Lactobacillus delbrueckii subsp. bulgaricus is one of the two bacteria required for commercial yogurt production and is used synergistically with Streptococcus thermophilus on an industrial scale. Lactobacillus delbrueckii subsp. bulgaricus is also an important member of the uniquely diverse group of lactic acid bacteria (LAB) (Gyawali et al., Reference Gyawali, Oyeniran, Zimmerman, Aljaloud, Krastanov and Ibrahim2020) that has many industrial applications. LAB are generally used as starter cultures for the production of fermented dairy products (such as yogurt) and are also considered as probiotics due to their potential human health benefits (Aponte et al., Reference Aponte, Murru and Shoukat2020). In addition, Lactobacillus bulgaricus plays a vital role in the development of the organoleptic (Petry et al., Reference Petry, Furlan, Crepeau, Cerning and Desmazeaud2000) and probiotic properties of yogurt. Fermented functional foods containing Lb. bulgaricus are believed to enhance human health and promote wellness (Hayek et al., Reference Hayek, Gyawali, Aljaloud, Krastanov and Ibrahim2019; Ayivi et al., Reference Ayivi, Gyawali, Krastanov, Aljaloud, Worku, Tahergorabi, Silva and Ibrahim2020). Moreover, it has been reported to be a safe probiotic with several health benefits when administered in adequate amounts at an effective dose (Adolfsson et al., Reference Adolfsson, Meydani and Russell2004). The flavor, texture and organoleptic properties of yogurt are generally a result of the symbiotic interaction of Lb. bulgaricus and Streptococcus thermophilus. Consequently, an imbalance in these two bacteria will affect the sensorial characteristics and qualities of yogurt as a fermented dairy product (Sieuwerts, Reference Sieuwerts2016).
Lb. bulgaricus as a starter culture is a vital component of many fermentation processes. Consequently, their fermentation performance and functionality are enhanced based on their nutritional requirements and the overall enrichment of the fermentation medium (Ayivi et al., Reference Ayivi, Gyawali, Krastanov, Aljaloud, Worku, Tahergorabi, Silva and Ibrahim2020). The food or dairy industry is constantly exploring and in search of various probiotic bacteria for many food product applications with cost-effective fermentation processes including growth media requirements (Akabanda et al., Reference Akabanda, Owusu-Kwarteng, Tano-Debrah, Glover, Nielsen and Jespersen2013; Hayek and Ibrahim, Reference Hayek and Ibrahim2013; Mani-López et al., Reference Mani-Lopez, Palou and Lopez-Malo2014). Generally, the nutritional quality of the fermentation medium is enhanced by the supplementation of casein hydrolysates and yeast extract, which are key nitrogen sources that act as growth-promoters boosting high bacterial cell growth (Norton et al., Reference Norton, Lacroix and Vuillemard1994; Hayek and Ibrahim, Reference Hayek and Ibrahim2013). Growth media supplementation with hydrolyzed proteins essentially releases more nitrogen for uptake by LAB, thereby enhancing the bacteria's biofunctionality (Dąbrowska et al., Reference Dąbrowska, Babij, Szołtysik and Chrzanowska2017; Ponomarova et al., Reference Ponomarova, Gabrielli, Sévin, Mülleder, Zirngibl, Bulyha, Andrejev, Kafkia, Typas, Sauer, Ralser and Patil2017; Berisvil et al., Reference Berisvil, Astesana, Zimmermann, Frizzo, Rossler, Romero Scharpen, Olivero, Zbrun, Signorini Porchiett, Sequeira, Drago and Soto2020).
Significant biomass levels and high volumes of lactic acid production by LAB species have also been attributed to the presence of amino acids, vitamins and yeast extract in the fermentation medium (Alazzeh et al., Reference Alazzeh, Ibrahim, Song, Shahbazi and AbuGhazaleh2009; Manzoor et al., Reference Manzoor, Qazi, ul Haq, Mukhtar and Rasool2017; Ayad et al., Reference Ayad, El-Rab, Ibrahim and Williams2020). Researchers have recommended calcium supplementation for growth media development as it helps to promote bacterial cell division which impacts high cell counts (Wright and Klaenhammer, Reference Wright and Klaenhammer1983). Calcium or skim-milk powder is also typically used as a cryoprotectant in the dairy industry for probiotic cells during freeze-drying operations in order to significantly limit cell viability losses (Wright and Klaenhammer, Reference Wright and Klaenhammer1983; Chervaux et al., Reference Chervaux, Ehrlich and Maguin2000; Fenster et al., Reference Fenster, Freeburg, Hollard, Wong, Rønhave Laursen and Ouwehand2019).
