Food proteins provide essential amino acids and are also an energy source. The technological processing applied to milk such as pasteurisation, sterilisation, condensation, fermentation and cheese making are to a large extent determined by the properties of the milk proteins. Camel milk is endowed with a unique composition of protein components (El-Agamy, Reference El-Agamy2007; Hinz et al. Reference Hinz, O'Connor, Huppertz, Ross and Kelly2012). It has been proposed that functional food containing angiotensin converting enzyme (ACE) inhibitors and antioxidant peptides could be formulated using fermented camel milk (Moslehishad et al. Reference Moslehishad, Ehsani, Salami, Mirdamadi, Ezzatpanah, Naslaji and Moosavi-Movahedi2013) and camel milk is also reported to have protective proteins with immunologic, bactericidal and viricidal properties (El-Hatmi et al. Reference El-Hatmi, Girardet, Gaillard, Yahyaoui and Attia2007).
The production of camel cheese has until recently been difficult and camel whey has therefore not been available in commercial quantities. Recent innovations in separation and enzyme technologies, however, allow for the production of purified milk components directly from camel milk. We expect production of camel cheese to increase in the near future and thus make whey available as a source of specific camel proteins. Camel milk proteins as bioactive components of functional foods have the potential to open new market opportunities as it is well established that different functional properties can be ensured by incorporating milk proteins into various foods (Foegeding et al. Reference Foegeding, Davis, Doucet and McGuffey2002; Laleye et al. Reference Laleye, Jobe and Wasesa2008; Moslehishad et al. Reference Moslehishad, Ehsani, Salami, Mirdamadi, Ezzatpanah, Naslaji and Moosavi-Movahedi2013).
The functional properties of proteins are dependent on the amino acid sequence of the proteins as well as on their formulation and processing (Panyam & Kilara, Reference Panyam and Kilara1996; Foegeding et al. Reference Foegeding, Davis, Doucet and McGuffey2002; Laleye et al. Reference Laleye, Jobe and Wasesa2008). Some investigations on camel milk proteins regarding denaturation of whey proteins, formation of protein films, emulsions, foams, and milk gels have been conducted under specific conditions (Panyam & Kilara, Reference Panyam and Kilara1996). The absence of β-LG might explain some of the differences observed between camel and cow milk regarding technological properties such as thermal stability during drying, heat induced aggregation and adherence to heating surfaces (fouling properties) as well as the thin consistency found in fermented camel milk (Merin et al. Reference Merin, Bernstein, Bloch-Damti, Yagil, van Creveld, Lindner and Gollop2001; El-Agamy, Reference El-Agamy2007; El-Hatmi et al. Reference El-Hatmi, Girardet, Gaillard, Yahyaoui and Attia2007; Laleye et al. Reference Laleye, Jobe and Wasesa2008).
A comprehensive proteome of camel milk and of proteins secreted in the lactating mammary gland of camels is not yet available. Major and several minor constituents have, however, been characterised. These are the caseins, i.e. αs1-casein, αs2-casein, β-casein, and κ-casein, several whey proteins, i.e. α-lactalbumin (α-LA), lacto(trans)ferrin (LF), whey acidic protein (WAP), glycosylation-dependent cell adhesion molecule 1 (GlyCAM-1, lactophorin, PP3), peptidoglycan recognition protein short variant (PGRP-S), lactoperoxidase (LP), and immunoglobulins (Kappeler, Reference Kappeler1998), and some milk fat globule membrane proteins; fatty acid synthase, xanthine dehydrogenase/oxidase, butyrophilin, lactadherin, milk fat globule-EGF factor 8, and adipophilin (Saadaoui et al. Reference Saadaoui, Henry, Khorchani, Mars, Martin and Cebo2013). Furthermore, annotated genome sequences have been published for camels, and can be utilised for proteomic analysis (Wu et al. Reference Wu, Guang, Al-Fageeh, Cao, Pan, Zhou, Zhang, Abutarboush, Xing, Xie, Alshanqeeti, Zhang, Yao, Al-Shomrani, Zhang, Li, Manee, Yang, Yang, Liu, Zhang, Altammami, Wang, Yu, Zhang, Liu, Ba, Liu, Yang, Meng, Wang, Li, Li, Li, Wu, Zhang, Wang, Yin, Yang, Al-Swailem and Wang2014).
Better knowledge about the properties of the protein fractions and the effects of technological manipulations on the protein properties is important in developing new products from milk. Technology and functionality of cow milk proteins have been thoroughly investigated but there is limited information on camel milk proteins. Hence, this review emphasises composition and functional properties of camel milk proteins, potential health benefits and the technological properties in comparison to bovine milk.
