Susceptibility of the bovine mammary gland to new intramammary infections (IMI) is markedly increased during early involution and the periparturient period (Oliver & Sordillo, Reference Oliver and Sordillo1988). Although not fully understood, increased susceptibility to IMI has been related to changes during the involution process that may facilitate bacterial penetration of the streak canal, interfere with natural defence mechanisms and enhance bacterial growth (Oliver & Sordillo, Reference Oliver and Sordillo1988). Since fully involuted bovine mammary glands are markedly resistant to IMI (Oliver & Sordillo, Reference Oliver and Sordillo1988) it has been proposed that hastening mammary gland involution while increasing natural protective mechanisms during the early nonlactating period could favour prevention or treatment of IMI during the involution process (Oliver & Smith, Reference Oliver and Smith1982a).
An improved understanding of components implicated in the immunological response of the mammary gland has led researchers to address alternative approaches to classical mastitis control measures based on hygiene and antibiotic therapy, such as manipulation of local immune responses to mastitis pathogens. Among these, biological response modifiers (BRM) or immunomodulators are compounds capable of interacting with the immune system to regulate specific aspects of the host response. The type of activity of these compounds depends on their mechanism and site of action, dose, route and timing of administration (Tzianabos, Reference Tzianabos2000). BRM have been used in an attempt to enhance non-specific immune mechanisms against bovine mastitis pathogens (Zecconi, Reference Zecconi and Zecconi2000; Takahashi et al. Reference Takahashi, Odai, Mitani, Inumaru, Arai, Horino and Yokomizo2004). However, the exact mechanism of action and effect of compounds used on the bovine mammary tissue are not fully understood. Studies in cows showed that intramammary inoculation of Escherichia coli lipopolysaccharide (LPS) at drying off resulted in acceleration of mammary gland involution (Oliver & Smith, Reference Oliver and Smith1982a). In addition, intramammary infusion of LPS (Oliver & Smith, Reference Oliver and Smith1982b) and a LPS-based BRM (Dallard et al. Reference Dallard, Ruffino, Heffel and Calvinho2007) at drying-off transiently reduced the isolation of mastitis pathogens from mammary glands during the nonlactating period. LPS is a complex glycolipid with different biological effects that include non-specific activation of the immune system and induction of endotoxin-shock syndrome (Van Miert, Reference Van Miert1991).
Mammary gland remodelling relies on a dynamic equilibrium between two opposite processes: mitosis and apoptosis. The latter is responsible for the loss of cells during mammary involution after natural weaning or litter removal in rodents (Quarrie et al. Reference Quarrie, Addey and Wilde1996) and during drying-off in ruminants (Quarrie et al. Reference Quarrie, Addey and Wilde1994; Wilde et al. Reference Wilde, Addei, Li and Fenig1997). Biochemically, apoptosis is characterized by the activation of caspases, highly specific proteinases that cleave a wide array of intracellular substrates (Thornberry & Lazebnik, Reference Thornberry and Lazebnik1998). The variations in the expression of pro- and anti-apoptotic proteins network in the bovine mammary gland have not yet been fully explored, and the exact role of apoptosis-regulating genes during mammary gland infection is not entirely clear (Sheffield, Reference Sheffield1997). In addition, little has been reported concerning the signalling events involved in mammary cell apoptosis in vitro following Staphylococcus aureus infection (Wesson et al. Reference Wesson, Deringer, Liou, Bayles, Bohach and Trumble2000) and there is no information on cell death mechanisms in chronically Staph. aureus-infected mammary glands during involution.
The objective of this study was to describe the effects of a single intramammary infusion of a LPS-based BRM on death mechanism in uninfected and Staph. aureus-infected bovine mammary glands during involution.
Materials and Methods
Biological response modifier
The product contained LPS of Esch. coli strain (LN02) at a concentration of 0·35 μm and 4·5 mg of membranous and ribosomal fractions of the same strain incorporated into liposomes contained in 10 ml of aqueous-based vehicle (Laboratorio Neomar, Buenos Aires, Argentina). Liposomes (The Liposystem Complex®, I.R.A., Milan, Italy) composition was 40% phospholipids and 60% hydrophilic medium and active principle. Concentration of LPS was determined by quantification of 2-keto-3-deoxyoctonate by a colorimetric method (Osborn, Reference Osborn1963). The BRM dose used in the present study was selected based on a previous dose-response trial. This dose proved to be safe for cows, causing only mild inflammation and no systemic adverse effects (Calvinho et al. Reference Calvinho, Ortega, Dallard, Iguzquiza, Salvetti and Quaino2004).
