A healthy intra-uterine environment is essential to normal development of all organisms, including humans. Maternal physiology and proper nutrition have been shown to be important factors affecting the growth and development of fetuses. These factors are related to optimal birth weight and successful completion of the entire critical gestational period. In contrast, circumstances such as poverty, poor nutrition and other types of maternal hardship, could result in poor adult health caused by a number of chronic diseases,Reference Barker and Osmond1 and also raises the possibility of effects on the health of future generations.Reference Gillman, Smith and Hanson2
The concept of the developmental origins of adult disease, which developed as a result of observed effects of poor nutrition on fetal growth and development associated with health outcomes in adulthood, is now understood to extend to non-nutritional exposures as well. The proof of principal of nutritional effects provides the foundation for understanding the effects of environmental chemicals on molecular, cellular and organ systems. This concept also provides the basis to understand overt health effects impacting childhood and adulthood in populations exposed to chemicals early in life. In addition to alterations in nutrition, a variety of chemical exposures can produce an unhealthy environment, endangering the fetus and increasing susceptibility to diseases later in life. Thus, the current body of knowledge now suggests that the developmental origins of disease paradigm relates to both nutrition and exposures to environmental chemicals during development, and that such exposures can alter disease susceptibility later in life. For the purposes of discussion in this paper, environment is broadly defined as any factors that are non-genetic in nature. This can include things such as environmental chemicals, radiation, biological agents, pharmaceuticals, nutritional factors and psychosocial and behavioral stressors.
Through new methods of biomonitoring, at least nine classes of pharmaceuticals and environmental chemicals have been detected in a variety of biospecimens that reflect the fetal environment.Reference Barr, Bishop and Needham3 Data from chemical analyses documenting the prevalence of certain classes of environmental chemicals, such as pesticides and industrial chemicals found in biospecimens from pregnant mothers and newborn babies, continues to mount.Reference Whyatt, Camann and Perera4–Reference Ye, Pierik and Angerer6 In order to adequately determine whether environmental chemicals have life-long or multigenerational effects on exposed populations, it is important to define the periods of vulnerability according to biologic and toxicological principles. It is also important to acknowledge the challenges of extrapolating findings from animals to human studies so that the strength of the evidence can be weighted appropriately. Finally, it is also critical to communicate research findings and the weight of evidence in ways that provide individuals with practical approaches to prevent or reduce exposure as additional research is being conducted.
Windows of susceptibility: development
Normal development is immensely complex. The establishment of the proper hormone milieu is critical for providing the appropriate biologic signaling necessary for normal growth and development. Every organ system has its own developmental trajectory, and a number of organ systems continue to develop after birth as seen in Fig. 1.Reference Altshuler and Berg7
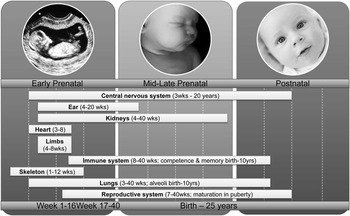
Fig. 1 Prenatal and postnatal windows of susceptibility for organ system effects of environmental exposures.
Development is one of the most sensitive windows for environmental exposures. Basic biological processes involved in development and in the body's ability to respond to environmental exposures progress at different rates.Reference Suk and Collman8 Since the protective systems that metabolize harmful chemicals or repair early damage may not be fully functional until later in childhood, these processes impact the mechanisms of susceptibility of the fetus and the young child. For example, scientists have shown that PON 1, the enzyme that metabolizes chlorpyrofos and other organophosphate pesticides, does not become fully functional in cells until 9 years of age.Reference Huen, Harley and Brooks9 Children with different PON1 genotypes show altered enzyme phenotypes, which results in differing susceptibility.
The DNA repair system is another example of a protective process that continues to develop after birth and, as a result, can be damaged by environmental exposures during development. As DNA repair systems are not fully functional in all cells during development, early life exposures to pesticides may lead to cellular damage that increases the risk of disease later in life. For instance, DNA repair systems may become fully functional at different stages in life. In this system, the fetal liver does not have the ability to detoxify many chemicals, so the fetus relies on maternal detoxification. However, metabolites that are potentially safe for the mother may not be safe for the fetus which could result in higher fetal exposures. In addition, the blood–brain and other organ barriers are not fully developed during gestation, thus tissues can be exposed to chemicals during development that would otherwise be unable to cross these barriers later in life. Another critical aspect of development is programming, or the change in gene expression due to epigenetic markings, which vary throughout life.Reference Waterland and Michels10 The combination of all of these factors leads to a fetus that is both exposed to environmental chemicals and more vulnerable/sensitive to such exposures.