Protein composition of camel milk
The chemical composition of camel milk is 3·8 ± 1·1% fat, 3·4 ± 0·6% total protein, 4·5 ± 1·0% lactose, 12·5 ± 1·5% dry matter, and 0·8 ± 0·1% ash (Konuspayeva et al. Reference Konuspayeva, Faye and Loiseau2009). Table 1 gives the composition of camel milk in comparison to milk from humans and ruminants.
Table 1. Chemical composition of milk from different mammals
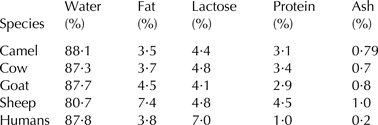
Sources: Fox & McSweeney (Reference Fox and Mcsweeney1998); Al Haj & Al Kanhal (Reference Al haj and Al Kanhal2010).
The protein fraction may be divided into three broad classes, namely caseins, whey proteins, and milk fat globule membrane proteins (MFGMP).
Quantification of the major caseins is presented in Table 2. There is a similar relative abundance of β-casein in camel milk as in human milk.
Table 2. Composition of casein in camel, bovine and human milk

† Corresponding coding sequence absent in genome.
Sources: aKappeler et al. (Reference Kappeler, Farah and Puhan1998); bEl-Agamy (Reference El-Agamy, Park and Haenlein2006); cMalacarne et al. (Reference Malacarne, Martuzzi, Summer and Mariani2002).
Major whey proteins are shown in Table 3. β-lactoglobulin (β-LG), α-lactalbumin (α-LA), serum albumin (SA), LF, and different immunoglobulins (Ig) are the main components of whey proteins in milk of mammals. Their relative abundance and presence varies from one species to the other (El-Agamy, Reference El-Agamy2000; Merin et al. Reference Merin, Bernstein, Bloch-Damti, Yagil, van Creveld, Lindner and Gollop2001; Kappeler et al. Reference Kappeler, Heuberger, Farah and Puhan2004; El-Hatmi et al. Reference El-Hatmi, Girardet, Gaillard, Yahyaoui and Attia2007). β-LG and α-LA are major whey proteins (70–80%) in cow's milk (Chatterton et al. Reference Chatterton, Smithers, Roupas and Brodkorb2006; Hinz et al. Reference Hinz, O'Connor, Huppertz, Ross and Kelly2012). In camel milk, β-LG is absent and a high amount of SA has been reported in addition to α-LA (Table 3). Major constituents are furthermore IgG and GlyCAM-1, whereas WAP, peptidoglycan recognition protein (PGRP-S) and LF are present in minor amounts (El-Agamy, Reference El-Agamy2000; Merin et al. Reference Merin, Bernstein, Bloch-Damti, Yagil, van Creveld, Lindner and Gollop2001; Kappeler et al. Reference Kappeler, Heuberger, Farah and Puhan2004; El-Hatmi et al. Reference El-Hatmi, Girardet, Gaillard, Yahyaoui and Attia2007). Colostral whey is abundant in Ig and in addition camel colostral whey contains a high amount of SA (ca. 12·5 g/l on day 1, ca. 9·2 g/l on day 8), whereas bovine colostral whey is rich in β-LG (Merin et al. Reference Merin, Bernstein, Bloch-Damti, Yagil, van Creveld, Lindner and Gollop2001; El-Hatmi et al. Reference El-Hatmi, Levieux and Levieux2006). Camel colostrum furthermore contains elevated amounts of α-LA and LF (Merin et al. Reference Merin, Bernstein, Bloch-Damti, Yagil, van Creveld, Lindner and Gollop2001; El-Hatmi et al. Reference El-Hatmi, Levieux and Levieux2006).
Table 3. Composition of whey protein in camel, bovine and human milk

† Corresponding coding sequence absent in genome.
‡ Strong correlation with daily milk yield, somatic cell count, and stage of lactation.
Mid-lactation values of pooled samples from mastitis-free milk were taken, where available. Figures are indicative, since literature values strongly vary, and have been rounded, as appropriate.
