We performed an extensive and critical review of the literature in order to explore the manifestations of endothelial dysfunction in individuals born after intrauterine growth restriction (IUGR) and examined which mechanisms may be incriminated and which preventive strategies could represent promising approaches. We used the following terms in the Pubmed library (MESH terms and free text), without time or language limits: (Prenatal Exposure Delayed Effects OR Late Effect, Prenatal Exposure OR Nutrition Disorders/Physiopathology OR Fetal Growth restriction) AND (Cardiovascular Diseases/Etiology OR Hypertension/Etiology) AND (Impaired Endothelial Function OR Oxidative Stress/Senescence). We included the most significant human and animal studies. From the references of the retrieved papers, additional articles were selected for this review. One author (C.Y.) read the titles and abstracts and selected the articles to be included.
IUGR: definition and risk factors
Definition
IUGR is defined as the inability of the fetus to reach its genetically determined potential size.Reference Brodsky and Christou 1 , Reference Jang, Jo, Lee, Kim and Lee 2 IUGR affects ~5–15% of all pregnancies in the United States and Europe, but its incidence varies widely and appears to be higher in low-income countries (it affects 30–55% of infants born in South Central Asia, 15–25% in Africa and 10–20% in Latin America).Reference Saleem, Sajjad and Fatima 3 Using the ReCoDe classification system, IUGR has been considered the most commonly identified factor in stillborn infants.Reference Mongelli and Gardosi 4 Therefore, the management of growth-restricted fetuses in terms of choosing the optimal delivery time is important to decrease perinatal mortality/morbidity. Fetal growth restriction is difficult to detect because of the lack of international consensus on the definition and diagnostic criteria for IUGR. In clinical practice, growth-restricted fetuses are usually identified based on birth weight (<10th percentile). However, some propose that using <3rd or <5th percentile as the criterion would better identify individuals at a higher risk of adverse perinatal outcomes. 5 Moreover, low estimated fetal weight (<10th percentile), certain ultrasound findings of fetal growth (abdominal circumference <2.5th percentile) and altered Doppler velocimetry indices, such as abnormal umbilical artery waveforms or decreased pulsatility of the middle cerebral artery, that suggest abnormalities in fetal circulation are also indicative of IUGR.Reference Alberry and Soothill 6 To better understand abnormal fetal growth and to detect IUGR, specific computerized fetal growth charts that consider fetal gender and maternal characteristics such as height, weight, parity and ethnic origin were developed by Gardosi et al. Reference Figueras and Gardosi 7 Pathological factors, including maternal systemic hypertension (HTN), diabetes, tobacco use and preterm delivery, were excluded from the model to predict the optimum weight that a baby can reach at term during a normal pregnancy. As it is necessary to distinguish between growth-restricted and constitutive ‘small for gestational age’ fetuses, longitudinal assessments of fetal growth trajectories are required to identify pathological fetal growth restriction, even if the altered growth trajectory is above the 10th centile limit.Reference Barker, McAuliffe and Alderdice 8 More recently, universal standards of fetal growth have been proposed by the Intergrowth project.Reference Villar, Cheikh Ismail and Victora 9 , Reference Villar, Giuliani and Fenton 10
Risk factors for IUGR
IUGR can result from a multitude of risk factors, including maternal and fetal causes. Several maternal factors have been identified, such as undernutrition, which notably affects the activity and/or expression of placental nutrient and ion transporters;Reference Rosario, Jansson and Kanai 11 – Reference Settle, Sibley and Doughty 13 chronic diseases, such as preeclampsia;Reference Chaiworapongsa, Chaemsaithong, Yeo and Romero 14 bacterial infection during pregnancy, particularly with Escherichia coli, group B Streptococcus, Listeria monocytogenes, Treponema pallidum or Trichomonas vaginalis; parasitic diseases, such as malaria; viral infection (e.g. human cytomegalovirus or rubella virus);Reference Adams Waldorf and McAdams 15 , Reference Derricott, Jones and Heazell 16 young age (adolescent pregnancy); and alcohol and/or tobacco consumption.Reference Baba, Wikstrom, Stephansson and Cnattingius 17 In addition, pregnancy-induced HTN, preeclampsia and placental insufficiency are known causes of asymmetrical IUGR (defined as restriction of weight followed by length).Reference Maruyama, Shinozuka and Kondoh 18 Among the fetal causes, chromosomal anomalies (including trisomy of chromosome 13, 18 or 21; tri- and polyploidies; Mulibrey nanism; 3-M, Bloom and Turner syndromes; and Majewski osteodysplastic primordial dwarfism type II)Reference Hall 19 and fetal structural defects, such as congenital heart disease,Reference Malik, Cleves and Zhao 20 result in symmetrical IUGR (defined as global growth restriction), which is usually more severe than asymmetrical IUGR.Reference Maruyama, Shinozuka and Kondoh 18
Along with maternal causes, paternal health has also been identified as a possible contributor to IUGR. Insulin resistance, smoking habits, elevated blood pressure, endothelial dysfunction, upper body fat distribution and an atherogenic lipid profile have all been suggested as potential paternally determined factors that correlate with IUGR.Reference Hillman, Peebles and Williams 21 These factors presumably impact fetal growth through epigenetic processes.Reference Li, Tsuprykov, Yang and Hocher 22
IUGR is now considered a critical public health issue because of its high perinatal mortality rate and long-term consequences. As numerous epidemiological studies have reported, infants born with fetal growth restriction have an increased risk of developing non-communicable chronic diseases, notably cardiovascular (e.g. systemic HTN and coronary artery disease) and renal [chronic kidney disease (CKD)] diseases, later in life. These observations are consistent with the concept of Developmental Origins of Health and Disease, which suggests that conditions affecting specific sensitive developmental periods, from conception throughout pregnancy to early infancy, ‘program’ tissue/organ structure and function throughout life in a process known as developmental plasticity that is adapted to short term, prevailing environmental conditions but possibly not to the further life course. The underlying mechanisms are not clearly defined. In parallel with the long-term effects of decreased nephron numbers and hyperactivity of the hypothalamic–pituitary–adrenal axis in these infants, endothelial dysfunction may also contribute to the development of certain chronic diseases in adulthood.
Endothelium dysfunction in individuals born with IUGR
The endothelium: a major role in vascular homeostasis
The endothelium plays a major role in maintaining vascular homeostasis and is one of the largest organs in the human body, consisting of more than 1014 cells lining the vascular network. It is intimately involved in the balance between vasodilation and vasoconstriction and between thrombogenesis and fibrinolysis, the inhibition and promotion of smooth muscle cell proliferation and migration, and the prevention and stimulation of platelet adhesion and aggregation.Reference Minshall, Tiruppathi, Vogel and Malik 23 All these functions are mediated by the release of numerous vasoactive factors, such as nitric oxide (NO) and endothelin. In this respect, the maintenance of endothelial structural and functional integrity is essential for vascular homeostasis; therefore, impaired endothelial function can lead to the development of vascular-related diseases.
