INTRODUCTION
The marked deficit of home-produced high-protein feedstuff and the increasing attention paid to various aspects of sustainable agriculture (soil fertility, diversity of cultivated crops, symbiotic nitrogen fixation, and reduction of greenhouse gas emissions) emphasize the interest of pulse crops such as pea (Pisum sativum L.), faba bean (Vicia faba L.) and white lupin (Lupinus albus L.) for European agriculture. The shift from spring to autumn sowing can raise the yield and the yield stability of these crops, exploiting two potential advantages: (i) increased crop yield potential, through the longer crop cycle; and (ii) lower extent of terminal drought and heat stresses, through earlier crop maturity (Lawes et al. Reference Lawes, Bond, Poulsen and Hebblethwaite1983; Lejeune-Hénaut & Wery Reference Lejeune-Hénaut, Wery, Ney, Duchêne, Carrouée and Angevin1994; Papineau & Huyghe Reference Papineau and Huyghe2004). However, this strategy requires enhancement of crop winter hardiness, which is determined by both genetic and management factors. The optimal autumn sowing date is site-specific and, to some extent, year-specific depending on the temperature pattern, because greater plant mortality in winter may arise from floral initiation caused by sowing too early, or from insufficiently thick root parenchyma caused by sowing too late (Etévé Reference Etévé, Hebblethwaite, Heath and Dawkins1985; Huyghe & Papineau Reference Huyghe and Papineau1990). In addition, frost tolerance is markedly affected by the degree of frost-hardening (cold-acclimation) of the plant (Herzog Reference Herzog1989; Lejeune-Hénaut & Wery Reference Lejeune-Hénaut, Wery, Ney, Duchêne, Carrouée and Angevin1994; Papineau & Huyghe Reference Papineau and Huyghe2004). Hardening, which involves various physiological mechanisms that lower the extent and the effects of ice crystal formation in the cells (McKersie & Leshem Reference McKersie and Leshem1994; Bourion et al. Reference Bourion, Lejeune-Hènaut, Munier-Jolain and Salon2003), requires a period of low non-freezing temperatures; it may be reversed by mild winter temperatures and is less pronounced in aged plants or after a pre-hardening period characterized by mild temperatures (Herzog Reference Herzog1989; Wery et al. Reference Wery, Turc, Lecoeur, Singh and Saxena1993). Plant mortality may be greater in mild winter environments, characterized by frost events of limited duration and severity, than in cold winter environments with long, severe frosts (Etévé Reference Etévé, Hebblethwaite, Heath and Dawkins1985), because of the slower and lower hardening and the possible dehardening of the plants. The wide temperature variation across the cool season that characterizes the Mediterranean climate makes winter hardiness a key trait for variety adaptation to Italian environments (Ranalli Reference Ranalli2001; Monotti et al. Reference Monotti, Stagnari, Conti, Petrini, Cappelli, Cocchiarella, Raggi, Gargano, Orfei and Quattrucci2004).
Winter hardiness of genotypes is mainly related to the intrinsic frost tolerance of the hardened plant and to delayed floral initiation (Herzog Reference Herzog1988; Huyghe & Papineau Reference Huyghe and Papineau1990; Lejeune-Hénaut & Wery Reference Lejeune-Hénaut, Wery, Ney, Duchêne, Carrouée and Angevin1994). Breeding crops for southern Europe is complicated by the fact that delayed flowering, which depends mainly on vernalization requirement in white lupin (Huyghe Reference Huyghe1997), on heat unit requirement and photoperiodic response in pea (Lejeune-Hénaut et al. Reference Lejeune-Hénaut, Bourion, Etévé, Cunot, Delhaye and Desmyter1999) and on all of these mechanisms in faba bean (McDonald et al. Reference McDonald, Adisarwanto and Knight1994), may imply greater susceptibility to terminal drought and heat stress. In addition, the genotype ranking for winter hardiness is affected by hardening and dehardening dynamics, and artificial freezing tests may fail to predict the field responses of cultivars which have not completed the hardening process well (Herzog Reference Herzog1988). While relying largely on field testing (also in view of its lower costs), the breeding for winter hardiness in southern Europe requires information on the consistency of genotype responses across contrasting environments, the impact of frost tolerance on the grain yield, and the value of simple morphophysiological traits as indirect selection criteria.
