Tuberculosis caused by Mycobacterium tuberculosis and Mycobacterium bovis was in the mid-twentieth century one of the illnesses most frequently associated with consumption of raw milk (Claeys et al., Reference Claeys, Cardoen, Daube, De Block, Dewettinck, Dierick, De Zutter, Huyghebaert, Imberechts, Thiange, Vandenplas and Herman2013). Early this century Mycobacterium avium subsp. Paratuberculosis complex (MAP), the aetiologic agent of Johne's diseases in ruminants, was associated with Crohn's disease in humans (Hermon-Taylor et al., Reference Hermon-Taylor, Bull, Sheridan, Cheng, Stellakis and Sumar2000; Chacon et al., Reference Chacon, Bermudez and Barletta2004). Dairy products, including pasteurized bovine milk, have been suggested as potential sources, since MAP was detected in retail supplies of whole pasteurized milk in the UK (Millar et al., Reference Millar, Ford and Sanderson1996). As a result, the dairy industry are concerned about the possibility that pasteurization treatments may not be effectively inactivating this microorganism, although there is some controversy regarding this particular issue (Grant et al., Reference Grant, Hitchings, McCartney, Ferguson and Rowe2002; Lund et al., Reference Lund, Gould and Rampling2002).
In order to obtain safer milk and dairy products whilst avoiding the disadvantages of thermal treatments, new non-thermal technologies such as High Hydrostatic Pressure (HHP) and Ultra-High Pressure Homogenization (UHPH) have been suggested as alternatives to traditional pasteurization. Although HHP and UHPH are both pressure-based technologies, the physical principles that rule their effect on microorganisms are not exactly the same.
HHP treatments consist of subjecting a previously packaged liquid or solid food submerged in a water solution (hydrostatic) to pressures usually above 200 MPa. In HHP, treatments interfere with various cellular structures or functions, such as cytoplasmic membrane (Isaacs et al., Reference Isaacs, Chilton, Mackey, Ledward, Johnston, Earnshaw and Hasting1995; Chilton et al., Reference Chilton, Isaacs, Mackey, Stenning and Heremans1997; Pagan and Mackey, Reference Pagan and Mackey2000), ribosomes (Isaacs et al., Reference Isaacs, Chilton, Mackey, Ledward, Johnston, Earnshaw and Hasting1995; Niven et al., Reference Niven, Miles and Mackey1999) and specific enzymes (Isaacs et al., Reference Isaacs, Chilton, Mackey, Ledward, Johnston, Earnshaw and Hasting1995; Simpson and Gilmour, Reference Simpson and Gilmour1997; Ritz et al., Reference Ritz, Jugiau, Rama, Courcoux, Semenou and Federighi2000). UHPH is similar to homogenization processes where a liquid food is forced through a very narrow gap of an adjustable valve causing an increase of pressure above 100 MPa immediately before passing through this gap, and causing different physical changes to the microorganisms and food structures (Dumay et al., Reference Dumay, Chevalier-Lucia, Picart-Palmade, Benzaria, Gracia-Julia and Blayo2013). In UHPH treatments microorganisms are subjected to a combination of shear forces, cavitation shock waves and impingement (Tahiri et al., Reference Tahiri, Makhlouf, Paquin and Fliss2006; Velazquez-Estrada et al., Reference Velazquez-Estrada, Hernandez-Herrero, Lopez-Pedemonte, Brinez-Zambrano, Guamis-Lopez and Roig-Sagues2011). There is a significant increase of the temperature when the sample passes through the valve, although this lasts for a very short period of time (Amador-Espejo et al., Reference Amador-Espejo, Hernandez-Herrero, Juan and Trujillo2014).
