Supplementation of ruminant diets with lipids has proved to be an efficient means for modifying milk fatty acids (FA) composition. Fats can be added to change the milk FA profiles to better match current human dietary concerns (Palmquist et al. Reference Palmquist, Lock, Shingfield and Bauman2005). In recent years, increasing the conjugated linoleic acid (CLA) concentration and mainly the cis-9 trans-11 isomer (rumenic acid, RA) representing 75 to 90% of total CLA, has been of particular interest.
Results from comparisons of different plant oils suggest that those rich in linoleic acid, such as sunflower oil (SO), may increase CLA most effectively (Collomb et al. Reference Collomb, Sieber and Bütikofer2004; Palmquist et al. Reference Palmquist, Lock, Shingfield and Bauman2005). However, most work has been undertaken with dairy cows and goats, and less is known about dairy ewes. Chilliard et al. (Reference Chilliard, Ferlay, Rouel and Lamberet2003) compiled research on the effects of lipid supplements on milk FA synthesis and concluded that there were many similarities among ruminants, but also substantial differences.
Unprotected oils are seldom used in ruminant diets because they are supposed to impair ruminal fermentation (Jenkins, Reference Jenkins1993; Harfoot & Hazlewood, Reference Harfoot, Hazlewood and Hobson1997) and, consequently, milk production and composition. However, more recent research (Mele et al. Reference Mele, Buccioni, Petacchi, Serra, Banni, Antongiovanni and Secchiari2006; Gómez-Cortés et al. Reference Gómez-Cortés, Frutos, Mantecón, Juárez, De la Fuente and Hervás2008) has shown that diets highly supplemented with soybean oil modified the FA profile without detrimental effects in animal performance. It is also demonstrated, in contrast to cow milk, that milk fat concentration is less likely to decrease when supplementary lipids are fed to ewes (Caja & Bocquier, Reference Caja and Bocquier2000; Casals et al. Reference Casals, Caja, Pol, Such, Albanell, Gargouri and Casellas2006; Gargouri et al. Reference Gargouri, Caja, Casals and Mezghani2006).
The objective of this work was to study the effect of a highly SO supplemented diet on ewe ruminal fermentation, and milk production and composition, paying particular attention to the FA profile and CLA isomer composition. The final aim was to produce a milk with a healthier lipid fraction composition and a higher RA concentration.
Materials and Methods
Animals, experimental design, and milking management
Twenty-four primiparous Spanish Assaf ewes in mid-lactation were used. Ewes were distributed in 4 lots of 6 animals, balanced for milk production and live weight, and allocated at random to two experimental treatments: two lots were used as the control (Control) and the other two received the diet supplemented with sunflower oil (SO).
Ewes were milked at 8.30 and 18.30 h in a 1×12 stall-milking parlour (DeLaval, Madrid, Spain). The experiment lasted for a total of 5 weeks in mid-lactation, and was carried out in accordance with the Spanish Royal Decree 1201/2005 for the protection of animals used for experimental purposes.
Experimental diets
The diets consisted of a high concentrate total mixed ration based on alfalfa hay and concentrate supplemented with 0 (Control) or 60 g sunflower oil/kg DM (SO). The ingredients and chemical composition of the experimental diets are given in Table 1. For the first week of the trial (adaptation week), all the animals received the control diet. Fresh diets were offered daily, ad libitum, at 9.00 and 19.00 h.