The freeze-drying of LAB cells is also highly dependent on the growth medium, fermentation pH, cryoprotectant, and other intrinsic factors (Carvalho et al., Reference Carvalho, Silva, Ho, Teixeira, Malcata and Gibbs2004). This was also confirmed by a study by Chen et al. (Reference Chen, Huang, Shi, Li and Liu2017) who reported how a supplemented MRS medium significantly impacted the growth and freeze-drying viability of Lb. bulgaricus strains. An enriched growth medium has also been credited with improving the freeze-drying survival rate of LAB due to the medium's composition (carbon, nitrogen and lipids such as Tween 80) which efficiently helps to reduce bacterial stress during the freeze-drying process (Li et al., Reference Li, Liu and Liu2012).
However, the limiting factor for supplementing fermentation media with high amounts of nitrogen source is linked to its exorbitant cost (Manzoor et al., Reference Manzoor, Qazi, ul Haq, Mukhtar and Rasool2017; Hayek et al., Reference Hayek, Gyawali, Aljaloud, Krastanov and Ibrahim2019). Moreover, the standard LAB fermentation medium deMann, Rogosa and Sharpe (MRS) is also expensive due to its nitrogen source (meat, peptone and yeast extract) and does not support the growth of all LAB and probiotic cultures. Available literature shows that significant research is warranted in order to find alternative cost-effective ingredients that can help to lower the cost of LAB growth media as well as obtain higher cell densities. Thus, the objective of this study was to investigate the growth and viability of Lb. bulgaricus cultivated with alternative nitrogen sources and to assess its impact on viability after freeze-drying. We hypothesized that alternative nitrogen sources such as selected yeast extracts from the X-Seed brand of complex nitrogen sources could efficiently support the fermentation performance and viability of Lactobacillus delbrueckii subsp. bulgaricus.
Materials and methods
Source of Lactobacillus bulgaricus and pre-culture fermentation medium
Ten Lb. bulgaricus strains (online Supplementary Table S1) were used for the preliminary study (all data not shown). Two strains (S9 and LB6) were then selected for the main study based on their growth rates. The origin of all strains is elucidated in the online Supplementary File. We used deMan, Rogosa Sharpe (MRS, Neogen Co, Michigan, USA), as the pre-culture fermentation medium. The detailed pre-culture fermentation procedure is shown in the online Supplementary File.
Optimized lactobacilli growth medium and validation of nitrogen sources
The optimized lactobacilli growth medium was prepared after various nitrogen sources (online Supplementary Table S3) and their total nitrogen content (online Supplementary Table S2) were validated. The optimized growth medium had a similar composition to that of MRS (online Supplementary Table S4). However, the nitrogen sources were replaced by X-Seed Nucleo Max, and optimized proportions of blends of X-Seed KAT, and X-Seed Carbo Max as alternative nitrogen sources. Online Supplementary Table S5 shows the summarized composition of MRS and the optimized medium.
Determination of pH and buffering capacity with l-arginine and l-histidine supplementation
The pH values of each medium were measured in duplicate at the start and end of fermentation. Buffering capacity of all media were evaluated with 4 g/l of l-arginine and l-histidine respectively. The online Supplementary File highlights the pH and the buffer capacity determination.
Bacterial enumeration in growth media supplemented with and without l-histidine
Bacterial enumeration for the KCMax media blends with l-histidine supplementation is detailed in the online Supplementary File. All plates were anaerobically incubated for 48 h at 42°C and plates with 25–250 colonies were counted. Bacterial populations were expressed in log CFU/ml.
Impact of calcium on the growth, freeze-stability and viability of Lb. bulgaricus
The optimized KCMax (10/10) medium was supplemented with different concentrations of calcium and inoculated with the S9 bulgaricus strain as detailed in the online Supplementary File. Freeze-dried cells from calcium supplemented KCMax (10/10) medium were enumerated by the bacterial count method.
Statistical analyses
SAS version 9.4 (Cary, NC, USA) was used for data analysis. One-way Analysis of Variance (ANOVA) was used to determine significant differences between the values obtained for the final optimized growth media blends but not for the preliminary study. Significant differences (P < 0.05) between treatment means were compared using Tukey's test. Bacterial population counts were expressed as log 10 before analyses were made.
