In Latin American, Middle Eastern and some European countries, fresh rennet cheeses, such as Queso Blanco, Queso fresco de Burgos, Italian fresh cheese and Halloumi, are produced with little or no culture. Fresh cheeses are particularly susceptible to contamination due to their relatively high pH and moisture content, as well as the absence of competing starter populations. They are usually held at the dairy premises for 24 h under refrigeration, marketed the day after manufacture and consumed within the next 3–7 d, although the labelled expiry date is usually of 14 d. During storage, fresh cheeses may undergo syneresis resulting in a block of cheese embedded within expelled whey. Although some syneresis is desired in order to maintain the wet aspect, familiar to consumers, when excessive, it can worsen the quality of the product.
Milk for the manufacture of starter-free fresh cheeses is generally pasteurised at 70–80°C for 15–40 s. Higher temperatures have adverse effects on curd formation, namely longer coagulation times and weaker gels (Guinee et al. Reference Guinee, Gorry, O'Callaghan, O'Kennedy, O'Brien and Fenelon1997; Singh & Waungana, Reference Singh and Waungana2001), and on curd syneresis producing cheeses with higher moisture content (Walstra et al. Reference Walstra, van Dick and Geurts1985; Pearse & Mackinlay, Reference Pearse and Mackinlay1989; Rynne et al. Reference Rynne, Beresford, Kelly and Guinee2004). In order to break milk fat globules into fine lipid droplets, preventing cream separation, thereby increasing stability and shelf-life of milk emulsion, two-stage homogenisation is usually performed at 60°C with pressures of up to 20 MPa (Wilbey, Reference Wilbey and Roginski2002). Although homogenisation of whole milk has detrimental effects on curd forming properties (Emmons et al. Reference Emmons, Kalab and Larmond1980) and curd syneresis (Humbert et al. Reference Humbert, Driou, Guerin and Alais1980; Green et al. Reference Green, Marshall and Glover1983), it improves rennet action (Humbert et al. Reference Humbert, Driou, Guerin and Alais1980; Robson & Dalgleish, Reference Robson and Dalgleish1984) and increases cheese yield due to better fat recovery (Peters, Reference Peters1956; Peters & Moore, Reference Peters and Moore1958; Jana & Upadhyay, Reference Jana and Upadhyay1992). However, many studies have shown that homogenisation of milk affects the quality of a great variety of cheeses by altering their composition, i.e., moisture, fat and protein content, their body and texture, and their colour (see review by Jana & Upadhyay, Reference Jana and Upadhyay1992). Concerning the technological aspects of the cheese-making process, many studies have shown that homogenisation has a detrimental effect by weakening rennet curds and resulting in shattering and improper curd matting (Peters, Reference Peters1956; Peters & Moore, Reference Peters and Moore1958; Humbert et al. Reference Humbert, Driou, Guerin and Alais1980; Green et al. Reference Green, Marshall and Glover1983; Jana & Upadhyay, Reference Jana and Upadhyay1992; Tunick et al. Reference Tunick, Malin, Smith, Shieh, Sullivan, Mackey and Holsinger1993).
Ultra-high pressure homogenisation (UHPH) has been proven to provoke microbial inactivation of milk through the reduction of total counts and lactococci by 3–4 log cycles (Thiebaud et al. Reference Thiebaud, Dumay, Picart, Guiraud and Cheftel2003; Picart et al. Reference Picart, Thiebaud, René, Guiraud, Cheftel and Dumay2006; Pereda et al. Reference Pereda, Ferragut, Quevedo, Guamis and Trujillo2007), and the complete destruction of coliforms, lactobacilli and enterococci (Pereda et al. Reference Pereda, Ferragut, Quevedo, Guamis and Trujillo2007). The potential of UHPH as alternative to heat pasteurisation to inactivate food-borne pathogens has been also demonstrated in milk (Vachon et al. Reference Vachon, Kheadr, Giasson, Paquin and Fliss2002; Diels et al. Reference Diels, Callewaert, Wuytack, Masschalck and Michiels2005; Briñez et al. Reference Briñez, Roig-Sagués, Hernández-Herrero and Guamis-López2006a, Reference Briñez, Roig-Sagués, Hernández Herrero and Guamis Lópezb). Previous studies on the cheese-making characteristics of UHPH-treated milk have focused on the rennet coagulation properties. UHPH has been proven to enhance the coagulation properties of milk by decreasing the rennet coagulation time and increasing both the curd firming rate and the gel firmness (Hayes & Kelly, Reference Hayes and Kelly2003; Sandra & Dalgleish, Reference Sandra and Dalgleish2007; Zamora et al. Reference Zamora, Ferragut, Jaramillo, Guamis and Trujillo2007; Lodaite et al. Reference Lodaite, Chevalier, Armaforte and Kelly2009). However, to date, the effects of UHPH on the manufacture of starter-free fresh cheese, in order to evaluate UHPH as an alternative to pasteurisation for cheese production, have not yet been studied.