Table 1. Ingredients, chemical composition and nutritional values of experimental diets
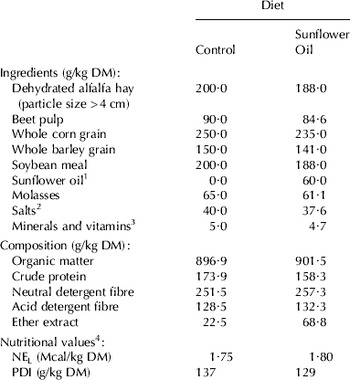
1 Sunflower oil contained (g/100 g of total fatty acids) C16:0 (7·5), C18:0 (4·1), cis-9 C18:1 (34·7), other C18:1 (0·9), C18:2 (51·4), C18:3 (0·2), C20:0 (0·3), C22:0 (0·6) and C24:0 (0·3)
2 Containing NaHCO3 (1·5%), CaCO3 (1·4%), Ca2HPO4 (0·6%) and mine salt (0·5%)
3 INA OV1 (EVIALIS, Madrid, Spain)
4 Net energy for lactation (NEL) and intestinal digestible protein (PDI) were estimated from INRA tables (Jarrige, Reference Jarrige1988) using INRATION 2.6 software
Measurements, sample collection, and chemical analysis
Samples of offered and refused diets were collected once a week, stored at –30°C and then freeze-dried. The intake therefore was recorded weekly for each experimental lot. Procedures described by the AOAC (2006) were used to determine dry matter (DM), ash, Kjeldahl nitrogen, and ether extract. Neutral detergent fibre (NDF) and acid detergent fibre (ADF) were determined by the methods described by Van Soest et al. (Reference Van Soest, Robertson and Lewis1991) and Goering & Van Soest (Reference Goering and Van Soest1970), adding sodium sulphite to the solution. NDF was assayed with α-amylase.
The individual milk production was recorded one day per week, both at morning and evening milking. Milk samples for determination of fat, protein and total solid concentrations (AOAC, 2006) were also collected one day per week from each animal. FA composition was determined in untreated samples of milk from each experimental lot, composited according to individual milk production. Milk fat was extracted following the Luna et al. (Reference Luna, Juárez and De la Fuente2005) method. Fatty acid methyl esters (FAME) were prepared by base-catalysed methanolysis of the glycerides according to the ISO-IDF procedure (2002). Analysis of FAME in hexane was performed on a gas-liquid chromatograph (Agilent 6890 N Network System, Palo Alto, USA) with auto injector, fitted with a flame ionization detector. FAME profile was determined by split injection (1:100) onto a CP-Sil 88 fused silica capillary column (100 m×0·25 mm i.d., 0·20 μm film thickness, Varian, Middelburg, The Netherlands) using a programmed temperature gradient method. Silver-ion HPLC (Ag+-HPLC) separation of CLA methyl esters was conducted using a Shimadzu HPLC apparatus (Model SPE-MA10AVP, Kyoto, Japan) equipped with a diode array detector operated at 233 nm at 29°C. Three ChromSpher 5 Lipids analytical silver-impregnated columns (250 mm×4·6 mm i.d. stainless steel; 5 μm particle size; Varian) were used in series. The mobile phase was 0·1% acetonitrile in hexane and operated isocratically at a flow rate of 1·0 ml/min. A more detailed description of the chromatographic procedures for FA identification and quantification was carried out by Gómez-Cortés et al. (Reference Gómez-Cortés, Frutos, Mantecón, Juárez, De la Fuente and Hervás2008).
In vitro ruminal fermentation
Differences between diets in rumen fermentation were studied using a modification of the gas production technique of Theodorou et al. (Reference Theodorou, Williams, Dhanoa, McAllan and France1994), adapted by Mauricio et al. (Reference Mauricio, Mould, Dhanoa, Owen, Channa and Theodorou1999).
Two rumen fluid inocula were collected, after an overnight fast, from 4 ruminally cannulated ewes that had been fed the control diet ad libitum for two weeks. Six samples (3 bottles/inoculum×2 inocula) of each diet (control and SO; ≈500 mg) were incubated in 125 ml serum bottles at 39°C with 10 ml strained rumen fluid and 40 ml phosphate-bicarbonate buffer (Goering & Van Soest, Reference Goering and Van Soest1970). The rate and extent of gas production were determined by measuring head-space gas pressure at 2, 4, 6, 9, 12, 16, 21, 27, 35, 48, 72 and 96 h post-inoculation. Pressure values were used to generate gas volume estimates using a predictive equation (Hervás et al. Reference Hervás, Frutos, Giráldez, Mora, Fernández and Mantecón2005). In vitro DM disappearance (DMD; g/kg) after 96 h incubation was estimated by filtering residues.