Sources: aMalacarne et al. (Reference Malacarne, Martuzzi, Summer and Mariani2002); bChatterton et al. (Reference Chatterton, Smithers, Roupas and Brodkorb2006); cEl-Hatmi et al. (Reference El-Hatmi, Levieux and Levieux2006); dShamsia (Reference Shamsia2009); eFox (Reference Fox and Smit2003); fKappeler (Reference Kappeler1998); gHettinga et al. (Reference Hettinga, van Valenberg de Vries, Boeren, van Hooijdonk, van Arendonk and Vervoort2011); hKappeler et al. (Reference Kappeler, Heuberger, Farah and Puhan2004); iBoehmer et al. (Reference Boehmer, Ward, Peters, Shefcheck, McFarland and Bannerman2010); jLiu et al. (Reference Liu, Xu, Gupta and Dziarski2001); kde Wit (Reference De wit1998); klEl-Agamy et al. (Reference El-Agamy, Ruppanner, Ismail, Champagne and Assaf1996); mFarah (Reference Farah1996).
Molecular properties of camel milk proteins
αS1-casein of camel milk has two isoforms A and B with 207 and 215 amino acid residues and respective molecular weights of 24·668 and 24·755 kDa for variant A, containing five or six phosphorylated serines, and of 25·707 for variant B containing five phosphorylated serines (Kappeler et al. Reference Kappeler, Farah and Puhan1998). Other apparent molecular weight (MW) values have been reported for αS1-casein 33 kDa (El-Agamy, Reference El-Agamy, Park and Haenlein2006), and 27·6 kDa (Salmen et al. Reference Salmen, Abu-Tarboush, Al-Saleh and Metwalli2012). The isoelectric point is about 4·40 (Kappeler et al. Reference Kappeler, Farah and Puhan1998). Camel milk αS2-casein has 178 amino acids and a MW of 21·993 kDa with an isoelectric point of 4·58 for αS2-casein-9P (Kappeler et al. Reference Kappeler, Farah and Puhan1998). El-Agamy (Reference El-Agamy, Park and Haenlein2006) however found a higher apparent MW of 25 kDa. The relative quantity of some essential amino acids (i.e. Met, Ile, Leu, and Phe) and all non-essential amino acids except Arg is higher in bovine α-casein compared to camel α-casein (Salmen et al. Reference Salmen, Abu-Tarboush, Al-Saleh and Metwalli2012).
β-casein of camel milk has 217 amino acid residues and a MW of 24·900 kDa (Kappeler et al. Reference Kappeler, Farah and Puhan1998). However, in the report of Salmen et al. (Reference Salmen, Abu-Tarboush, Al-Saleh and Metwalli2012) the estimated MW is 23·8 kDa. The isoelectric point of camel milk β-casein-4P is 4·66 (Kappeler et al. Reference Kappeler, Farah and Puhan1998). It contains a lower relative amount of Val, Phe, His, Gly and Ser (Salmen et al. Reference Salmen, Abu-Tarboush, Al-Saleh and Metwalli2012).
Camel milk κ-casein has 162 amino acid residues and a MW of 22·294–22·987 kDa, as reported by Kappeler et al. (Reference Kappeler, Farah and Puhan1998), while Salmen et al. (Reference Salmen, Abu-Tarboush, Al-Saleh and Metwalli2012) reported 22·4 kDa. The protein contains five sialylated glycosylation sites at threonine positions 105, 109, 149, 152 and 153, and the isoelectric point of κ-casein-1P is 4·11 (Kappeler et al. Reference Kappeler, Farah and Puhan1998). The chymosin cleavage site is Phe97 – Ile98. The enzyme cuts off a C-terminal glyco-macropeptide of 65 amino acids and a MW of 6·774 kDa (Kappeler et al. Reference Kappeler, Farah and Puhan1998). Wangoh et al. (Reference Wangoh, Farah and Puhan1998) stated that camel milk casein cannot be separated from whey protein by precipitation at pH 4·6, as a result of isoelectric point difference of the individual caseins.
The camel milk casein micelles have a similar spherical shape as ruminant casein micelles. The diameter is however larger, i.e. 380, 150, 260 and 180 nm for camel, bovine, caprine and ovine milk, respectively (Bornaz et al. Reference Bornaz, Sahli, Attalah and Attia2009). According to Kherouatou et al. (Reference Kherouatou, Nasri and Attia2003), the size distribution of the casein micelles in solution is in the range of 0·4–0·5 µm, while that in cow's milk is in the range of 0·13–0·16 µm. The average diameter is inversely related to the κ-casein and calcium phosphate concentrations (Brulé et al. Reference Brulé, Lenoir, Remeuf, Eck, Gillis, Eck and JC2000; Bornaz et al. Reference Bornaz, Sahli, Attalah and Attia2009) which both affect milk gel formation. Increased surface area (small casein micelles) result in formation of a firmer curd (Gutiérrez-Adán et al. Reference Gutiérrez-Adán, Maga, Meade, Shoemaker, Medrano, Anderson and Murray1996) and camel milk casein micelles with their larger diameters will, therefore, have a poorer aggregation and gel formation behaviour compared to bovine casein micelles, resulting in a weaker coagulum (Ramet Reference Ramet2001; Bornaz et al. Reference Bornaz, Sahli, Attalah and Attia2009).
