Introduction
Polycystic ovary syndrome (PCOS) is one of the most common endocrine disorders in premenopausal women, affecting ~7% of reproductive age women. 1 , Reference Sirmans and Pate 2 It contributes to numerous morbidities ranging from metabolic dysfunction (including type 2 diabetes, glucose intolerance and obesity) to ovulatory disorders and infertility.Reference Hart and Doherty 3 – Reference Nader 5 Excess testosterone (T) is a hallmark of the disorder 6 , Reference Azziz, Carmina and Dewailly 7 and it has been hypothesized that excess prenatal T may be a key etiologic factor. Extensive evidence from animal models supports this hypothesis.Reference Abbott, Dumesic and Franks 8 – Reference Padmanabhan and Veiga-Lopez 10 In non-human primates, sheep, rats and mice, administration of T during critical gestational windows results in PCOS-like features when female offspring mature.Reference Abbott, Nicol, Levine, Xu, Goodarzi and Dumesic 9 , Reference van Houten, Kramer, McLuskey, Karels, Themmen and Visser 11 , Reference Comim, Hardy, Robinson and Franks 12 In humans, women with clinical conditions characterized by T excess during pregnancy (e.g. congenital adrenal hyperplasia, congenital virilizing tumors or certain sex steroid pathway mutations) have daughters who are at increased risk of PCOS later in life.Reference Barnes, Rosenfield and Ehrmann 13 – Reference Morishima, Grumbach, Simpson, Fisher and Qin 15
Typically, PCOS diagnosis is made after menarche, when menstrual cycle dysregulation and symptoms of T excess (e.g. hirsutism and acne) emerge. Even then, however, diagnosis is confounded and delayed by highly irregular menstrual cycles and hormone profiles common to normal female pubertal development.Reference Rosenfield 16 Diagnosis of PCOS before or during adolescence has therefore proven difficult, and as a result, the estimated prevalence of PCOS in adolescent girls is uncertain.Reference Kamangar, Okhovat and Schmidt 17 In theory, early identification of girls at risk of developing PCOS would be useful because it could facilitate interventions to prevent or ameliorate symptoms. For example, lifestyle modifications aimed at weight reduction, improved cardiovascular fitness and insulin resistance, or better nutrition, all diminish PCOS symptomology in adolescence.Reference Harden, Cowan, Velasquez-Mieyer and Patton 18 – Reference Lass, Kleber, Winkel, Wunsch and Reinehr 21
If excess prenatal T exposure also contributes to PCOS risk, as the animal models suggest, identifying biomarkers of prenatal T excess early in life would be invaluable. Although there are currently no direct methods for safely indexing the fetal hormonal milieu in normal human pregnancies, evidence has increasingly supported the use of anogenital distance (AGD), or the distance from the anus to the genitals, as a reliable biomarker of prenatal T exposure. In humans and other mammalian species, AGD is 50–100% longer in males than females.Reference Barrett, Parlett, Redmon and Swan 22 – Reference Fouqueray, Blumstein, Monclus and Martin 25 In animal models, AGD can be elongated in females by experimentally increasing the prenatal T environment. For example, female rodents,Reference Hotchkiss, Lambright, Ostby, Parks-Saldutti, Vandenbergh and Gray 26 rabbits,Reference Banszegi, Altbacker, Ducs and Bilko 27 sheepReference Recabarren, Padmanabhan and Codner 28 , Reference Manikkam, Crespi and Doop 29 and non-human primatesReference Abbott, Colman, Tiefenthaler, Dumesic and Abbott 30 exposed to fetal male T concentrations during gestation have longer AGD than controls.