In general, Gram positive bacteria are more resistant than Gram-negative bacteria to UHPH, while HHP resistance of both groups overlap (Smelt, Reference Smelt1998; Wuytack et al., Reference Wuytack, Diels and Michels2002). The effect of these technologies has been studied on both pathogen and spoilage microorganisms usually present in milk (Gervilla et al., Reference Gervilla, Ferragut and Guamis2000; Wuytack et al., Reference Wuytack, Phuong, Aertsen, Reyns, Marquenie, De Ketelaere, Masschalck, Van Opstal, Diels and Michiels2003; Diels et al., Reference Diels, Callewaert, Wuytack, Masschalck and Michiels2005; Briñez et al., Reference Briñez, Roig-Sagués, Hernández Herrero and Guamis-López2006a, Reference Briñez, Roig-Sagués, Hernández Herrero and Guamis-López2006b, Reference Briñez, Roig-Sagues, Hernandez-Herrero and Guamis-Lopez2007; Roig-Sagués et al., Reference Roig-Sagués, Velazquez, Montealegre-Agramont, Lopez-Pedemonte, Brinez-Zambrano, Guamis-Lopez and Hernández-Herrero2009; Amador-Espejo et al., Reference Amador-Espejo, Hernandez-Herrero, Juan and Trujillo2014). However, few surveys concerning HHP have included acid-resistant microorganisms (López-Pedemonte et al., Reference López-Pedemonte, Sevilla, Garrido, Aduriz, Guamis, Juste and Roig-Sagués2006; Donaghy et al., Reference Donaghy, Linton, Patterson and Rowe2007), and no previous references were found about the effect of UHPH on Mycobacterium spp.
Besides the high risk involved in manipulating pathogenic strains of Mycobacterium, they grow up very slowly in culture media, taking several weeks to form visible colonies. This makes it very difficult to quantify the lethal effect caused by treatments. For that reason, using a genetically and phenotypically faster growing species of this genus, such as M. smegmatis, has been suggested for studies of this kind (Lynch et al., Reference Lynch, Jordan, Kelly, Freyne and Murphy2007; Peterz et al., Reference Peterz, Butot, Jagadeesan, Bakker and Donaghyc2016). The objectives of this study were: to assess the efficiency of HHP or UHPH against strains of M. smegmatis inoculated into skimmed or whole milk and to discuss whether M. smegmatis can be considered a suitable surrogate for other pathogenic Mycobacterium spp. in high pressure inactivation trials using milk.
Materials and Methods
Bacterial strains
Three strains of M. smegmatis (CECT 3017, 3020 and 3032) were used. The strains were provided by the Spanish Type Culture Collection of the University of Valencia, Spain, as freeze-dried cultures. The strains were re-hydrated in tryptone soy broth (TSB, Oxoid Ltd., Basingstoke, Hampshire, UK) an incubated at 37°C for 18 h. Subsequently, the strains were streaked on tryptone soy agar plates (TSA, Oxoid) and incubated under the same conditions in order to obtain pure individual colonies to prepare Cryobeads (Nalgene System 100 Laboratories Microkit Iberica S.L., Madrid, Spain) to provide stock cultures for the following assays. Once prepared, the Cryobeads were kept at −22°C.
Milk sample preparation
Before each experiment, one cryobead of each strain was inoculated into 10 ml of TSB and incubated at 37°C for 20 h. After incubation, the broth was spread using a disposable loop on TSA slants and incubated at 37°C for 20 to 24 h. Subsequently, a cell suspension was prepared in 11 ml of a tryptone sodium chloride solution made of 1 g/l of tryptone pancreatic casein digestion (Oxoid) and 8.5 g/l of sodium chloride (Panreac, Montcada i Reixac, Barcelona, Spain), to obtain a final concentration of 9 to 9.5 Log CFU/ml, measured by optical density at 405 nm using a Cecil 9000 spectrophotometer (Cecil Instruments, Cambridge, UK). Then 10 ml of this cell suspension were used to inoculate 1 litre of commercial sterilized (UHT) skimmed or whole milk to reach a final concentration of approximately 7 Log CFU/ml.
High hydrostatic pressure treatments
To perform the HHP treatments, milk samples were dispensed in sterile hermetic 30-ml low-density polyethylene bottles (Azlon; Bibby Sterilin, Ltd., United Kingdom) and vacuum packed in a double layer of plastic bags (bb4.l; Cryovac Packaging, Sant Boi de Llobregat, Spain) and placed in a refrigerated water bath at 6 or 20°C depending on the treatment to be applied. Samples were pressurized using a discontinuous HHP equipment (model S-FL-850-9-W; Stansted Fluid Power, Stansted, United Kingdom), at two different temperatures (6 or 20°C) combined with three levels of pressure (300, 400, and 500 MPa) during 10 min. Non-pressurized control samples were used as reference to determine the initial counts (N 0). All three M. smegmatis strains (CECT 3017, 3020 and 3032) were used for HHP series of experiments.