Exposures to toxic chemicals change in quantity and quality begining from the womb, extending into early life, and continue as children go through childhood, puberty, adolescence and early adulthood. This variance in exposures is due to changing pathways through which environmental chemicals come in contact with the organism. Recent studies document detectable levels of a variety of endocrine disrupting chemicals such as phthalates, polybrominated diphenyl ethers and bisphenol A (BPA) in pregnant women, fetuses, newborns, young children and adolescents, due to their common use in products such as plastics that are ubiquitous in the environment.11–Reference Herbstman, Sjödin and Kurzon16 Exposures to polycyclic aromatic hydrocarbons, which result as a by-product of combustion, are also common place in women and children.
When considering the early effects of exposures, researchers can predict different effects for different organ systems.Reference Heindel and Newbold17 These effects differ depending on when exposure occurs, such as, within a specific windows of vulnerability, and depending on dosage levels. Exposures occurring early in pregnancy can be pivotal factors in determining short-term health effects on birth outcomes, such as birth weight and head circumference,Reference Wolff, Engel and Berkowitz13 while exposures later in pregnancy or during early childhood may lead to consequences in childhood development and learning.Reference Lovasi, Quinn and Rauh15 Studies such as these demonstrate that the developmental time period in utero is a critically sensitive window of vulnerability. The fetus is undoubtedly exposed to environmental chemicals, and researchers have noted overt and silent biological and health effects at birth and in early childhood that result from gestational exposures.
In recent years, there has been a change in scientific focus from studying gross effects, such as birth defects, to examining functional changes, which can be much more subtle or even non apparent at birth, but may emerge in later life.Reference Heindel and Newbold17 For example, in the past high dose toxicology research focused on teratogenic effects of chemicals used in agriculture or industry, which resulted in stillbirth, birth defects, or mortality. Current scientific methods facilitate the study of functional changes or molecular changes. These changes impact gene expression, protein activity, or the number of cells that compromise tissue function, rather than affecting birth weight or causing birth defects, and thus are not detected in typical teratology studies. Though these early damages may not be apparent for many years, under certain circumstances, or in individuals whom are susceptible, such damages can manifest themselves as disease or dysfunction later in life, as depicted in Fig. 2. Over the course of one's lifespan, additional exposures also occur to multiple stressors which may result in changes that are more harmful to the biological pathways related to disease. The risk of adulthood disease is due to the combined damage over the lifespan. Evidence now indicates that early life exposure to toxic chemicals may be associated with a host of long-term diseases, such as breast and prostate cancer, Parkinson's disease, obesity and many others.Reference Heindel and Newbold17–Reference Vandenberg, Maffini and Schaeberle19

Fig. 2 Shifting the paradigm: early life exposures may cause functional changes at celluar levels that lead to changes in physiological status and ultimately adult disease.
Developmental basis of disease and role of environmental exposures
Heindel and NewboldReference Heindel and Newbold17 define some key principles relevant to the understanding of how environmental chemicals can cause effects early in life that are not evidenced until later in life and contribute to the risk of adult diseases.
