Introduction
Food production will remain viable over time if sustainable production methods are practised. Hence, many countries have adopted systems for improving agricultural yields that are associated with ecosystem conservation, soil improvements and adaptation to climate change (United Nations, 2021). Satisfactory results have been achieved with the use of cover crops as they contribute to improved soil quality, due to high organic matter (Schipanski et al., Reference Schipanski, Barbercheck, Douglas, Finney, Haider, Kaye, Kemanian, Mortensen, Ryan, Tooker and White2014) and nutrient cycling (Pacheco et al., Reference Pacheco, Barbosa, Leandro, de Machado, de Assis, Madari and Petter2013). Consequently, easing the over-reliance on fertilizers and pesticides, which in turn are environmentally unsustainable. Moreover, cover crops could control pests (Schipanski et al., Reference Schipanski, Barbercheck, Douglas, Finney, Haider, Kaye, Kemanian, Mortensen, Ryan, Tooker and White2014) and weeds (Martins et al., Reference Martins, Gonçalves and Silva Junior2016), by covering the space or area needed by weeds.
Intercropping is the best management to introduce cover crops into cropping systems. This system results in minimal changes to the routine of farmers and combines economic returns with ecosystem services for the environment and soil conservation. Overall, intercropping systems combine growing several species with each other during a season as an alternative to monoculture. Intercropping has been attributed to the fourth green revolution since it makes agriculture ecologically promising by producing more biomass in the same area than sole cropping (Martin-Guay et al., Reference Martin-Guay, Paquette, Dupras and Rivest2018).
Among cereal crops, maize is prioritized for intercropping with cover crops. This is because, in addition to its economic and social importance (USDA, 2021), maize has vigorous vegetative growth stages. These traits are advantageous in intercropped systems, mitigating the competition between cover crops and grain yield (GY) of the cereal crop (de Oliveira et al., Reference de Oliveira, Kluthcouski, Favarin and de Santos2010; Kappes and Zancanaro, Reference Kappes and Zancanaro2015). Intercropping systems of maize with grasses (Kluthcouski et al., Reference Kluthcouski, Cobucci, Aidar, Yokoyama, Oliveira, Costa, Silva, Vilela, Barcellos and Magnabosco2000) and legumes (de Oliveira et al., Reference de Oliveira, Kluthcouski, Favarin and de Santos2010) are successful examples of maize intercropping systems that have generated economic, social and environmental benefits.
Using forage grasses, such as Urochloa ruziziensis, in the cropping system contributes to the chemical and physical aspects of the soil (Silveira et al., Reference Silveira, Silva, Lobo Junior and Cunha2011; Mingotte et al., Reference Mingotte, Jardim, Yada, Amaral, Chiamolera, Coelho, Lemos and Fornasieri Filho2020; Tan, Reference Tan2020). Additionally, they stand out for their high straw yield, further contributing to sustainability (Mingotte et al., Reference Mingotte, Yada, Jardim, Fiorentin, Lemos and Fornasieri Filho2014). Legume species, such as crotalarias, are called green manure crops which are associated with microorganisms that are capable of fixing nitrogen biologically (BNF), receive nutrients and release them into the soil through root exudates (Murrell et al., Reference Murrell, Schipanski, Finney, Hunter, Burgess, LaChance, Baraibar, White, Mortensen and Kaye2017) or decompose the crop remains (Marcelo et al., Reference Marcelo, Corá and Fernandes2012). Crotalaria spectabilis can supply up to 160 kg/ha of nitrogen (N) to the soil, reducing N fertilizer usage in the production system (Calegari and Carlos, Reference Calegari, Carlos, de Lima Filho, Ambrosano, Rossi and Carlos2014).
High N levels are usually recommended for crop fertilization management because N is the most extracted nutrient by plants and has complex dynamics in the soil–plant–atmosphere system. However, several recommendations do not consider the cropping systems, such as intercropped systems, used by crop producers. Hence, more specific N fertilization recommendations are needed in this context to optimize its use in agriculture.
Maize crops require 20–25 kg/ha of N to produce 1 Mg/ha of grains (Fan et al., Reference Fan, Wang, Liao, Ali Raza, Wang, Zhang, Chen, Feng, Wu, Liu, Yang and Yang2020). In the context of sole maize cropping, the N requirement for obtaining high maize yields reaches 30 kg/ha at sowing and 140 kg/ha with top-dressing fertilization (Raij and Cantarella, Reference Raij, Cantarella, Raij, Cantarella, Quaggio and Furlani1997). However, the intercropping of cover crops with maize may alter the N requirement for obtaining high maize yields because these cover crops may absorb the applied N, resulting in a lower N availability for maize. The species used for intercropping (grass or legumes) may also interfere with the dynamics of N in the soil, directly affecting the management of N fertilization. Therefore, further studies are needed to determine the amount of N required by maize in intercropping systems.
The present study thus aimed to verify whether the management of top-dressing N fertilization is changed by intercropping maize with grass (U. ruziziensis) and legume (C. spectabilis) in comparison with that by sole cropping of maize.
Materials and methods
Experimental area location and characterization
Experiments were conducted during the first cropping season (summer) of 2016/17 and 2017/18, respectively. Both experiments were conducted in the same area, located at the São Paulo State University (Unesp), School of Agricultural and Veterinarian Sciences, in Jaboticabal, São Paulo, Brazil (21°14′59″S, 48°17′13″W and 565 m of altitude).
The climate of the site is ‘Aw’, characterized as humid tropical with rainy season in summer and dry season in winter, according to Köppen's classification (Rolim et al., Reference Rolim, Camargo, Lania and Moraes2007). The soil is an Oxisol (Soil Survey Staff, 2014), with 540 g/kg of clay, was previously cultivated with maize in the conventional tillage system, and was kept in fallow until installation of the experiments.