Ultra-high pressure homogenization treatments
For each experiment 2.0 litre of milk samples inoculated with M. smegmatis CECT 3017 were previously tempered to 6°C (inlet temperature) and then subjected to a single cycle treatment of UHPH at 200, 300 and 400 MPa using an UHPH benchtop homogenizer Stansted FPG12500 (Stansted Fluid Power Ltd., Essex, UK) equipped with two intensifiers that allowed a constant flow rate of approximately 7 l/h and able to achieve a peak pressure of 400 MPa. To avoid an excessive increase in the temperature of the samples during the treatments, an external jacket with a constant circulation of ethylene glycol at 2°C was located immediately after the high pressure valve.
Microbiological analysis
For both HHP and UHPH treated and unpressurized control samples microbiological analyses were performed immediately after the treatments (day 0) and after 5 and 8 d of storage at 6°C. To assess the level of lethality achieved and the degree of injury caused by the treatments decimal dilutions were prepared in peptone water (10 g/l of peptone and 5 g/l of NaCl, Oxoid) and plated in TSAYE (tryptone soy agar with additional 6 g/l of yeast extract, Oxoid) and TSAYE + NaCl (the previously described medium with additional 30 g/l of NaCl). Plates were then incubated at 37°C for 24 or 48 h. This differential plating technique enables injuries to be monitored since both non-injured and injured cells can grow on TSAYE whereas only the non-injured cells are able to form colonies in the presence of NaCl (Patterson, Reference Patterson2005). Results were expressed as the logarithm of CFU/ml of milk and lethality was calculated as the difference between the logarithms of colony counts of the unpressurized (N 0) and treated samples (N) using the following formula:

Statistical analysis
All experiments were repeated three times with duplicate analysis. One-way analysis of variance (ANOVA) was used to compare the means. Differences were considered to be significant at P < 0.05. All statistical analyses were performed with the R system for statistical computation (R Foundation for Statistical Computing, Vienna, Austria 2014, http://www.R-project.org).
Results
Lethal effect of HHP treatments on Mycobacterium smegmatis strains
Table 1 shows the lethality caused by HHP treatments on M. smegmatis strains CECT3017, CECT3020 and CECT3032 inoculated into whole and skimmed milk, respectively. In all cases, the inactivation of the inoculated cells was almost complete after 500 MPa treatments. On the contrary, 300 MPa HHP treatments hardly inactivated any of the Mycobacterium strains. Differences between strains were only observed for 400 MPa treatments. At this pressure maximum lethality was achieved on strain CECT3032, which was significantly more sensitive to HHP than the other two strains. Maximum lethality observed was in average 1.6 Log CFU/ml higher than that observed for strain CECT3017 in whole milk treated at 6°C. Treatments at 20°C increased significantly lethality values related to treatments at 6°C only for strain CECT3032. At this temperature, the 400 MPa HHP treatment was more efficient in absence of fat. Significant differences were observed when the counts obtained in TSAYE and TSAYE + salt media were compared for samples treated at 6°C, which meant that HHP treatments caused a great number of injured cells which growth was inhibited as a consequence of the presence of salt. These differences oscillated between 1.59 and 2.46 Log CFU/ml. In samples treated at 300 MPa these differences were not appreciated due to the scarce lethal effect caused, while in samples treated at 500 MPa inactivation of cells was complete.
Table 1. Lethality values of M. smegmatis strains (Mean ± sd) inoculated into whole and skimmed milk after different HHP treatments at 300, 400 and 500 MPa at 6°C and 20°C

Results are the mean and standard deviation of the difference between the initial and final counts expressed as Log CFU/ml.
Lethal effect of UHPH treatments on Mycobacterium smegmatis CECT3017
Figure 1 shows the lethality caused by UHPH treatments when strain CECT3017 was inoculated in whole (A) and skimmed milk (B), respectively. In both cases, maximum lethality values were observed for both 300 and 400 MPa treatments. The fat content of milk did not cause significant differences on the efficacy of the treatments. Count means obtained in TSAYE were higher than those obtained in TSAYE + salt but differences were not statistically significant (P > 0.05) indicating that UHPH treatments caused low number of injured cells. For UHPH samples lethality values hardly increased 0.5 Log CFU/ml due to the pressure increment from 300 to 400 MPa.