Results and discussion
Preliminary study (validation of the nitrogen source on the growth of Lb. bulgaricus strains)
Evaluation of the nitrogen source on the growth of the different strains of Lb. bulgaricus was done in the preliminary study. Some evaluated strains such as the S9 and LB6 were fast-growing (OD610 nm), thus explaining their selection for the main study. The optimized medium had a basal formula with 2 g/l of Nucleo-Max (N-Max) in conjunction with various blends of KAT (K) and Carbo-Max (C-Max) (see results under online Supplementary File).
Growth and cell density in the final optimized growth media
The S9 and LB6 bulgaricus strains demonstrated varied growth patterns during twelve hours of fermentation at 42°C with confirmation of their viability in MRS. There was a significant difference (P < 0.05) in the OD610 nm results for both strains in the KAT and Carbo-Max media blends, with growth in the KCMax (10/10) medium outperforming that in MRS (Figs. 1a and 1b). The auxotrophic and fastidious nature of Lb. bulgaricus warrants supplementing the growth media with amino acids, peptides and vitamins, hence, yeast extracts are recommended as complex nitrogen sources for growth promotion (Atilola et al., Reference Atilola, Gyawali, Aljaloud and Ibrahim2015; Curk et al., Reference Curk, Peladan and Hubert1993; Klotz et al., Reference Klotz, Kuenz and Prüße2017). Bacterial growth in the MRS and KCMax media blends suggested their nitrogen content impacted bacterial growth (Hossain et al., Reference Hossain, Akter, Humayan, Mohanto, Begum and Ahmed2020; Papizadeh et al., Reference Papizadeh, Rohani, Hosseini, Shojaosadati, Nahrevanian, Talebi and Pourshafie2020). Generally, nutritional requirements for LAB growth are strain-dependent (Degeest and De Vuyst Reference Degeest and De Vuyst1999; Saguir and de Nadra, Reference Saguir and de Nadra2007), thus, alternative yeast extracts (X-Seed Nucleo Max, KAT, and Carbo Max) in the optimized medium demonstrated promising results as compared to conventional MRS. A basal medium (2 g/l of X-Seed Nucleo Max: N-Max) with KCMax (10/10) i.e. 10 g/l each of X-Seed KAT (K) and X-Seed Carbo Max (C-Max) yielded superior results to those of MRS. To obtain superior LAB growth (1.34 and 1.79 at OD610 nm) for S9 and LB6 strains respectively, the KCMax (10/10) medium is highly recommended, as compared to MRS (0.89 and 1.42 at OD610 nm) respectively.

Fig. 1. (a) Changes in the growth of S9 Lb. bulgaricus strain at OD610 nm in the different final growth media. The media included the following: MRS, basal medium (N-Max), and the basal medium supplemented with different nitrogen sources (KAT: K, and Carbo-Max: C-Max) at different proportions and blends after 12 h of fermentation at 42 °C. (b) Changes in the growth of LB6 Lb. bulgaricus strain at OD610 nm in the different final growth media. The media included the following: MRS, basal medium (N-Max), and the basal medium supplemented with different nitrogen sources (KAT: K, and Carbo-Max: C-Max) at different proportions and blends after 12 h of fermentation at 42 °C.
Enumeration of Lb. bulgaricus strains
There was a significant difference (P < 0.05) in the bacterial counts between the basal medium (N-Max), and all evaluated media blends for both evaluated strains. However, no significant difference (P > 0.05) in bacterial counts for the MRS and the KCMax media blends was recorded. Moreover, the bacterial count reported by the KCMax (10/10) medium for both strains were 7.2 and 6.5 log CFU/ml (S9 and LB6 respectively) as compared to MRS (7.6 and 7.1 log CFU/ml respectively). This suggested both media were efficient and promoted the viability of the bulgaricus strains (Savijoki et al., Reference Savijoki, Suokko, Palva and Varmanen2006). Thus, the KCMax media blend could be an alternative to MRS as a growth medium (Polak-Berecka et al., Reference Polak-Berecka, Waśko, Kordowska-Wiater, Podleśny, Targoński and Kubik-Komar2010) and will potentially support probiotic growth (Grześkowiak et al., Reference Grześkowiak, Endo, Collado, Pelliniemi, Beasley and Salminen2013). Table 1 shows the bacterial counts of the S9 and LB6 strains.
