In response to consumer demand for foods with health benefits, the food industry began to diversify its products. Although most foods containing probiotic bacteria are dairy products, there is also a growing demand for incorporating probiotics in other food products. Spray-drying is widely used in the food, pharmaceutical and other industries because of its ability to efficiently transform a liquid feed into a dry powder (Huang, Reference Huang2011). Recent references show that the spray drying technique is a suitable long-term preservation method for liquid and semi-liquid food, and it is also used for the stabilization of matrices containing probiotic bacteria, namely yogurt and other dairy products (Barbosa et al., Reference Barbosa, Brandao and Teixeira2017; Reale et al., Reference Reale, di Renzo, Preziuso, Panfili, Cipriano and Messia2019).
The shelf-life of yogurt can be considerably changed by spray-drying resulting in a stable powder of high quality, without the need for refrigeration (Kumar and Mishra, Reference Kumar and Mishra2004, Guergoletto et al., Reference Guergoletto, Sivieri, Tsuruda, Martins, Bertuol de Souza, Roig, Hirooka, Garcia and Benjamin2012). This yogurt powder can also be used as an ingredient for the manufacturing of several food products such as confectioneries, yogurt drinks, fruit mixes, etc., as well as for direct consumption after reconstitution (Kumar and Mishra, Reference Kumar and Mishra2004). According to the Food and Agricultural Organization (FAO), the microorganisms in yogurt must be viable and in sufficient amount to promote health benefits (Guergoletto et al., Reference Guergoletto, Sivieri, Tsuruda, Martins, Bertuol de Souza, Roig, Hirooka, Garcia and Benjamin2012); this is usually greater than 6–7 log of colony forming units (cfu) of viable microorganisms per gram of product at the time of being consumed (Aquilina et al., Reference Aquilina, Bach, Bampidis, Bastos, Flachowsky, Gralak, Hogstrand, Leng, López-Puente, Martelli, Mayo, Renshaw, Riychen, Saarela, Sejrsen, van Beelen, Wallace and Westendorf2013).
Survival of microorganisms in dried matrices or products depends on many factors including species and strain, drying conditions, the use of cell protective agents and storage conditions (i.e., water activity (a w), temperature and time). The decay of viable cell count is the main drawback when dehydrating probiotic formulations, and is associated with the severity of processing and storage conditions (Succi et al., Reference Succi, Sorrentino, di Renzo, Tremonte, Reale, Tipaldi, Pannella, Russo and Coppola2014; Aureli et al., Reference Aureli, Fiore, Scalfaro, Casale and Franciosa2010). Cell protectants have been used to increase the survival of lactic acid bacteria after drying and storage. Among them, milk powder, whey, trehalose, sucrose, lactose and some oligo and polysaccharides can be mentioned (Meng et al., Reference Meng, Stanton, Fitzgerald and Roos2007; Schoug, Reference Schoug2009). Very recently, Stefanello et al. (Reference Stefanello, Machado, Cavalheiroa, Santos, Nabeshima, Copetti and Fries2018) reported on the role of trehalose as a bioprotectant in freeze-drying.
The dried state limits the number of techniques that can be applied to study conformation and stability of intracellular biomolecules (Wolkers and Oldenhof, Reference Wolkers and Oldenhof2005). One of the few suitable techniques for in situ analysis of biomolecules in the dried state is Fourier Transform Infrared Spectroscopy (FTIR). On account of characteristic molecular vibrations that absorb in the infrared region, information can be derived on molecular conformation and intermolecular interactions of biomolecules in their native environment (Wolkers and Oldenhof, Reference Wolkers and Oldenhof2005).
The objective of this work was to obtain basic information for the development of a powdered drinkable ‘yoghurt’. For this reason, we utilized as raw material a commercial drinkable formulation containing not only Lactobacillus bulgaricus and Streptococcus thermophilus, but also, Lactobacillus casei and Bifidobacterium spp. In studies of the stability of yogurt powder it is customary to determine the survival of lactic acid bacteria during storage (Kumar and Mishra, Reference Kumar and Mishra2004). In the present study a different approach was selected. Instead of enumerating lactic bacteria during storage, whole milk was fermented using an inoculum of spray-dried yogurt previously stored for different times and under different conditions. The measured acidification kinetics by the lactobacilli present in dried yogurt was used as an indicator of the injuries caused by the different conditions of a w, time and temperature during storage. A FTIR analysis of the stored powder samples was also performed to evaluate the spectral differences among samples, arising from the different storage conditions assayed. The influence of the addition of trehalose, a well-known cell protectant, was also studied.