In the present study, a number of geographically diverse cultivars of faba bean (mostly released less than 20 years ago), pea and lupin (mostly released less than 10 years ago) were evaluated across climatically contrasting Italian locations and sowing times. The objectives were to assess the winter survival of species and varieties and to investigate, for each species: (i) the consistency of cultivar winter hardiness across locations and sowing times; (ii) the relationship of cultivar winter survival with grain yield and various morphophysiological traits; and (iii) the efficiency of indirect selection for winter survival across environments based on morphophysiological traits relative to direct selection for the trait.
MATERIALS AND METHODS
Experimental data
The study included 49 cultivars of pea, 24 of faba bean and 11 of white lupin, released by 29 private or public breeding institutions which provided recent varieties with actual or putative good adaptation to Italian cropping environments. The cultivars, listed in Table 1, could be classified as follows with respect to their geographic origin: 12 cultivars of pea, 15 of faba bean and three of lupin from southern Europe (Italy and Spain); 30 cultivars of pea, eight of faba bean and seven of lupin from western or central Europe (France, Germany, Denmark, UK and The Netherlands); two cultivars of pea and one of lupin from eastern Europe; five pea cultivars from Australia; and one faba bean entry from the International Center for Agricultural Research in the Dry Areas (ICARDA, Syria). In terms of major plant architecture (Davies Reference Davies, Casey and Davies1993; Huyghe Reference Huyghe1997), there were: (i) 39 semi-leafless and ten leafed pea genotypes; (ii) two dwarf, two determinate, one dwarf-determinate and six tall-indeterminate lupin cultivars. The faba beans were indeterminate types with large (weight >1·0 g), medium, or small seed (weight <0·7 g). Each of the three phenological types described by Stoddard et al. (Reference Stoddard, Balko, Erskine, Khan, Link and Sarker2006) for cool-season grain legumes, viz. spring, Mediterranean and winter types, was represented by some cultivars within each species.
Table 1. Name and geographic origin of the cultivars evaluated
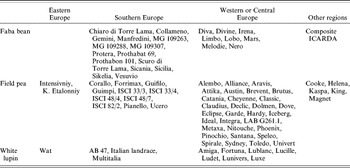
There were two evaluation sites. Lodi (Lombardy) has a sub-continental climate with extended frosts in winter and modest terminal drought and heat stress which is typical of the Po Valley. Foggia (Apulia) is a representative of the Mediterranean climate with short frost spells within mild winters and severe terminal drought and heat stress, typical of eastern and inland areas of central and southern Italy (Perini et al. Reference Perini, Beltrano, Dal Monte, Esposito, Caruso, Motisi and Marra2004). The soil lime content was negligible in Lodi and moderate in Foggia (47 g/kg, which is above the threshold of 25 g/kg indicated for the possible yield reduction in a lime-susceptible species such as white lupin: UNIP-ITCF 2002a). The evaluation included two sowing dates, i.e. 23 October and 7 November in Lodi and 7 and 21 November in Foggia, which represented an early and late autumn sowing in each site according to Ranalli (Reference Ranalli2001). Using more sowing dates increases the possibility of observing frost injuries in areas with fairly unpredictable temperature patterns (Etévé Reference Etévé, Hebblethwaite, Heath and Dawkins1985).
The experimental design combined features of strip-plot and group-block (Gomez & Gomez Reference Gomez and Gomez1984). The two sowing times were assigned to main plots and the cultivars grouped for species to subplots, using three replications per treatment. Pea and faba bean seeds were treated with Wakil (50 g Fludioxynil/kg+100 g Cymoxanil/kg+175 g Metalaxil/kg) at the rate of 2 g/kg of seed. Lupin seeds were treated with a.i. Iprodione (0·95 g/kg of seed) and Carbendazim (0·45 g/kg of seed), and were inoculated with NPPL HiStick (Becker Underwood) prior to sowing. The seed rate was 75 germinating seeds/m2 for pea, 45 for lupin and small-seeded faba bean, 36 for medium-seeded faba bean and 27 for large-seeded faba bean. The depth of sowing was 30 mm for pea and lupin and 60 mm for faba bean. The plots were 9 m2, and included 16 rows 3 m long and 180 mm apart for pea, and eight rows 3 m long and 360 mm apart for lupin and faba bean. The grain harvest area was 4·5 m2. Fertilizer was applied at 20 kg N/ha, 48 kg P2O5/ha and 112 kg K2O/ha at Lodi, and 54 kg N/ha and 138 kg P2O5/ha at Foggia.