Convincing evidence suggests that endothelial dysfunction during early childhood and persisting to adulthood in individuals born with IUGR is a key event in the development of HTN, atherosclerosis, coronary heart disease and CKD later in life. In these individuals, endothelial dysfunction primarily manifests as impaired endothelium-dependent vasodilation and vascular remodeling.
Impaired endothelium-dependent vasodilation in individuals born with fetal growth restriction
Endothelium-dependent vasodilation can be clinically evaluated using flow-mediated brachial artery tests, plethysmography or skin perfusion in response to acetylcholine using the laser Doppler technique.Reference Purnomowati, Kariadi, Achmad, Mose and Setianto 24 – Reference Martin, Lindblad and Norman 26 Impaired endothelium-dependent vasodilation has been described in children (9–11 years)Reference Leeson, Thorne and Donald 27 – Reference Goodfellow, Bellamy and Gorman 29 and young adults (20–28 years) born with fetal growth restrictionReference Leeson, Kattenhorn, Morley, Lucas and Deanfield 30 and in umbilical and placental vessels derived from growth-restricted fetuses.Reference Krause, Carrasco-Wong and Caniuguir 31
An association between fetal growth restriction and impaired NO-dependent vasorelaxation has also been observed in several animal models, mainly in rats, mice and sheep. IUGR can be induced in rats by exposure to a maternal low-protein diet (LPD, containing 9% casein)Reference Yzydorczyk, Gobeil and Cambonie 32 or restricted diet (50% of normal intake) and in sheep by single in utero umbilical artery ligation (at 105–110 days gestation); these diets and procedures result in low birth weight (LBW) offspring and lead to impaired endothelium-dependent vasodilation in small arteries,Reference Pladys, Sennlaub and Brault 33 , Reference Brawley, Itoh and Torrens 34 the aortaReference Franco, Arruda and Dantas 35 and coronary arteriesReference Tare, Parkington and Wallace 36 in adulthood.
The effects on endothelium-dependent vasodilation are more pronounced in males, whereas females seem to be protected by the NO-dependent vasoprotective role of estrogens. However, impaired endothelium-dependent vasodilation has been observed in female Wistar rats born with IUGR stemming from maternal undernutrition.Reference Franco, Arruda and Dantas 35 As suggested by Borwick et al.,Reference Borwick, Rhind, McMillen and Racey 37 fetal undernutrition may decrease estrogen synthesis, ultimately leading to ovarian damage. Interestingly, estrogen-mediated vasoprotective activity has been reported in humans; specifically, postmenopausal women taking conjugated equine estrogens (0.625 mg for 28 days) showed improved vascular NO-dependent relaxation of the brachial artery.Reference Hurtado, Celani and Geber 38
Vascular remodeling in individuals born with fetal growth restriction
Endothelial activation
Endothelial dysfunction is associated with leukocyte infiltration and the adhesion of monocytes, macrophages and low-density lipoprotein (LDL), which is oxidized to OxLDL in the arterial wall. This leads to foam cell formation and initiates atherogenesis. In addition, monocytes and macrophages secrete higher levels of cytokines and pro-inflammatory proteins such as interleukin-6 (IL-6), tumor necrosis factor-α and C-reactive protein (CRP).Reference Gleeson, Bishop and Stensel 39 These events create a vicious cycle: neutrophils and macrophages produce higher levels of IL-6 in response to inflammation, which in turn increases CRP production in the liver. CRP decreases NO availability and increases endothelin-1 production, thereby contributing to impaired endothelium-dependent vasodilation and leading to irreversible vascular damage.Reference Gleeson, Bishop and Stensel 39 , Reference Leinonen, Hurt-Camejo and Wiklund 40 Elevated levels of pro-inflammatory markers and endothelial activators are characteristic of middle-aged adults (45–64 years) born with LBW, indicating that endothelial dysfunction is patent in these individuals.Reference Pellanda, Duncan and Vigo 41
Vascular structural changes
Histopathological analyses showed that the first atherosclerotic lesions begin to develop in the abdominal aorta.Reference Teeninga, Schreuder, Bokenkamp, Delemarre-van de Waal and van Wijk 42 Increased arterial wall thickness, measured using non-invasive assessments of the intima-media or carotid intima-media thickness, has been observed in newbornsReference Skilton, Evans, Griffiths, Harmer and Celermajer 43 – Reference Cosmi, Visentin, Fanelli, Mautone and Zanardo 46 and young childrenReference Crispi, Figueras and Cruz-Lemini 47 , Reference Crispi, Bijnens and Figueras 48 and persists in adults (27–30 years) born after IUGR, and it is particularly apparent in those with exaggerated postnatal growth.Reference Oren, Vos and Uiterwaal 49
Hypoxia and oxidative stress in vascular remodeling
Hypoxia and oxidative stress have been implicated in vascular remodeling.