For each diet, 6 more samples (i.e., 3 bottles/inoculum×2 inocula) were incubated for 24 h. Immediately afterwards, the pH was measured, samples were collected for ammonia-N (NH3-N) and volatile fatty acid (VFA) analysis, and in vitro true substrate digestibility (ivTSD) was determined, as described by Frutos et al. (Reference Frutos, Hervás, Giráldez and Mantecón2004). The amount of methane (CH4) produced was calculated according to Blümmel et al. (Reference Blümmel, Makkar and Becker1997).
Calculations and statistical analyses
Gas production data from the in vitro ruminal fermentation trial were fitted to an exponential model (SAS, 1999) to provide parameters describing gas release (G) in terms of cumulative gas production (A, ml gas/g OM incubated) and fractional fermentation rate (c, /h). Average fermentation rate (AFR, ml gas/h) and extent of degradation in the rumen (ED, g/kg DM) were estimated assuming a ruminal particulate outflow (kp, /h) of 0·042.



where t is the gas reading time (h) and DMD is DM disappearance after 96 h incubation.
Data on DM intake, milk production and composition as well as milk FA composition and CLA profile were analysed by repeated measures analysis (Wang & Goonewardene, Reference Wang and Goonewardene2004), using the MIXED procedure of the Statistical Analysis System program (SAS, 1999) and assuming a compound symmetric structure on the basis of Schwarz's Bayesian Information model fit criteria. The statistical model included the fixed effects of diet (D), time (T), their interaction (D×T), and the initial record measured at 0 wk (covariate). For all data collected either individually (milk production and composition) or per lot (DM intake, FA composition and CLA profile), the lot nested within the diet was considered as a random effect and used to contrast the effect of the SO supplementation. One-way analysis of variance (SAS, 1999) was applied to the data from the in vitro trial. Least square means were reported throughout, and significance was considered at P<0·05.
Results and Discussion
Intake and milk yield and composition
Supplementation of diets with vegetable oils rich in unsaturated FA often results in a reduction of DMI (Shingfield et al. Reference Shingfield, Reynolds, Hervás, Griinari, Grandison and Beever2006), which is mainly related to potential detrimental effects on ruminal fermentation (Jenkins, Reference Jenkins1993). In the current study, however, the presence of SO in the diet had no consequences on either DM intake or milk yield (P>0·10, Table 2), which might be accounted for by the no negative effects of the SO diet on rumen processes.
Table 2. Effects of sheep diet supplementation with 60 g of sunflower oil (SO)/kg dry matter (DM) on DM intake (kg/day), milk production (g/day) and milk composition (g/100 g of raw milk)

† Refers to diets containing 0 (control) or 60 g of SO/kg DM
‡ Probability of significant effects due to diet supplementation with SO (D), time on diet (T), and their interaction (D×T)
§ SED: Standard error of the difference
The higher milk fat concentration in ewes fed the SO diet (P<0·05) is in coincidence with previous reports for dairy sheep supplemented with lipids (Caja & Bocquier, Reference Caja and Bocquier2000; Gargouri et al. Reference Gargouri, Caja, Casals and Mezghani2006; Reynolds et al. Reference Reynolds, Cannon and Loerch2006) but in contrast with studies conducted in dairy cows (Palmquist & Jenkins, Reference Palmquist and Jenkins1980; Shingfield et al. Reference Shingfield, Reynolds, Hervás, Griinari, Grandison and Beever2006). Reasons for this inconsistency are still uncertain, but they might be related to differences in lipid metabolism among ruminant species (Chilliard et al. Reference Chilliard, Ferlay, Rouel and Lamberet2003; Reynolds et al. Reference Reynolds, Cannon and Loerch2006) as discussed below.