Human data on this topic is limited, however interest is growing. Supporting the hypothesis that excess prenatal androgen exposure contributes to PCOS, two recent studies (in Chinese and Mediterranean women) have found that AGD is longer in adult women with PCOS compared with controls.Reference Sanchez-Ferrer, Mendiola and Hernandez-Penalver 31 , Reference Wu, Zhong, Chen, Zheng, Liao and Xie 32 One of the two studies additionally found positive associations between AGD measurements and circulating T levels.Reference Wu, Zhong, Chen, Zheng, Liao and Xie 32 In healthy young women, longer AGD has been linked to multi-follicular ovaries and higher serum testosterone levels.Reference Mendiola, Roca and Minguez-Alarcon 33 , Reference Mira-Escolano, Mendiola and Minguez-Alarcon 34 However studies in adults cannot rule out postnatal influences on AGD or ovarian function. Indeed, cross-sectional studies in adult women have found that AGD is positively related to ageReference Wainstock, Shoham-Vardi, Sheiner and Walfisch 35 and inversely associated with use of hormonal contraception,Reference Mendiola, Roca and Minguez-Alarcon 33 suggesting that there may be some plasticity in adulthood. Examining newborns circumvents this issue and may yield important clues. In a small study of infants, girls with congenital adrenal hyperplasia, who experienced excess adrenal androgen production during gestation, had longer AGD than controls.Reference Callegari, Everett, Ross and Brasel 36 Here we extend this research to examine the daughters of women with PCOS, who may be exposed to elevated T during fetal development. Women with PCOS have elevated T during pregnancy compared with controls,Reference Caanen, Kuijper and Hompes 37 and it is plausible that the female fetuses they carry may also produce excess T. Our objective in this study was to translate these lines of fetal programming research to humans, and test the previously unexplored hypothesis that AGD is elongated in infant girls born to mothers with PCOS. To this end, we used data from a large pregnancy cohort study, classifying women as having: (1) diagnosed PCOS; (2) hirsutism, a diagnostic criterion for PCOS, without diagnosis; or (3) neither PCOS diagnosis nor symptoms.
Materials and methods
Study overview and population
The Infant Development and Environment Study (TIDES) was designed to examine environmental exposures during pregnancy in relation to infant reproductive system development. Study recruitment and methods have been described elsewhere.Reference Barrett, Sathyanarayana and Janssen 38 , 39 In brief, pregnant women were recruited at prenatal clinics affiliated with four U.S. academic medical centers [University of California-San Francisco (UCSF); University of Minnesota (UMN); University of Rochester (URMC); and University of Washington (UW)] between 2010 and 2012. Eligibility criteria included: <13 weeks pregnant, age 18 or older, ability to read and write in English (or Spanish at the UCSF center), no major medical conditions, pregnancy non-medically threatened, and planning to deliver at an affiliated study hospital. Subjects participated in study visits in each trimester, during which they completed questionnaires and gave urine samples. Before implementation, TIDES was approved by The University of Rochester Research Subjects Review Board, The University of Minnesota Human Research Protection Program Institutional Review Board, The University of Washington Human Subjects Review Committee and the University of California San Francisco Human Research Protection Program Committee on Human Research. When the TIDES Coordinating Center moved from the University of Rochester to the Icahn School of Medicine at Mount Sinai, the latter’s Human Research Protection Program Institutional Review Board also approved the study. All subjects signed written informed consent.
Characterization of maternal PCOS status
In the first trimester, subjects completed a questionnaire including items on basic demographic information including race, ethnicity, age, height and body weight immediately prior to pregnancy. In the second trimester, subjects completed a questionnaire detailing their reproductive health and history. Women were asked whether they were ever given a PCOS diagnosis. They were also asked a series of questions aimed at assessing possible undiagnosed PCOS, including an item on hirsutism, defined on the questionnaire as ‘excess hair growth on the upper lip, chin, chest or abdomen prior to pregnancy’. An additional item on oligomenorrhea (another diagnostic criterion for PCOS, defined in the questionnaire as having ‘fewer than eight menstrual periods per year when not taking hormonal contraception, pregnant or breast-feeding’) was included. In other cohorts, self-reported symptoms have been reliably associated with PCOS diagnosis.Reference Taponen, Ahonkallio and Martikainen 40 – Reference Dunaif 44 Based on their responses to these items, women were categorized as: (1) reporting ever having received a PCOS diagnosis (irrespective of hirsutism and menstrual regularity); (2) reporting hirsutism but no history of PCOS diagnosis (irrespective of menstrual regularity); or (3) non-PCOS (comparison group), with neither PCOS, hirsutism, nor oligomenorrhea. We did not pursue analyses specific to the group reporting oligomenorrhea because it became clear that it was a heterogeneous group that did not represent women with probable PCOS.