The aim of the present study was to evaluate the cheese-making properties of milk treated by UHPH and to determine the microbial shelf-life of the resulting starter-free fresh cheeses.
Material and Methods
Milk supply and treatment
Raw whole bovine milk was obtained from a local dairy farm (S.A.T. Can Badó, Roca del Vallès, Spain). Milk was standardised at 3·5±0·2% (w/v) fat and kept overnight at 4°C. Before all treatments, the milk was warmed to approximately 20°C. UHPH treatment was carried out by subjecting milk to 300 MPa using a Stansted high-pressure homgeniser (model FPG11300, Stansted Fluid Power Ltd., Harlow, UK) at an inlet temperature of 30±0·5°C. This homogeniser comprised a high-pressure valve made of ceramics able to support 350 MPa and a second pneumatic valve able to support up to 50 MPa located behind the first one. The high pressure system consisted of two intensifiers driven by a hydraulic pump. The flow rate of milk in the homogeniser was 120 l h−1. Inlet temperature of milk was kept at the desired temperature by a heat exchanger located behind the feeding tank (Garvía S.A., Barcelona, Spain). Thermocouples and pressure gauges placed at the two valves measured temperature and pressure changes during processing. In order to minimise temperature retention after treatment, two spiral type heat-exchangers (Garvía S.A.) located behind the second valve were used. In addition, a refrigeration system between the two valves was incorporated in order to minimise the heat effect of the treatment, which allowed an instant cooling and a concomitant decrease of the outlet temperature. Milk temperature reached during treatment was 104±1°C (for ∼0·7 s) at the valve level and 47±2°C just after the refrigeration system. The outlet temperature of milk never exceeded 20 °C. UHPH conditions were selected in order to avoid lipolysis after treatment by the inactivation of milk LPL (Pereda et al. Reference Pereda, Ferragut, Quevedo, Guamis and Trujillo2008).
UHPH-treated milk was compared with conventional treatments. Pasteurised milk (PA; at 80°C for 15 s) and homogenised–pasteurised milk (HPA; with 15+3 MPa at 60 °C, and at 80°C for 15 s) were chosen since these treatments are commonly used for cheese-milk. Two-stage homogenisation and pasteurisation were performed with a Niro Soavi homogeniser (model X68P Matr. 2123, Niro Soavi, Parma, Italy) and a Finamat heat-exchanger (model 6500/010, GEA Finnah GmbH, Ahaus, Germany), respectively.
Particle size and distribution of milk
The particle size distribution in milk samples was determined using a Beckman Coulter laser diffraction particle size analyser (LS 13 320 series, Beckman Coulter, Fullerton, CA) as described by Zamora et al. (Reference Zamora, Ferragut, Jaramillo, Guamis and Trujillo2007). Both volume- and surface-weighted mean diameters [D(4,3) and D(3,2)] were determined.