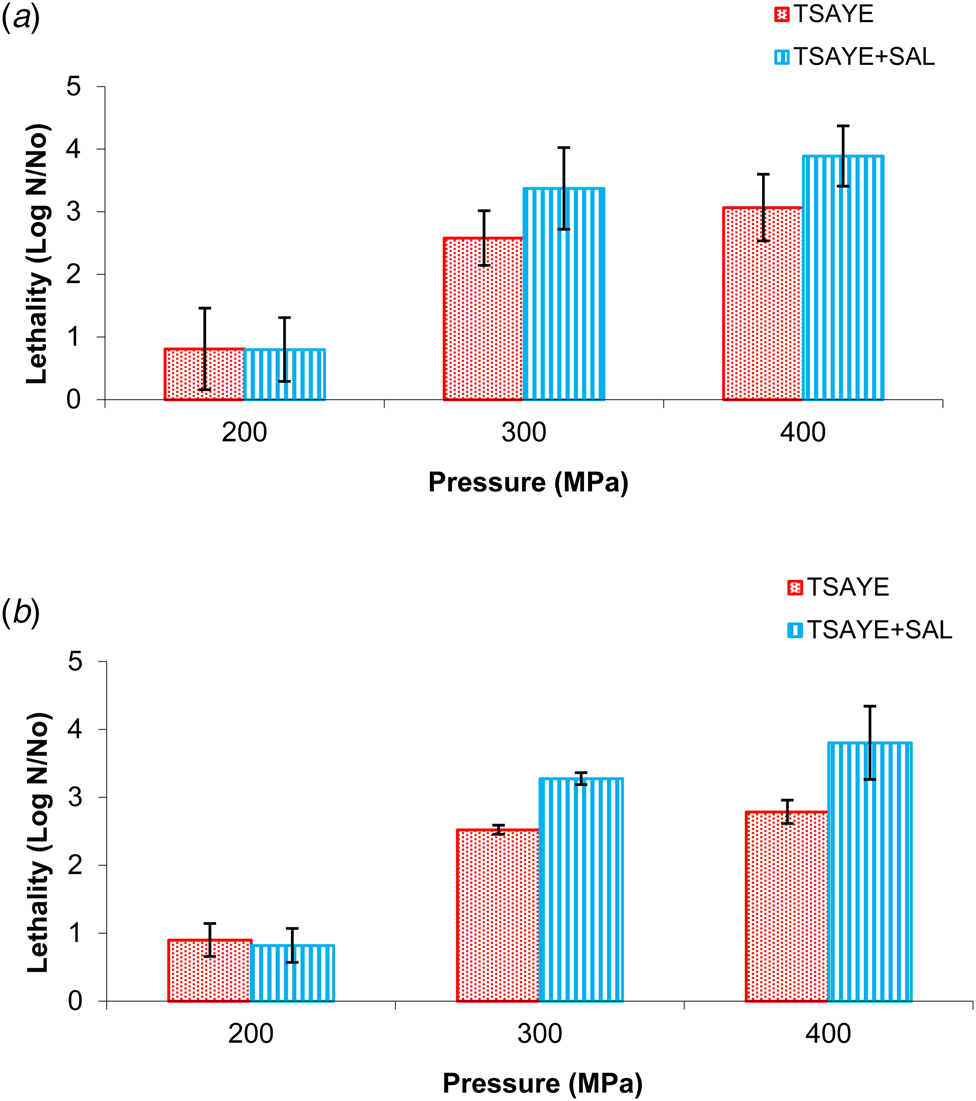
Fig. 1. Lethality values of M. smegmatis CECT3017 inoculated into whole (a) and skimmed (b) milk after different UHPH treatments at 200, 300 and 400 MPa.
Evolution of HHP treated Mycobacterium smegmatis strains during cold storage
None of the inoculated strains increased their counts significantly during the 8 d of storage at 4°C after the HHP treatments either in whole (Fig. 2) or skimmed milk (Fig. 3). When counts in TSAYE and TSAYE + salt were compared during this period it was observed that the differences were similar, indicating that injured cells were unable to repair, although a decrease in counts was not observed. Similar behaviour was observed for all tested strains and neither the kind of matrix nor the temperature of the treatment apparently had any significant influence. Figure 4 shows the same evolution for strain CECT3017 in whole (A) and skimmed (B) milk after UHPH treatments. Similar behaviour to HHP treated samples was observed.