Birth exams
Shortly after birth (median and 75th percentile at 1 day old) trained teams of study coordinators administered standardized physical examinations to infants born to TIDES participants. These methods have been described elsewhere 45 and are similar to those used in our previous study of infant reproductive development.Reference Swan 46 In brief, for infant girls, two measurements were made using Vernier dial calipers: (1) the distance from the center of the anus to the anterior tip of the clitoral hood (AGD-AC); and (2) the distance from the center of the anus to the base of the posterior fourchette (AGD-AF). All measurements were taken with the infant lying flat on her back with the legs held in a ‘frog leg’ position by the mother or a study assistant. Each measurement was repeated three times (with the calipers zeroed between measurements) and the average of those three values was used in analyses. 39 , 45 Finally, the study team measured infant weight and length at exam and recorded the infant’s gestational age at birth from the medical record.
Statistical methods
We calculated descriptive statistics (mean, standard deviation, maximum, minimum, frequencies) for the combined study population as well as stratified by PCOS status. We examined differences between the two groups in bivariate analyses, using analysis of variance for continuous variables and χ2 tests for categorical variables. We fit two sets of multivariable linear regression models to examine infant AGD in relation to maternal PCOS status. In both sets of models, our outcomes were infant AGD (AGD-AF or AGD-AC). In the first set of models, we examined AGD in daughters of mothers with diagnosed PCOS compared with daughters of mothers without PCOS. In the second set of models, we examined AGD in daughters of mothers with diagnosed PCOS or hirsutism (possibly indicating undiagnosed PCOS, or at least elevated T levels) compared with daughters of mothers without PCOS.
We selected a number of covariates for a priori inclusion based on our previous work demonstrating that they predict AGD in infants. 39 , 45 , Reference Barrett, Hoeger, Sathyanarayana, Redmon, Nguyen and Swan 47 , 48 These covariates were gestational age at birth, infant age at physical examination, weight-for-length z-score, maternal age and study center. Because of their associations with PCOS, we considered the inclusion of race (dichotomized as white v. all others) and pre-pregnancy body mass index (BMI) as additional covariates. Race, but not BMI, changed estimates by more than 10% and was retained in final models. Thus the final set of covariates included in models was: infant age at exam, weight-for-length z-score, gestational age at birth, study center, maternal age and race.
Finally, we performed a set of sensitivity analyses limited to term infants (⩾37 weeks gestation) because it has been hypothesized that delays in measuring preterm infants after birth (due to their fragility) may make their AGD measurements fundamentally different than those of term babies. All analyses were performed in SAS Enterprise Version 5.1 (SAS Institute, Cary, NC, USA) and results for which P⩽0.05 were considered statistically significant.
Results
In total, 737 women in the TIDES study completed 2nd trimester questionnaires and had infants who underwent genital measurements shortly after birth. Of those, 376 gave birth to female infants and 85% had complete data needed for the current analyses. Among those missing data, 17 were lacking data on covariates and the remainder had not fully answered all questions on PCOS and related symptoms. Of the 300 subjects who were therefore included in this analysis, 23 were categorized as having PCOS, 45 were categorized as having hirsutism without a PCOS diagnosis and 232 were categorized as having neither PCOS nor diagnostic criteria (Fig. 1). Compared to women not included in the analysis, those included were slightly older (31.4 v. 30.1 years) and had a lower pre-pregnancy BMI (27.1 v. 28.9 kg/m2) (not shown).