Cheese production
Milk (60 l) was placed in a 150 l-vat and warmed under manual stirring until coagulation temperature was reached. The pH of the milk was recorded with a portable pH-meter (PH 25, Crison, Alella, Spain) before and after adding sodium chloride (1% v/v) and 35% (w/v) calcium chloride at 0·01% (v/v) in milk. Coagulation of the milk was performed at 32°C for 45 min after addition of chymosin (Maxiren 180, DSM Food Specialties, Seclin Cedex, France) at 0·03% (v/v). Curd was manually cut and kept undisturbed for 15 min at 37°C. Curd grains of about 1 cm, obtained by gently stirring for 10 min, were poured into polypropylene moulds (250 g packaging for Ricotta, ETS A. Coquard, Villefranche-sur-Saône, France). Filled moulds were allowed to drain at 7°C for 90 min, and collected whey was weighted. Packaged cheeses were kept at 4°C during the storage period. The pH of whey was also recorded with a portable pH-meter (PH 25, Crison).
Coagulation properties
Rennet coagulation properties, such as rennet coagulation time (RCT), rate of curd firming (RCF), and curd firmness at 45 min (CF), were assessed on-line with a Gelograph-NT (GelInstrumente, Thalwil, Sweden) as described by Buffa et al. (Reference Buffa, Trujillo and Guamis2001).
Cheese pH and whey expelled during storage
Both packaged cheeses and whey, which remained in the packaging after taking away the cheese for microbiological analysis, were weighted. The amount of expelled whey was calculated as percentage of the packaged cheese weight. The pH was measured with a pH meter (Micro-pH 2001, Crison) in a cheese/distilled water (1:1) slurry.
Microbiological analysis
The microbiological quality of raw and treated milk samples was assessed on day 1. For cheeses, the analysis was carried out every 2 d until the total counts reached at least 6 log cfu g−1. The whole cheese was transferred into a sterile plastic bag and manually crushed. Ten grams of homogenised cheese were diluted with 90 ml of sterile peptone water (Oxoid Ltd, Basingstoke, Hampshire, UK), in a stomacher bag and mixed for 1 min with a paddle blender (Stomacher 400 Circulator, Seward Ltd., London, UK).
Decimal dilutions of both milk and cheese homogenates were performed with peptone water, and microbiological counts of total bacteria, psychrotrophs, lactococci, lactobacilli, coliforms, Escherichia coli, yeasts & moulds, and Staphylococcus aureus were determined. The following media and incubation conditions were used (APHA, 1992): pour-plating in Plate Count Agar (Oxoid) incubated at 30°C for 48 h (total counts) and at 20°C for 72 h (psychrotrophs); pour-plating in M17 Agar and Rogosa Agar (Oxoid) incubated at 30°C for 48 h (lactococci) and 72 h (lactobacilli); pour-plating in Violet Red Bile Agar (Oxoid) and/or ColiID (bioMérieux S.A., Marcy L'Etoile, France), with a covering layer of the same medium, incubated at 37°C for 24 h (coliforms and Esch. coli); pour-plating in Rose-Bengal Chloramphenicol Agar (Oxoid) incubated at 20°C for 5 d (yeasts & moulds); pour-plating in Baird-Parker RPF Agar (Oxoid) incubated at 37°C for 24–48 h (Staph. aureus).
The absence of Listeria monocytogenes and Salmonella spp. in cheese on day 1 was confirmed with modified standard methods (ISO, 1996, 2002, 2004). The method for List. monocytogenes entailed a selective pre-enrichment in half-Fraser broth followed by a selective enrichment in Fraser broth (bioMérieux) and detection with solid agar plates of Palcam (Oxoid) and ALOA (AES Chemunex, Bruz, France). Crushed cheese (25 g) and 225 ml of half-Fraser broth were transferred into a sterile plastic bag and mixed for 1 min with a Stomacher (Seward Ltd.). After 24 h incubation at 30°C, subsequent enrichment was done in Fraser broth and incubated at 37°C for 24 h. The later broth was subcultured by streaking on to the solid agar plates, which were read after incubation at 37°C for 24 h.
For Salmonella spp., the pre-enrichment was done with a non-selective broth, i.e., buffered peptone water, for 24 h at 37°C. Subsequent enrichment was done in two selective broths: Rappaport–Vassiliadis with soy and Muller–Kauffmann Tetrathionate–Novobiocin (bioMérieux). After 24 h incubation at 42°C and 37°C, respectively, the selective broths were subcultured by streaking on to XLD (Oxoid) and SM ID 2 (bioMérieux) agar plates. Reading was carried out after incubation at 37°C for 24 h.