Notably, effects from environmental chemicals may be both tissue specific and time specific. Since some tissues continue to develop into the first years and even the first decade of life, the term developmental exposures pertains not just to exposures in utero but may also include exposures during the first few years of life. The overarching principle is that as long as a specific tissue is developing, it remains very sensitive to disruption by environmental chemical exposures.Reference Heindel and Newbold17
Another important principle is the initiating biologic effect during gestation may be due to a single stressor or a result of multiple events. In addition, it is possible that the damage caused during pregnancy from one or more environmental chemicals may be compounded by additional exposures/stressors throughout one's lifespan. This concept is sometimes referred to as the cumulative impacts of environmental exposures. This theory predicts that the combination of these effects will predict risk. Disease risk could be manifested in a variety of ways – new disease, earlier onset of disease or a more severe form of disease – due to the early impact of an environmental chemical. Chemical exposures may cause development programming changes that permanently alter tissue, organ or pathway function. This is likely due to epigenetic changes, but more research is needed to fully determine the mechanism under varying conditions. In addition, the effects of chemical stressors can be transgenerational, meaning that they can be transmitted through the germ line for as many as three generations.Reference Nilsson, Anway, Stanfield and Skinner20, Reference Skinner, Manikkam and Guerrero-Bosagna21
The effects of hazardous chemicals and other environmental exposures may not work through the same mechanism that influence birth weight, as seen with nutritional impacts. This notion involves considering that the dose–response curves for exposure-disease relationships may be difficult to quantify and may not be linear or monotonic. In addition, the dose–response curves for individual chemical exposures are unlikely to be identical. Harmonizing those effects across multiple classes of agents will be a challenge because the dose–response curves have such different shapes.Reference Heindel and Newbold17
Importantly scientists must consider interactions as well as when considering cumulative impacts across the lifespan. The exposure to a single stressor may have a weak effect or no effect, and may only be potentiated with later life exposures. Effects of environmental agents vary with genetic strain when dosing occurs in adulthood.Reference Harrill, Ross, Gatti, Threadgill and Rusyn35, Reference Bauer and Kleeberger36 This has been documented in animal experiments focusing on the liver and the lung. This concept needs to be extended to include effects of early life exposure to environmental chemicals. It is likely that environmental influences will differ with differences in genetic background in animals and human populations.
In additional to increasing our understanding of the etiology of disease, considering the developmental origins of disease may lead to disease prevention. Animal data show that developmental exposures to environmental chemicals, even for just a few days at environmentally low relevant doses, can lead to increased incidence of a number of major diseases in the human population. Indeed, many of these diseases are those whose incidence has increased greatly over the past 40 years, further implicating the role of environment in their etiology. These diseases (as seen in Figure 3), include, attention deficit hyperactivity disorder and learning and behavioral problems, obesity, type 2 diabetes, early puberty, breast, prostate and uterine cancers, reproductive problems (uterine fibroids, endometriosis, premature menopause, infertility), cardiovascular disease, and neurodegenerative diseases.Reference Heindel and Newbold17 Preventing exposures during this critical time period can prevent many diseases in the population.

Fig. 3 Age of onset for diseases and conditions that may result from early life exposures.
A final aspect of this new paradigm of disease is that some of the harms of exposures during development have been shown to be transmitted via the germ line for at least three generations.Reference Nilsson, Anway, Stanfield and Skinner20, Reference Skinner, Manikkam and Guerrero-Bosagna21 As animal studies are replicated and expanded upon, we will gain additional evidence showing that many present day diseases have their origins in past generations. Indeed, the diseases we are experiencing today may have been transmitted to us, not just from our parents and not just due to genetic changes, but though epigenetic changes transmitted via the germ line from our grandparents and great grandparents.
Challenges to conducting developmental origins of disease-based studies
Many challenges facing animal and human studies exist under the developmental origins of disease rubric. Animal studies should be viewed as multi-endpoint studies, designed to look at the multisystem effects of early life exposures. Biomarkers or intermediate phenotypes need to be developed and tested as integral components of these experiments. Animals may have to be aged longer than usual to account for the long latency period of effects during gestation. Work should be conducted using multiple species with varying genetic backgrounds to identify similarities and differences across animals. Epigenetic analysis needs to be conducted in a variety of tissue types and at different points in the life span to obtain the data necessary to study mechanisms, and perhaps, develop biomarkers of exposure and disease susceptibility.
Measuring exposures in human populations during gestation, or in any given window of time over the lifespan, is an immense challenge. However, researchers have made significant progress toward being able to quantify a diverse array of environmental chemicals using analytic chemistry techniques or other high-throughput techniques.11 Scientists’ ability to measure many endocrine disrupting chemicals in multiple media reflects the routes of elimination and the persistence of the chemicals themselves. Low doses methods now allow measurements in very small quantities of biospecimens.