Animals
The study used Holstein non-pregnant cows in late lactation (weeks 31–36) from the Rafaela Experiment Station of INTA herd. Cows used in this study were from parity 3 to 5, milked twice daily and produced an average of 8 kg milk/d before interruption of lactation. Based on previous bacteriological studies and somatic cell counts (SCC), animals were either uninfected or infected with Staph. aureus. Infections were naturally acquired either in the previous dry period or during the first 2 months of the lactation preceding initiation of the study. Cows with similar lactation number were included in each group.
Experimental design
The infection status of mammary quarters was determined within 6 months before initiation of the experiment and confirmed 20 d and 3 d before inoculation. A quarter was considered to be infected if Staph. aureus was isolated from both samples. Infected quarters were randomly selected from cows showing at least two quarters infected with Staph. aureus. Only animals with subclinical IMI at the time of milking interruption were included. From these cows, only two infected quarters were infused either with BRM or placebo (vehicle alone). Uninfected quarters were selected from cows free of infection at the time of sampling. Only two quarters from each cow were infused either with BRM or placebo. Cows were slaughtered at 7, 14 and 21 d after infusion. Uninfected (n=6) and Staph. aureus-infected (n=6) mammary quarters were included in each group (7, 14 and 21 d). In all cases milking was interrupted after intramammary infusion and cows were fed only alfalfa hay and had free access to water for the remainder of the experiment. Mammary secretion samples were aseptically collected for bacteriological analysis using standard procedures (Hogan et al. Reference Hogan, Harmon, González, Nickerson, Oliver, Pankey and Smith1999) 3 d before BRM administration, immediately before inoculation and every 48 h after infusion. Animals included in the three groups were slaughtered at 7, 14 and 21 d after inoculation at a local abattoir and samples for histological analysis were taken. According to the eligibility criterion used, from 44 cows initially available, 36 were finally included in the study.
Bacteriological examination
Mammary secretion samples (10 μl) were streaked onto blood agar plates supplemented with 5% bovine blood and incubated for 48 h aerobically at 37°C. Plates were examined for bacterial growth at 24 h and 48 h. Isolated colonies were identified according to standard procedures (Hogan et al. Reference Hogan, Harmon, González, Nickerson, Oliver, Pankey and Smith1999). Presence of one colony of Staph. aureus on blood agar was considered a positive identification.
Tissue sample preparation
Immediately after cows were slaughtered tissue samples were taken from the dorso-lateral portion of the gland (deep parenchyma) at a depth of 4 cm following previous descriptions (Nickerson et al. Reference Nickerson, Owens, Boddie and Boddie1992). Tissue samples of approximately 1 cm3 were fixed in 10% neutral buffered formalin washed in phosphate-buffered saline (PBS, pH 7·4) and processed as described by Ortega et al. (Reference Ortega, Salvetti, Amable, Dallard, Baravalle, Barbeito and Gimeno2007). Serial 5-μm sections were mounted on glass previously treated with 3-aminopropyltriethoxysilane (Sigma-Aldrich, St. Louis MO, USA) and stained with haematoxylin–eosin for a preliminary observation.
Immunohistochemistry
A streptavidin-biotin immunoperoxidase method was performed as described by Dallard et al. (Reference Dallard, Ruffino, Heffel and Calvinho2007). Briefly, sections were deparaffinized, hydrated and microwave pre-treated (antigen retrieval). The endogen peroxidase activity was inhibited with 1% H2O2 and non-specific binding was blocked with 10% normal goat serum. Sections were incubated overnight at 4°C with rabbit polyclonal antiserum against Bax protein (BioGenex, San Ramon CA, USA), active caspase-3 polyclonal antibody (R&D Systems, Minneapolis MN, USA) and Ki-67 monoclonal antibody (BioGenex). Slides were washed with PBS and incubated for 30 min at room temperature with rat preabsorbed biotinylated secondary antibodies selected specifically against one of each of the two types of primary antibodies used (monoclonal or polyclonal). Visualization of antigens was by the streptavidin-peroxidase method (BioGenex) and 3.3-diaminobenzidine (Liquid DAB-Plus Substrate Kit-Zymed, San Francisco CA, USA) was used as chromogen. Finally, the slides were washed in distilled water and counterstained with Mayer's haematoxylin, dehydrated and mounted. To verify specificity, adjacent control sections were subjected to the same immunohistochemical method replacing primary antibodies by rabbit and mouse non-immune serum. Each primary antibody was probed with an absorption test involving the respective antigen (15 μg/ml) (Sigma-Aldrich).