Statistical analysis
The complete experiment was repeated on 3 independent occasions. All analyses were carried out in either duplicate or triplicate. Results were processed by multifactor analysis of variance (ANOVA) using the general linear models procedure of Statgraphics (Statgraphics Inc., Chicago, IL, USA), taking into account both treatment and production factors, as well as their interaction. Either LSD or Tukey's tests were used for comparison of sample data, and evaluations were based on a significance level of P<0·05.
Results and Discussion
Milk: particle size, microbiological quality and coagulation properties
Both particle size distributions of raw and pasteurised milk samples were characterised by a main peak at ∼4 μm and a lower peak at 0·2 μm, which corresponded to fat globules and casein micelle particles, respectively (data not shown). As expected, both homogenisation treatments markedly altered the size distribution of milk. In conventionally treated milk, the main peak was found at ∼0·4 μm followed by a second peak at 1·5 μm; in other words, conventional homogenisation mainly reduced the size of fat globules. In contrast, UHPH-treated milk showed only one peak at ∼0·1 μm. Thus, UHPH triggered a greater reduction of the fat globule size together with some disruption of casein micelles. Indeed, Sandra & Dalgleish (Reference Sandra and Dalgleish2005) reported a decreased micelle average size in skimmed milk due to dissociation of their surface rather than complete dissociation. Consequently, both D(4,3) and D(3,2) of milk differed significantly (P<0·05) depending on the treatment (Table 1). UHPH resulted in significantly lower values than conventional homogenisation–pasteurisation. The obtained results were broadly in accordance with previous studies (Hayes & Kelly, Reference Hayes and Kelly2003; Zamora et al. Reference Zamora, Ferragut, Jaramillo, Guamis and Trujillo2007).
Table 1. Particle size (nm) of raw, pasteurised, pasteurised–homogenised, and ultra-high pressure homogenised milk samples†

a–dMean value±se; n=9; values without common superscripts were significantly different (P<0·05) by LSD test
† D(4,3), volume-weighted mean diameter; D(3,2), surface-weighted mean diameter
‡ PA, pasteurisation; HPA, homogenisation–pasteurisation; UHPH, ultra-high pressure homogenisation
For total bacteria and psychrotrophs, conventional treatments provoked reductions of ∼2·2 and ∼2·7 log cfu ml−1, respectively (Table 2). Conventionally homogenised–pasteurised milk samples showed significantly lower amounts of lactococci than pasteurised milk samples; however, their reductions were within the same logarithm (2·6 vs. 2·3 log cfu ml−1). Significantly greater reductions were obtained by UHPH treatment, i.e., reductions of ∼3 log cfu ml−1. Lactobacilli, coliforms, Esch. coli, Staph. aureus, yeasts and moulds were not detected in either conventionally or UHPH-treated milk samples. These results are broadly in accordance with those obtained in previous studies (Pereda et al. Reference Pereda, Ferragut, Quevedo, Guamis and Trujillo2007; Smiddy et al. Reference Smiddy, Martin, Huppertz and Kelly2007).
Table 2. Milk microbiology (log cfu ml−1 milk)

a–dMean value±se; n=6; n.d.: not detected with a detection limit of 1 cfu ml−1; values in rows without common superscripts were significantly different (P<0·05) by Tukey's test
† TC, total bacteria; PSY, psychrotrophs; LC, lactococci; LB, lactobacilli; COL, coliforms; EC, Escherichia coli; YM, yeasts & moulds; SA, Staphylococcus aureus
‡ PA, pasteurisation; HPA, homogenisation–pasteurisation; UHPH, ultra-high pressure homogenisation
Concerning the coagulation properties of milk samples, no significant differences (P<0·05) were observed between conventional treatments for RCT (Table 3). In contrast, UHPH decreased RCT from 17 min down to 14 min. Shortening of RCT has been attributed to a casein-spreading effect produced by UHPH (Zamora et al. Reference Zamora, Ferragut, Jaramillo, Guamis and Trujillo2007). The reduction of fat globules with a concomitant increase of their interfacial surface together with partial dissociation of casein micelles would trigger the adsorption of caseins to the newly formed fat globules increasing the availability of κ-caseins and reducing the critical level for chymosin action. Moreover, conventional pasteurisation–homogenisation led to significantly lower RCF compared with pasteurisation, which resulted in lower CF at 45 min. In contrast, UHPH increased RCF, which, combined with lower RCT, resulted in significantly higher CF compared with pasteurisation treatment. Such results are in accordance with those obtained in a previous study on the rennet coagulation properties of UHPH-treated milk under laboratory conditions by using an Optigraph® probe (Zamora et al. Reference Zamora, Ferragut, Jaramillo, Guamis and Trujillo2007).