Since 2001, CDC (Centers for Disease Control and Prevention) has analyzed the blood and urine specimens collected through NHANES (National Health and Nutrition Examination Survey) which is a series of recurring cross-sectional studies examining the health of people the United States and biennially produces a chemical report card.11 These studies provide a reference set of values for many environmental chemicals being studied in populations across the United States and the world. It is an invaluable resource, but it does not provide descriptive data about the sensitive windows of exposure that we need. However, other studies of cohorts of pregnant women and their offspring around the world now provide additional data in populations selected for their unique characteristics including potential for high exposure to pesticides, metals, and other chemicals of concern.Reference Davidson, Myers and Cox22–Reference Eskenazi, Gladstone and Berkowitz25 Additional cohorts of individuals maturing through different life stages will help provide exposure data during key windows of susceptibility, such as adolescence.Reference Hiatt, Haslam and Osuch26 Collectively, these studies include biomonitoring data from pre- and postnatal periods and also collect useful exposure information by questionnaire.
Well-designed studies include multiple time points for exposure characterization, the collection of multiple types of biospecimens and long-term storage of these important specimens. Studies that build in additional collections to determine long-term storage and viability, can measure exposure level trends or calculate half-lives, and can determine the validity of single measurements v. multiple collections. This methodology will make valuable contributions to addressing the complex questions these types of environmental epidemiologic studies require. Biobanks, repositories of biological samples that include DNA samples, are critical for supporting studies covering joint role of genetic, epigenetic and environmental factors related to diseases of interest.
In addition, there are formidable challenges to successfully amass human data from multiple generations. Ideally, one would like to have biospecimens and relevant questionnaire data from multiple generations to compare the body burden estimates across generations and to use the most appropriate data for linking association with disease. Questionnaire data provided by recall from an index study subject cannot possibly provide the reliability or precision needed for useful epidemiologic analysis. This is especially true for diseases later in life, such as Parkinson's Disease, which cause cognitive impairment on the subject and will reduce the availability of information a past life events. Documenting the deaths of close relatives (i.e. parents) also complicates matters in these studies.Reference Van den Berg, Christianson and Oechsli27
Cohort study designs that builds in specimen collections during the pertinent windows of development are critical, but they are also prohibitively long and expensive. However, currently there are a few studies being conducted around the world that have collected specimens from multiple generations. One example is the US-based children's health and development study.Reference Barlow, Cory-Slechta, Richfield and Thiruchelam28 This study began in the 1950s by amassing a cohort of pregnant women and collecting blood specimens and questionnaire data. These women and their offspring have been monitored for many childhood and adult health endpoints. Their offspring are now reaching childbearing age and continued contact and enrollment of their children could create a multigenerational study opportunity. The Collaborative Perinatal Program has also been a valuable resource to study exposures during pregnancies in the 1960s and their health consequences later in life.Reference McGlynn, Guo and Graubard37
The biological and toxicological principals previously defined, and the challenges delineated here, point to the complexity of research needed to understand the effects of early life exposures on adult diseases. These principles emphasize the need to study index exposures during pregnancy before collecting data on the contributing co-stressors, such as other classes of chemicals, socio-cultural factors and diet/nutritional status. Conducting research in a consortia arrangement, one in which epidemiologic and animals studies can replicate important combinations of exposures and look across organ system endpoints, will provide much needed focus evidence about the occurrence and mechanism of health effects.
One example of a consortium that has had success with this strategy is the Breast Cancer and the Environment Research Centers (BCERC) supported by the National Institute of Environmental Health Sciences (NIEHS) and NCI (National Cancer Institute). In this consortium, four research groups are conducting complementary animal experiments and an epidemiologic study of the environmental and genetic determinants of pubertal timing in young girls.Reference Hiatt, Haslam and Osuch26 Animal work focuses on gestational and pubertal dosing of animals using different endocrine disrupting chemicals to understand endpoints later in life – pubertal timing and breast tumors. Research in adolescent girls focuses on quantifying the natural history of breast development, pubic hair development and timing of menarche, as well as measuring over 20 environmental chemicals and other co-factors in the girls to determine the relationship between these factors.
The BCERC work is transdisciplinary, such that research results in the animal work feeds back to the human studies and vice versa. Furthermore, NIEHS recently established a consortium of animal and epidemiological studies of the effects of early life exposure to BPA. This new opportunity will allow scientists to look at multi-organ system effects across species and to compare animal and human results on a number of chronic disease endpoints.
Shifting the paradigm and communicating results
Even when research studies are able to account for all of these challenges, finding robust effects in human populations that recapitulate animal studies is still immensely difficult. Both the scientific community and the public are frustrated by the frequent inconsistencies between the results of animal and human studies. It is not uncommon that researchers fail to find the results in human populations that seem to be true from animal studies.