Fig. 2. Evolution of M. smegmatis strains inoculated into whole milk after a 400 MPa HHP treatment at 6°C (a) and 20°C (b) during cold storage at 6°C

Fig. 3. Evolution of M. smegmatis strains inoculated into skimmed milk after a 400 MPa HHP treatment at 6°C (a) and 20°C (B) during cold storage at 6°C
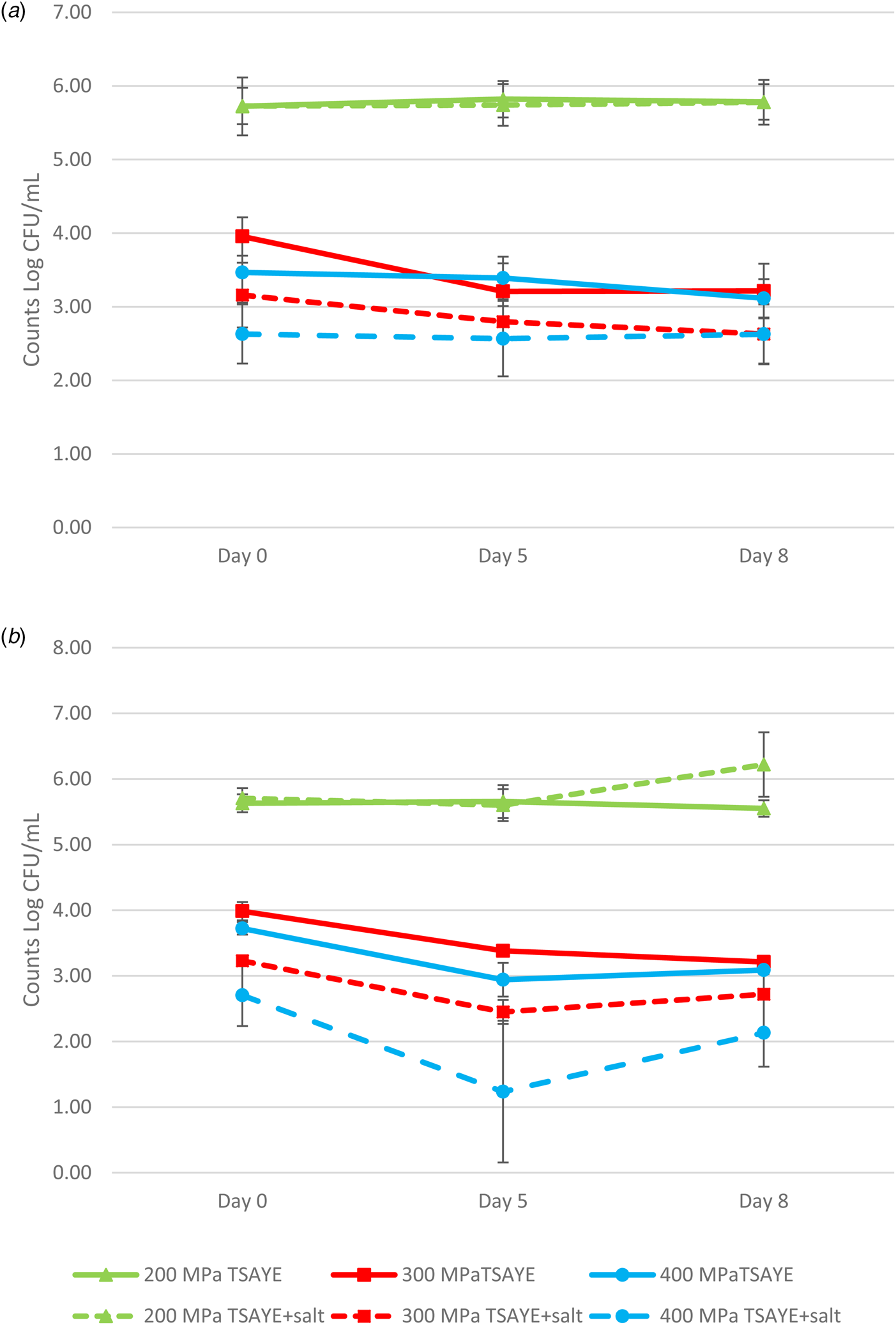
Fig. 4. Evolution of M. smegmatis strain CECT3017 counts in whole (a) and skimmed (b) milk during cold storage at 6°C after UHPH treatments at 200, 300 and 400 MPa.
Evolution of UHPH treated Mycobacterium smegmatis strains during cold storage
Figure 4 shows the evolution for strain CECT3017 in whole (A) and skimmed (B) milk after UHPH treatments. Counts did not increase during the 8 d storage at refrigeration temperature. Counts on TSAYE and TSAYE + salt did not differ.