Camel α-LA, as bovine α-LA, has a length of 123 amino acids, but with 39 positional differences. The MW is 14·4 kDa and the isoelectric point at pH 5·01. The protein seems not to be modified after translation. Differential cleavage of the signal peptide by three amino acids may result in an N-terminally shortened variant that is less abundant, has an MW of 14·0 kDa, and an isoelectric point at pH 4·87 (Beg et al. Reference Beg, von Bahr-Lindström, Zaidi and Jörnvall1985).
Camel LF has 689 amino acids residues, a MW of 75·3 kDa and an isoelectric point at pH 8·63 (Kappeler et al. Reference Kappeler, Ackermann, Farah and Puhan1999). Camel LF is the first protein from the transferrin superfamily that has been found to display the two characteristic functions of iron scavenging (lactoferrin) and iron release (transferrin) simultaneously. This is because the N-lobe releases iron at a pH below 4·0 and the C-lobe releases iron at about pH 6·5 (Khan et al. Reference Khan, Kumar, Paramasivam, Yadav, Sahani, Sharma, Srinivasan and Singh2001). Whereas the N-lobe exerts mainly an antimicrobial activity, the C-lobe contains five highly variable sequences that differ between species and have a demonstrated affinity for hepatitis C virus in the case of the camel variant (Sharma et al. Reference Sharma, Sinha, Kaushik, Kaur and Singh2013c). The heat stability of the camel orthologue is similar to that of other species and the antimicrobial activity against the Gram-negative bacterium E. coli is higher than for other orthologues, including the human LF (Conesa et al. Reference Conesa, Sánchez, Rota, Pérez, Calvo, Farnaud and Evans2008).
The PGRP-S is a protective, antibiotic protein that is secreted from white blood cells and has the ability to depolarise bacterial membranes. It is bactericidal against both Gram-positive and Gram-negative bacteria, by inducing oxidative, thiol, and metal stress (Kashyap et al. Reference Kashyap, Rompca, Gaballa, Helmann, Chan, Chang, Hozo, Gupta and Dziarski2014). PGRP-S has been found in non-mastitic camel milk (Table 3), but not in non-mastitic human or ruminant milk. The protein has 172 amino acids, calculated and measured MWs of 19·1 kDa, excluding the possibility of posttranslational modification or binding of cation ligands, and an isoelectric point at pH 9·02. Its concentration decreased in the course of lactation by 19% and increased in the event of severe mastitis by 45% (Kappeler et al. Reference Kappeler, Heuberger, Farah and Puhan2004). The structure of camel PGRP-S has been elucidated as a homotetramer from two dimers of opposite orientation. This property allows camel PGRP-S to form contiguous chains that can bind lipopolysaccharide, lipoteichoic acid, and peptidoglycan in either of the grooves, a peculiarity the human orthologue does not have (Sharma et al. Reference Sharma, Dube, Sinha, Yadav, Kaur, Sharma and Singh2013a).
The lactoperoxidase (LP) mode of action is highly conserved between species and a high sequence identity of about 85% is reported between the respective human, camel and ruminant variants (Sharma et al. Reference Sharma, Singh, Kaushik, Sinha, Singh, Sharma, Sirohi, Kaur and Singh2013b). Camel LP has 611 amino acids after removal of signal and propeptide; the unmodified chain has a MW of 69·3 kDa and an isoelectric point at pH 8·50 (Kappeler, Reference Kappeler1998). It exerts a reportedly high activity in raw milk. The enzyme is even more heat labile than the ruminant variants and activity is below limit of detection after pasteurisation (Lorenzen et al. Reference Lorenzen, Wernery, Johnson, Jose and Wernery2011; Tayefi-Nasrabadi et al. Reference Tayefi-Nasrabadi, Hoseinpour-fayzi and Mohasseli2011). It may therefore be used for conservation of raw camel milk in the field in combination with thiocyanate and hydrogen peroxide, and as an indicator for pasteurisation (Kamau et al. Reference Kamau, Lamuka and Wangoh2010).