TUNEL assay
Detection of apoptotic cells in situ was performed using a commercial TUNEL kit (ApopTag Plus Peroxidase, Chemicon International, Temecula CA, USA) following manufacturer's directions. Briefly, after deparaffinization and hydration, slides were incubated with proteinase K for 15 min at 25°C. Tissue sections were quenched in 3% H2O2 in PBS, incubated in equilibration buffer for 10 min, and incubated with terminal deoxynucleotidyl transferase (TdT) for 60 min at 37°C. Sections were washed with stop wash buffer for 10 min and then incubated with antidigoxigenin-peroxidase for 30 min at 25°C. Tissue sections were washed in PBS and then incubated with diaminobenzidine for approximately 6 min at room temperature for colour development. Sections were washed in distilled water, counterstained with Mayer's haematoxylin, dehydrated and mounted. For every four slides processed, one negative control was performed without active TdT but including proteinase K digestion to control for non-specific nucleotide incorporation or for non-specific enzyme conjugate binding. Tissue sections were viewed by light microscopy with an Olympus BH-2 light microscope (Olympus Co., Japan) to quantify labelled cells. All cells in each field at 40×magnification were counted (at least 1500 cells) using Image Pro-Plus 3.0.1® (Media Cybernetics, Silver Spring MA, USA). Cells were classified as epithelial, stromal, labelled epithelial or labelled stromal cells. Cells were counted as epithelial if they were part of the secretory tissue, which included epithelial and myoepithelial cells, and possibly leucocytes if they were present in the alveolar lumen. All cells of the connective tissue were classified as stromal cells, including fibroblasts, adipocytes and endothelial cells. Labelled brown nuclei were readily visible and a cell was classified as labelled when the nuclear staining was at least twice as intense as the background. Quantification of epithelial and stromal cells labelled with anti-Bax protein and active caspase-3 was performed in a similar manner as for TUNEL.
Cellular proliferation
Nuclei of proliferating cells were labelled with anti-Ki-67 monoclonal antibody similar to that described for Bax protein and active caspase-3. All immunostained mammary cells showing a characteristic nuclear staining pattern were considered positive. A minimum of 1000 cells in at least 20 microscopic fields at 40×magnification were counted in each slide. Epithelial and stromal cells showing an intense nuclear staining were quantified and results were expressed as a percentage of immunopositive cells.
Ratio of apoptotic to proliferating cells
Balance between dying and proliferating cells was estimated using the ratio of apoptosis to cell proliferation (A/P). The mean ratio of the number of TUNEL- or Ki-67-positive cells to total cell number in each set of tissue sections was calculated following previous descriptions (Colitti et al. Reference Colitti, Wilde and Stefanon2004).
Statistical analysis
A three-way factorial model with fixed factors for treatment (BRM and placebo), infection status (Staph. aureus-infected and uninfected) and days of involution (7, 14 and 21 d) was applied. A Proc GLM was used and means were compared by Duncan test (SAS, 1999).
Results
Evaluation of apoptosis
Bax immunostaining:
Results of BRM treatment effect on percent of Bax immunostained epithelial and stromal cells in mammary tissue of uninfected and Staph. aureus-infected quarters during involution is shown in Table 1. Regarding epithelial cells immunostaining, interactions among the three factors were detected. Analysis of interactions in uninfected quarters showed no differences in percent immunostained cells between BRM and placebo-treated glands at every observation period.
Table 1. Effect of treatment with a biological response modifier (BRM) on percent of active caspase-3 and Bax immunostained cells in mammary tissue of uninfected and Staph. aureus-infected quarters during involution. Values represent means of percent epithelial and stromal stained cells±sem

In Staph. aureus-infected quarters, the BRM treatment produced a significant increase in percent of stained epithelial cells for Bax at every observation period compared with placebo-treated quarters (P<0·05).