The mean weight of the sown seed was recorded. The proportion of plants killed in winter was based on plant counts performed at the onset of winter and of spring along one linear m in each of two rows. A visual 9-level cold susceptibility score was recorded about 1 week after the main frost event and defined by proportions of damaged leaves/stipules and plant mortality as follows: 1=no damage; 2=no plant mortality, less than 0·10 damaged leaves/stipules; 3=no mortality, 0·10–0·20 damaged leaves/stipules; 4=negligible mortality, 0·20–0·40 damaged leaves/stipules; 5=less than 0·05 mortality, 0·40–0·60 damaged leaves/stipules; 6=0·05–0·25 mortality, more than 0·60 damaged leaves/stipules; 7=0·25–0·50 mortality; 8=0·50–0·75 mortality; 9=more than 0·75 mortality (failure to meet one condition led to the lower susceptibility class). Seedling height, vegetative stage as number of true leaves (or tendrils), and seedling height/vegetative stage ratio, was observed around mid-January on four random plants. Seedling dry weight was observed around mid-January on two random plants. The onset of flowering was recorded as the number of days after 1 March when half of the plants had the first open flower. Grain yield was harvested on individual plots when ripe (i.e. when straw and pods were almost completely yellowish-brown) and expressed at seed moisture of 130 g/kg. In Lodi, the cold susceptibility score was recorded after each of two main frost events, retaining a posteriori only that implying the greater plant damage.
Statistical analysis
Plot data were submitted to analysis of variance (ANOVA) holding location, sowing time, species and cultivar as fixed factors. ANOVA error terms were defined according to the features of strip-plot and group-block designs (Gomez & Gomez Reference Gomez and Gomez1984). A separate ANOVA for each species and location included the factors sowing time, cultivar and block. A second ANOVA for each species also included the location factor. Plant mortality and grain yield data were also subjected to a separate ANOVA for each site which included the factors species, sowing time, cultivar within species and block, and to a fourth ANOVA including also the location factor. The set of most winter-hardy entries included those not different for winter survival at P<0·05 according to Dunnett's one-tailed test.
The consistency of cultivar plant mortality across sowing times in each location was assessed for each species in terms of genetic correlation (r g) for entry proportion of winterkilled plants between the two environments represented by the sowing times, estimating r g according to Burdon (Reference Burdon1977) as:

where r is the phenotypic correlation for entry value between the two environments, and h 1 and h 2 are the square root of the broad-sense heritability on an entry mean basis for each of the two environments. For each environment, broad sense heritability, h 2, was estimated as:

where s g2 and s e2 are variance components for genotype (here random factor) and experiment error, respectively, estimated by a Restricted Maximum Likelihood method, and n is the number of replications. The consistency of entry plant mortality across locations was assessed likewise for each species, by computing the genetic correlations between all possible pairs of environments belonging to different sites and then averaging the four genetic correlation coefficients. Averaging was performed on values previously submitted to the z (inverse tanh) transformation, back-transforming to correlation value the average z value.
For each species, phenotypic correlations were computed: (i) between winter mortality and grain yield of cultivars, in each location and over locations; (ii) between winter mortality and seed, seedling or phenology traits of cultivars over locations. Despite no significant correlation (P<0·30) between winter mortality and sown seed weight, partial correlation coefficients were computed to partial out the influence of entry seed size on the relationships of winter mortality with seedling or phenology traits. This provided a better understanding of trait relationships as they may occur in material with similar seed size, as envisaged by Austin & MacLean (Reference Austin and MacLean1972) for common bean in relation to the limited commercial scope for selecting very large-seeded varieties (given the higher sowing cost that they entail).