Lysozyme C has been measured in scarce amounts in both camel and bovine milk, and is reported to have a similar MW of 14·4 KDa (El-Agamy et al. Reference El-Agamy, Ruppanner, Ismail, Champagne and Assaf1996; Poltronieri et al. Reference Poltronieri, Cappello and D'Urso2012). The enzyme is not, however, expressed in the healthy lactating mammary gland and was not found in mastitis-free milk (Kappeler et al. Reference Kappeler, Heuberger, Farah and Puhan2004). The traces reported in camel milk are probably transferred from the blood serum.
The MWs of camel and bovine SA are 69·6, and 66 kDa, respectively (El-Agamy et al. Reference El-Agamy, Ruppanner, Ismail, Champagne and Assaf1996). A considerably higher concentration of SA has been reported for camel milk, as compared to bovine and human milk (Table 3).
Two whey protein components i.e. GlyCAM-1 and PGRP-S, are found in a higher concentration in camel milk than in bovine milk (El-Hatmi et al. Reference El-Hatmi, Girardet, Gaillard, Yahyaoui and Attia2007). Camel whey further contains IgG2 and IgG3, two immunoglobulin variants of an additional type not present in bovine milk (El-Agamy et al. Reference El-Agamy, Ruppanner, Ismail, Champagne and Assaf1996; El-Hatmi et al. Reference El-Hatmi, Girardet, Gaillard, Yahyaoui and Attia2007; Poltronieri et al. Reference Poltronieri, Cappello and D'Urso2012; Abbas et al. Reference Abbas, Ashraf, Nazir and Sarfraz2013). These variants may provide camel milk with additional functionalities.
Technological significance of the protein composition of camel milk
Thermal treatment of camel milk
The molecular properties of whey proteins can be influenced by heating temperature, pH and salt content (NaCl) (Boye et al. Reference Boye, Alli, Ismail, Gibbs and Konishi1995). LF and IgG of camel milk are more heat resistant than the homologous proteins of bovine milk (El-Agamy, Reference El-Agamy2000). The thermal denaturation of camel milk whey proteins depends on the physical state of the proteins. Camel and cow milk whey proteins in liquid form have a similar thermal resistance, whereas upon drying the thermal stability of whey protein from camel milk has been reported to decrease as a result of absence of β-LG in camel milk (Merin et al. Reference Merin, Bernstein, Bloch-Damti, Yagil, van Creveld, Lindner and Gollop2001; Laleye et al. Reference Laleye, Jobe and Wasesa2008). Therefore, when manufacturing milk powder from camel milk, modified thermal treatment and atomisation conditions should be applied. For manufacture of acidified milk products it has been shown using scanning electron microscopy that thermal treatment at 85 °C\30 min for production of labneh from camel milk resulted in acidified milk protein gels consisting of smaller particles compared to more extensive heat treatment (90 °C\30 min) which caused casein particles to fuse and form larger aggregates (Desouky et al. Reference Desouky, Shalaby and Soryal2013). Unheated milk exhibited a more open, loose and less dense protein matrix. The higher abundance of α-LA in camel milk whey resulted in greater sensitivity of camel whey solubility upon pH change (Laleye et al. Reference Laleye, Jobe and Wasesa2008) and in bovine milk this is known to cause acid denaturation (Paulsson et al. Reference Paulsson, Hegg and Castberg1985). Bovine α-LA will form aggregates in acidic medium unlike β-LG; which will form aggregates upon heating in both alkaline and acidic mediums (Boye et al. Reference Boye, Alli, Ismail, Gibbs and Konishi1995). Camel SA is less heat sensitive than SA from bovine or buffalo milk and denaturation of camel SA at 100 °C for 20 min has been shown to be comparable to bovine and buffalo SA heated at 85 °C for 20 min (El-Agamy, Reference El-Agamy2000). However, the fouling properties (adherence of milk proteins to heated surfaces) of camel milk has been attributed mainly to α-LA and SA whereas β-LG is the main foulant in cow milk (Felfoul et al. Reference Felfoul, Lopez, Gaucheron, Attia and Ayadi2015).