No interactions among the three factors were detected for stromal cells. A significant increase of immunostained stromal cells for Bax was observed in BRM-treated compared with placebo-treated quarters (P<0·05). Infected quarters showed a significant increase of immunostained cells compared with uninfected quarters (P<0·05), and a significant increase of immunostained cells was observed at 14 and 21 d (Fig. 1A) compared with 7 d of involution (P<0·05).
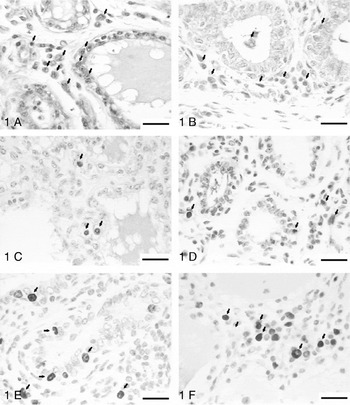
Fig. 1A. Immunohistochemical identification of Bax. Bovine mammary gland infected with Staph. aureus, treated with biological response modifier at 21 d of drying off. Arrows indicate epithelial and stromal cells Bax immunostaining.Fig. 1B. Immunohistochemical identification of active caspase-3 in bovine mammary gland infected with Staph. aureus, treated with biological response modifier at 7 d of drying off. Arrows indicate epithelial and stromal cells active caspase-3 immunostaining.Fig. 1C. Apoptosis detection using TUNEL assay. Bovine mammary gland infected with Staph. aureus, treated with placebo at 14 d of drying off. Arrows indicate TUNEL-positive cells immunostaining in mammary gland epithelium and stroma.Fig. 1D. Apoptosis detection using TUNEL assay. Bovine mammary gland infected with Staph. aureus, treated with placebo at 21 d of drying off. Arrows indicate TUNEL-positive cells in mammary gland epithelium and stroma.Figs 1E and 1F. Immunohistochemical identification of Ki-67 in bovine mammary gland infected with Staph. aureus, treated with biological response modifier at 7 d of drying off. Arrows indicate strong staining in the nucleus of epithelial and stromal cells. Scale bars represent 25 μm.
Active caspase-3 immunostaining:
Results of BRM treatment effect on percent of active caspase-3 immunostained epithelial and stromal cells in mammary tissue of uninfected and Staph. aureus-infected quarters during involution is shown in Table 1. No interactions between the three factors were detected for epithelial cells. Interactions between treatment and days of involution were detected (P<0·05). A significant increase of immunostained epithelial cells was observed in BRM-treated compared with placebo-treated at 7 d and 14 d of involution (P<0·05) (Fig. 1B). Interactions between infection status and treatment were detected (P<0·05). A significant increase of immunostained epithelial cells was observed in Staph. aureus-infected compared with uninfected quarters at 7 d and 14 d of involution (P<0·05).
No interactions between the three factors were detected for stromal cells. Interactions between treatment and week of involution were observed (P<0·05). A significant increase of immunostained stromal cells was observed in BRM-treated compared with placebo-treated quarters at every observation period (P<0·05). Interactions between infection status and week of involution were detected (P<0·05). A significant increase of immunostained stromal cells was observed in Staph. aureus-infected compared with uninfected quarters at 7 d and 21 d of involution.
Detection of apoptosis in situ (TUNEL)
Results of BRM treatment effect on percent apoptotic epithelial and stromal cells in mammary tissue of uninfected and Staph. aureus-infected quarters during involution is shown in Table 2.
Table 2. Effect of treatment with a biological response modifier (BRM) on percent of apoptotic cells and Ki-67 immunostaining, and the ratio of apoptotic to proliferating cells (A/P ratio) in uninfected and Staph. aureus-infected mammary quarters during involution. Values represent means of percent epithelial and stromal stained cells±sem

No interactions between the three factors were detected for epithelial cells. Interactions between treatment and week of involution were detected (P<0·05). A significant increase of immunostained epithelial cells was observed in BRM-treated compared with placebo-treated quarters at 7 d and 21 d of involution (P<0·05). Interactions between infection status and week of involution were detected (P<0·05). A significant increase of immunostained epithelial cells was observed in Staph. aureus-infected compared with uninfected quarters at 7 d and 21 d of involution. Interactions between treatment and infection status were detected (P<0·05). A significant increase of immunostained epithelial cells was observed in Staph. aureus-infected quarters treated with BRM compared with uninfected BRM-treated quarters (P<0·05) (Figs 1C and 1D).