An optimal index of indirect selection for low winter mortality was selected for each species through a stepwise multiple regression of proportion of winterkilled plants as a function of seedling and phenology traits of the cultivars, using entry values averaged across environments and setting P<0·20 for a variable to enter or stay in the model. Sown seed weight was included among the candidate traits only if it tended to correlate (P<0·30) with winter mortality (thereby accounting for its influence). The partial regression coefficients in the selected multiple regression model were used as the index weights (Wricke & Weber Reference Wricke and Weber1986).
Direct selection in one environment can be viewed as an indirect selection criterion for the target trait over the target population of environments when the trait is subject to genotype×environment interaction (Falconer Reference Falconer1989). Thus, direct selection for low winter mortality and indirect selection based on the cold susceptibility score or the optimal selection index were compared for each species on the basis of the correlated selection response over target environments as predicted from indirect selection theory, using the procedure described by Annicchiarico & Pecetti (Reference Annicchiarico and Pecetti1998). Each of the four environments, defined by the combination of two locations by two sowing times, acted in turn as selection environment, with the remaining three as test environments. The genetic correlation of each selection criterion in the selection environment with mean plant mortality over the test environments was computed according to Eqn (1) where, in this case: r is the phenotypic correlation between values in the selection environment and mean proportion of winterkilled plants over the test environments of the entries; h 1 is the square root of the broad-sense heritability on an entry mean basis of the criterion in the selection environment, estimated as in Eqn (2); and h 2 is the square root of the broad-sense heritability of plant mortality over the test environments, estimated as:

where s g2, s ge2 and s e2 are variance components for genotype, genotype×environment interaction and pooled experiment error, respectively, and e and n are numbers of environments and replications. The predicted correlated response (CR) of low plant mortality over three environments when selecting in a fourth environment is (Falconer Reference Falconer1989):

where i is the standardized selection differential, and s p2 is the square root of the phenotypic variance of plant mortality over the test environments (equal to the denominator of Eqn (3)). Assuming a constant intensity of selection, i, h 2 and s p2 in Eqn (4) are constant for all selection criteria, and the relative efficiency of each criterion depends on its heritability and its genetic correlation with the target trait. The results for the single selection environments were averaged.
The proportion of plant mortality was submitted to angular transformation prior to ANOVAs (back-transforming mean±s.e. values to proportions), but original data were preferred for correlation and regression analyses because of their greater meaningfulness for germplasm selection. However, original and transformed data provided very similar correlation coefficients and the same variables were selected in the stepwise multiple regression (data not reported).
The Statistical Analysis System (SAS) software was used for all analyses.
RESULTS
Variation for winter hardiness
Lodi was much colder than Foggia in terms of number of frost days (54 v. 8), absolute minimum temperature (−7·8 v. −3·4°C) and mean temperatures over the late autumn and winter months (Table 2). The similarity between test year and long-term temperature values (Table 2) suggested that the test years represented the ordinary pattern of late autumn and winter temperatures at each site fairly well. In Foggia, the lowest temperatures occurred around mid-February, after the onset of flowering of all faba bean entries in the early sowing and of the early entries in the remaining species-sowing time combinations. The difference in mean onset of flowering between sowing times ranged from 5 days for lupin to 8 days for pea in Lodi, and from 3 days for lupin and pea to 5 days for faba bean in Foggia.
Table 2. Test-year and long-term values of absolute minimum temperature, number of frost days and mean daily temperature across late-autumn and winter in the two test locations

On average, winter plant mortality was greater in Foggia than in Lodi for all species (Table 3). The sowing time with minimal plant mortality in each location differed with species. Greater mortality was associated with earlier sowing of faba bean and pea but with later sowing of lupin in Lodi, and with earlier sowing of lupin in Foggia (Table 3). The differences in mortality between sowing times (Table 3) highlighted the susceptibility of lupin and, to a lesser extent, faba bean to winter injuries derived from suboptimal sowing time.
Table 3. Comparisons between sowing times within location and between locations, for proportion of winterkilled plants and grain yield in three grain legume species (mean±s.e.)

* d.f.=2.
† d.f.=4.
Species comparison for winter plant mortality was affected by species×location and within-site species×sowing time interactions (P<0·01). On average, lupin exhibited lower mortality than faba bean in Lodi and greater mortality than faba bean in Foggia (P<0·05).