Table 1. Bacterial count (mean ± SD; n = 3) of Lactobacillus bulgaricus strains expressed as log CFU/ml in different growth media after incubation at 42°C for 48 h

a,b,c,d Means with different superscripts within a row differ significantly (P < 0.05).
pH value changes in the different growth media
The initial pH values were adjusted to 6.5 before fermentation commenced. However, at the end of fermentation, the S9 strain exhibited a rapid decline in pH (between 5.33–5.62) for all evaluated media (MRS, KAT, and blends of KAT and Carbo-Max). This pH decline was also observed for the LB6 strain with post-fermentation pH values between 5.06–5.72. There was a significant difference (P < 0.05) for the optimized medium in comparison to MRS (Liu et al., Reference Liu, Yu, Sun, Song, Wang, Wang, Wuren, Zha, Menghe and Heping2016). There was no significant difference (P > 0.05) for the N-Max and C-Max medium after fermentation. The observed pH changes for the various media (online Supplementary Fig. S3) could be attributed to the nitrogen sources in the media that impacted the media's buffering capacity.
Buffering capacity of growth media with l-arginine and l-histidine supplementation
Buffering capacity of a growth medium is essential, as it provides a stable pH for enhanced LAB metabolic activity. This stable pH promotes high cell density (biomass), as well as limiting the production of organic acids such as lactic acid, which could cause cell injury thereby decreasing growth (Hayek and Ibrahim, Reference Hayek and Ibrahim2013). The buffering capacity was thus evaluated with two essential amino acids (l-arginine and l-histidine). Significant changes in buffering capacity were only observed with l-histidine supplementation. Generally, the three non-supplemented KCMax media blends had low buffering values (15.24, 15.24, and 15.50 respectively) as compared to non-supplemented MRS (21.57). The l-histidine supplemented blends; KCMax (5/10), KCMax (10/5), and KCMax (10/10), had the highest significant buffering values of 36, 36.5, and 39 respectively (P < 0.05) as compared to the l-histidine supplemented MRS (35.22). It was evident that the KCMax (10/10) medium with l-histidine had the highest value (39) and thus promoted enhanced biomass production during fermentation, thus is recommended for LAB fermentations. Online Supplementary Table S6 summarizes the different buffering capacities for the l-arginine and l-histidine supplemented media. In addition, online Supplementary Figs S4 to S13 inclusive report the different pH buffering of the evaluated amino acids.
Impact of l-histidine supplementation on the growth of S9 Lb. bulgaricus strain
Supplementing the growth media KCMax (5/10), KCMax (10/5), and KCMax (10/10) with varying l-histidine concentrations (4 g/l or 0.4% and 0.5 g/l or 0.005%) impacted cell growth during fermentation (Aumiller et al., Reference Aumiller, Stevens, Scheffler, Guvener, Tung, Grimaldo, Carlson, Deutschbauer, Taga, Marco and Ludington2021). The bacterial count for the buffered MRS and the KCMax blends (0.4% and 0.005% l-histidine) elucidated its impact on strain viability. There was no significant difference (P > 0.05) for bacterial counts for the 0.4% l-histidine supplemented and the non-supplemented media. There was, however, a significant difference (P < 0.05) for the bacterial count for the 0.005% l-histidine supplementation (online Supplementary Fig. S13). In general, the unbuffered control media (MRS and the KCMax blends) had higher counts than the l-histidine supplemented media. The KCMax (10/10) medium did, however, have the same count (8.1 log CFU/ml) for both 0.4% and 0.005% l-histidine treatments. This infers a higher nitrogen blend such as KCMax (10/10) could mask l-histidine's impact. Also, cell morphologies were larger and more aggregated (Fig. 2) after l-histidine treatment than in the control sample. Zeidan et al. (Reference Zeidan, Zara, Viti, Decorosi, Mannazzu, Budroni, Giovannetti and Zara2014) also confirmed that l-histidine modified the cell wall of yeast bacteria. Furthermore, the reduced cell viability could be attributed to the inhibitory effect of l-histidine (Lamberti et al., Reference Lamberti, Purrotti, Mazzoli, Fattori, Barello, Coïsson, Guinta and Pessione2011; Suzuki et al., Reference Suzuki, Ishii, Li, Kawai, Masuda and Oda2014; Zeidan et al., Reference Zeidan, Zara, Viti, Decorosi, Mannazzu, Budroni, Giovannetti and Zara2014).