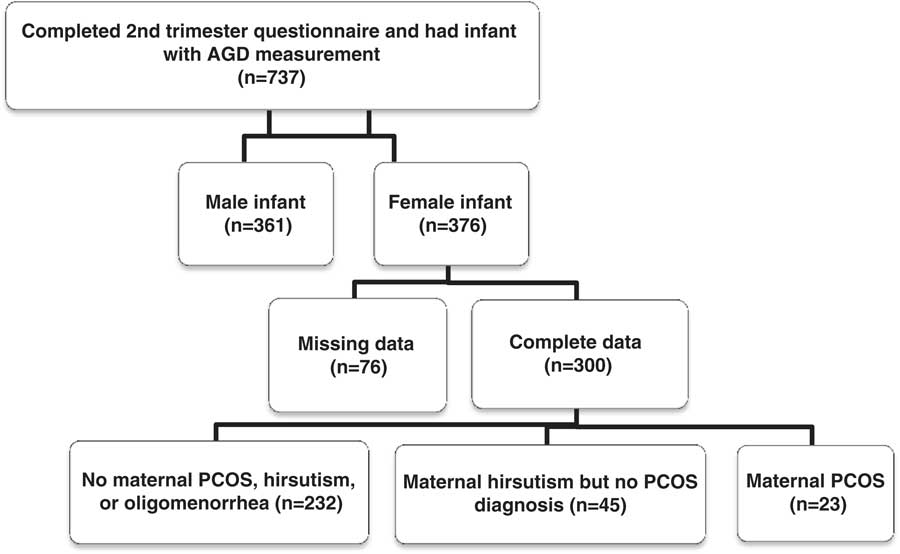
Fig. 1 Determination of final sample for current The Infant Development and Environment Study (TIDES) analyses. AGD, anogenital distance; PCOS, polycystic ovary syndrome.
Focusing on the women included in the main analyses, in bivariate analyses, women with PCOS and the non-PCOS comparison group were similar in age and gestational age at delivery. Women with PCOS (or related symptoms) tended to have a higher pre-pregnancy BMI and were more likely to self-identify as white, and less likely to identify as black or Asian (Table 1). Women with hirsutism, but not a PCOS diagnosis, were more likely to report smoking during pregnancy. Not surprisingly, women who reported having PCOS or hirsutism, were more likely to also report oligomenorrhea and a history of infertility than the non-PCOS comparison group. Infants born to the three groups were similar in size at birth and crude AGD measurements.
Table 1 Characteristics of The Infant Development and Environment Study (TIDES) mothers and daughters (n=300)Footnote a

PCOS, polycystic ovary syndrome; AGD-AF, anofourchette distance; AGD-AC, anoclitoral distance.
a Exact n may vary due to missing data.
b P-values based on results of analysis of variance and χ2 tests.
In multivariable linear regression models, the daughters of mothers with PCOS had longer AGD-AF (β=1.21, 95% CI: −0.01, 2.43, P=0.05) but not AGD-AC (β=1.05, 95% CI: −0.49, 2.60, P=0.18) than daughters born to women without PCOS (Table 2). In the second set of analyses (which grouped women with PCOS and those reporting hirsutism but no PCOS diagnosis), both AGD-AF and AGD-AC were longer among the daughters of possible PCOS cases compared with daughters of women without PCOS (AGD-AF: β=0.82, 95% CI: 0.06, 1.58, P=0.03; AGD-AC: β=1.15, 95% CI: 0.19, 2.11, P=0.02).
Table 2 Regression models comparing anogenital distance (AGD) in infant girls born to women with (a) polycystic ovary syndrome (PCOS) or (b) PCOS or hirsutism; to infant girls born to a non-PCOS comparison group [(β coefficient, 95% CI); P-value]

AGD-AF, AGD-anofourchette distance; AGD-AC, AGD-anoclitoral distance.
a The comparison group (‘non-cases’) includes women who reported: (1) not having PCOS; (2) not being hirsute; (3) not having eight or fewer menstrual periods per year.
b Adjusting for infant age at exam, weight-for-length z-score, gestational age at delivery, study center, maternal age, race.
c Due to one missing AGD-AC value, the sample size is one less than for the AGD-AF analysis.
In sensitivity analyses, we restricted our main models to only include term births. In these analyses, effect sizes were larger and P-values smaller, despite the smaller sample size. Among the term daughters of women diagnosed with PCOS (n=19), AGD-AF (β=1.65, 95% CI: 0.30, 2.99; P=0.02) and AGD-AC (β=1.43, 95% CI: −0.27, 3.12; P=0.09) were longer than in daughters of non-PCOS women. Similarly, in the term daughters of women with PCOS or hirsutism, both AGD measures were longer (AGD-AF: β=0.99, 95% CI: 0.19, 1.79; P=0.02; AGD-AC: β=1.22, 95% CI: −0.15, 2.91, P=0.08) than among daughters of women without PCOS (Table 2).