Regarding stromal cells immunostaining, interactions among the three factors were detected. Analysis of interactions showed significant differences in percent immunostained cells between BRM and placebo-treated glands at every observation period (P<0·05). In addition, a significant increase of stromal cells immunostaining was observed in Staph. aureus-infected compared with uninfected quarters at every observation period (P<0·05).
Cellular proliferation
Proliferation of epithelial and stromal cells was evaluated by measuring expression of Ki-67 antigen and is summarized in Table 2. Regarding epithelial cells immunostaining, no interactions among the three factors were detected. Interactions between infection status and week of involution were detected (P<0·05). A significant increase of epithelial cells immunostaining was observed in Staph. aureus-infected compared with uninfected quarters at every observation period (P<0·05) (Fig. 1E).
Regarding stromal cells immunostaining, no interactions among the three factors were detected. Interactions between infection status and week of involution were detected (P<0·05). A significant increase of stromal cells immunostaining was observed in Staph. aureus-infected compared with uninfected quarters at every observation period (P<0·05) (Fig. 1F).
Ratio of apoptotic to proliferating cells
Ratio of apoptotic to proliferating cells is shown in Table 2. Regarding epithelial cells A/P ratio, no interactions among the three factors were detected. Interactions between infection status and week of involution were detected (P<0·05). A significant increase of A/P ratio was observed in Staph. aureus-infected compared with uninfected quarters at 7 d and 14 d of involution (P<0·05). Interactions between treatment and infection status were detected (P<0·05). Uninfected placebo-treated quarters showed a significant increase of A/P ratio compared with uninfected BRM-treated quarters (P<0·05), while no differences between treatments were detected in Staph. aureus-infected quarters.
Regarding stromal cells A/P ratio, no interactions among the three factors were detected. A significant increase of A/P ratio was observed at 14 d and 21 d compared with 7 d of involution (P<0·05). In addition, a significant increase of A/P ratio was observed in Staph. aureus-infected compared with uninfected quarters (P<0·05) and in BRM-treated compared with placebo-treated quarters (P<0·05).
Discussion
Apoptosis is responsible for the loss of cells during mammary involution after natural weaning or litter removal in rodents (Quarrie et al. Reference Quarrie, Addey and Wilde1996) and during drying off in ruminants (Quarrie et al. Reference Quarrie, Addey and Wilde1994; Wilde et al. Reference Wilde, Addei, Li and Fenig1997). The Bcl-2 family of genes encodes for proteins that are key regulators of apoptosis (Schorr et al. Reference Schorr, Li, Krajewski, Reed and Furth1999; Reed, Reference Reed2000) and possesses either apoptotic or anti-apoptotic (survival)-promoting activity. The mechanisms through which these proteins, that include anti-apoptotic Bcl-2 and Bcl-xl and pro-apoptotic Bax and Bcl-xs proteins, regulate apoptosis are not completely defined (Schorr et al. Reference Schorr, Li, Krajewski, Reed and Furth1999) but involve changes in the permeability of the mitochondrial membrane. Bcl-2 resides in the outer membrane, which is the site at which Bax and Bcl-2 can homo- or hetero-dimerize via the BH3 domain (Mahajan et al. Reference Mahajan, Linder, Berry, Gordon, Heim and Herman1998). Bcl-2 members contain a pore-forming domain (Reed, Reference Reed1997) which could control the formation of permeability transition pores in the mitochondrial membrane. Both Bax and Bcl-xs have been implicated in promoting involution of mammary tissue (Capuco et al. Reference Capuco, Li, Long, Ren, Hruska, Schorr and Furth2002). Mouse mammary gland involution is associated with a high level of Bax and a decrease in anti apoptotic Bcl-2 protein (Merlo et al. Reference Merlo, Graus-Porta, Cella, Marte, Taverna and Hynes1996). Bax and Bcl-xs mRNA levels in mammary epithelial cells increase within the first day after weaning, coinciding with the induction of apoptosis (Heermeier et al. Reference Heermeier, Benedict, Li, Furth, Nunez and Hennighausen1996). Studies in goat mammary glands during lactation and involution revealed that Bax expression was lowest at lactation peak, significantly increased in late lactation and remained elevated during involution (Wareski et al. Reference Wareski, Motyl, Ryniewicz, Orzechowski, Gajkowska, Wojewodzka and Ploszaj2001). In addition, increase in Bax expression during secretory tissue involution in sows has also been shown (Motyl et al. Reference Motyl, Gajkowska, Wojewodzka, Wareski, Rekiel and Ploszaj2001). In accord with these findings, in the present study immunostaining for Bax was seen in the alveolar epithelium and ducts at every sampling period and a significant increase of stromal immunostaining for Bax was observed at 14 d and 21 d compared with 7 d of involution.