Materials and methods
Drinkable yogurt
A commercial, drinkable yogurt without added sugar (‘SER’, Danone, Argentina) was used. The yogurt had the following composition: 8.15% (± 0.02) dried extract, 4.45% carbohydrates, 3.15% protein, 0% fat and pH 4.40 (± 0.02). The list of ingredients included: defatted milk, lactic ferments (Lactobacillus delbrueckii subsp. bulgaricus, Streptococcus thermophilus, Bifidobacterium spp., Lactobacillus casei), modified starch, stabilizers (gelatin, gellan gum), intensive sweeteners and artificial strawberry flavor.
Water activity, moisture content and pH
Water activity (a w) was measured using a dew point hygrometer ‘Aqualab’ Series 3 (Decagon Devices, USA) previously calibrated in the range of interest with standard saturated salt solutions. Moisture content was determined gravimetrically (2 g sample) in a forced convection constant temperature oven at 90 °C during 6 h. The pH of fresh yogurt and inoculated milk were measured with a pH meter (Hanna Instruments, Italy) previously calibrated with appropriate buffers. All measurements were carried out in triplicate.
Reagents
Salts for relative humidity control (reagent grade) were obtained from Biopack (Buenos Aires, Argentina). Food grade trehalose (dehydrate) was obtained from Hayashibara Co. Ltd. (Japan).
Spray-drying
The drinkable yogurt, as such or after the addition of 4% trehalose, was spray-dried in a mini spray-dryer Büchi model B-290 (Büchi Laboratoriums Technik, Switzerland). The main operating conditions were selected so that the air outlet temperature did not exceed 70 °C, being this an important condition to obtain an adequate survival of lactobacilli (Zhang et al., Reference Zhang, Lin and Zhong2016). A combination of an inlet air temperature of 140 °C and a feed (yogurt) flow rate of 500 g/h allowed to obtain an outlet temperature of 68–70 °C as well as a low a w (about 0.20). The spray-drying runs were performed in duplicate and the yield was around 50%.
Plate counting
Bacterial viability was determined before and after spray-drying and in the presence (4%) or absence (0%) of trehalose. For each determination, samples were rehydrated in 1 ml 0.85% w/w NaCl. Bacterial suspensions were serially diluted, plated on MRS agar (de Man et al., Reference de Man, Rogosa and Sharpe1960) and incubated at 37 °C for 48 h in aerobic conditions. Results are expressed in log cfu/ml and are the average of three independent determinations.
Storage conditions
Spray-dried yogurt powders (0% and 4% trehalose) were stored in the following conditions:(a) hermetically sealed flasks to preserve its initial a w. Some were kept in a constant temperature oven at 38 °C and others in a cyclical temperature chamber where the temperature was maintained at 10 °C for 12 h and then 12 h at 20 °C. This cyclical variation of temperature adequately represents the daily variation of temperature in Buenos Aires between the months of August through November. The temperature of 38 °C is often utilized for studies of accelerated storage of foods (Labuza TP and Saltmarch, Reference Labuza and Saltmarch1982).
Total storage time was 12 weeks; one sample from each condition was removed each week and kept in a freezer at −72 °C until the different analyses were carried out. Table 1 shows abbreviated codes used for stored samples of dried yogurt.
Table 1. Description of abbreviated codes used for stored samples of dried yogurt
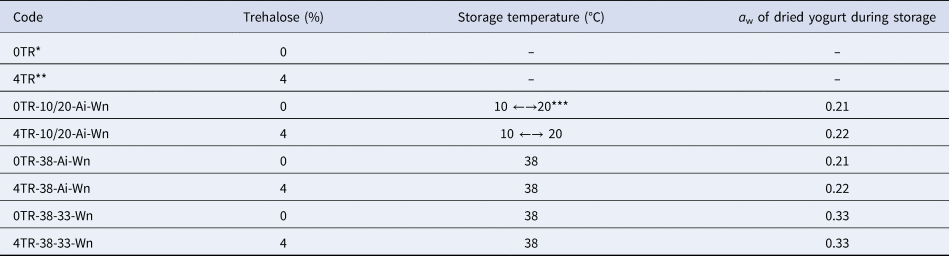
*0TR = 0% of trehalose addition.
**4TR = 4% of trehalose addition.
***Cyclic temperatures: 12 h cycles (20 °C and 10 °C) resembling the daily variation of temperature in a temperate climate region.