Placental insufficiency is related to reduced nutrient and oxygen delivery to the fetus, contributing to the development of fetal growth restriction. Several maternal factors, such as living at a high altitude, HTN, anemia, pulmonary disease, preeclampsia, drugs and/or tobacco consumption can contribute to fetal hypoxia,Reference Jensen and Moore 50 which can induce IUGR, LBWReference Lueder, Kim, Buroker, Bangalore and Ogata 51 and increase the risk of cardiovascular diseases (CVD) later in life.Reference Barker 52 , Reference Barker, Osmond, Golding, Kuh and Wadsworth 53 During fetal development, hypoxia plays a crucial role by driving vasculogenesis/angiogenesis, hematopoeisis and chondrogenesis.Reference Giaccia, Simon and Johnson 54 However, prolonged in utero hypoxia can lead to detrimental effects. In growth-restricted fetuses, circulating levels of angiopoietin-2, an angiogenic factor up-regulated by hypoxia, were increased at postnatal day 4 compared with appropriate-for-gestational age infants, thus contributing to postnatal vascular remodeling.Reference Malamitsi-Puchner, Boutsikou and Economou 55
Oxidative stress can be defined by decreased antioxidant defenses and increased reactive oxygen species (ROS) production. Under physiological conditions, ROS play an important role as a regulator of vascular functions such as migration, growth, smooth muscle and endothelial cell survival, and the secretion of extracellular matrix proteins. However, uncontrolled ROS production can contribute to vascular diseases.Reference Griendling and Harrison 56 , Reference Irani 57 ROS have been implicated in the hypertrophy and hyperplasia of vascular smooth muscle cells. In vascular cells (endothelial cells and vascular smooth muscle cells, adventitial fibroblasts), the main enzyme responsible for ROS production is nicotinamide adenine dinucleotide phosphate (NADPH) oxidase.Reference Touyz and Schiffrin 58
Angiotensin II (AngII), via Angiotensin type 1 receptor (AT1R), has been implicated in increasing superoxide anion levels followed by increased hydrogen peroxide production, which induces long-term outcomes of AngII, such as hypertrophy and hyperplasia of vascular smooth muscle cells.Reference Ushio-Fukai, Zafari, Fukui, Ishizaka and Griendling 59 , Reference Griendling, Minieri, Ollerenshaw and Alexander 60 The flavoprotein inhibitor diphenyleneiodonium (DPI)Reference Griendling, Minieri, Ollerenshaw and Alexander 60 and catalase overexpressionReference Zafari, Ushio-Fukai and Akers 61 inhibit these vascular defects. In a rat model of IUGR induced by maternal LPD associated with adult HTN, we observed increased ex vivo vasoreactivity of the carotid rings to AngII, mediated by AT1R, which was normalized by DPI and apocynin (NADPH oxidase inhibitor) pre-incubation.Reference Yzydorczyk, Gobeil and Cambonie 32
The regulation of extracellular matrix proteins such as collagen and elastin can be modulated by ROS. Elastinolysis and collagenolysis play crucial roles in arterial remodeling and vascular diseases.Reference Tyagi and Simon 62 Metalloproteinases (MMPs) and their related tissue inhibitors of metalloproteinases are enzymes secreted by macrophages and vascular smooth muscle cells. MMP-2 and MMP-9 cleave gelatin, collagen and elastin, and have been associated with vascular diseases.Reference Chow, Cena and Schulz 63 ROS have been demonstrated to activate MMPs.Reference Rajagopalan, Meng, Ramasamy, Harrison and Galis 64 Increased circulating levels of MMP-2 and MMP-9 and increased MMP-2/TIMP-2 and MMP-9/TIMP-2 ratios have been observed in children who were small for gestational age and are positively correlated with systolic blood pressure and vascular function.Reference Sesso and Franco 65 In a developmental programming animal model of HTN induced by neonatal oxygen exposure, we observed increased aortic MMP-2 and TIMP-1 and reduced TIMP-2 staining as early as 4 weeks of age, indicating a shift in the balance toward degradation of the extracellular matrix and increased collagen deposition.Reference Huyard, Yzydorczyk and Castro 66 These data suggest that early changes could contribute to the onset of the elevated blood pressure and arterial stiffness observed at adulthood in this animal model.Reference Yzydorczyk, Comte and Cambonie 67 , Reference Mivelaz, Barbier, Cloutier, Fouron and Nuyt 68
Mechanisms involved in endothelial dysfunction in individuals born with fetal growth restriction
Impaired NO bioavailability
The endothelium-mediated release of NO is widely accepted as the key determinant of endothelial function, and reduced NO bioavailability has been linked to most serious vascular pathologies.Reference Chatterjee, Black and Catravas 69 In particular, the loss of NO production contributes to impaired endothelium-dependent vasodilation and to endothelium activation by improving the recruitment of pro-inflammatory cytokines, such as vascular cell adhesion molecule-1 and ICAM-1, and the infiltration of leukocytes into the vessel wall.Reference Förstermann and Münzel 70 – Reference De Caterina, Libby and Peng 72 In normal pregnancies, NO synthesis is up-regulated, as reflected by increased nitrite/nitrate concentrations in maternal and fetal circulation, thus mediating maternal cardiovascular adaptations and the low systemic and umbilical vascular resistance in the fetus. In pregnancies complicated by IUGR, research findings are inconsistent. Some have displayed a decrease in NO metabolite concentrations in maternal and/or fetal serum, reflecting reduced NO synthesis compared with controls.Reference Hata, Hashimoto and Manabe 73 , Reference Singh, Singh and Sharma 74 Other have found higher nitrite/nitrate concentrations in umbilical venous plasmaReference Malamitsi-Puchner, Boutsikou and Economou 55 or an increase in endothelial nitric oxide synthase (eNOS) protein staining in placental vessels compared with normal pregnancies, suggesting that increased NO production could be a compensatory response to improve blood flow in the placenta.Reference Lyall, Greer, Young and Myatt 75 , Reference Myatt, Eis, Brockman, Greer and Lyall 76
Decreased NO synthesis, evaluated in terms of nitrate/nitrite production, was observed in animal models of IUGR induced by a reduction in utero placental perfusion pressureReference Payne, Alexander and Khalil 77 or maternal LPDReference Sathishkumar, Elkins, Yallampalli and Yallampalli 78 , Reference Bourdon, Parnet and Nowak 79 and in a rat model of developmental programming of HTN induced by exposing pregnant rats to androgens.Reference Sathishkumar, Elkins, Yallampalli, Balakrishnan and Yallampalli 80
Reduced NO bioavailability may result either from altered NO synthesis or from NO scavenging by other molecules, such as ROS.
Impaired eNOS functionality
Under physiological conditions, NO is synthetized in the vasculature by eNOS, using L-arginine (L-Arg) as a substrate and tetrahydrobiopterin (BH4) as a cofactor. There are contradictory data on eNOS expression in individuals born after IUGR. In humans, independent studies have indicated that eNOS expression is increased in the umbilical arteries of babies born after fetal growth restriction, suggesting that activated NO synthesis may be a compensatory mechanism to improve placental blood flow.Reference Krause, Carrasco-Wong and Caniuguir 31 , Reference Hracsko, Hermesz and Ferencz 81 However, these results are controversial because they could not be replicated.Reference Dellee, Tobias, Li and Mildenberger 82 Moreover, differences in eNOS expression have been observed in human endothelial cells isolated from the umbilical arteries (HUAECs) or veins (HUVECs) of IUGR newborns. eNOS expression is increased in IUGR-HUAECs but decreased in IUGR-HUVECs. These differences may be explained by the type of vessel (artery v. vein) or could be the consequence of altered blood flow and oxygen levels in pregnancies complicated by IUGR.Reference Maruyama, Shinozuka and Kondoh 18
In animal studies, eNOS expression varies depending on the animal model of IUGR used. In Dahl-S rats fed a high-salt diet to induce fetal growth restriction, the placental eNOS messenger RNA (mRNA) expression level was significantly increased compared with controls.Reference Takushima, Nishi and Nonoshita 83 In an animal model of IUGR induced by placental insufficiency using hyperthermic exposure, placental and umbilical artery eNOS protein in the placenta was decreased at mid-gestation but increased near term.Reference Arroyo, Anthony, Parker and Galan 84
However, eNOS expression and activity and the gender effect seem particularly sensitive to undernutrition. In fact, decreased eNOS expression and/or activity have been reported in animal models of IUGR induced by intrauterine undernourishment.Reference Franco, Arruda and Dantas 35 In a rat IUGR model induced by intrauterine undernourishment,Reference Franco, Arruda and Dantas 35 eNOS expression was decreased only in males, whereas eNOS activity was decreased in both males and females. This reduction in eNOS activity in females, which is probably the consequence of decreased estrogen levels, could explain the impaired endothelium-dependent vasodilation observed in this animal model.Reference Franco, Arruda and Dantas 35 The modulation of eNOS activity by estrogens has been confirmed in vitro. Long-term estrogen treatment of cultured human and bovine endothelial cells up-regulates eNOS activity.Reference Tolbert, Thompson, Bouchard and Oparil 85
Upregulation of the arginase pathway
Arginases produce urea and ornithine, using L-Arg as a substrate. By competing with eNOS for the bioavailability of L-Arg, arginases can indirectly contribute the reduction of NO synthesis by eNOS. Accordingly, arginase up-regulation is an important factor that drives endothelial dysfunction. Increased arginase-2 expression was observed in human umbilical endothelium from IUGR fetuses.Reference Krause, Costello and Munoz-Urrutia 86 Pre-incubation with S-(2-boronoethyl)-L-cysteine, an arginase inhibitor, improved ex vivo endothelium-dependent relaxation in umbilical and placental vessels from babies born after fetal growth restrictionReference Krause, Carrasco-Wong and Caniuguir 31 and in aortic rings from a rat IUGR model induced by maternal LPD (personal unpublished data). These data suggest that arginase activity was increased in these vessels.