Discussion
There have been few studies of the effect of HHP on Mycobacterium spp. and more specifically on MAP, while no references were found concerning UHPH. López-Pedemonte et al. (Reference López-Pedemonte, Sevilla, Garrido, Aduriz, Guamis, Juste and Roig-Sagués2006) studied the effect of HHP treatments (300, 400 and 500 MPa) on two strains of MAP inoculated into sterilized milk. The level of inactivation for both strains of MAP was proportional to the increase in the pressure applied. Nevertheless, they reported mean reductions of 4.58 log CFU/ml after a 500 MPa, but only 1.59 log CFU/ml after a 400 MPa treatment. These authors suggested that it would be feasible to inactivate this microorganism by means of HHP provided that the load of MAP in raw milk is less than 4 Log CFU/ml, and that these inactivation values were comparable to those obtained in previous pasteurization studies. However, these reductions are considerably lower than the ones obtained in this survey with M. smegmatis using similar processing conditions. Considering our most resistant strain to HHP (CECT 3017), the lethality obtained at 400 MPa oscillated between 2.28 and 3.74 Log CFU/ml. Nevertheless, Donaghy et al. (Reference Donaghy, Linton, Patterson and Rowe2007) in a similar survey using two strains of MAP obtained a mean log reduction of 2.56 Log CFU/ml at 400 MPa while after a 500 MPa treatment the mean log reduction achieved was 6.52 Log CFU/ml into sterilized whole milk, that were closer to the values obtained by us.
Therefore, the usefulness of M. smegmatis in this case is questionable since it demonstrated equal or lower sensitivity to HHP treatments than MAP, and consequently the complete inactivation of a load of 5-Log CFU/ml or above would not necessarily imply the inactivation of an equal load of MAP or any other pathogenic Mycobacteria.
M. smegmatis has also been tested as potential surrogate for other kind of treatments. Lynch et al. (Reference Lynch, Jordan, Kelly, Freyne and Murphy2007) observed that strain mc (2)155 of M. smegmatis showed a greater D value at 65°C (25 s) than MAP strain 19698 (20 s), but Peterz et al. (Reference Peterz, Butot, Jagadeesan, Bakker and Donaghyc2016) could not confirm M. smegmatis as a surrogate of MAP for direct steam injection (DSI) at 105°C since greater spiking levels of M. smegmatis in samples were consistently achievable, making difficult to compare results with MAP. Altaf et al. (Reference Altaf, Miller, Bellows and O'Toole2010) previously considered that M. smegmatis as surrogate of M. tuberculosis was significantly less effective in the detection of anti-M. tuberculosis compounds.
Temperature in HHP treatments did not affect lethality values, except for strain CECT3032, which showed to be more sensitive to HHP than the other strains tested. López-Pedemonte et al. (Reference López-Pedemonte, Sevilla, Garrido, Aduriz, Guamis, Juste and Roig-Sagués2006) also reported no effect of the temperature with the HHP treatment on MAP. Nevertheless, previous surveys indicated that in other microorganisms such as Listeria innocua, decreasing the temperature from 20°C to 2°C increased significantly the efficiency of the HHP treatments (Gervilla et al., Reference Gervilla, Capellas, Ferragut and Guamis1997). Different piezo-tolerances between strains have also been previously described for MAP (López-Pedemonte et al., Reference López-Pedemonte, Sevilla, Garrido, Aduriz, Guamis, Juste and Roig-Sagués2006; Donaghy et al., Reference Donaghy, Linton, Patterson and Rowe2007) and for other species (Alpas et al., Reference Alpas, Kalchayanand, Bozoglu, Sikes, Dunne and Ray1999; De Lamo-Castellví et al., Reference De Lamo-Castellví, Roig-Sagues, Capellas, Hernandez-Herrero and Guamis2005).
Concerning the effect of the fat content of milk, although no differences were observed in this survey between whole and skimmed milk, some studies suggested that the level of fat in milk influences negatively the microbial sensitivity to HHP (Patterson et al., Reference Patterson, Quinn, Simpson and Gilmour1995). This effect has been attributed to the presence of liposoluble substances with antimicrobial effect, which may cause interchanging of triglycerides of milk with lipoproteins of cellular membrane, altering the permeability of microorganisms (Gervilla et al., Reference Gervilla, Ferragut and Guamis2000).