Discussion
In this pregnancy cohort, we demonstrated for the first time that women with PCOS gave birth to daughters with longer AGD, a measure of prenatal T exposure, than daughters born to women without PCOS. These results support previous work indicating that during PCOS pregnancies, female fetuses may encounter elevated T levelsReference Sir-Petermann, Maliqueo, Angel, Lara, Perez-Bravo and Recabarren 49 , Reference Barry, Kay and Navaratnarajah 50 and suggest that AGD may provide a postnatal ‘read-out’ of their prior intrauterine hormonal environment. They are also consistent with recent findings from two studies comparing AGD in adult women with PCOS and controls. In both studies, PCOS cases had significantly longer AGD than controls. In fact, the odds of having PCOS was 3–19-fold greater among women in the longest AGD tertile compared with women in the shortest AGD tertile.Reference Sanchez-Ferrer, Mendiola and Hernandez-Penalver 31 , Reference Wu, Zhong, Chen, Zheng, Liao and Xie 32 One of the two studies, furthermore, showed that AGD was positively associated with circulating T levels, particularly among PCOS cases.Reference Wu, Zhong, Chen, Zheng, Liao and Xie 32 Similar results have been found in a cross-sectional study of healthy, adult women. Women in the longest tertile of AGD were 3–6 times as likely to have multi-follicular ovaries (⩾6 follicles per ovary) as women in the shortest tertile of AGD.Reference Mendiola, Roca and Minguez-Alarcon 33 In that study, too few women had diagnosed PCOS to draw conclusions, however other work has indicated that multi-follicular ovaries may be indicative of hyperandrogenic ovarian dysregulation.Reference Adams, Franks and Polson 51 , Reference Padmanabhan and Veiga-Lopez 52 In the same study population, moreover, AGD-AF was positively correlated with serum T concentrations in the early follicular phase.Reference Mira-Escolano, Mendiola and Minguez-Alarcon 34
This body of work is also consistent with non-human primates (both the well-characterized prenatally androgenized model and naturally occurring high T females), whereby T exposure is positively correlated with AGD.Reference Abbott, Colman, Tiefenthaler, Dumesic and Abbott 30 , Reference Abbott, Dumesic and Lewis 53 For these reasons, AGD should be explored further as a possible early indicator of PCOS risk. Although an extensive literature has examined AGD as a marker of prenatal T in boys (particularly in relation to anti-androgenic environmental chemicals), 39 , Reference Swan 46 , Reference Bornehag, Carlstedt and Jonsson 54 , Reference Suzuki, Yoshinaga, Mizumoto, Serizawa and Shiraishi 55 this is one of the first studies to demonstrate that AGD may also be a useful biomarker in girls, particularly in the context of a hyperandrogenic hormonal milieu.
These results are also noteworthy in relation to the growing body of research on health and development among the children of women with PCOS. Several studies have noted neurodevelopmental alterations among offspring of PCOS mothers that, as in our study, may be indicative of excess prenatal androgen exposure. For example, prenatal androgens organize the male brain and it has been hypothesized that excess androgens may contribute to autism spectrum disorders.Reference Baron-Cohen, Auyeung and Norgaard-Pedersen 56 , Reference Baron-Cohen, Knickmeyer and Belmonte 57 In a recent nationwide case-control study in Sweden, the odds of autism spectrum disorders, were nearly 60% higher in the offspring of mothers with PCOS compared with controls.Reference Kosidou, Dalman and Widman 58 In separate work on typically developing children, girls born to women with PCOS scored significantly higher than controls on ‘systematizing’ scales (which measure conscious focus on working out the rules of a social/communication system) and lower on empathy scales, a pattern more common in males and indicative of non-clinical autism spectrum-like development.Reference Palomba, Marotta and Di Cello 59 , Reference Baron-Cohen 60 As further evidence of the effects of prenatal androgens on the female brain, in a macaque model, prenatally androgenized females exhibit masculinized patterns of play and mounting behavior concomitant with decreases in female-typical mating behavior.Reference Goy, Bercovitch and McBrair 61 , Reference Thornton and Goy 62 Finally, recent evidence from a rodent PCOS model suggests that prenatally androgenized animals display alterations in sex steroid pathways in key brain regions (e.g. the amygdala and hippocampus) leading to an anxiety phenotype characteristic of women with PCOS.Reference Dokras, Clifton, Futterweit and Wild 63 , Reference Hu, Richard and Maliqueo 64 Whether AGD at birth is predictive of any of these neurodevelopmental alterations is unknown. There is, however, evidence that AGD at birth is associated with sex-typed play behavior in boys later in childhoodReference Pasterski, Acerini and Dunger 65 and similar studies are needed in girls.