Increased asymmetric dimethylarginine (ADMA) levels
ADMA, an endogenous NO synthase inhibitor, is also considered an early marker and mediator of endothelial dysfunction. ADMA acts as a competitor of L-Arg, thereby inhibiting NO synthesis by eNOS. However, the observations in human studies are controversial. In pregnancies complicated by IUGR, ADMA levels in maternal serum were found to be either increasedReference Laskowska, Laskowska and Oleszczuk 87 , Reference Gumus, Atalay, Cetinkaya Demir and Sahin Gunes 88 or decreased compared with those in normal gestations during the first (11–14 weeks), second (20–24 weeks) and third trimesters (28–35 weeks).Reference Rizos, Eleftheriades and Batakis 89 Estrogen therapy could improve endothelial function by reducing ADMA levels. Clinical data revealed that estrogen therapy, chiefly the oral form, decreased plasma ADMA concentrations and therefore improved NO production in healthy postmenopausal women.Reference Post, Verhoeven and van der Mooren 90 , Reference Karkanaki, Vavilis, Traianos, Kalogiannidis and Panidis 91
In animal models of atherosclerosis (rabbits and monkeys), endothelial dysfunction was associated with increased ADMA levels.Reference Yu, Li and Xiong 92 , Reference Boger, Bode-Boger, Sydow, Heistad and Lentz 93 To the best of our knowledge, ADMA levels have not been assessed in animal models of IUGR.
Oxidative stress
Oxidative stress plays an important role in endothelial dysfunction. ROS, particularly the superoxide anion (O2 −), play a central role in vascular physiology, and their overproduction is especially relevant to vascular pathologies.Reference Griendling and FitzGerald 94 In IUGR placentae, markers of oxidative stress, such as 8-hydroxy-2’-deoxyguanosine, redox factor-1,Reference Burton and Jauniaux 95 , Reference Takagi, Nikaido and Toki 96 malondialdehyde and oxidized LDL, are increased in venous cord blood.Reference Maisonneuve, Delvin and Edgard 97 Therefore, it has been suggested that oxidative stress is involved in both the short- and long-term modulation of endothelial function in individuals born after IUGR.Reference Webster, Roberts and Myatt 98 Oxidative stress affects the NO pathway by influencing NO synthesis and bioavailability. NO rapidly reacts with O2 − to form peroxynitrite, a highly reactive and toxic species, which reduces endothelium-dependent relaxationReference Kossenjans, Eis, Sahay, Brockman and Myatt 99 and accelerates the development of pre-atherosclerotic lesions.Reference Santilli, D’Ardes and Davi 100
As mentioned above, L-Arg and BH4 are crucial for NO production. A deficit in substrate and/or cofactor leads to enzymatic uncoupling, which causes eNOS to produce O2 − rather than NO,Reference Yzydorczyk, Comte and Huyard 101 thus contributing to endothelial dysfunction and impaired endothelium-dependent vasodilation.Reference Vasquez-Vivar, Kalyanaraman and Martasek 102 , Reference d’Uscio, Santhanam and Katusic 103 Decreased BH4 bioavailability can contribute to eNOS uncoupling. Regardless of whether the BH4 level is sufficient, the oxidation of L-Arg is coupled with the reduction of oxygen molecules to form L-citrulline and NO. However, BH4 bioavailability can be decreased through reduced production,Reference Yang, Huang, Kaley and Sun 104 increased oxidationReference Landmesser, Dikalov and Price 105 or impaired recycling of the oxidized form (BH2)Reference Chalupsky and Cai 106 therefore leading to eNOS uncoupling.
Increased O2 − production up-regulates ADMA levels, thus worsening endothelial dysfunction.Reference Sydow and Munzel 107 In humans, impaired NO-dependent vasodilation in placental vessels from IUGR pregnancies is coupled with a higher sensitivity to oxidative stress.Reference Schneider, Hernandez and Farias 108
Increased O2 − production mediated by NADPH oxidase and eNOS uncoupling was associated with defective endothelial function in a rat model of HTN induced by deoxycorticosterone and saline treatmentReference Mitchell, Cook, Danchuk and Puschett 109 and in a rat model of IUGR induced by maternal diet restriction (50% of ad libitum intake throughout gestation) or LPD (9% casein).Reference Yzydorczyk, Gobeil and Cambonie 32 , Reference Oliveira, Akamine and Carvalho 110
Endothelial progenitor cells (EPCs) dysfunction
Endothelial dysfunction is characterized by impaired vasculogenesis and decreased repair capacity, functions that are mediated by circulating EPCs. These cells are bone marrow-derived stem cells that can differentiate into mature endothelial cells, thus contributing to postnatal vasculogenesis and endothelial repair at damage sites.Reference Asahara, Murohara and Sullivan 111 EPCs subsets are differentiated by their phenotype and functional properties. The myeloid subset represents early EPCs, called colony-forming unit-endothelial cells, that appear early in cultures and display endothelial markers but do not form vessels in vivo.Reference Yoder, Mead and Prater 112 , Reference Purhonen, Palm and Rossi 113 Endothelial colony-forming cells (ECFCs), the true angioblasts, appear later and display properties such as proliferation, auto-renewal, migration and differentiation; additionally, they can support vascular growth and neovascularization. Both loss and impaired function of EPCs have been identified as markers of endothelial dysfunction, as described by Hill et al. Reference Hill, Zalos and Halcox 114 In adult men with different degrees of cardiovascular risk but without a history of cardiovascular disease, levels of circulating EPCs have been identified as a surrogate biological marker of vascular function and cumulative cardiovascular risk.Reference Hill, Zalos and Halcox 114 In pregnancy-related complications, most notably IUGR, aberrant vasculature and abnormal endothelial function were found on both the maternal and fetal sides of the placenta, and it is believed that altered fetal circulating EPCs contribute to these complications.Reference Sipos, Crocker, Hubel and Baker 115 We and others have evaluated ECFCs isolated from LBW newborns and observed altered angiogenic properties in vitro, as evidenced by decreased numbers of colonies and sprouts,Reference Hwang, Kwon and Kwon 116 and in vivo, as shown by a reduction in the number of perfused vessels.Reference Ligi, Simoncini and Tellier 117 Moreover, an imbalance between angiogenic and anti-angiogenic factors was noted.Reference Ligi, Simoncini and Tellier 117 , Reference Ligi, Simoncini and Tellier 118 These data suggest that the impairment of early angiogenic properties (structural and functional) could predispose LBW infants to endothelial dysfunction later in life.