Functional properties of whey protein products are affected by the technological processing (i.e. heat treatment) implemented during the manufacturing process. The effect of temperature on solubility of camel and bovine milk whey proteins is different. However, it is reported that the effect is pH dependant and bovine whey protein is more soluble than camel whey protein (Laleye et al. Reference Laleye, Jobe and Wasesa2008). Poorer stability of camel milk at higher temperatures (120–140 °C) compared to bovine milk has been reported to possibly be due to lack of β-LG and presence of smaller amounts of κ-casein (Farah & Atkins, Reference Farah and Atkins1992). Heat treatment at 65 °C/30 min of camel, cow and buffalo milk does not affect lysozyme and LF contents but the protective proteins for microbial factors such as Ig lose their activity (El-Agamy, Reference El-Agamy2000). In bovine milk β-LG ensures highly viscoelastic absorbed surface layers due to high packing density and strong intermolecular interactions whereas adsorbed casein layers have a looser, more mobile structure (Dickinson, Reference Dickinson1999). Therefore, the effect of β-LG absence in camel milk on the quality of milk powder and other dairy products needs to be studied. Thermal treatment of camel milk in the range of 63–90 °C for 30 min and also 72 °C for 15 s resulted in reduced rennetability of camel milk (Hattem et al. Reference Hattem, Naeim, Sakr and Abouel-Einin2011). A shift in gelation time occurs as a result of temperature change from 30 to 40 °C (Hailu et al. Reference Hailu, Hansen, Seifu, Eshetu and Ipsen2016). A comparable change is observed in rennet induced gelation of bovine milk due to change in pH (Lopez et al. Reference Lopez, Lomholt and Qvist1998; Mellema et al. Reference Mellema, Walstra, Van Opheusden and Van Vliet2002). This could be due to the higher amount of hydrophobic casein, i.e. β-casein, in camel milk than bovine milk (Kappeler et al. Reference Kappeler, Farah and Puhan1998; El-Agamy, Reference El-Agamy, Park and Haenlein2006). A synergetic effect of CaCl2 addition up to 20 mg/100 ml of camel milk and thermal treatment of camel milk, in reducing rennet clotting time using bovine chymosin was indicated by Hattem et al. (Reference Hattem, Naeim, Sakr and Abouel-Einin2011). Hailu et al. (Reference Hailu, Hansen, Seifu, Eshetu and Ipsen2016), however, reported that the effect of CaCl2 on camel milk gelation time using camel chymosin is pH dependent and the effect was observed at pH 6·3. LF in camel milk is stable at low pH and heat treatment, making it a good preservative for maintaining food products (Abbas et al. Reference Abbas, Ashraf, Nazir and Sarfraz2013).
Hydrolysis of camel milk proteins
Enzymatic hydrolysis of milk proteins enhances the functionality through the release of peptides with lower MW, different hydrophobicity and solubility properties and an increased number of ionisable groups (Panyam & Kilara, Reference Panyam and Kilara1996). High hydrolysis of proteins by endo-peptidase enzymes result in less amount of free amino acids for better bioavailability (Foegeding et al. Reference Foegeding, Davis, Doucet and McGuffey2002). The extent of hydrolysis of enzymes determines the digestibility of the proteins via the availability of free amino acids in the product. The camel caseins contain a higher number of potential pepsin cleavage sites in their primary structure (Saliha et al. Reference Saliha, Dalila, Chahra, Saliha and Abderrahmane2013). Camel milk β-casein was highly degraded by pepsin but resistant to chymotrypsin, trypsin and papain (Saliha et al. Reference Saliha, Dalila, Chahra, Saliha and Abderrahmane2013), therefore, pepsin can be used for modification of camel milk protein properties. Camel αS1-casein was hydrolysed more efficiently by trypsin, chymotrypsin or papain in comparison to β-casein, (Saliha et al. Reference Saliha, Dalila, Chahra, Saliha and Abderrahmane2013). The kinetics of camel milk к-casein hydrolysis is similar to bovine milk к-casein hydrolysis. However, casein aggregation in camel milk to induced gelation is observed after >95% of the к-casein has been hydrolysed by camel chymosin (Hailu et al. Reference Hailu, Hansen, Seifu, Eshetu and Ipsen2016), unlike bovine milk where this occurs at a substantially lower level (60–70%) of к-casein hydrolysis (Lomholt & Qvist, Reference Lomholt and Qvist1997).
Texture, gelation and foaming properties of camel milk
Proteins in food contribute to food quality and stability by forming interfacial films to stabilise emulsions and foams as well as by interacting with each other in a network to form foams, edible films and ensure thermal stability (Foegeding et al. Reference Foegeding, Davis, Doucet and McGuffey2002). Caseins have a flexible and disordered structure that results in low viscoelastic properties, while the globular whey proteins (lysozyme, β-LG, bovine SA, α-LA) provide surface active properties (foams and films with high rigidity) for the products (Graham & Phillips, Reference Graham and Phillips1980). β-casein is also rather surface active as a result of its amphipathic nature and plasmin hydrolysed bovine β-casein has shown improved foam forming and stabilising properties compared to its intact form (Caessens et al. Reference Caessens, Gruppen, Visser, Van Aken and Voragen1997). The gelling and thickening properties of whey proteins are due to unfolding and denaturation followed by aggregation (Foegeding et al. Reference Foegeding, Davis, Doucet and McGuffey2002). The study of Laleye et al. (Reference Laleye, Jobe and Wasesa2008) on functional properties of camel milk whey indicated that absence of β-LG in camel milk led to protein denaturation occurring at a decreased temperature compared to bovine whey protein.