Our results suggest that female fetuses in PCOS pregnancies experience elevated T exposure, however the course of that exposure is unclear. It is conventionally believed that during pregnancy, barrier mechanisms (including high levels of sex hormone binding globulin and aromatase) protect the fetus from maternal T exposure and that only in exceptional cases is the placental capacity for androgen metabolism exceeded.Reference Kanova and Bicikova 66 , Reference Xita and Tsatsoulis 67 It is plausible that in PCOS pregnancies, these mechanisms are altered or insufficient. At term, placental steroidogenesis, particularly 3βHSD-1 and P450 aromatase activity, are altered in PCOS placentae compared with controls, in patterns that may promote increased placental androgen production.Reference Maliqueo, Lara, Sanchez, Echiburu, Crisosto and Sir-Petermann 68 In addition, morphometric and vascular differences between PCOS and non-PCOS placentae have been noted, further implicating placental dysfunction.Reference Palomba, Russo and Falbo 69 , Reference Palomba, Russo and Falbo 70 Perhaps most plausibly, PCOS daughters may themselves produce excess ovarian or adrenal androgens during gestation, possibly due to a genetic predisposition.Reference Govind, Obhrai and Clayton 71 – Reference Lunde, Magnus, Sandvik and Hoglo 73 While our results cannot distinguish among the possible sources of excess T in PCOS pregnancies (i.e. mother, fetus, placenta), this is clearly an area of great interest that merits additional research.Reference Puttabyatappa, Cardoso and Padmanabhan 74 , Reference Abbott, Levine and Dumesic 75
Our results also contribute to the small literature on sex steroid activity and ovarian function in the daughters of women with PCOS. In one study, mid-gestation amniotic testosterone levels were elevated among women with PCOS than controls, however this was only true for women carrying female fetuses.Reference Palomba, Marotta and Di Cello 59 This fits with evidence that by mid-gestation, much as in non-human primates, the human fetal ovary has androgen receptors and can produce androgens.Reference Cole, Hensinger, Maciel, Chang and Erickson 76 – Reference Fowler, Anderson and Saunders 78 In infancy, daughters born to women with untreated PCOS have higher anti-Mullerian hormone and stimulated estradiol levels (suggestive of greater ovarian mass and/or a larger growing follicular pool) than daughters from non-PCOS or metformin-treated PCOS women.Reference Crisosto, Echiburu and Maliqueo 79 These endocrine differences in PCOS daughters appear to persist over time. In adolescence, daughters of women with PCOS have higher free androgen index, testosterone and insulin levels (2 h after a glucose tolerance test), as well as larger ovarian volumes than controls, all symptoms suggestive of an emergent PCOS phenotype.Reference Crisosto, Codner and Maliqueo 80 Thus maternal PCOS predicts a subsequent PCOS-like phenotype in daughtersReference Dumesic, Abbott, Eisner and Goy 81 and incorporating AGD measurements into evaluations at birth (or even later in childhood) may ultimately help to predict which daughters are most likely to go on to develop PCOS, facilitating early intervention.
Our study has several notable strengths including a diverse cohort recruited from four medical centers. Our AGD measurement protocols were particularly rigorous, and included extensive centralized training of study examiners as well as regular quality control measures. 45 Importantly, our protocols were specifically designed to facilitate AGD measurement shortly after birth to minimize the possibility that postnatal factors might affect AGD as the infant ages. Although several cohorts have now measured infant AGD (mostly in the context of maternal environmental exposures), to our knowledge, no other study has examined AGD in relation to PCOS or other hyperandrogenic conditions in the mother. Because this analysis was situated in the context of an ongoing epidemiological cohort study, we will continue to follow these mother–daughter dyads forward and have the potential to track the development of PCOS-like symptoms in the daughters in the future.