Ai = As is; in relation to the water activity of the powder.
Wn: weeks stored, n = 1 to 12.
Kinetics of milk fermentation using yogurt powder as inocula
Pasteurized whole milk was fermented by adding an inoculum of the spray-dried yogurt (previously stored at the different conditions described previously) and the kinetics of fermentation were evaluated by determining their rate of acidification (pH decrease). The following procedure was used: (1) sterilization of glass jars, (2) preheating of milk at 43 °C, (3) inoculation of 20 ml of milk in the jars with 1.0 g of yogurt powder without trehalose, or 1.45 g of yogurt powder with 4% trehalose, and (4) glass jars were kept in a constant temperature bath at 43 °C and pH was measured every 30 min during a 6 h period; pH measurements were made in triplicate. An excess of inoculum (1.45 g instead or 1.0 g) was used in the yogurt powder runs with trehalose to compensate for the dilution of yogurt caused by the addition of trehalose.
Fourier transform infrared spectra (FTIR)
About 5 mg of dried yogurt powder stored under the different conditions were used to register the FTIR spectra. Samples were placed on the sample holder of an ATR-FTIR Thermo Nicolet iS10 spectrometer (Thermo Scientific, MA, USA). Spectra were registered in the 4000–500 cm−1 range by co-adding 100 scans with 4 cm−1 spectral resolution, using OMNIC software (version 8.3, Thermo Scientific, MA, USA). At least three spectra were recorded.
Data analysis
Multivariate analysis and data pre-processing as mean centering and extended multiplicative scatter correction were performed on the FTIR spectra, using The Unscrambler software (version 10.2, CAMO, Norway). To evaluate the spectral differences among samples, arising from the different storage conditions assayed, a principal component analysis (PCA) was performed on the FTIR spectra. Taking into account the spectral differences in the PCA scores plot, PLS models were calibrated to determine the lag time (Esbensen, Reference Esbensen2005). FTIR spectra covering the whole range of values were used to define the models. To set-up the PLS models, carried out on the raw spectra, 54 spectra were registered for calibration. Leave-one-out-cross validation method (LOOCV) was used for validation, and 7 PLS-factors were used for prediction. Lag times were used as reference values. The reliability and robustness of the calibrated models were determined as a function of their correlation values, R-square, BIAS and their calibration errors (RMSEC).
Results and discussion
Moisture content, a w and microbiological analysis of spray-dried yogurt
Fresh drinkable yogurt with (4%) and without added trehalose was spray-dried using the operating conditions described previously. The outlet air temperature not only influences the survival of lactic bacteria but also the a w of the powder, so a compromise value was taken since a decreased outlet temperature improves survival but also increases final a w. The yogurt powder spray-dried with 140 °C air inlet temperature and 68/70 °C outlet air temperature had the following a w and moisture content (dry basis): 0.206 ± 0.005 and 5.32 ± 0.15, respectively for yogurt without trehalose, and 0.225 ± 0.001 and 5.16 ± 0.15, respectively for yogurt with 4% trehalose. Kumar and Mishra (Reference Kumar and Mishra2004) suggested that for a better survival of Streptococcus, Lactobacillus and Bifidobacterium spp. during storage the a w of yogurt powder should be about 0.20, which is in good agreement with the present values. Microbiological analysis indicated that fresh yogurt had a total bacterial count of 8.51 log cfu/ml, plain dried yogurt had 8.48 log cfu/g and dried yogurt added with trehalose (4%) had 8.89 log cfu/g. Reconstituted (to their initial solids contents) spray-dried yogurts were also analyzed and total bacterial counts were 7.81 log cfu/g for plain yogurt (0TR) and 7.96 log cfu/g for trehalose-supplemented yogurt (4TR). These values indicate satisfactory survival of yogurt cultures (Bielecka and Majkowska, Reference Bielecka and Majkowska2000; Zhang et al., Reference Zhang, Lin and Zhong2016) and are very similar to those reported by Koc et al. (Reference Koç, Yilmazer, Balkır and Ertekin2010) for spray-dried yogurt.