The tendency to a lower milk protein concentration found in SO ewes (P<0·10) was in agreement with the increased milk fat concentration. It is well known that milk fat is the major energy cost of milk synthesis (Schmidt, Reference Schmidt1971). Consequently, an increase in this component would change the distribution of nutrients and would most likely reduce protein synthesis (Lock et al. Reference Lock, Teles, Perfield, Bauman and Sinclair2006). Furthermore, diets were not isoproteic.
Values of milk, milk protein and milk fat production remained relatively steady throughout the 4 weeks. On the other hand, the milk fat, protein and total solid percentages (P<0·01) decreased with time.
Fatty acid profile
Dietary supplementation with SO resulted in marked changes in milk FA profile throughout the period monitored, which were characterized by a reduction in C6:0 to C16:0 (P<0·01), at the expense of an increase in C18:0 and C18:1 isomers (Table 3). This pattern has been given for cows' and goats' milk (Chilliard & Ferlay, Reference Chilliard and Ferlay2004) and has also been reported in milk fat from ewes fed with high levels of soybean oil in diets (Mele et al. Reference Mele, Buccioni, Petacchi, Serra, Banni, Antongiovanni and Secchiari2006; Gómez-Cortés et al. Reference Gómez-Cortés, Frutos, Mantecón, Juárez, De la Fuente and Hervás2008).
Table 3. Effects of sheep diet supplementation with 60 g of sunflower oil (SO)/kg dry matter on milk fatty acid (FA) composition (g/100 g total FA)

† Refers to diets containing 0 (control) or 60 g of SO/kg DM
‡ Probability of significant effects due to diet supplementation with SO (D), time on diet (T), and their interaction (D×T)
§ SFA: saturated fatty acids; MUFA: monounsaturated fatty acids; PUFA: polyunsaturated fatty acids; CLA: conjugated linoleic acids
¶ SED: Standard error of the difference
Lipid supplementation caused nearly a four-fold enrichment in RA (from 9·4 to 36·0 mg/g total FA; P<0·01). As expected, increases in trans-11 C18:1 (vaccenic acid, VA) followed a similar pattern because VA formed by biohydrogenation in the rumen is the main precursor of RA in the mammary gland via delta-9 desaturase (Palmquist et al. Reference Palmquist, Lock, Shingfield and Bauman2005). From a nutritional point of view, most of these changes were in the direction of a healthier FA profile.
It should be stressed that the high levels of RA attained with SO were accompanied by high levels not only of VA but also of other C18:1 trans-isomers, mainly trans-10, and trans-10 cis-12, its precursor in the rumen. Remarkable increases in trans-10 C18:1 level were also reported in ewes' milk fed with diet supplemented with high levels of unprotected oil (Mele et al. Reference Mele, Buccioni, Petacchi, Serra, Banni, Antongiovanni and Secchiari2006; Reynolds et al. Reference Reynolds, Cannon and Loerch2006; Gómez-Cortés et al. Reference Gómez-Cortés, Frutos, Mantecón, Juárez, De la Fuente and Hervás2008). Increased concentration of these intermediates in milk fat is generally attributed to a shift in the biohydrogenation pathways, due to alterations in the rumen environmental conditions as a result of dietary changes (Chilliard & Ferlay, Reference Chilliard and Ferlay2004; Palmquist et al. Reference Palmquist, Lock, Shingfield and Bauman2005). Mele et al. (Reference Mele, Buccioni, Petacchi, Serra, Banni, Antongiovanni and Secchiari2006) demonstrated that a raise in the proportion of concentrate in diet generated significant increases of trans-10 C18:1 and trans-10 cis-12 C18:2 in ewes' milk fat. The significant decrease (P<0·05) in the milk secretion of most of the odd and branched FA in supplemented rations (table 3), often resulting from microbial FA metabolism in the rumen, would support the idea that the diet assayed, high in lipids and concentrate, was efficient in disturbing ruminal FA metabolism.