Table 3. Coagulation properties of milk†

a–cMean value±se; n=6; values without common superscripts were significantly different (P<0·05) by LSD test
† RCT, rennet coagulation time; RCF, rate of curd firming; CF, curd firmness at 45 min
‡ PA, pasteurisation; HPA, homogenisation–pasteurisation; UHPH, ultra-high pressure homogenisation
Technological aspects of cheese-making
The addition of sodium and calcium chloride salts caused a slight decrease in pH (Table 4). Rennet coagulation of milk is pH-dependent, with optimum activity for hydrolysis of the Phe105-Met106 in κ-casein at pH 6·0 (Banks & Horne, Reference Banks, Horne and Roginski2002). Decreasing the natural pH of milk also encourages gel formation by solubilisation of calcium phosphate and a decrease in the charge on the casein micelles. The slight decrease in pH provoked by the addition of calcium chloride promotes the primary enzymatic phase of renneting, while the secondary stage of aggregation is enhanced by calcium addition (Banks & Horne, Reference Banks, Horne and Roginski2002). Since no starter was used, further acidification of the milk was not observed. Whey pH at the end of the production was similar to that of the initial milk supplemented with salt and calcium chloride. No significant differences were observed between treatments indicating that all productions were carried out under the same conditions.
Table 4. Milk and whey pH and whey drainage
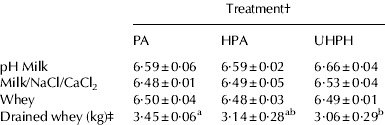
a,bMean value±sd; n=3; values without common superscripts were significantly different (P<0·05) by LSD test
† PA, pasteurisation; HPA, homogenisation–pasteurisation; UHPH, ultra-high pressure homogenisation
‡ Total amount of whey collected from all cheeses after 90 min of drainage through gravitational forces
The transfer of milk into the vat provoked great foaming for UHPH-milk, which was visually whiter and less viscous than those conventionally treated. In fact, UHPH-milk somewhat resembled skim milk. The analysis of milk colour and viscosity performed in our laboratories by Pereda et al. (Reference Pereda, Ferragut, Quevedo, Guamis and Trujillo2007) showed that UHPH at 300 MPa with an inlet temperature of 30°C provoked whitening of the milk by increasing the lightness and decreasing both a* and b* colour components, but did not affect the viscosity of milk.
After 45 min of coagulation, some spontaneous expelled whey was observed at the surface of undisturbed curds from PA-milk. Indeed, syneresis in curd occurs as coagulation takes place by a change in solubility, and rearrangement and shrinkage of the network (Walstra et al. Reference Walstra, van Dick and Geurts1985). Although gels formed from UHPH-milk showed analytically higher firmness, the cutting was somehow more difficult; some attempts were made in order to use both horizontal and vertical blade knives, but the former provoked great destruction of the curds from both HPA- and UHPH-samples.
Homogenisation of milk, especially UHPH, provoked lower amounts of expelled whey. Whey obtained from UHPH-milk was visually more transparent and greenish than those of conventionally treated samples. However, during stirring, the curd was somewhat brittle resulting in curd shattering and a concomitant increase of curd fines in whey. When using raw or pasteurised milk, two main reasons are responsible for curd shattering. If it occurs at an early stage, i.e., when cutting the curd, it indicates that the curd was not firm enough to be cut and a longer coagulation time would solve the problem. In contrast, if shattering occurs when stirring the curd, it indicates that the size of the blocks after cutting were too big, since big grains tend to shatter; thus reducing the size of the blade knifes would solve the problem. However, in the present study, no shattering was observed in curds from PA-milk; since it was a peculiarity of homogenised milk, no changes in the technological process itself were done.