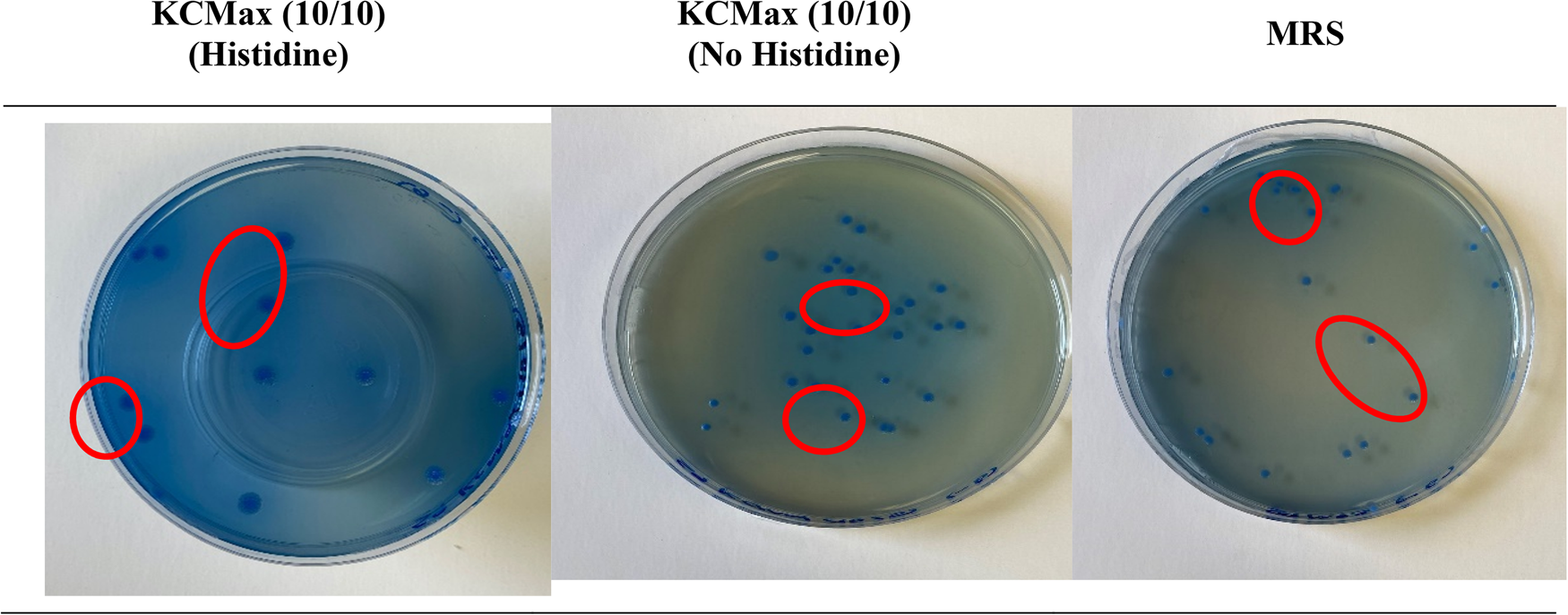
Fig. 2. Morphology of Lb. bulgaricus colonies (encircled in red) on the growth media plates: KCMax (10/10) supplemented with L- histidine, KCMax (10/10) without l-histidine supplementation and (MRS).
Calcium effect on the growth and viability of Lactobacillus delbrueckii subsp. bulgaricus
The KCMax 10/10 blend with calcium supplementation had a significant impact on the growth of Lb. bulgaricus (Chervaux et al., Reference Chervaux, Ehrlich and Maguin2000). The cell-wash treatment after fermentation decreased the total calcium absorbed by the cell. It was thus observed that the control sample had significant (P < 0.05) bacterial counts (8.2 and 8.6 log CFU/ml, respectively) for the unwashed and washed S9 bulgaricus cells. Calcium supplementation (0.4%) for both unwashed and washed cell treatments had bacterial counts of 8.3 and 8.4 log CFU/ml respectively. There was higher calcium content in the unwashed cells (256.4 ppm) than in the washed cells (125.8 ppm). Furthermore, the 0.8% calcium supplementation for both unwashed and washed cells had bacterial counts of 7.9 and 8.2 log CFU/ml respectively. Calcium content was thus directly proportional to the cell treatment, hence, calcium supplementation (1.2%) corresponded to the calcium content of 616.6 ppm (unwashed) and 585.5 ppm of (washed) treatments (online Supplementary Fig. S15). However, this concentration (1.2%), decreased bacterial count significantly (P < 0.05) for both unwashed (7.5 log CFU/ml) and washed cell (7.7 log CFU/ml) treatments (online Supplementary Fig. S16). Thus, excessive calcium content is detrimental to the viability of Lb. bulgaricus strains (Isshiki and Azuma, Reference Isshiki and Azuma1995). Dixon et al. (Reference Dixon, Flint, Palmer, Love, Chabas and Beuger2018), also confirmed that the excess addition of 0.5 M calcium inhibited biofilm formation in dairy wastewater.