Vascular senescence
Vascular senescence can contribute to endothelial dysfunction.Reference Minamino and Komuro 119 It is characterized by a state of irreversible (replicative senescence) or reversible (stress-induced senescence) growth arrest, the expression of negative cell cycle regulators (such as p53 and p16) and increased senescence-related β-galactosidase staining.Reference Erusalimsky and Fenton 120 Senescent endothelial cells have a decreased ability to form new vascular structures; therefore, they contribute to impaired endothelial function. Sirtuins (SIRTs), particularly SIRT1, belong to a family of proteins involved in the regulation of many cellular processes, including senescence. SIRT1 is highly expressed in endothelial cells, wherein it regulates numerous functions, such as NOS expression and cellular senescence.Reference Borradaile and Pickering 121 The depletion of SIRT1 expression in endothelial cells led to endothelial dysfunction and premature senescence in several models of cardiovascular diseases, whereas overexpression of SIRT1 protected endothelial cells from senescence-associated morphological and molecular changes.Reference Ota, Akishita and Eto 122 ECFCs from LBW newborns exhibit stress-induced vascular senescence characterized by growth arrest, increased β-galactosidase activity, and p16INK4a expression, all of which are mediated by decreased SIRT1 levels.Reference Vassallo, Simoncini and Ligi 123 Therefore, stress-induced vascular senescence is coincident with impaired angiogenic properties and could participate in the endothelial dysfunction observed later in life in individuals born after IUGR.
Relationship between IUGR and cardiovascular and renal outcomes later in life
Early endothelial dysfunction observed in individuals born after IUGR could persist for the long term and lead to the onset of cardiovascular-related diseases.
Systemic HTN
Epidemiological studies have highlighted an inverse correlation between LBW and increased blood pressure in infancy,Reference Fattal-Valevski, Bernheim and Leitner 124 adolescence,Reference Rossi, Tauzin and Marchand 125 , Reference Chiolero, Cachat, Burnier, Paccaud and Bovet 126 young adulthoodReference Leon, Johansson and Rasmussen 127 , Reference Nilsson, Ostergren, Nyberg, Soderstrom and Allebeck 128 and adulthood.Reference Gennser, Rymark and Isberg 129 – Reference Law and Shiell 132 Some authors have questioned these results, suggesting that the data were inappropriately adjusted for confounding factorsReference Huxley, Neil and Collins 133 that could potentially damage kidneys and/or vascular endothelial cells early in life (e.g. nephrotoxic drugs or umbilical catheter placement). Recent data have indicated that the risk of HTN is not only linked to birth weight but is also amplified by postnatal overfeeding, leading to exaggerated catch-up growth.Reference Tauzin, Rossi and Grosse 134
Several animal models have shown that IUGR induced by ligation of the bilateral uterine vessels, prenatal exposure to hypoxia (11.5 v. 21% O2) or glucocorticoids, maternal global undernutrition, caloric restriction or LPD during gestation induces HTN in adulthoodReference Yzydorczyk, Gobeil and Cambonie 32 , Reference Wlodek, Westcott, Siebel, Owens and Moritz 135 – Reference Gilbert, Lang, Grant and Nijland 141 and is often associated with vascular dysfunction.Reference Yzydorczyk, Gobeil and Cambonie 32 , Reference Ozaki, Nishina, Hanson and Poston 142 , Reference Cambonie, Comte and Yzydorczyk 143 However, it is not well established whether HTN precedes endothelial dysfunction. Some clinical investigations have suggested that endothelial dysfunction is a primary defect in essential HTN that appears before the increase in blood pressure,Reference Taddei, Virdis, Mattei, Arzilli and Salvetti 144 but other observations have hinted that endothelial dysfunction is a consequence of elevated blood pressure. Different animal models of HTN induced by aortic coarctation (rabbits),Reference Miller, Pinto and Mullane 145 a high-salt diet (rats)Reference d’Uscio, Barton, Shaw, Moreau and Luscher 146 or neonatal hyperoxia (rats)Reference Yzydorczyk, Comte and Cambonie 67 showed selective impairment of endothelium-dependent vasodilation secondary to increased blood pressure. However, in an animal model of IUGR caused by maternal LPD during gestation, impaired endothelium-dependent relaxation preceded the onset of increased blood pressure (personal unpublished data).