The cohesion of the grains was impaired by UHPH treatment of milk, which led to difficulties in obtaining homogeneous grains. Moreover, during moulding, grains did not get completely packed due to poor deformability. Such difficulties were also found to a lesser extent with HPA-milk. Indeed, homogenisation affects protein structure and causes casein micelles and whey proteins to become associated with the fat globule membrane (Michalski et al. Reference Michalski, Cariou, Michel and Garnier2002). These interactions between fat and proteins lead to lower curd firmness during rennet coagulation, curd shattering during cutting, and improper curd matting (Peters, Reference Peters1956; Peters & Moore, Reference Peters and Moore1958; Humbert et al. Reference Humbert, Driou, Guerin and Alais1980; Green et al. Reference Green, Marshall and Glover1983; Jana & Upadhyay, Reference Jana and Upadhyay1992; Tunick et al. Reference Tunick, Malin, Smith, Shieh, Sullivan, Mackey and Holsinger1993).
Both homogenisation treatments reduced the amount of whey expelled by gravitation before storage compared with pasteurisation treatment (Table 4). However, the effect of UHPH was more important than that of conventional homogenisation–pasteurisation (11% vs. 9% reduction, respectively). Indeed, homogenisation of milk increases moisture content of grains, by decreasing the amount of whey expelled, and decreases fat losses to whey (Peters, Reference Peters1956; Green et al. Reference Green, Marshall and Glover1983; Jana & Upadhyay, Reference Jana and Upadhyay1992).
Cheese pH and whey expelled during storage
Independently of milk treatment, the initial pH of all cheeses ranged from 6·7 to 6·8. These values are in accordance with those obtained by Hernando (Reference Hernando1998) with starter-free fresh cheese (Burgos cheese) made from pasteurised milk. During the storage period (13 d for PA- and HPA-cheeses, and 19 d for UHPH-cheeses), almost no changes in the pH of cheeses were observed (Fig. 1).

Fig. 1. Evolution of whey drainage (solid lines; g 100 g−1 cheese) and pH (dotted lines) in cheeses from pasteurised (light grey), homogenised–pasteurised (dark grey), and ultra-high-pressure homogenised (black) milk samples.
Concerning the amount of whey expelled during storage, significant differences between treatments were already observed at day 1 (Fig. 1); expelled whey represented 17, 10 and 7% of the total weight in PA-, HPA- and UHPH-cheeses, respectively. Indeed, moisture content of fresh-cheeses varied significantly from 67 to 70 g per 100 g cheese (Zamora et al. Reference Zamora, Ferragut, Juan, Guamis and Trujillo2011). During storage, a sharp increase was observed throughout the first week; since the rate for cheeses from homogenised milk samples was slightly higher than that of PA-cheeses, smaller differences in the amount of whey expelled at day 7 were observed, especially between PA- and HPA-cheeses. Confocal microscopy of starter-free fresh cheeses showed textural differences (Zamora et al. Reference Zamora, Ferragut, Juan, Guamis and Trujillo2011). The matrices of HPA- and, specially, PA-cheeses showed discrete serum pockets or channels from which whey could drain. On the contrary for UHPH-cheeses, micrographs showed a continuous and dense protein matrix with no serum pockets. A decrease in the amount of whey expelled between 7 and 9 d was observed in both HPA- and UHPH-cheeses. Hernando (Reference Hernando1998) estimated whey drainage by centrifugation and observed a slight decrease during the first week, followed by a great increase during the second week and no further changes beyond 14 d. Although not being comparable with the results of the present study, since whey drainage was estimated by centrifugation, these results confirm that by 7 d, unknown changes in the cheese, most probably related to a rearrangement of the cheese matrix such as compaction and disappearance of the discrete serum pockets, must have taken place.