Long et al. (Reference Long, Capuco, Wood, Sonstegard, Tomita, Paape and Zhao2001) evaluated the relationship between mastitis and mammary cells in vivo after injection of Esch. coli into mammary gland in lactating Holstein cows. Infection elicited an increased expression of pro-apoptotic genes (Bax and interleukin-1β converting enzyme) whereas expression of the anti-apoptotic gene (Bcl-2) was decreased. In this study, the percentage of epithelial and stromal cells stained for Bax were significantly higher in Staph. aureus-infected than uninfected quarters. In addition, BRM treatment in infected quarters induced a significant increment in percentages of epithelial cells immunostained with Bax at the three involution stages studied and in A/P ratio of stromal cells. Infection with Gram-positive bacteria similarly appears to induce apoptosis. Streptococcus agalactiae-induced bovine mastitis increased the expression of an apoptosis marker, RTPM-2 (Sheffield, Reference Sheffield1997), and Staph. aureus was shown to induce apoptosis of MAC-T cells, a bovine mammary epithelial cell line (Wesson et al. Reference Wesson, Deringer, Liou, Bayles, Bohach and Trumble2000).
Bax accumulation on organelle membranes precedes the activation of caspase-3, an apoptosis executor (Zhang et al. Reference Zhang, Heim and Meyhack1998). Immunohistochemical labelling of CPP-32 (caspase-3) in goat mammary glands revealed a significant increase of this enzyme content concurrent with a degree of mammary tissue involution, suggesting the involvement of caspase-dependent pathways in the execution of apoptosis in this tissue (Wareski et al. Reference Wareski, Motyl, Ryniewicz, Orzechowski, Gajkowska, Wojewodzka and Ploszaj2001). In addition, the induction of CPP-32 (caspase-3) from lactation peak to dry period was accompanied by a progressive loss of mammary epithelial cells and the increase in apoptotic cell numbers (Wareski et al. Reference Wareski, Motyl, Ryniewicz, Orzechowski, Gajkowska, Wojewodzka and Ploszaj2001). In the present study, BRM treatment induced a significant increment in the percentage of stromal cells labelling with anti-active caspase-3 antibody at every observation period and of epithelial cells at 7 d and 14 d of involution. In addition, in stromal cells, BRM treatment induced a significant increase in percentages of TUNEL-positive immunostaining at every observation period suggesting that BRM treatment promoted programmed cell death of mammary stroma by the caspase-3-dependent pathway. BRM treatment induced a significant increase of TUNEL-positive epithelial cells at 7 d and 21 d of involution compared with placebo-treated quarters. However, uninfected BRM-treated quarters showed a decreased epithelial cell A/P ratio compared with placebo-treated quarters, suggesting a simultaneous effect promoting cell proliferation. Taken together, these results show an effect of BRM in mammary cell remodelling during involution.
The mechanism by which Staph. aureus induces apoptosis in bovine mammary epithelial cells in vitro involves caspase-8 and caspase-3, two key enzymes of a proteolytic cascade leading to apoptosis (Wesson et al. Reference Wesson, Deringer, Liou, Bayles, Bohach and Trumble2000). In this study, a significant increase of TUNEL positive epithelial cells was observed in Staph. aureus-infected compared with uninfected quarters at 7 d and 21 d of involution and of TUNEL positive stromal cells at every observation period. These results are in accordance with previous studies in vitro (Wesson et al. Reference Wesson, Deringer, Liou, Bayles, Bohach and Trumble2000) and corroborate the role of caspase-3 in bovine mammary cells apoptosis induced during Staph. aureus infection. In addition, a significant increase in percentages of epithelial cells staining with active caspase-3 was observed in BRM-treated Staph. aureus-infected quarters compared with uninfected quarters at 7 d and 14 d of involution, resulting in an apoptosis increase of epithelial cells through mechanisms involving caspase-3 activation.