Coronary heart disease
Impaired endothelial function plays a major role in the development and progression of atherosclerosis,Reference Verma and Anderson 147 , Reference Ludmer, Selwyn and Shook 148 which ultimately leads to coronary heart disease. Many studies have proposed a relationship between birth weight and coronary heart disease: some showed an inverse relationship between LBW and increased risk of coronary heart disease,Reference Barker, Gluckman and Godfrey 149 – Reference Wang, Shu and Sheng 151 whereas others found no significant correlationReference Eriksson, Tibblin and Cnattingius 152 or a positive correlation only in males.Reference Banci, Saccucci and Dofcaci 153 Interestingly, the risk of coronary heart disease decreases with increasing birth weight. In fact, a 1-kg increase in birth weight was associated with a 10–20% decreased risk of coronary heart disease later in life.Reference Wang, Shu and Sheng 151
CKD
The role of vascular components in the renal system is of particular significance because the kidneys receive ~20–25% of the total cardiac output. However, the contribution of the endothelial compartment to kidney development has been the subject of many hypotheses. Previous experiments showed that a significant proportion of the renal endothelium is derived from a resident precursor, the metanephric mesenchyme.Reference Abrahamson, Robert, Hyink, St John and Daniel 154 Sprouting angiogenesis from the major renal vessels plays a significant role in forming the kidney endothelium, thus giving rise to most of the renal vessels and glomerular capillaries.Reference Hyink, Tucker and St John 155 Endothelial dysfunction is involved in the development and progression of CKD.Reference Stehouwer, Henry and Dekker 156 Patients with CKD display microalbuminuria, which is thought to reflect endothelial damage in the capillary system of the renal medulla and increased endothelial permeability.Reference Stehouwer, Henry and Dekker 156 – Reference Perticone, Maio and Perticone 159 Capillary damage is characterized by increased plasma concentrations of endothelium-derived proteins, such as von Willebrand factor, tissue-type plasminogen activator and urokinase-type plasminogen activator, and increased concentrations of markers of endothelial cell injury, such as soluble thrombomodulin. Decreased endothelium-dependent vasodilation occurs in end-stage kidney disease.Reference Gris, Branger and Vecina 160 Several epidemiological and experimental studies have shown that intrauterine insults are associated with the development of CKD. In humans, birth weight is positively correlated with glomerular number and inversely correlated with glomerular volume.Reference Manalich, Reyes, Herrera, Melendi and Fundora 161 In a meta-analysis of 18 studies, infants born after fetal growth restriction appeared to have a significantly higher risk of albuminuria [odds ratio (OR), 1.81; 95% confidence interval (CI), 1.19–2.77], end-stage renal disease (OR, 1.58; 95% CI, 1.33–1.88), or a low estimated glomerular filtration rate (OR, 1.79; 95% CI, 1.31–2.45).Reference White, Perkovic and Cass 162 Similar to HTN, the impairment of glomerular and tubular function secondary to IUGR is further amplified by environmental insults, such as drug exposure during the neonatal periodReference Giapros, Papadimitriou, Challa and Andronikou 163 or overweight in adulthood.Reference Silverwood, Pierce and Hardy 164
Several animal models have enabled the identification of mechanisms involved in the development of renal dysfunction later in life. Rat models of IUGR induced by exposure to maternal LPD followed by early postnatal overnutrition during the lactation period or not according litter size reduction or increased protein intake to induce accelerated postnatal growth displayed alterations in renal structural development and a risk of chronic renal failure later in life.Reference Vehaskari, Aviles and Manning 165 – Reference Boubred, Daniel and Buffat 169 Decreased glomerular number potentially leads to reduced filtration capacity, reduced salt and water retention and the subsequent development of HTN. Furthermore, early loss of nephron numbers/mass may result in a state of hyperfiltration in the remaining nephrons, which will lead to focal segmental glomerulosclerosis and further loss of glomeruli, thus initiating a vicious circle.Reference Anderson, King and Brenner 170 However, it is not clear whether endothelial dysfunction precedes or is a consequence of CKD. Regarding the impact of postnatal nutrition on renal maturation, rodent models could likely be more affected than humans because nephrogenesis is completed at ~36 weeks of gestation in humans, whereas in rats, this process is completed during postnatal life (between 7 and 10 days of life).
Potential preventive approaches
Several interventions have been identified to potentially prevent IUGR, improve endothelial function and thus antagonize the development of detrimental cardiovascular issues.
Breastfeeding
Breast milk could represent a promising approach, and the easiest one, for improving endothelial function in offspring. In fact, breastfeeding, as opposed to feeding with commercial infant formulas, is one of the best approaches for fighting neonatal oxidative stress because of breast milk’s ability to ‘trap’ free radicals. Breast milk contains enzymatic and non-enzymatic components such as superoxide dismutase, glutathione peroxidase, vitamins (A, C and E), α-carotene, lactoferrin and trace amounts of iron. Breastfeeding could improve endothelial function, primarily due to the presence of lactoferrin, an iron-binding glycoprotein with antioxidant, anti-inflammatory, pro-angiogenic and NO-dependent vasodilator properties. Daily treatment with lactoferrin after unilateral hind limb surgery-induced ischemia in C57BL/6J mice promoted angiogenesis, activated endothelial function via an NO-dependent mechanismReference Ikeda, Tajima and Izawa-Ishizawa 171 and protected HUVECs against hydrogen peroxide-induced oxidative stress.Reference Safaeian, Javanmard, Mollanoori and Dana 172
Folate supplementation
Epidemiological studies have shown that folate deficiency is associated with increased cardiovascular risk.Reference Verhaar, Stroes and Rabelink 173 , Reference Robinson, Arheart and Refsum 174 Because of the homocysteine-lowering and antioxidant effects of folate and its ability to modulate eNOS activity and cofactor availability, folic acid supplementation could improve vascular endothelial structure and function.
In a study including patients with coronary heart disease, the circulating form of folic acid, 5-methyltetrahydrofolate, increased NO-dependent vasodilation, reduced vascular superoxide production, and improved enzymatic coupling of eNOS by increasing the availability of BH4.Reference Antoniades, Shirodaria and Warrick 175 Folate supplementation in patients with acute ischemic strokeReference Xia, Li and Wang 176 or HTNReference Wu, Wang and Li 177 decreased plasma ADMA levels, suggesting that folate intake may also be beneficial in these contexts. Moreover, folic acid supplementation during pregnancy increased the birth weight of newborns.Reference Li, Qu and Dang 178
Folate deficiency in ApoE−/− mice was associated with the development of atherosclerotic lesions, which can be prevented by folate supplementation.Reference Alessio, Santos and Debbas 179 Moreover, folate supplementation of a maternal LPD diet prevented the development of increased blood pressure and restored endothelium-dependent vasodilation and eNOS mRNA expressionReference Torrens, Brawley and Anthony 180 and enzyme activity.Reference Stroes, van Faassen and Yo 181
Vitamin supplementation
Studies of animal models of IUGR and developmental programming of CVD have demonstrated that maternal diet supplementation with vitamins C and E can prevent adverse perinatal and long-term outcomes. In an animal model of IUGR induced by high maternal cholesterol levels during the early stages of gestation, maternal dietary supplementation with vitamin E was found to prevent growth restriction in fetuses. Vitamin E has been shown to regulate molecular pathways controlling cell proliferation and viabilityReference Zingg and Azzi 182 and to increase the release of vasodilator prostanoids from human aortic endothelial cellsReference Wu, Liu, Meydani and Meydani 183 and HUVECs,Reference Tran and Chan 184 thus improving placenta–fetal blood flow and thereby increasing nutrient delivery to the fetus.
Vitamin C was found to protect chick embryos against the developmental toxicity of ethanol. Indeed, concomitant injection of vitamin C and ethanol in chick embryos prevented the decreased survival, growth retardation and malformations induced by ethanol alone.Reference Memon and Pratten 185
However, in human studies, these treatments have failed to show clear benefits in terms of birth weight and associated long-term diseases.Reference Hovdenak and Haram 186 – Reference Rumbold, Crowther and Haslam 189 A possible explanation is the potential confounding effects of maternal endogenous antioxidant defenses and redox status and maternal vitamin intake resulting from diversified nutrition. Differences in vitamin metabolism between humans and animals could also be involved in the discrepancy between human and animal studies.