Microbiological quality and shelf-life of cheese
As previously mentioned, starter-free fresh cheeses are particularly susceptible to contamination due to their relatively high pH and moisture content as well as the absence of competing starter populations. Pasteurisation of the milk used in the manufacture of such cheeses generally kills pathogens, i.e., Staph. aureus, Salmonella spp. and List. monocytogenes, that could be present in the starting milk substrate. However, this does not alleviate concerns regarding post-processing contamination, such as improper handling or storage by the manufacturer or the consumer.
In the present study, the screening for pathogens confirmed that all cheese samples were free of List. monocytogenes and Salmonella spp. Moreover, Staph. aureus and Esch. coli were not detected throughout the storage period. UHPH-cheeses presented the lowest rate of growth of total bacteria and psychrotrophs (Table 5), with a concomitant increase in the shelf-life of cheeses, i.e., when 6 log cfu g−1 were reached, from 11–13 (PA- and HPA-cheeses) to 17–19 d. Since cheeses were stored at ∼4°C, psychrotrophs growth was enhanced resulting in counts close to those of total bacteria. Concerning specific microbial populations, in the present study, lactobacilli were not detected throughout the storage period regardless of the applied treatment. However, lactococci were the main population among those studied (Table 5). Major differences were observed between UHPH- and cheeses from conventionally treated milk samples; UHPH-cheeses showed significantly lower counts of lactococci than PA- and HPA-cheeses on day 1; however, by day 5, counts did not differ. Pereda et al. (Reference Pereda, Ferragut, Quevedo, Guamis and Trujillo2007) also observed higher counts of lactococci in UHPH-treated milk during storage at 4°C from day 14. UHPH might have triggered some unknown changes in the milk which enhanced the growth of lactococci in cheese at an early stage of the storage period. The heat effect of UHPH has been proven to be less than that of conventional pasteurisation through the determination of thermal indicators, e.g., hydroxymethylfurfural and total sulphydryl content of milk (Pereda et al. Reference Pereda, Ferragut, Quevedo, Guamis and Trujillo2009), thus UHPH could be less aggressive towards thermolabile compounds, which could act as growth factors. Yeast and moulds presented higher variability. However, their general trends were to increase over time (Table 5). Lower levels were observed in UHPH-cheeses especially at the end of the shelf-life.
Table 5. Cheese microbiology (log cfu g−1 cheese)
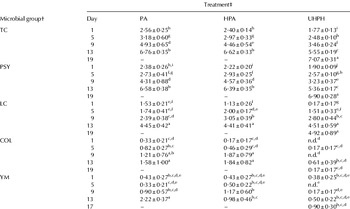
a–jMean value±se; n=6; n.d.: not detected with a detection limit of 10 cfu g−1; values per microbial group without common superscripts were significantly different (P<0·05) by Tukey's test
† TC, total bacteria; PSY, psychrotrophs; LC, lactococci; COL, coliforms; YM, yeasts & moulds
‡ PA, pasteurisation; HPA, homogenisation–pasteurisation; UHPH, ultra-high pressure homogenisation
Conclusions
In contrast to conventional homogenisation, UHPH enhanced the coagulation properties of milk. However, both homogenisation treatments impaired to some extent the cheese-making, i.e., difficulty at cutting the curd due to crumbling and improper curd matting due to poor cohesion of the grains. UHPH-treated cheese-milk had higher microbial quality than milk obtained by conventional treatments. During storage, cheeses from UHPH-treated milk expelled less whey than those from conventionally treated milk samples; UHPH treatment prevented the excessive syneresis which worsens the quality of fresh cheeses by resulting in a block of cheese embedded within expelled whey. In addition, UHPH-treatment of milk delays microbial growth in fresh cheeses with a concomitantly longer microbiological shelf life; the growth of lactococci was somehow enhanced at an early stage, but yeasts and moulds were inhibited throughout the storage period. In conclusion, UHPH treatment could be an alternative to conventional treatments of milk for fresh cheese production in order to obtain longer shelf-life, although the need of some modifications on the traditional process of fresh cheese manufacture is foreseen.
The authors acknowledge the Ministerio de Educación y Ciencia (AGL2004-01943) and the Commission of the European Communities (EU project 512626) for the financial support given to this investigation. Anna Zamora acknowledges the predoctoral fellowship from the Ministerio de Educación y Ciencia.