For each species at each site, cultivar variation for proportion of winterkilled plants over sowing times was significant (P<0·01) and fairly wide, with a range of at least 0·22 between the top- and bottom-ranking entry, and at least 0·27 for the least winter-hardy entry (Table 4). The three species did not differ markedly for mortality of the top-surviving material or for range of entry mortality over locations (Table 4). Range values for entries (Table 4) and species (Table 3) indicated that within-species variation for winter mortality was much larger than between-species variation. The set of most winter-hardy entries over sites included: (i) the large-seeded varieties Sicilia and Protera and the small-seeded variety Diva, for faba bean; (ii) the varieties Luxe and Lunivers and the Italian landrace, for lupin; (iii) the Ukrainian variety Kharkov Etalonniy, many cultivars bred in western Europe (Eclipse, Dolmen, Austin, Alembo, Spirale, Claudius, Dove, Santana, Declic, Pinochio, Cheyenne, Guifilo, Attika and Speleo), and the Australian varieties King, Magnet and Kaspa, for pea. With regard to cultivar phenology type (as reported by UNIP-ITCF (2002b) or breeder's information), the material with greater winter-hardiness belonged to winter or Mediterranean types for faba bean and lupin, and to all possible types (winter, Mediterranean or spring) for pea.
Table 4. Ranges of proportion of plants killed in winter and of grain yield (t/ha) in each location and over locations for cultivars of three grain legume species

Cultivar values averaged across two sowing times. Cultivar variation within species and location always significant at P<0·01.
Cultivar×sowing time interaction was present in pea and lupin in both locations (P<0·05), whereas cultivar×location interaction was observed in all species (Table 5). The consistency of entry responses for winter mortality, as indicated by the closeness to unity of the genetic correlation between environments, was high across sowing times and low across locations for faba bean, moderate across sowing times and fairly low across locations for pea, and fairly high across locations or sowing times for lupin (Table 5). Hence, the largest inconsistencies for cultivar response took place for faba bean and, to a lesser extent, for pea across locations. Most of the cultivar×location interaction in faba bean was accounted for by the relatively better response in Foggia than in Lodi showed by several large seeded and/or Mediterranean entries (Prothabon 101, Sicilia, Protera and Gemini). The cultivar×location interaction in pea was partly due to the response of winter-type germplasm, which tended to greater winter survival in Lodi but similar survival in Foggia relative to spring-type and Mediterranean material. For lupin, the consistency of entry response was due to greater mortality of the early-flowering, spring-type material in all environments.
Table 5. The proportion of winter killed plants across two sowing times in two locations and across two locations as measured by coefficients of genetic correlation (rg) of entry values between relevant environments, and P level of the relevant genotype×environment interaction, for three grain legume species

Relationship of winter hardiness with grain yield
The combination of greater frost injury and greater terminal drought and heat stress could account for the distinctly lower yield showed by all species in Foggia relative to Lodi (Table 3). The yield gap was smaller for the earliest-flowering species, i.e. faba bean, and larger for the latest-flowering one, i.e. lupin (Table 3). The susceptibility to soil lime further lowered the lupin yields in Foggia but hardly affected sizably the winter mortality, since the lime-induced chlorosis of plants appeared only in spring.
For each species in each site, the sowing time which showed least winter mortality tended to have greater grain yield (Table 3). Sowing time affected also the species comparison for grain yield. For instance, lupin showed higher yield and winter survival in the early sowing but non-different yield and lower winter survival in the late sowing of Lodi in comparison with faba bean (P<0·05). However, pea exhibited higher mean yield than faba bean or lupin in any environment (P<0·05).
All species displayed variation among cultivars for grain yield in both locations (P<0·01). The three species-location combinations which showed the largest entry variation for winter mortality, i.e. faba bean in Lodi, and pea and lupin in Foggia, were also characterized by large entry variation for grain yield (Table 4) and a strict inverse correlation of winter mortality with grain yield of cultivars (Table 6). The relationship between winter hardiness and grain yield emerged also for pea and lupin in Lodi. It was not significant for faba bean in Foggia (Table 6) owing to the outstanding yield response of Mediterranean germplasm with early flowering and only moderate winter-hardiness (the importance of earliness was revealed by the strict negative association between yield and days to flowering: r=−0·73, P<0·01). Lower winter mortality was associated with higher grain yield of cultivars across locations in all species (r⩽−0·43; Table 6).
