findings related to the dopaminergic and endocannabinoid systems in early-weaned males and females at PN180. ARC: arcuate nucleus; D1r: dopamine receptor type 1; D2r: dopamine receptor type 2; DS: dorsal striatum; EW: early weaning; FAAH: fatty acid amide hydrolase; LH: lateral hypothalamus; MAGL: monoacylglycerol lipase; NAc: nucleus accumbens; NAPE: N-arachidonoyl-phosphatidylethanolamine phospholipase-D; PFC: prefrontal cortex; PN: postnatal day; TH: tyrosine hydroxylase; VTA: ventral tegmental area.
The sex dimorphism detected in the food preference test corroborates the results obtained in a programming model of energy restriction during gestation.Reference Palou, Priego, Sanchez, Palou and Pico49 To understand whether the alterations found in the food preference test of the offspring programmed by early weaning were associated with the reward system, we evaluated the dopaminergic system markers in specific regions of the brain because the literature describes an inverse relationship between food intake and dopaminergic system activity, with hyperphagia and reduced dopaminergic tone.Reference Pinheiro, Moura and Manhaes42,Reference Corwin, Wojnicki and Zimmer50 Dopaminergic regulation is linked to feeding reward behavior, and it is well described that after an exposure to addictive substances, this system changes at the molecular and cellular levels.Reference Baik51 These modifications in the dopamine pathways occur by distinct mechanisms and are mediated by D1r and D2r, the most abundant dopamine receptors in the brain.Reference Jaber, Robinson, Missale and Caron52 Dopamine seems to have more affinity to D2r than to D1r and it is associated with different patterns (tonic or phasic) of dopamine release. Considering this, D1r would be more activated at high levels of dopamine and, in contrast, D2r would detect basal or low levels of dopamine. In addition, the intracellular pathway of both receptors also differs. Both receptors are G-protein coupled; however, D1r is coupled to a Gαs -protein that stimulates adenylyl cyclase (AC) activity and cyclic adenosine monophosphate (cAMP) production, which stimulates protein kinase A (PKA), while D2r is coupled to a Gαi-protein, which is a negative regulator of this same pathway.Reference Baik51 According to Avena,Reference Avena46 the reduction of D2r in the NAc is related to binge eating, increased consumption of sugary diets, and a greater predisposition for using drugs of abuse.Reference Hu and Mackie25 In our study, EW males exhibited lower D2r in the NAc and DS, suggesting a lower effect of dopamine in these tissues and thus explaining their hyperphagia and preference for palatable diets. In order to eliminate the bias involving the one-day test, it would be interesting to test feeding behavior on different days in order to check whether the preference for the palatable diet is consistent. This may constitute a limitation of the present study.
In the hypothalamus, dopaminergic signaling inhibits feeding.Reference Hoebel, Hernandez, Schwartz, Mark and Hunter37 However, in our study, early weaning in adult animals of either sex did not program the content of D2r in the ARC. Under a standard isocaloric diet, EW females apparently exhibited mild lifelong hyperphagia (5% vs. CONT females), less than the observed hyperphagia in males (16% vs. CONT males), which did not reflect the choice of diets rich in sugar or fat. Despite this finding, we observed a reduction of TH in the VTA, a reduction of TH and D1r in the NAc, and a reduction of D2r in the PFC in EW females, indicating a possible reduction in the synthesis and/or action of dopamine. Thus, a decreased activity of the brain dopaminergic reward system in EW females may increase the risk of developing food addiction.Reference Moore, Leonard and Micovic53,Reference Geiger, Haburcak and Avena54 It is conceivable that EW females will eventually show compulsion for a palatable diet. In addition, other pathways of the reward system may modulate the hyperphagic phenotype of EW animals of either sex, such as opioidsReference Selleck, Zhang, Samberg, Padival and Rosenkranz55 or oxytocin,Reference Iwasaki, Maejima and Suyama56 both directly related to the regulation of food intake.
It is well known that there is a direct relationship between animal hyperphagia and elevation of central endocannabinoids.Reference Di Marzo, Ligresti and Cristino57,Reference Rodriguez de Fonseca58 Concerning the ECS in the LH, EW males had lower levels of FAAH and MAGL when compared to controls, enzymes that are responsible for the degradation of anandamide and 2-AG, respectively, suggesting that endocannabinoids are more available in this nucleus, stimulating the higher food intake. In EW females, we observed lower levels of NAPE-PLD and higher levels of FAAH, respectively, synthesis and degradation enzymes of anandamide. These findings are paradoxical, since we expected that the ECS in the LH would also be increased in these animals due to the hyperphagia phenotype. It is possible that the apparent decrease in dopaminergic action surpasses the decrease in ECS effects.