At a pH value of 5·0 poor and unstable emulsions are formed from camel milk whey protein as a result of partial denaturation of the protein and resulting aggregation (Laleye et al. Reference Laleye, Jobe and Wasesa2008). In bovine milk β-LG is primarily involved in aggregation caused by thermal treatment (Graham & Phillips, Reference Graham and Phillips1980; Dickinson, Reference Dickinson1999), and the absence of β-LG can thus partially explain why camel milk whey protein behaves differently (Merin et al. Reference Merin, Bernstein, Bloch-Damti, Yagil, van Creveld, Lindner and Gollop2001; El-Agamy, Reference El-Agamy2007; El-Hatmi et al. Reference El-Hatmi, Girardet, Gaillard, Yahyaoui and Attia2007; Laleye et al. Reference Laleye, Jobe and Wasesa2008; Hinz et al. Reference Hinz, O'Connor, Huppertz, Ross and Kelly2012; Saliha et al. Reference Saliha, Dalila, Chahra, Saliha and Abderrahmane2013). Laleye et al. (Reference Laleye, Jobe and Wasesa2008) reported that camel milk whey can form stable foams at pH 7, which could provide a basis for formulation of foods with high nutritional value and appealing functional features.
Camel milk protein solubility is different from that of cow's milk (Wangoh et al. Reference Wangoh, Farah and Puhan1998). At higher salt content of 0·5 M the αs1-casein is insoluble (Dziuba & Dziuba, Reference Dziuba and Dziuba2014). The heat stability of camel milk whey is decreased at the iso-electric point (4·5 pH) (Laleye et al. Reference Laleye, Jobe and Wasesa2008) as a result of reduced solubility because of less electrostatic repulsion between proteins. The pattern of casein demineralisation in camel milk is different from that of bovine milk casein, in that it is initiated at around pH 5·8 but starts already at the beginning of acidification in bovine milk casein (Attia et al. Reference Attia, Kherouatou, Nasria and Khorchani2000). This can be related to abundance of insoluble salts in camel milk casein micelles that provide resistance to pH change (Kherouatou et al. Reference Kherouatou, Nasri and Attia2003). Solubilisation of P and citrate in milk occurs at 4·8 and 5·2 pH in camel and cows’ milk, respectively (Attia et al. Reference Attia, Kherouatou, Nasria and Khorchani2000).The lower amount of к-casein reported (Kappeler et al. Reference Kappeler, Farah and Puhan1998; Ramet, Reference Ramet2001; El-Agamy, Reference El-Agamy, Park and Haenlein2006) results in formation of a casein network that can easily disrupt during cutting of cheese curd, leading to loss of dry matter (casein) to the whey (Ramet, Reference Ramet2001; Bornaz et al. Reference Bornaz, Sahli, Attalah and Attia2009).
Specific health effects of camel milk proteins
A variety of biologically active compounds can be obtained from milk and milk products which provides an opportunity to combat various diseases (Dziuba & Dziuba, Reference Dziuba and Dziuba2014). The absence of β-LG in camel milk (similar to human milk) points to camel milk being better able to substitute human milk for infant diet formulation than bovine milk (El-Hatmi et al. Reference El-Hatmi, Girardet, Gaillard, Yahyaoui and Attia2007; Saliha et al. Reference Saliha, Dalila, Chahra, Saliha and Abderrahmane2013). The presence of whey protein components in higher amounts in camel milk (El-Agamy, Reference El-Agamy2000; Merin et al. Reference Merin, Bernstein, Bloch-Damti, Yagil, van Creveld, Lindner and Gollop2001; Kappeler et al. Reference Kappeler, Heuberger, Farah and Puhan2004) might provide a comparative functional advantage to camel milk consumers. Colostrum from bovine and camel differs in composition; SA is more abundant in camel colostrum whereas β-LG is the main whey protein present in bovine colostrum (Merin et al. Reference Merin, Bernstein, Bloch-Damti, Yagil, van Creveld, Lindner and Gollop2001). Camel colostrum can thus be proposed to have other health benefits (i.e. immune factors) compared to bovine colostrum.