Table 6. Correlation coefficients of proportion of plants killed in winter with grain yield of cultivars in each location and over locations, for three grain legume species

Cultivar values averaged across two sowing times. d.f.=22 for faba bean, 47 for pea and 9 for lupin.
Traits associated with winter hardiness
Genetic variation for cold susceptibility score, seedling traits and onset of flowering was detected for all species in both locations (P<0·01). Range values of entries over locations are reported for these traits in Table 7. In general, the cold susceptibility score recorded in winter implied lower values of plant mortality than those observed for winter survival at the onset of spring. The range of entry variation for days to onset of flowering was larger in pea and lupin than in faba bean (Table 7). However, the distribution of entry values for this trait implied several cultivars with extreme values for faba bean and lupin, and only one outstandingly late cultivar for pea (data not reported).
Table 7. Range and coefficients of simple or partial correlation with the proportion of plants killed in winter, for cultivar values of seed, seedling and phenology traits and an index of indirect selection for low winter mortality averaged over two locations and two sowing times, for three grain legume species

d.f.=22 for faba bean, 47 for pea and 9 for lupin, for simple correlation; 21 for faba bean and 8 for lupin, for partial correlation.
* After partialling out the effect of sown seed weight.
† Observed in January; vegetative stage of seedling as number of true leaves.
‡ Indexes are: I=−0·238+0·1238 CS+0·0808 HSR−0·000215 SW, for faba bean; I=0·017+0·0251 CS+0·0352 HSR, for pea; I=−0·203+0·116 CS, for lupin; where I=Index of winter mortality, CS=Cold susceptibility score; HSR=Height/stage ratio; SW=Sown seed weight.
The relationship of lower winter mortality with larger sown seed was clear in the species with largest seed size variation (faba bean), but absent in that with lowest seed size variation (pea, Table 7) and poor in lupin (P<0·30), where the Italian landrace showed the largest seed. The correlation of winter mortality with the cold susceptibility score ranged from moderate in pea to very high in lupin (Table 7). Lower winter mortality was also associated with a rosette-like winter growth habit (defined by lower values of the seedling height/vegetative stage ratio) and shorter seedling in pea, and with seedling biomass in faba bean (Table 7). In faba bean and lupin, heavier seed was closely associated with seedling biomass (r>0·92, P<0·01) and poorly correlated with seedling height and seedling height/vegetative stage ratio (r>0·47, P<0·15). Partialling out the influence of sown seed weight highlighted, for winter survival: (i) its very high correlation with low cold susceptibility score, in faba bean; (ii) its association with a rosette-like winter growth habit, in faba bean and lupin; (iii) its association with shorter seedling and lateness of flowering, in lupin; (iv) the dependence on seed weight of its correlation with seedling biomass, in faba bean (Table 7).
Selection criteria for winter hardiness
The optimal index of selection for low winter mortality across environments issued by stepwise multiple regression analysis included the cold susceptibility score for all species, the seedling height/vegetative stage ratio for pea and faba bean, and the sown seed weight for faba bean. The index formula for estimating the entry proportion of winterkilled plants is reported for each species as a footnote in Table 7. The correlation between observed values of entry mortality over environments and values predicted by the index was very high for faba bean and lupin and moderate for pea (Table 7).
On average, index-based selection exhibited distinctly higher predicted efficiency than direct selection for winter survival in faba bean (+29%), owing to higher broad-sense heritability and higher genetic correlation with the target trait in the individual selection environments (Table 8). For lupin and pea, the index showed higher heritability but somewhat lower genetic correlation with the target trait relative to direct selection (Table 8), allowing for a modest efficiency gain (+10% for lupin; +4% for pea). In faba bean and pea, the indirect selection based on the cold susceptibility index alone was less efficient than direct selection (Table 8).