Kinetics of milk fermentation using inoculum of yogurt powder
Whole milk was fermented using inocula of spray-dried yogurt previously stored for different times and conditions. Figure 1 shows the measured kinetics of acidification using yogurt powders inocula; for the purposes of clarity only eight curves were displayed. The standard deviation for pH values ranged between 0.04 and 0.06. The eight conditions plotted in Fig. 1 adequately illustrate the general shape of pH curves obtained after mild or drastic storage conditions. However, as will be shown later, a much larger number of conditions (several combinations of a w, temperature, presence of trehalose and time) will be utilized for further calculations. The measured kinetics of fermentation was used as an indication of the metabolic activity. Golowczyc et al. (Reference Golowczyc, Silva, Abraham, De Antoni and Teixeira2010) also measured the acidification kinetics in milk by fresh and dried lactobacilli to evaluate the injuries caused by spray-drying on lactobacilli isolated from kefir grains.

Fig. 1. Kinetics of milk fermentation using yogurt powder inocula for some of the storage conditions for samples with (black reference) and without (gray reference) trehalose. Data shown are the average of three independent determinations. Error bars for pH are not observed because they overlap with data symbols.
In all acidification curves, a period during which the pH remains approximately constant (lag time) was observed; in most of them this was followed by a rapid fall in the pH values. The lag time is associated with the time for repair of sub-lethal injuries experienced by lactobacilli stored under various stress conditions. In cases of extreme storage conditions (38 °C and a w = 0.33), the lag time was very long and even 400 min was not enough for obtaining the final pH of around 4.5. In order to better understand the relationship between storage condition, time and addition of trehalose, all acidification curves were fitted using a logistic type equation (Eq. 1) (Romano et al., Reference Romano, Schebor, Mobili and Gómez-Zavaglia2016).

where,
pH(t): is the pH at different fermentations times (every 30 min)
pH0: is pH at the beginning of milk fermentation
t: time (minutes)
The obtained variables were,
c: time at which the inflection point occurs in the fitted function
p: exponential adjustment factor
pHf: pH at the end of the fermentation
The lag time was calculated as the intersection between the tangent line at t = c and pH0. Figure 2 shows the effect of storage time of yogurt powder (inocula) on the lag time during milk acidification.

Fig. 2. Effect of storage time of yogurt powder on the lag time during milk acidification. Data shown are the average of three independent determinations.
It can be seen that the lag time for yogurt powder of both samples stored at the most gentle condition, which was 10/20 °C and a w = 0.22, remains approximately constant over the 12 weeks of storage (samples codes 0TR-10/20-Ai-Wn and 4TR-10/20-Ai-Wn, Table 1). For these samples, trehalose showed a modest decrease in the lag period, suggesting a small effect as cell protector; this was only perceived under this gentle storage condition. At all other conditions of a w and temperature, the lag time increased rapidly with storage time up to a point (8 weeks) at which all samples either leveled off or tended to vary in a more or less erratic way (data not shown). It is clear that both the a w and the temperature of storage are key factors determining bacterial viability during storage; even small variations in a w (0.21/0.22→0.33) and temperature (10/20→38 °C) were highly detrimental of cell functioning.
FTIR
FTIR spectroscopy was used to monitor the changes in lag period experienced by spray-dried yogurt stored under different conditions. Figure 3 depicts three representative spectra corresponding to 0TR-10/20-Ai-0 (blue spectrum), 0TR-38-33-12 (green spectrum) and 4-38-33-6 (red spectrum). The main spectral differences were observed in the 1200–900 cm−1 region, a complex region rich in bands attributed essentially to the C–O–C glycosidic linkage, the δCOH and the νC–C vibrational modes (Santos et al., Reference Santos, Araujo-Andrade, Tymczyszyn and Gomez-Zavaglia2014a, Reference Santos, Araujo-Andrade, Esparza-Ibarra, Tymczyszyn and Gómez-Zavaglia2014b). That is why this region is known as fingerprint region for sugars, as bands in this region collectively provide a complex pattern that univocally characterizes pure sugars. All the analyzed dehydrated yogurts contained different sugars, which explains the typical pattern in such region. Moreover, it is worth to mention that the presence of trehalose in some of the samples introduces an additional complexity to spectra, arising from the fact that trehalose also strongly absorbs in that region, thus masking slighter differences arising from the bacterial kinetic parameters.

Fig. 3. FTIR spectra corresponding to 0TR-10/20-Ai-0 (blue, top), 0TR-38-33-12 (green, middle) and 4-38-33-6 (red, bottom) in the 1800–700 cm−1 region.