Antioxidant therapy
Supplementation with resveratrol, a polyphenolic molecule found at high concentrations in red grapes, berries and peanuts, has been identified as a potential therapeutic strategy for the treatment of cardiovascular diseases, primarily due to its antioxidant properties and ability to modulate the NO signaling pathway. In spontaneously hypertensive rats, maternal dietary supplementation with resveratrol during the perinatal period prevented the onset of HTN in adult offspring.Reference Care, Sung and Panahi 190 Resveratrol also modulates SIRT1 expression. Pre-incubation with resveratrol restored angiogenic capacity and reversed the accelerated senescence of ECFCs from LBW newborns.Reference Vassallo, Simoncini and Ligi 123
Lazaroid is a potent inhibitor of free radical formation, notably O2 −-mediated lipid peroxidation. Treatment with Lazaroid reversed HTN in several rat models,Reference Vaziri, Ding, Ni and Gonick 191 , Reference Vaziri, Ni, Oveisi and Trnavsky-Hobbs 192 and the addition of Lazaroid to a maternal LPD diet throughout gestation increased birth weight and reversed later vascular dysfunction in offspring by decreasing oxidative stress.Reference Cambonie, Comte and Yzydorczyk 143
In a guinea pig model of IUGR induced by progressive uterine artery occlusion starting at mid-gestation, maternal N-acetylcysteine treatment during the second half of gestation restored ex vivo eNOS-dependent relaxation in the fetal aorta and umbilical artery and normalized eNOS expression in fetal and umbilical endothelial cells.Reference Herrera, Cifuentes-Zuniga and Figueroa 193
Melatonin, a hormone with antioxidant and anti-inflammatory properties, is involved in regulating circadian and circannual rhythmsReference Hardeland, Cardinali and Srinivasan 194 and could improve endothelial function. Melatonin exhibits direct scavenging activity on O2 −,Reference Tare, Parkington and Wallace 36 , Reference Reiter, Tan, Terron, Flores and Czarnocki 195 , Reference Franco Mdo, Akamine and Aparecida de Oliveira 196 up-regulates antioxidant enzymes such as superoxide dismutase and glutathione peroxidase, and down-regulates pro-oxidant enzymes such as lipoxygenase,Reference Galano, Tan and Reiter 197 thereby increasing NO production and improving its availability to induce vasodilationReference Lopez, Garcia and Escames 198 in different vascular beds.Reference Herrera, Macchiavello and Montt 199 – Reference Das, Balonan, Ballard and Ho 202
L-citrulline supplementation
L-citrulline is a precursor of L-Arg. L-citrulline is a non-protein amino acid, which is absent from the regular diet, escapes liver metabolism, has high bioavailability, and is quantitatively converted to arginine in vivo.Reference Curis, Nicolis and Moinard 203 Data have suggested that L-citrulline supplementation improves fetal growth in animal model of IUGR induced by in utero exposure to maternal LPD,Reference Bourdon, Parnet and Nowak 79 probably by improving maternal nutritional status and fetal growth through increased NO synthesis as a result of enhanced L-Arg availability in fetal circulation. L-citrulline can also exert a protective role on vascular endothelium. In fact, it has been proposed that L-citrulline supplementation could represent an alternative to L-Arg supplementation to improve vascular function,Reference Romero, Platt, Caldwell and Caldwell 204 , Reference Chien, Lin and Kuo 205 and it attenuated blood pressure in young normotensive men.Reference Figueroa, Trivino, Sanchez-Gonzalez and Vicil 206 In animal models, ex vivo pre-incubation with L-citrulline prevented endothelial dysfunction induced by ADMA in porcine coronary artery; indeed, such incubation favors L-citrulline to L-Arg recycling and the restoration of NO production, as a consequence of eNOS expression and activity up-regulation, the inhibition of superoxide anion production, and activation of the cyclic guanosine monophosphate (cGMP)Reference Xuan, Lun and Zhao 207 Such direct beneficial effects of L-citrulline on endothelium-dependent relaxation suggest that L-citrulline supplementation could be an efficient way to improve endothelial function in individuals born after fetal growth restriction.
Supplementation with L-Arg and NO mediators
It was reported that L-Arg could be administered to increase maternal NO levels to enhance birth weight and decrease neonatal morbidity.Reference Singh, Singh and Sharma 74 More recently, the combined results of 10 small trials showed that L-Arg supplementation can increase the body weight and gestational age at birth of IUGR fetuses.Reference Chen, Gong, Chen, Luo and Zhang 208 However, this study contrasts with others that reported no benefit of L-Arg therapy.Reference Gui, Jia and Niu 209 , Reference Vadillo-Ortega, Perichart-Perera and Espino 210 Such differences could be explained by the different route of administration (oral or intravenous). In fact, with oral administration, 40% of L-Arg is degraded by the small intestine and metabolized by arginase in the liver. Therefore, poor L-Arg availability in the blood could decrease its efficacy.Reference Curis, Nicolis and Moinard 203 , Reference Wu, Bazer and Cudd 211
Among NO modulators, phosphodiesterase inhibitors are promising agents for improving uterine perfusion in pregnancies complicated by IUGR. Type 5 phosphodiesterase (PDE5) is one of the enzymes responsible for the degradation of cGMP to GMP in smooth muscle. Therefore, inhibiting PDE5 delays the breakdown of cGMP and increases vasorelaxation. Sildenafil citrate (Viagra®) is probably the most famous PDE5 inhibitor. In women whose pregnancies were complicated by IUGR, sildenafil citrate improved fetoplacental perfusionReference Dastjerdi, Hosseini and Bayani 212 and decreased the ex vivo vasoconstriction (in response to the thromboxane analogue U46619) of myometrial small arteries.Reference Wareing, Myers, O’Hara and Baker 213
In animal models, parenteral administration of L-Arg (from day 60 of pregnancy to parturition) to underfed ewes prevented fetal growth restriction,Reference Lassala, Bazer and Cudd 214 and in a rat model of IUGR induced by maternal LPD, pre-incubating the aortic rings with L-Arg restored impaired endothelium-dependent vasodilation (personal unpublished data). Sildenafil citrate supplementation reversed the maternal effects of preeclampsia by improving uteroplacental and fetal perfusionReference Herraiz, Pellicer and Serra 215 in a Wistar rat model and increased fetal size in pregnant rats exposed to hypoxia at the end of gestation (18–20 days).Reference Refuerzo, Sokol and Aranda 216
Epigenetic markers of endothelial dysfunction
Epigenetics plays a major role in the developmental origins of health and diseases.Reference Cutfield, Hofman, Mitchell and Morison 217 Epigenetics can be defined as a phenomenon of altered phenotypic expression of heritable genetic information without changes in the DNA sequence. Three main pathways can silence, activate or regulate the level and time of expression of many genes: DNA methylation, histone modifications (acetylation, methylation, ubiquitination, phosphorylation or ADP-ribosylation) and small non-coding RNAs, such as microRNAs (miRNAs).Reference Chen and Zhang 218 , Reference McKay and Mathers 219 In general, these three epigenetic mechanisms appear to work together to regulate gene expression. DNA methylation or histone modifications can alter the expression of miRNAs, which can in turn regulate the epigenetic processes of DNA methylation and histone modifications.