Table 8. Average values across four selection environments of broad-sense heritability on an entry mean basis (h2), coefficient of genetic correlation with proportion of winterkilled plants over the test environments (rg) and predicted selection efficiency (E), for direct and indirect selection criteria for low winter plant mortality

* Using by turns one location-sowing time combination as selection environment and three location-sowing time combinations as test environments.
† Equals 100 for direct selection.
‡ Reported as a footnote in Table 7.
DISCUSSION
The results, despite the limitation of 1-year duration of the assessment, are reinforced by the consistency between test-year and long-term values of winter temperatures on the sites. The trend towards higher plant survival and/or grain yield of the late sowing for all grain legumes in Foggia, the late sowing for pea and faba bean in Lodi and the early sowing for lupin in Lodi requires further verification before defining optimal sowing times for each species in the two regions represented by the test sites. The results, however, highlight the following aspects of frost tolerance of the species: (i) the high winter mortality of grain legumes that may arise in Mediterranean environments, owing to chilling of incompletely-hardened or dehardened plants; (ii) the negative impact of suboptimal autumn-sowing time on the winter survival and grain yield of the crops, especially white lupin; and (iii) the dependence of species comparison on sowing time and location (due to species×environment interactions) and on the specific sample of entries (due to the large within-species variation in winter hardiness). In Foggia, the main frost events occurred after a period of winter mean daily temperatures often exceeding 8°C (Table 2), which is insufficient for complete hardening and sufficient for dehardening to commence (Prieur & Cousin Reference Prieur and Cousin1978; Herzog Reference Herzog1989). The narrow optimal autumn-sowing time of white lupin is well-documented (Huyghe & Papineau Reference Huyghe and Papineau1990; Papineau & Huyghe Reference Papineau and Huyghe2004) and may account for the greater mortality of the late sowing in Lodi, likely caused by insufficient development of the seedling root parenchyma, and the greater mortality of the early sowing in Foggia, related to widespread floral initiation. The trend towards somewhat greater winter hardiness of faba bean relative to pea reported by Murray et al. (Reference Murray, Eser, Gusta, Etévé and Summerfield1988) does not seem to apply to recent germplasm, given the absence of statistical differences in winter mortality between these species and the slight trend towards greater survival over environments of pea (Table 3). This result, and the distinctly greater grain yield of pea relative to the other species that emerged in any environment, may partly derive from the greater breeding effort devoted to this species in the last decades.
The large within-species variation in winter survival and its sizeable impact on cultivar adaptation and grain yield that were found in the three species emphasize the importance of winter hardiness for breeding and variety choice in southern Europe. Winter survival of entries, here used for estimating winter hardiness, may also derive from tolerance to waterlogging or winter-occurring diseases and pests besides tolerance to frost (Murray et al. Reference Murray, Eser, Gusta, Etévé and Summerfield1988). While some waterlogging occurred in Lodi, no major biotic stress was observed in either location during winter.
The poor consistency of entry winter hardiness across locations observed for faba bean can be related to the fairly poor consistency of entry frost tolerance that may occur between conditions of complete and limited hardening (Herzog Reference Herzog1988). The relatively better frost tolerance showed by Mediterranean germplasm in Foggia, i.e. under conditions of incomplete hardening and partial dehardening, may arise from evolutionary adaptation to short frosts occurring within mild winters. The same trend showed by large-seeded material may derive from the strict relationship of larger size with earlier plant development and the positive relation of plant biomass with frost tolerance in incompletely-hardened material (Herzog Reference Herzog1988). Breeding faba bean for wide adaptation to contrasting regions such as those represented by the test sites is complicated by the magnitude of the entry×location interaction for grain yield which arises from the entry×location interaction for winter hardiness, and the different adaptive value of early flowering in the two regions. However, the relatively small commercial importance of faba bean, pea or white lupin in southern Europe probably justifies their breeding for wide adaptation. In this context, our results support the parallel selection for faba bean winter hardiness across contrasting locations since early selection stages, adopting just one sowing time per site. Useful genetic resources may be restricted to winter and Mediterranean phenology types. For pea entries, the moderate to fairly low consistency of winter hardiness across locations or sowing times and the lack of relationship of winter survival over locations with onset of flowering or phenology type (spring, Mediterranean and winter) revealed a more complex pattern of variety responses relative to those in higher latitudes (Lejeune-Hénaut & Wery Reference Lejeune-Hénaut, Wery, Ney, Duchêne, Carrouée and Angevin1994). This complex pattern, which may be due to entry variation for rate of hardening and dehardening and to partly different variety rank for intrinsic frost tolerance passing from fully-hardened to partly-hardened material, supports the parallel selection across contrasting locations and autumn sowings. Artificial freezing tests, which are valuable for predicting the frost tolerance and discriminating between spring and winter types in fully-hardened pea or faba bean material (Herzog Reference Herzog1988; Lejeune-Hénaut & Wery Reference Lejeune-Hénaut, Wery, Ney, Duchêne, Carrouée and Angevin1994), may be less efficient than field testing for areas, such as Foggia, where chilling tends to occur on incompletely-hardened plants.