In the adipose tissue, the endocannabinoids act by increasing the formation and storage of triglycerides.Reference Pagano, Pilon and Calcagno59,Reference Silvestri, Ligresti and Di Marzo60 Kuipers et al.Reference Kuipers, Kantae and Maarse61 studied the deregulation of endocannabinoids in rats with diet-induced obesity and observed increased serum levels of anandamide and 2-AG, and increased gene expression of DAGL in gonadal fat. In this study, we did not find changes in ECS markers in the adipose tissue of either sex in offspring programmed by early weaning, which is surprising, since these animals have increased visceral adiposity. However, we cannot discard the possibility that circulating endocannabinoids are high in our experimental model.
In the liver, mice with CB1 activation exhibit increased gene expression of SREBP-1c and lipogenic enzymes, acetyl-CoA carboxylase-1 and fatty acid synthase, and a high-fat diet increases the hepatic effects of anandamide via CB1r.Reference Osei-Hyiaman, DePetrillo and Pacher62 On the other hand, CB2r exhibits beneficial effects in the liver. In cirrhosis, CB2r is upregulated in fibrogenic cells and seems to play an important antifibrogenic and anti-inflammatory role during chronic liver injury.Reference Julien, Grenard and Teixeira-Clerc63,Reference Louvet, Teixeira-Clerc and Chobert64 As previously reported, adult males programmed by early weaning show oxidative stress and hepatic microsteatosis.Reference Franco, Lisboa and Lima13 In the current study, we observed a reduction of CB2r in the liver of EW offspring from either sex. We suggest that this finding could be related to a hepatic dysfunction that has been previously described in EW males. A distinct mechanism is possible for EW females, since they seem to be protected from hepatic dysfunctions.Reference Bertasso, Pietrobon and da Silva65
Several studies have described the influence of sex hormones in metabolic programming.Reference Dearden, Bouret and Ozanne66 Low or high concentrations of sex hormones during critical windows could permanently change the neuronal structure.Reference McEwen and Milner67 Estradiol, for example, participates in the regulation of several mechanisms in both the CNS, such as neurogenesis, cell migration, and apoptosis during early development of hypothalamus and other brain areas.Reference McCarthy68,Reference McCarthy69 Sex hormones also regulate the energy metabolism, compromising food intake and body mass. In addition, the hypothalamus, a fundamental structure for the control of energy homeostasis, and other brain regions show differences between males and females since before birth and throughout life, which are caused, in part, by different patterns of stimulation by the sex hormones on these structures during development and adult life.Reference Arnold and Gorski70 Thus, the sexual dimorphism observed in this study could be associated with the plasma levels of the sex hormones and/or their receptor contents in this model. Pietrobon et al.Reference Pietrobon, Bertasso and Silva14 showed that EW males have increased testosterone and a trend to decreased estradiol levels, while EW females had unchanged sex steroids.
The increase in obesity prevalence and eating disorders nowadays has encouraged research efforts aimed to understand the etiology of abnormal eating behaviors. To the best of our knowledge, this study is the first one to demonstrate that early weaning induces long-term alterations in the biomarkers of both the dopaminergic and ECSs, partially explaining the increase in food consumption in EW animals and the higher preference for palatable food observed only in the early-weaned males. Given the sexually dimorphic effects of EW on long-term palatable food preference, future studies addressing how sex hormones influence the molecular and behavioral changes associated with EW are warranted. Interestingly, in general, women are more prone to develop binge-eating disorder than men. However, it is possible that early life stressors, such as early weaning, have a role in the onset of these disturbances first in men.
Acknowledgments
The authors are grateful to Mr. Ulisses Risso Siqueira, Mrs. Fabiana Gallaulckydio, and Mr. Leandro Fraga Bezerra for animal care and technical assistance.
Financial support
This research was supported by Coordenação de Aperfeiçoamento de Pessoal de Nível Superior (CAPES), Conselho Nacional de Desenvolvimento Científico e Tecnológico (CNPq), and Fundação Carlos Chagas Filho de Amparo à Pesquisa do Estado do Rio de Janeiro (FAPERJ).
Conflicts of interest
None.
Supplementary material
To view supplementary material for this article, please visit https://doi.org/10.1017/S2040174421000039