In order to discriminate spectra according to the physical conditions used for storage, and to avoid the interference of trehalose, strongly absorbing in the infrared region of the electromagnetic spectrum, two different PCA was carried out on spray-dried yogurt without (Fig. 4a) and with the protective compound trehalose (Fig. 4b). Samples of yogurt powders spray-dried without trehalose, were mainly distributed along PC2, which explained 25% of the variance. In turn, yogurt powders containing trehalose were mainly grouped along PC1, which explained 58% of the variance. However, regardless of the presence of trehalose, sample grouping was associated to differences in the lag times. FTIR spectroscopy was used by Kher et al. (Reference Kher, Udabage, Mc Kinnon, Mc Naughton and Agustin2007) to examine the conformation of proteins in spray-dried milk protein concentrate prepared from a range of processing conditions and after storing for 4 weeks at 21 °C. They reported that FTIR spectroscopy could be used to predict protein behavior since the obtained data correlated well with changes in solubility of the dry powders on storage.

Fig. 4. PCA performed on the spray-dried yogurt samples, stored at different a w and temperatures. (a) without trehalose; (b) with trehalose. Squares: 0TR-10/20-Ai or 4TR-10/20-Ai; circles: 0TR-38-33 or 4TR-38-33; triangles: 0TR-38-Ai or 4TR-38-Ai. Full black symbols (squares, circles and triangles): samples stored for one week; full gray symbols: samples stored for 6 weeks; empty symbols: samples stored for 12 weeks.
According to the loading plots in PC1 (Fig. 5), the main differences among samples were observed in the 1200–900 cm−1 region, associated to the C–O–C glycosidic linkage, the δCOH and the νC–C vibrational modes (Santos et al., Reference Santos, Araujo-Andrade, Tymczyszyn and Gomez-Zavaglia2014a). For example, 0TR-38-33 samples stored for 12 weeks showed the largest lag times and were observed at the highest PC1 values (Fig. 4a, open circles). On the contrary, sample 0TR-10/20-Ai stored for shorter times (squares-open, gray or black in Fig. 4a) were observed in the other extreme of the plot. Samples with lag times within these two extremes were observed at intermediate positions. A similar behavior was observed along PC1 for yogurt powders containing trehalose (Fig. 4b).

Fig. 5. 1-D Loading plots in PC1 and PC2, performed on the FTIR spectra of powder yogurts without (a) and with (b) trehalose.
A PLS model was defined to determine the lag time directly from the FTIR spectra. In the definition of this model, results obtained in section ‘Kinetics of milk fermentation using inoculum of yogurt powder’ were used as references. The PLS model was calibrated using 54 spectra (correlation of 0.989; R 2, 0.977; Root Mean Square Error of Calibration (RMSEC), 15.95; Standard Error of Calibration (SEC), 16.101; BIAS, −1.907 × 10−6), and cross-validated (Fig. 6). Using spectra covering a broad range of lag times strengthened the predictive capacity of the model. The mean of the predicted values fitted nicely to those shown in Fig. 2 and was not dependent on the presence (or absence) of trehalose in the samples. This broadens the application of the obtained model, supporting its use to investigate unknown samples. Considering that the lag time is closely related to the functionality of yogurt powders, using the defined PLS model would allow determination of this parameter just by registering FTIR spectra.

Fig. 6. Predicted vs. reference values for lag time.
In conclusion, whole milk was fermented using inocula of spray-dried yogurt (with and without added trehalose) previously stored for up to 12 weeks at different a w and temperature. The lag time in the kinetics of milk acidification was associated with the impact of the storage condition and the presence of trehalose. Lag times for yogurt powder of samples stored at the most gentle condition, which was 10/20 °C and a w = 0.22, remained approximately constant over the 12 weeks of storage. For these samples, trehalose showed only a modest decrease in the lag time, suggesting a small effect as cell protector which was only perceived under this gentle storage condition. At the other conditions of a w and temperature, the lag time increased rapidly with storage time and the presence of trehalose could not counterbalance the negative effect of temperature and a w. Both, a w and temperature of storage were key factors determining sublethal injuries during storage; even small variations in a w (0.21/0.22→0.33) and temperature (10/20 °C→38 °C) were highly detrimental of cell functioning. Partial Least Squares (PLS) models enabled a fairly good correlation between lag time of fermentation and FTIR spectra which might allow prediction of lag time just by registering FTIR spectra.
Acknowledgements
The authors acknowledge financial support from Facultad de Ingenieria y Ciencias Agrarias, Pontificia Universidad Catolica Argentina. This work was also supported by the Argentinean Agency for the Scientific and Technological Promotion (ANPCyT) (Projects PICT/2014/0912; PICT start up 2016/4808; PICT/2016/3047; PICT2017/1344).