In Staph. aureus-infected quarters, apoptosis of epithelial and stromal cells was significantly greater than in uninfected controls either at most or all observation periods considered. These results agree with those obtained by Long et al. (Reference Long, Capuco, Wood, Sonstegard, Tomita, Paape and Zhao2001) in Esch. coli-induced mastitis, where the apoptosis index was significantly higher in mastitic tissue compared with uninfected controls. In the former study, infection elicited increases in Ki-67 labelling index and expression of pro-apoptotic gene (Bax), whereas expression of anti-apoptotic gene (Bcl-2) was decreased. In the present investigation, a high percentage of staining for Ki-67 in chronically infected quarters at every observation period, suggests that increased cell proliferation during chronic Staph. aureus mastitis may serve as a mechanism to ameliorate tissue damage. In infected quarters, BRM treatment induced an increase in percentages of epithelial cells TUNEL-positive compared with uninfected BRM-treated quarters. Similarly, BRM treatment caused a significant increase of percent apoptotic epithelial cells at 7 d and 21 d of involution and of stromal cells at every observation period, compared with placebo-treated quarters, suggesting that BRM can enhance mammary gland remodelling during involution.
Mammary gland remodelling depends on a dynamic equilibrium between two opposite processes: mitosis and apoptosis. In the present study, placebo-treated uninfected quarters showed greater percentages of proliferating epithelial than stromal cells, as indicated by Ki-67 immunostained cells at every observation period during involution. In a recent study, using proliferation cell nuclear antigen (PCNA) as cellular proliferation marker, we observed that PCNA expression in uninfected controls increased as involution progressed (Dallard et al. Reference Dallard, Ruffino, Heffel and Calvinho2007). In addition, treatment of uninfected quarters with BRM produced a transient increase in PCNA expression at 7 d of mammary involution compared with placebo-treated quarters. Although a similar trend was found in the present study, no interactions between treatment and week of involution for Ki-67 antigen expression were detected and lower percentages of immunostained cells were observed with anti-K-67. Discrepancy in results of both studies may derive from differences between proliferation markers. PCNA is a highly conserved 36-kd auxiliary protein of the DNA polymerase-δ with a half life of up to 20 h (Löhr et al. Reference Löhr, Teifke, Failing and Weiss1997). The evidence that PCNA expression is possibly stimulated by cytokines through stabilization of the PCNA mRNA without having to induce DNA synthesis (Hall et al. Reference Hall, Coates, Ansari and Hopwood1994) and repair activity during G0-phase may lead to a false positive staining for PCNA of cells not belonging to the S-phase or even the growth fraction. This could explain the higher values for the growth fraction encountered with PCNA in contrast with Ki-67.
Ki-67 is expressed 24–36 h after cell stimulation in the G1-phase and remains immunohistologically detectable throughout the interphase of the cell cycle, reaching its maximal level during mitosis (du Manoir et al. Reference du Manoir, Guillaud, Camus, Seigneurin and Brugal1991). Immediately after mitosis, the cellular Ki-67 antigen content decreases to a minimum due to the short half life of <1 h, not being detectable anymore in G0-phase (Gerdes et al. Reference Gerdes, Lemke, Baisch, Wacker, Schwab and Stein1984). Therefore, immunohistology with antibodies against Ki-67 traces specifically proliferating, but not resting cells. In the present study, although a transient increment in Ki-67 expression was observed both in epithelial and stromal cells of uninfected BRM-treated quarters, differences with placebo treated quarters were not significant. However, it has to be taken into account that epithelial cells in uninfected BRM-treated quarters showed a lower A/P ratio than placebo-treated quarters, indicating increased cell proliferation as a result of BRM administration and confirming that BRM treatment did not interfere with mammary cell proliferation as was previously reported (Dallard et al. Reference Dallard, Ruffino, Heffel and Calvinho2007).
In conclusion, BRM treatment in uninfected mammary glands increased apoptogenic proteinases staining and apoptosis of both epithelial and stromal mammary cells. Apoptosis was markedly increased in Staph. aureus chronically infected quarters. BRM treatment in Staph. aureus-infected mammary glands induced an increase in pro-apoptotic proteins (Bax), apoptogenic proteinases and apoptosis, without inhibiting mammary cell proliferation.
The authors express their appreciation to O. Warnke, M. Marín, V. Canavesio and V. Neder for technical assistance; to O. Quaino for assistance with statistical analysis and to R. Almeida for critical manuscript review. This work was supported by CAI+D (Universidad Nacional del Litoral) and Fundación ArgenINTA.