Bacterial cells exposed to different physical and chemical treatments suffer injuries that can be reversible in food materials during storage (Bozoglu et al., Reference Bozoglu, Alpas and Kaletunc2004). Several authors have detected injured cells after high pressure treatments (Patterson et al., Reference Patterson, Quinn, Simpson and Gilmour1995; Simpson and Gilmour, Reference Simpson and Gilmour1997; Chen and Hoover, Reference Chen and Hoover2003), suggesting that under favourable conditions such as prolonged storage in a suitable substrate, these cells may be able to repair. This potential repairing is problematic for foodborne pathogens if they can later grow under the matrix conditions (McClements et al., Reference McClements, Patterson and Linton2001; Bozoglu et al., Reference Bozoglu, Alpas and Kaletunc2004). Traditionally, injured cells produced by HHP treatments have been detected using classic culture techniques (comparing viable counts on non-selective and selective culture media). The use of salt to act as selective agent and inhibit growth of injured cells after HHP treatments was suggested by Patterson et al. (Reference Patterson, Quinn, Simpson and Gilmour1995). Enriching the culture media (in our case with TSA) with yeast extract increases the possibility of injured cells to recover and enables injury to be monitored. Both non-injured and injured cells were able to form colonies on TSAYE whereas only non-injured cells formed colonies in the presence of NaCl. These authors observed differences between TSAYE and TSAYE + salt depending on the specie and/or the strain.
Another factor that has been suggested to be one of the contributory factors to the apparent heat resistance to some Mycobacterium, such as MAP, is its tendency to form large clumps, with the cells at the periphery protecting those at the centre of such clumps (Rowe et al., Reference Rowe, Grant, Dundee and Ball2000). Nevertheless, HHP acts in an isostatic manner so that all parts of the food, and presumably cells within clumps, are subjected to the same pressure at exactly the same time nullifying, to some extent, effects of the clumping phenomenon and making all cells equally susceptible to this process (Patterson, Reference Patterson2005; Donaghy et al., Reference Donaghy, Linton, Patterson and Rowe2007).
No references concerning the effect of UHPH on Mycobacteria have been found in the literature, but the lethality values observed on CECT3017 strain were lower than those reported for other microorganisms such as E. coli (Briñez et al., Reference Briñez, Roig-Sagués, Hernández Herrero and Guamis-López2006a), Listeria innocua (Briñez et al., Reference Briñez, Roig-Sagués, Hernández Herrero and Guamis-López2006b) and Listeria monocytogenes (Roig-Sagués et al., Reference Roig-Sagués, Velazquez, Montealegre-Agramont, Lopez-Pedemonte, Brinez-Zambrano, Guamis-Lopez and Hernández-Herrero2009) using similar conditions, but significantly higher than those reported for some Gram positive cocci such as Staphylococcus spp. (Briñez et al., Reference Briñez, Roig-Sagues, Hernandez-Herrero and Guamis-Lopez2007) which proved to be the most resistant vegetative bacteria to UHPH. For UHPH processing, it is also necessary to take into account the temperature rise in the fluid that occurs immediately after passing through the high pressure valve. Due to the sudden pressure decrease to atmospheric pressure, energy is dissipated in the form of heat and heating of the fluid is usually controlled by means of heat-exchangers circulating a refrigerating fluid at less than 4°C; thus heating of the fluid food can be reduced to less than 0.5 s (Datta et al., Reference Datta, Hayes, Deeth and Kelly2005; Chevalier-Lucia et al., Reference Chevalier-Lucia, Blayo, Gracia-Julia, Picart-Palmade and Dumay2011). In our case, milk samples were UHPH processed at an inlet temperature of 6 ± 2°C and temperature values after the high pressure homogenizing are estimated to reach values between 42 to 78°C for 200 to 400 MPa (according to Picart, Reference Picart2004; using a similar ultra-high pressure homogenizer with one intensifier). With the available cooling system (by means of an external jacket built around the pipeline immediately after the high pressure and secondary valves, and a coil after the second valve, both carrying ethylene glycol at 2°C), milk temperature decreased to approximately 18 to 20°C at the outlet and then quickly cooled and stabilized at 6°C. Thus, thermal contribution to inactivation of M. smegmatis CECT3017 cannot be ruled out. Regarding the influence of fat content of food matrixes on UHPH lethality values, results of our research did not differ between whole or skimmed milk, However, the results reported are sometimes contradictory on this issue (Vachon et al., Reference Vachon, Kheadr, Giasson, Paquin and Fliss2002; Diels et al., Reference Diels, Callewaert, Wuytack, Masschalck and Michiels2005; Briñez et al., Reference Briñez, Roig-Sagués, Hernández Herrero and Guamis-López2006a; Roig-Sagués et al., Reference Roig-Sagués, Velazquez, Montealegre-Agramont, Lopez-Pedemonte, Brinez-Zambrano, Guamis-Lopez and Hernández-Herrero2009). Diels et al. (Reference Diels, Callewaert, Wuytack, Masschalck and Michiels2005) proposed that bacterial inactivation decreases with increasing viscosity of the matrix, this effect being more pronounced at higher pressures. Roig-Sagués et al. (Reference Roig-Sagués, Velazquez, Montealegre-Agramont, Lopez-Pedemonte, Brinez-Zambrano, Guamis-Lopez and Hernández-Herrero2009) observed that increasing the fat content to 10% increases the lethality caused by a single cycle UHPH treatment at pressures up to 400 MPa on Listeria monocytogenes. Fat content also reduced the ability of L. monocytogenes to recover and grow during the subsequent cold storage. This could be a consequence of higher maximum temperatures reached during UHPH treatments in samples with higher levels of fat, which could contribute to the lethal effect achieved. Diels et al. (Reference Diels, Callewaert, Wuytack, Masschalck and Michiels2005) and Briñez et al. (Reference Briñez, Roig-Sagués, Hernández Herrero and Guamis-López2006a) also observed that Escherichia coli presented higher inactivation ratios when it was UHPH-treated in whole milk than in skimmed milk.