Animal studies can be quite sophisticated and mechanistically based. Collectively they have provided the proof of principle of the developmental basis of disease, and have convincingly established some exposure effect relationships. However, scientists and public policy makers tend to express concerns about whether the animal studies really illustrate the reality of what is happening in the human population. Developing new ways of integrating the collective data and evaluating the weight of the evidence in ways that we can properly measure the important findings in the animal studies and move forward in protecting human health are needed.
One way of improving this evaluation process would be to better incorporate data from biomarkers and intermediate phenotypes in animal and human studies. Biomarkers are assays of molecular or cellular evidence or the changes that are indicative of the functional changes discussed earlier (Fig. 4Reference Groopman, Johnson and Kensler29). For example, changes in receptor function or changes in gene expression, or proteomic patterns that occur as a result of exposure, are all functional changes and occur long before disease manifestation. The key to improving our understanding would be to look at biological pathways that may be involved in a disease of interest, by using biomarkers that capture early changes.Reference Schwartz and Collins30 Then a comparison is necessary to see how chemical insult changes the levels of these pathway specific biomarkers. Incorporating markers, such as proteomic signaturesReference Colquhoun, Goldman and Cole31, Reference Betancourt, Mobley, Russo and Lamartiniere32 or epigenetic markers,Reference Perera, Tang and Herbstman33 in human studies before overt disease is established, may allow for extrapolation of animal to human effects.
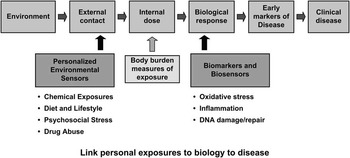
Fig. 4 Opportunities for using sensors and biomarkers to measure environmental exposures throughout the exposure to disease trajectory.
Another concept relevant to improving data extrapolation is to incorporate the study of intermediate phenotypes, such as changes in clinical characteristics that are predictive of later disease into epidemiologic and other human studies. Rather than waiting for a diagnosis of disease to occur, early changes in physiology or early signs of pathology could be measured. For instance, some intermediate phenotypes such as early onset puberty, increased insulin resistance or menstrual cycle changes could be incorporating into our evidence-based determination of causality.
It is difficult to conceive that we can conduct many studies from cradle to disease, so we must shift our approach to weighing evidence by exploring the strength of the association in each window of susceptibility using relevant intermediate markers of clinical changes. Understanding the effects of exposure on the measures of functional changes, exposures in the intermediate phenotype, and the path from insult to phenotypes, would be helpful for clarifying extrapolations to disease risk. If the scientific community is capable of determining how exposure produces early cellular changes and how these are linked to intermediate phenotypes and later disease, perhaps new and different strategies to assess the consistency of the accumulating body of research can accompany this work.
The public demands precautionary approaches and the recent President's Cancer Panel34 confirms the need for preventive measures to fully protect the American public from the health risks associated with environmental exposures. Given the body of scientific literature available, legislative actions in the European Union and the United States have been proposed or are waiting to be implemented. In the current risk assessment model, assessing the strength of the evidence is all too often dependent on ‘that definitive human study’, which, as already discussed, is infrequent and complex. Given the challenges discussed here, obtaining evidence from human populations is difficult. However, working across disciplines, consensus opinions can be developed by acknowledging the body of literature where there is certainty and assessing the unknowns.
There are many communication campaigns that are being conducted by non-profit organizations such as environmental groups that highlight exposure sources and body burdens. We need to be very mindful about how research produced in our laboratories is factored into what these groups are doing. The public wants concrete actions they can take to protect themselves, and more importantly, protect their children, from the harmful effects of environmental chemicals. The public is interested in knowing what options they have in personal choices for consumer products, behavior changes, or public policy, that can reduce exposures to harmful chemicals. Given the many new tools available, scientist can contribute to the evidence base for these actions. By adopting a new paradigm, scientists can conduct meaningful research on these emerging questions, and as the body of evidence unfolds, scientists can evaluate the evidence in ways that acknowledge what we know and where the uncertainties lie. Despite the uncertainty, our goal as researchers should be to provide information to end users of our science that enables them to protect themselves and their future family members from the developmental effects of environmental chemicals that manifest themselves across the life span.