In comparison with previous studies on dairy cows, increases of trans-10 C18:1 and trans-10 cis-12 C18:2 in the present work did not substantially reduce RA and VA. In cow milk, VA and RA response to lipid supplementation is transient, with a maximum during the first week, immediately followed by a decrease along with an increase in milk fat trans-10 C18:1 concentration (Shingfield et al. Reference Shingfield, Reynolds, Hervás, Griinari, Grandison and Beever2006). This raises the question of the sustainability of high CLA responses in dairy ewes, but RA and VA were maintained at least for the four-week period.
Most of the CLA isomer levels rose substantially in milk fat from ewes fed with SO (Table 3). Following the same trend as most of the FA, responses to SO in the diet did not vary with time and CLA isomer composition of the control treatment milk remained constant throughout the experiment. Significant differences were found for 7-9, 8-10, 9-11 and 10-12 trans-trans and cis-trans plus trans-cis CLA (Table 3). Very recent research in milk fat from ewes fed with diet enriched with high levels of soybean oil supplies similar results (Mele et al. Reference Mele, Buccioni, Petacchi, Serra, Banni, Antongiovanni and Secchiari2006; Gómez-Cortés et al. Reference Gómez-Cortés, Frutos, Mantecón, Juárez, De la Fuente and Hervás2008) These data also coincide with others reported by Collomb et al. (Reference Collomb, Sieber and Bütikofer2004) in cow milk. These authors find significant strong positive correlations between the daily intake of linoleic acid and the concentrations of the trans-trans CLA (10-12, 9-11, 8-10 and 7-9), cis-trans and trans-cis (10-12, 9-11, 8-10 and 7-9) in milk fat from cows fed diets with high sunflower seed levels. The present research would be an evidence of the existence of similar metabolic routes in sheep.
In contrast to cows, increases in trans-10 cis-12 C18:2, trans-9 cis-11 C18:2 and trans-10 C18:1 levels as a consequence of PUFA supplementation, were not followed by a concomitant decrease in ewe milk fat (Table 3). Previous research on lactating dairy cows shows unequivocally that trans-10 cis-12 CLA exerts anti-lipogenic effects (Palmquist et al. Reference Palmquist, Lock, Shingfield and Bauman2005), and trans-9 cis-11 C18:2 reduces milk fat synthesis (Perfield et al. Reference Perfield, Lock, Griinari, Sæbo, Delmonte, Dwyer and Bauman2007). Reasons for this discrepancy between bovine and ovine responses to supplementary oils in milk fat concentration remain uncertain, but it could be attributed to the level of these isomers, which may not be enough to affect milk fat synthesis in the lactating sheep (Lock et al. Reference Lock, Teles, Perfield, Bauman and Sinclair2006). In fact, percentages of trans-10 cis-12, and trans-9 cis-11 C18:2 measured in milk fat from SO diet were lower than 0·10% (Table 3), values generally reported for these CLA isomers in cow affected by milk fat depression (Shingfield et al. Reference Shingfield, Reynolds, Hervás, Griinari, Grandison and Beever2006; Perfield et al. Reference Perfield, Lock, Griinari, Sæbo, Delmonte, Dwyer and Bauman2007). Nonetheless in other studies, despite large increases in trans-10 cis-12 C18:2 (up to 0·20%), milk fat content was not decreased by feeding unsaturated oils to ewes (Reynolds et al. Reference Reynolds, Cannon and Loerch2006). Reasons for these discrepancies are unclear, and were related to the fact that feeding rumen protected trans-10 cis-12 C18:2 (Lock et al. Reference Lock, Teles, Perfield, Bauman and Sinclair2006; Sinclair et al. Reference Sinclair, Lock, Early and Bauman2007) may have increased the absorption and produced a greater effect on the mammary gland than feeding oils. More research would be therefore needed to clarify this matter.