On UHPH treated samples, although mean counts were numerically greater on TSAYE than in TSAYE + salt, there were no significant differences between them in any treated sample. Our results are in agreement with previous research performed in milk and in other food matrices such as fruit juices or whole egg (Briñez et al., Reference Briñez, Roig-Sagués, Hernández Herrero and Guamis-López2006a, Reference Briñez, Roig-Sagués, Hernández Herrero and Guamis-López2006b; Briñez et al., Reference Briñez, Roig-Sagues, Hernandez-Herrero and Guamis-Lopez2007; Roig-Sagués et al., Reference Roig-Sagués, Velazquez, Montealegre-Agramont, Lopez-Pedemonte, Brinez-Zambrano, Guamis-Lopez and Hernández-Herrero2009; Velazquez-Estrada et al., Reference Velazquez-Estrada, Hernandez-Herrero, Lopez-Pedemonte, Brinez-Zambrano, Guamis-Lopez and Roig-Sagues2011). Wuytack et al. (Reference Wuytack, Diels and Michels2002) compared the resistance of several species of both Gram-positive and Gram-negative bacteria to UHPH (100–300 MPa) treatments, failing to observe large differences among strains. This finding led the authors to suggest that UHPH does not cause sub-lethal injury reflecting the ‘all or nothing’ impact of this technique on bacteria. UHPH is a technology that produces mainly physical or structural damage on the cell. Some authors reported the complete cell rupture and loss of cytoplasmic contents caused by UHPH treatments on transmission electron micrographs (Vachon et al., Reference Vachon, Kheadr, Giasson, Paquin and Fliss2002; Tahiri et al., Reference Tahiri, Makhlouf, Paquin and Fliss2006). The higher resistance of Gram positive bacteria can be explained by the composition of the cell wall, since they have a thicker peptidoglycan layer than Gram negative (Wuytack et al., Reference Wuytack, Phuong, Aertsen, Reyns, Marquenie, De Ketelaere, Masschalck, Van Opstal, Diels and Michiels2003). No previous references have been found concerning the effect of UHPH on Mycobacterium species, but these microorganisms are characterized by the presence of a cell wall that is thicker than in many other bacteria and is hydrophobic and waxy, all of which makes a substantial contribution to the resilience of this genus. Regarding the tendency of Mycobacterium cells to form clumps and thus lowering the efficiency of inactivation processes, since UHPH is able to disrupt cell structures it would be logical to think that it could disintegrate the agglomerates naturally formed by the cells of this microorganism.
In conclusion, based on the results obtained and the results previously reported in the literature, we can conclude that using M. smegmatis as surrogate of pathogenic Mycobacteria (especially for MAP) to evaluate the efficacy of HHP treatments is not adequate, since M. smegmatis is more sensitive to these treatments than MAP. For UHPH treatments, there are no previous references on UHPH inactivation of pathogenic strains of Mycobacteria. Nevertheless, we showed that M. smegmatis is more sensitive to these treatments than other pathogenic bacteria usually present in milk (notably Staphylococcus aureus), and consequently M. smegmatis would not be a good marker to study the efficiency of UHPH treatments for pasteurization-like purposes.