There are also several notable limitations to our study. Because this large cohort study was not designed to examine PCOS, although our overall prevalence of PCOS was similar to national estimates, the absolute number of PCOS women was relatively small and replication in a larger cohort is needed. Lacking medical records, furthermore, we relied upon self-reported PCOS and we did not explicitly query women about other relevant conditions, such as familial hirsutism, congenital adrenal hyperplasia and ovarian tumors that may have contributed to hirsutism and other PCOS-like symptoms. However given that this was a healthy pregnant population without any known major medical conditions, such disorders are unlikely. In addition, recent reports suggest that for research purposes, self-reported PCOS may be as good as clinical diagnosis of PCOS.Reference Day, Hinds and Tung 43 , Reference Dunaif 44 To address this possibility of under-reporting of PCOS (due to the reliance on self-report), our second set of analyses additionally considered women reporting hirsutism but not PCOS. The strength of the associations was reduced when women with hirsutism (without a PCOS diagnosis) were included, which may indicate that it was a heterogeneous group. Future work examining this question should thoroughly assess all possible causes of PCOS-like symptoms and confirm with clinical records to avoid misclassification. Finally we did not collect data on use of metformin or other ovulation inducing drugs during pregnancy. Metformin can lower testosterone levels in non-pregnant women with PCOSReference Stadtmauer, Wong and Oehninger 82 and is sometimes used through the first trimester when the fetal reproductive system is forming. Although studies in pregnant women with PCOS have shown no differences in androgen levels in mothers in the metformin and placebo groups,Reference Carlsen and Vanky 83 , Reference Vanky, Salvesen, Heimstad, Fougner, Romundstad and Carlsen 84 some endocrine differences have been noted in infant daughters of PCOS women who did and did not take metformin during pregnancy.Reference Crisosto, Echiburu and Maliqueo 79 The same may be true of other ovulation inducing drugs that were not assessed. Importantly, these medications would be used more widely among the women with PCOS in our study, thus if there were effects on T levels, they would likely be in the direction of reducing fetal androgen exposure and attenuate associations with infant AGD. Nevertheless, future studies on this topic should consider the use of metformin and other hormonal treatments in relation to androgen-sensitive outcomes in offspring.
In conclusion, our results suggest that among infant girls, AGD may be a biomarker of excess T exposure in utero and may be worth further investigation as a possible predictor of subsequent PCOS risk. Because AGD can be easily measured in all children at birth (and throughout childhood), it could prove particularly useful as an early risk phenotype identifying girls who could benefit from early lifestyle modification programs aimed at reducing obesity, and thereby PCOS incidence. We will continue to follow this cohort as they age to study downstream sequelae of longer AGD in girls.
Financial Support
Funding for this research was provided by the following grants: NIH R01ES016863-04; R01ES016863-02S4; P30 ES005022.
Conflicts of Interest
None.
Ethical Standards
The authors assert that all procedures contributing to this work comply with the ethical standards of the relevant national guidelines on human experimentation and with the Helsinki Declaration of 1975 as revised in 2008, and has been approved by the institutional committees at the University of Rochester, the University of California-San Francisco, the University of Washington, the University of Minnesota, and the Icahn School of Medicine at Mount Sinai.
Acknowledgments
The authors thank the contributions of the TIDES Study Team: Coordinating Center: Fan Liu, Erica Scher; UCSF: Marina Stasenko, Erin Ayash, Melissa Schirmer, Jason Farrell, Mari-Paule Thiet, Laurence Baskin; UMN: Heather L. Gray Chelsea Georgesen, Brooke J. Rody, Carrie A. Terrell, Kapilmeet Kaur; URMC: Erin Brantley, Heather Fiore, Lynda Kochman, Lauren Parlett, Jessica Marino, William Hulbert, Robert Mevorach, Eva Pressman; UW/SCH: Kristy Ivicek, Bobbie Salveson, Garry Alcedo and the families who participated in the study. The authors also thank the TIDES families for their participation and the residents at URMC and UCSF who assisted with birth exams.