The effect of calcium on the freeze-stability and viability of Lb. bulgaricus after freeze-drying
Calcium as a cryoprotectant aids in minimizing probiotic cell viability after freeze-drying (Wright and Klaenhammer, Reference Wright and Klaenhammer1983; Fenster et al., Reference Fenster, Freeburg, Hollard, Wong, Rønhave Laursen and Ouwehand2019). Initial bulgaricus counts for both media (KCMax (10/10) and MRS) was 8.5 log CFU/ml. The population counts for the treatments with and without (0.4%) calcium supplementation in MRS after overnight freezing (−80°C) were 8.9 and 8.8 log CFU/ml respectively. Furthermore, the KCMax (10/10) medium under the same treatments, had higher population counts (9.2 and 9.1 log CFU/ml respectively). There was, however, no significant difference (P > 0.05) between counts for both media after freezing. It was noteworthy that the freeze-dried cell counts with and without calcium supplementation in MRS decreased to 6.5 and 5.8 log CFU/ml respectively. The same trend was observed for the KCMax (10/10) freeze-dried cells and counts were 6.7 and 5.2 log CFU/ml respectively (Fig. 3). Thus calcium-treated cells had slightly higher counts than non-calcium-treated cells for both media. The freeze-dried cell counts, however, increased after recovery in skimmed milk which could be attributed to the resuscitation of stressed and injured cells after freeze-drying (Celik and O'Sullivan, Reference Celik and O'Sullivan2013). The MRS recovered freeze-dried cell counts were 7.8 and 6.5 log CFU/ml respectively with and without calcium treatment. Consequently, the KCMax (10/10) recovered freeze-dried cell counts were also 7.8 and 7.3 log CFU/ml respectively. The effect of calcium and milk recovery on probiotic viability has been confirmed by Wright and Klaenhammer (Reference Wright and Klaenhammer1981, Reference Wright and Klaenhammer1983).

Fig. 3. Viability and bacterial count of S9 Lb. bulgaricus strain before and after freezing and freeze-drying with and without 0.4% calcium supplementation after fermentation in MRS and KCMax (10/10) medium.
In conclusion, our results demonstrated that X-Seed Nucleo Max, KAT, and Carbo Max efficiently supported the growth and viability of Lb. bulgaricus. l-Histidine enhanced the buffering capacity of the KCMax 10/10 medium. Consequently, 0.4% l-histidine supported higher bacterial growth than 0.005% and caused cell aggregation and larger cell morphology than that observed in MRS. Research is warranted to understand l-histidine's mechanism on the growth of Lactobacillus species as only a few studies are available. The calcium supplemented KCMax (10/10) medium enhanced cell viability after freezing and freeze-drying, resulting in high bacterial counts and more rounded cell morphologies than in MRS. A future study is thus needed to examine the morphological change due to calcium. Our optimized medium has cryoprotectant properties and can potentially support the growth and viability of Lb. bulgaricus and is thus recommended for LAB fermentations.
Supplementary material
The supplementary material for this article can be found at https://doi.org/10.1017/S0022029922000541
Acknowledgements
This publication was made possible by grant number NC.X-267-5-12-170-1 from the National Institute of Food and Agriculture (NIFA) and in part by NIZO Food Research BV, The Netherlands, Ohly GmbH, Germany, THT Prayon, Belgium, Jarrow Formulas, USA, and the Department of Family and Consumer Sciences and the Agriculture Research Station at North Carolina Agriculture and Technical State University (Greensboro, NC 27411, USA).