Hydrolysis of camel β-casein by chymotrypsin results in increased antioxidant properties and inhibition of ACE, which suggests camel milk casein as a natural anti-hypertensive agent similar to what can be obtained from bovine milk (Salami et al. Reference Salami, Moosavi-Movahedi, Moosavi-Movahedi, Ehsani, Yousefi, Farhadi, Niasari-Naslaji, Saboury, Chobert and Haertlé2011). Camel milk has antioxidant properties (Salwa & Lina, Reference Salwa and Lina2010) as a consequence of its high vitamin C contents which helps to control tissue damage (Abbas et al. Reference Abbas, Ashraf, Nazir and Sarfraz2013). The casein macro peptides (CMP) released as a result of the hydrolysis of κ-casein have functional properties in binding cholera enterotoxins and E. coli, inhibit bacterial and viral adhesion, modulate immune activity, enhance the growth of bifidobacteria, minimise gastric juice secretion and regulate blood flow (Dziuba & Dziuba, Reference Dziuba and Dziuba2014). Enzymatic hydrolysis of proteins can release bioactive peptides with higher physiologic activity. The tripeptides Val-Pro-Pro and Ile-Pro-Pro are inhibitors of the angiotensin converting enzyme (ACE) properties of those peptides that regulate the blood pressure (Dziuba & Dziuba, Reference Dziuba and Dziuba2014). Camel milk fermented with Lactobacillus rhamnosus PTCC 1637 contains significant amounts of peptides with ACE-inhibitory and antioxidant properties (Moslehishad et al. Reference Moslehishad, Ehsani, Salami, Mirdamadi, Ezzatpanah, Naslaji and Moosavi-Movahedi2013) compared to bovine milk. This difference could be attributed to the structural difference between camel and bovine milk proteins as camel milk β-casein is richer in proline, is shorter by two amino acid residues compared to bovine and its N-terminal fragment is different from that of other species. The peptide fragment identified from β-casein N-terminal of camel milk contains opioid peptides similar to proteose peptones from bovine casein (Beg et al. Reference Beg, Bahr-Lindström, Zaidi and Jörnvall1986b). Peptides generated by enzymatic digestion of LF have an antimicrobial potential (Abbas et al. Reference Abbas, Ashraf, Nazir and Sarfraz2013), which makes it a suitable additive for food preservation. Glycosylation dependent cell adhesion molecule (PP3) found in both cow and camel milk have a similar role in preventing the infection of respiratory and gastrointestinal tract of sucking young (Girardet et al. Reference Girardet, Saulnier, Gaillard, Ramet and Humbert2000). Hence, the presence of PP3 in higher concentration in camel milk El-Hatmi et al. (Reference El-Hatmi, Girardet, Gaillard, Yahyaoui and Attia2007) might provide an added advantage to camel milk in prevention of infection.
Beg et al. (Reference Beg, Bahr-Lindstrom, Zaidi and Jornvall1986a) indicated the presence of peptides resembling insulin in camel milk whey protein and a hypoglycaemic effect of camel milk has been reported by Hamad et al. (Reference Hamad, Abdel-Rahim and Romeih2011). Consumption of camel milk has been indicated to result in reduction of insulin dose for type 1 diabetic patients (Agrawal et al. Reference Agrawal, Jain, Shah, Chopra and Agarwal2011). In-vivo trials on rats fed milk from different mammals (i.e. bovine, goat, camel and buffalo) for type-1 diabetes showed, enhancement of the insulin level and improvement in hyperglycaemia and oxidative damage of type-1 diabetes as a result of camel milk feeding (Meena et al. Reference Meena, Rajput, Pandey, Sharma and Singh2016). An antihyperglycaemic effect of camel milk has also been indicated in the review paper of Shori (Reference Shori2015), which states that a number of studies conducted to test the effect on reducing blood glucose level and enhancing availability of insulin in the plasma showed a positive effect for camel milk compared to milk form other mammals.
Conclusion
The composition and molecular properties of camel milk protein is different from bovine and human milk. Camel milk has comparatively higher functional contribution to human health as a result of the unique composition of caseins and whey proteins (SA, α-LA, LF, PGRP-S, and IgG with the different variants IgG1, IgG2, and IgG3). The absence of β-LG in camel milk has been indicated and its possible effect on camel milk processing into milk powder as well as other dairy products needs to be studied. Relatively higher concentrations of camel milk whey proteins for utilisation in functional food production need to be elucidated. Thermal properties and pH sensitivity of camel milk should be considered in processing and analysing camel milk proteins and their products. Surface active properties of camel milk proteins also need to be investigated to enable utilisation of camel milk proteins in food formulation.