The substantial consistency of lupin entry responses for winter hardiness suggests the limited need for multi-environment testing of this trait. The expected relationship between earliness of flowering and frost susceptibility was manifest only in this species, whose wide genetic variation for phenology encompassed material with sufficiently delayed reproductive development also in the late sowing of the warmer site. While early-flowering, spring-type germplasm is less adapted than winter or Mediterranean material, the selection of frost-tolerant, early varieties may be required to achieve sufficiently high lupin yields also in southern Italy.
The practical interest of breeding for wide adaptation justifies the assessment of correlations of winter mortality with morphophysiological traits and the definition of selection indexes with reference to entry values averaged across locations. The positive relationship between winter survival and seed size in faba bean and lupin was expected on the basis of earlier studies (Herzog Reference Herzog1988; Papineau & Huyghe Reference Papineau and Huyghe2004). Partialling out the seed size effect highlighted for all species the relationship of winter hardiness over locations with a rosette-like winter growth habit, confirming the earlier indications of Lawes et al. (Reference Lawes, Bond, Poulsen and Hebblethwaite1983) in faba bean, Etévé (Reference Etévé, Hebblethwaite, Heath and Dawkins1985) in pea and Huyghe & Papineau (Reference Huyghe and Papineau1990) in lupin. For plants at the same vegetative stage, a shorter seedling may contribute to greater winter survival through its relationships with lower relative plant water content (Herzog Reference Herzog1988) and later flowering initiation (Papineau & Huyghe Reference Papineau and Huyghe2004). Its correlation with winter survival may be obscured by the effect of seed size, because genetically or environmentally determined larger seeds tend to produce larger and taller seedlings (Huyghe Reference Huyghe1993) and contribute to greater winter survival via the thicker root parenchyma of the larger seedling (Papineau & Huyghe Reference Papineau and Huyghe2004). In lupin, dwarfing genes have a very limited effect on basal internodes (Harzic Reference Harzic1996) and were unlikely to bias the assessment of traits related to winter survival by sizably reducing the seedling height and the height/vegetative stage ratio of the three cultivars in which they were present.
Selection indices for winter hardiness over locations were defined and compared with direct selection to verify the practical value as indirect selection criteria of the visual cold tolerance score and the other traits correlated with winter survival. The indications were not conclusive, as the sample of test environments was neither large nor randomly chosen and, for lupin, also the entry sample was rather small. The efficiency gain predicted for indirect selection encourages the adoption of the selection index in faba bean to substitute or, at least, to complement the direct selection for winter survival. The index-based selection may still be envisaged for lupin, in view of its modest increase in selection efficiency accompanied by its lower cost of observation (limited to the cold susceptibility index) relative to direct selection. For pea, the results suggest that the difficulty of direct selection across the contrasting environments may hardly be overcome by indirect selection. The scope for indirect selection, here assessed for simple, low-cost morphophysiological traits, may be widened by the contribution of physiological and biochemical tests (Stoddard et al. Reference Stoddard, Balko, Erskine, Khan, Link and Sarker2006), especially after improving our understanding of the physiological mechanisms that contribute to cold tolerance under the wide variation in winter temperatures typical of Mediterranean environments.
This work was carried out within the project ‘Increase of protein feed production’ funded by the Italian Ministry of Agricultural and Forestry Policies. The authors wish to thank Sandro Proietti and Vito Miullo for their technical assistance.