DNA methylation
DNA methylation has been known to be particularly sensitive to an adverse early environment. DNA methylation occurs through the binding of a methyl group in position 5 of the cytosine ring dinucleotide CpG sequences present in the DNA by DNA-methyltransferase, which can methylate and demethylate the DNA, thus making the modification reversible.Reference Kangaspeska, Stride and Metivier 220 In general, low levels of DNA methylation (hypomethylation) are associated with increased gene activity, whereas high levels of methylation (hypermethylation) are associated with gene repression.Reference Lorenzen, Martino and Thum 221 Moreover, hydroxymethylated cytosine [5-hydroxymethylcytosine (5 hmeC)] has been identified as another functional DNA modification, representing an intermediate state of active DNA demethylation and also influencing gene expression.Reference Ito, D’Alessio and Taranova 222 , Reference Kriaucionis and Heintz 223
eNOS expression in HUAECs and HUVECs of IUGR pregnancies can be controlled by DNA methylation levels. eNOS protein and mRNA levels were increased in HUAECs but decreased in HUVECs from IUGR pregnanciesReference Krause, Costello and Munoz-Urrutia 86 and were associated in the eNOS promoter with decreased DNA methylation at CpG −352 in IUGR-HUAECs and an increased in IUGR-HUVECs. In addition, in human umbilical artery endothelial cells from patients with placental insufficiency, levels of 5 hmeC at the eNOS transcription start site directly correlated with elevated eNOS levels.Reference Postberg, Kanders and Forcob 224 In a guinea pig model of IUGR, increased eNOS expression was associated with decreased DNA methylation levels in eNOS promoter of endothelial cells derived from aorta, femoral and umbilical arteries; such modifications were prevented by maternal administration of N-acetylcysteine.Reference Herrera, Cifuentes-Zuniga and Figueroa 193
Histone modifications
In the nucleus, DNA is packaged into chromatin as repeating units of nucleosomes, which form a ‘beads-on-a-string’ structure that can compact into higher order structures to affect gene expression. Nucleosomes are composed of 146-bp DNA wrapped in histone octamers (composed of two H2A, H2B, H3 and H4) and are connected by a linker DNA, which can associate with histone H1 to form heterochromatin. Histone proteins contain a globular domain and an amino-terminal tail, which can be post-translationally modified. The post-translational modification of lysine (acetylation, methylation, ubiquitination, sumoylation), arginine (methylation) and serine and threonine (phosphorylation) are the most commonly described modifications.Reference Chen and Zhang 218 , Reference Canani, Costanzo and Leone 225 In general, the acetylation of histones H3 and H4 is associated with increased gene expression and has been shown to regulate the angiogenic function of endothelial cells.
Levels of H3K9ac and H2A.Zac were significantly higher at the eNOS transcription start site and were directly correlated with elevated eNOS levels observed in the human umbilical artery endothelial cells from patients with placental insufficiency.Reference Postberg, Kanders and Forcob 224 In addition, increased histone H3 acetylation in the endothelin-1 promoter of pulmonary vascular endothelial cells and in the peripheral leucocytes in a IUGR rat model induced by maternal undernutrition has been correlated with higher endothelin-1 expression, which could increase the risk of pulmonary disorders (pulmonary HTN or asthma) later in life.Reference Xu, Xu and Fu 226 Recently, we observed that SIRT1 repression in ECFCs from LBW newborns, associated with premature senescence, could be modulated by changes in ‘active’ or ‘repressive’ epigenetic marks. The ‘active’ marks trimethyl-H3K4 associated with the SIRT1 promoter were significantly decreased in LBW newborns compared with controls, whereas the ‘repressive’ marks trimethyl-H3K9, associated with heterochromatin formation, were increased.Reference Vassallo, Simoncini and Ligi 123
Non-coding RNAs
MiRNAs are small single-strand RNAs that do not encode proteins. Each miRNA binds to specific mRNAs, resulting in the degradation of target mRNA or the inhibition of its translation into protein. miRNAs regulate the post-transcriptional expression level of many genes and processes such as apoptosis, cell growth and differentiation in a large range of tissues,Reference Shruti, Shrey and Vibha 227 , Reference Sayed and Abdellatif 228 and notably in the regulation of endothelial functions. MiR-21 expression is increased in cases of shear stress, which helps to protect endothelial cells by decreasing apoptosis and increasing eNOS expression and NO production.Reference Weber, Baker, Moore and Searles 229 However, in atherosclerotic plaques, an up-regulation of miR-21 decreases the function of superoxide dismutase, which leads to increased ROS production and decreased migration of the progenitor cells.Reference Fleissner, Jazbutyte and Fiedler 230 MiR-221 and miR-222 are highly expressed in endothelial cellsReference Liu, Cheng, Yang, Xu and Zhang 231 and exert antiangiogenic, antiproliferative, antimigration and proapoptotic effects on endothelial cells,Reference Liu, Cheng, Yang, Xu and Zhang 231 which can be partly caused by reduced eNOS expression.Reference Suarez, Fernandez-Hernando, Pober and Sessa 232 In addition, miRNAs can modulate SIRT1 expression. Increased expression of miR-217 and miR-34a have been observed in endothelial senescence, which leads to loss of SIRT1 function, notably by reducing eNOS expression.Reference Xu, Seeger and Castillo 233
Conclusions
In individuals born after fetal growth restriction, early endothelial dysfunction plays an important role in the subsequent development of HTN, coronary heart disease and CKD. Decreased NO synthesis and bioavailability caused by defective eNOS function and oxidative stress, decreased EPCs number and function, and vascular senescence have all been shown to be involved in endothelial dysfunction (Fig. 1). Preventive approaches, including breastfeeding and supplementation with folate, vitamins, antioxidants, L-citrulline, L-Arg and NO modulators, represent promising and simple ways to prevent fetal growth restriction, improve endothelial function and vasodilation responses early in life and delay/prevent detrimental cardiovascular issues.
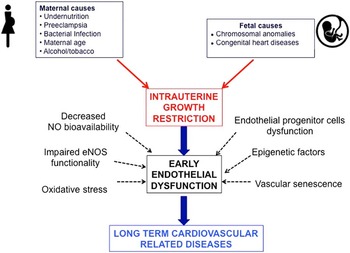
Fig. 1 Association between intrauterine growth restriction, early endothelial dysfunction and long-term cardiovascular-related diseases. eNOS, endothelial nitric oxide synthase; NO, nitric oxide synthase.
Epigenetic modulation of gene expression appears to be one of the main contributors to the long-term effects of an adverse perinatal environment. The identification of early biomarkers of endothelial dysfunction, especially epigenetic biomarkers, could allow early screening and follow-up of individuals at risk of developing CVD, thus contributing to the development of preventive and therapeutic strategies to avert the long-term effects of endothelial dysfunction in infants born after IUGR.
Acknowledgments
The authors thank Dolorès Mosig, Katya Nardou and Dr Fabienne Maurer for their invaluable contributions.
Financial Support
This research received no specific grant from any funding agency in the commercial or not-for-profit sectors.
Conflicts of interest
None.