Ruminal fermentation
It has been long documented that lipid supplements might adversely affect the metabolism of some rumen microorganisms, thereby impairing ruminal fermentation (Harfoot & Hazelwood, Reference Harfoot, Hazlewood and Hobson1997). However, the absence of significant changes in the in vitro fermentation parameters observed in this study (Table 4) suggests that SO supplementation would hardly affect sheep ruminal fermentation.
Table 4. Effects of sheep diet supplementation with 60 g of sunflower oil (SO)/kg dry matter on ruminal fermentation: in vitro gas production parameters (A, cumulative gas production; ml/g OM and c, fractional fermentation rate; /h), average fermentation rate (AFR; ml/h), in vitro dry matter degradation (DMD; g/kg), extent of degradation (ED; g/kg), and in vitro true substrate digestibility (ivTSD; g/kg) in 96-hour incubations, and, and NH3-N concentration (mg/L), total volatile fatty acid production (VFA; mmol), molar proportions of particular VFAs (mmol/mmol) and methane production (mmol) after 24 h incubation

† Refers to diets containing 0 (control) or 60 g of SO/kg DM
‡ Probability of significant effects due to diet supplementation with SO
§ Calculated as the sum of isobutyrate, isovalerate, valerate and caproate
¶ SED: Standard error of the difference
Final pH values (ranging between 6·79 and 6·86) were not affected by SO supplementation (P>0·10), which is in agreement with findings observed by Szumacher-Strabel et al. (Reference Szumacher-Strabel, Martin, Potkanski, Cieslak and Kowalczyk2004) using batch cultures and supplementing a concentrate diet with 5% SO. There are inconsistent reports on the effects of supplementation with oils on fibre degradation, with reductions, no effects or even increases being reported (Doreau et al. Reference Doreau, Demeyer, Van Nevel, Welch, Burns, Davis, Popay and Prosser1997; Sinclair et al. Reference Sinclair, Cooper, Chikunya, Wilkinson, Hallett, Enser and Wood2005). In the present work, no differences between treatments in parameters such as DM degradation, extent of degradation, and in vitro true digestibility (P>0·10) suggest no effects on fibre degradation. Most reports on the effect of adding oil indicate an increase in propionate production at the expense of acetate; effects commonly associated with decreased rumen protozoa (Doreau et al. Reference Doreau, Demeyer, Van Nevel, Welch, Burns, Davis, Popay and Prosser1997) and fibre degradation (Jalč et al. Reference Jalč, Kišidayova and Nerud2007). No reduction of fibre degradation in the SO diet would explain the lack of differences in these VFAs. In accordance with these data, methanogenesis was not affected either. Concerning rumen ammonia concentration, data in the literature show that lipid supplementation commonly results in no variation in this parameter (Doreau & Ferlay, Reference Doreau and Ferlay1995, Szumacher-Strabel et al. Reference Szumacher-Strabel, Martin, Potkanski, Cieslak and Kowalczyk2004), as observed in the present trial. It is worth mentioning that the interest of using rumen inoculum from ewes fed the control diet is that differences in ruminal fermentation can be directly attributed to the effect of adding SO, but different results might have been obtained if the inoculum would have been withdrawn from SO supplemented ewes.
Altogether, these results confirm that dairy sheep milk RA concentration could be substantially increased by adding SO to the diet, with noticeable changes in milk FA composition and with no negative effects on ruminal fermentation and animal performance.
This work was supported by the Ministerio de Educación y Ciencia (Project AGL2005-04760 and Consolider CSD2007-063) and the Comunidad Autónoma de Madrid (Project S-0505/AGR/000153). The authors wish to thank J. López and M. V. Rodríguez-Pino for their skilled technical assistance.