Experimental design and treatments
The experimental design used was randomized complete block design in a split-plot structure, with four replicates. The plots consisted of three cropping systems (sole maize, maize intercropped with U. ruziziensis and maize intercropped with C. spectabilis) and the subplots were four N levels (0, 70, 140 and 210 kg/ha), applied broadcast as top-dressing at V6 stage of maize. The fertilizer used as the source of the nutrient was Kimcoat® polymer-coated urea, with 44% of N. Each subplot consisted of five maize rows with length of 10 m and spaced at 0.45 m, in a total area of 22.5 m2. The three central rows were considered usable area, disregarding 0.5 m at the ends.
Experiment installation and conduction
Pretreatment
Before the installation of the experiment in 2 years, the soil attributes were determined in the 0.00–0.20 m layer (Table 1).
Table 1. Soil chemical attributes of the 0.00–0.20 m layer analysed prior to the start of the experiment

OM, organic matter; SB, sum of bases; CEC, cation exchange capacity; V, base saturation.
a pH CaCl2; bP resin.
Conventional tillage was performed in both years, with plowing as well as operations with disc harrow and levelling harrow. In addition, 2 t/ha of dolomite limestone with 90% relative total neutralizing power were applied 90 days before experiment in 2017/18. This management was necessary because 6 months after the harvest during the first year of the experiment, we verified that the soil base saturation was below 50%. Thus, the application of lime was necessary to increase the soil base saturation level and promote ideal conditions for plant growth in the second year of the experiment. After liming, the soil base saturation increased to 67% (Table 1).
Sowing
Sowing in the cropping systems was performed at the same location in the two experiments. Cover crops were distributed broadcast, using 20 kg/ha of C. spectabilis seeds and 15 kg/ha of U. ruziziensis seeds. Then, a seeder-fertilizer was used to sow the maize hybrids at the site, which led to the deposition of soil on the seeds of the cover crops.
The maize sowing in the first year was carried out on 11/22/2016, using the hybrid DOW 2B710 PW, in the interrow spacing of 0.45 m and with an adjusted population of 60 000 plants per ha. The crop management used for this maize hybrid was carried out as recommended by the manufacturer. The sowing furrow fertilization was calculated as a function of the soil attributes determined before the experiment installation and according to the recommendations of Raij and Cantarella (Reference Raij, Cantarella, Raij, Cantarella, Quaggio and Furlani1997). At seed furrow, 20 kg/ha of N, 70 kg/ha of P2O5 and 40 kg/ha of K2O were applied using the formulated fertilizer 04-14-08 (urea + single superphosphate + potassium chloride).
The maize sowing in the second year was carried out on 11/10/2017, using the hybrid DOW 2B810 PW, in the interrow spacing of 0.45 m and with an adjusted population of 88 000 plants per ha. The crop management used for this maize hybrid was carried out as recommended by the manufacturer. The seed furrow fertilization was calculated as a function of the soil attributes determined before the experiment started and according to the recommendations of Raij and Cantarella (Reference Raij, Cantarella, Raij, Cantarella, Quaggio and Furlani1997). At seed furrow, 25 kg/ha of N, 89 kg/ha of P2O5 and 51 kg/ha of K2O were applied using the formulated fertilizer 08-28-16 (urea + triple superphosphate + potassium chloride).
Fertilization input
In two years, top-dressing fertilization was performed at the V6 stage of the crop, on 12/24/2016 and 11/28/2017, with N levels defined by the treatments. In addition to N, 45 kg/ha of K2O was also applied on the same day in both years, using potassium chloride as a source.
Climate characterization in the period
The meteorological data recorded during the experiments are presented in Fig. 1. In 2016/17, the average maximum and minimum temperatures were 31.1 and 19.4 °C, respectively, and the accumulated precipitation was 761.6 mm. In 2017/18, the average maximum and minimum temperatures were 30.5 and 19.5 °C, respectively, and the accumulated precipitation was 760.4 mm.

Fig. 1. Maximum and minimum air temperature ( °C) and precipitation (mm) during the experimental period. Dashed arrows indicate the times of sowing of maize and cover crops (1), top-dressing nitrogen fertilization (2) and harvest (3) of sole maize and intercropped systems, in the 2016/17 (a) and 2017/18 (b) cropping seasons.
The plants were grown under rainfed conditions for two years; however, on the first and second day of maize sowing of 2016/17, conventional sprinkler irrigation with 10 mm water depths was performed, for the establishment of the plants. When necessary, the crop practices related to the control of pests and diseases were carried out based on monitoring. Chemical management of weeds was performed in the system with sole maize, at 25 days after emergence, using the herbicide potassium glyphosate at a rate of 2500 g/ha of a.i. in both years. Maize plants were mechanically harvested on 04/16/2017 and 04/06/2018, respectively.
Evaluations
The evaluated variables analysed in the two years were the same. Leaf nitrogen content (g/kg) was evaluated during the flowering (R1 stage) of maize, with the collection of the middle third without the midrib of ten leaves, located below and on the opposite side of the main ear, in ten plants per subplot (Cantarella and Raij, Reference Cantarella, Raij, Raij, Cantarella, Quaggio and Furlani1997). The samples were washed with running water and detergent at 1 ml/l, dried in an oven with forced air circulation at 65–70 °C, and then processed in a Wiley-type mill to determine the N content, according to semi-micro Kjeldahl method (Carmo et al., Reference Carmo, de Araújo, Bernardi and Saldanha2000).
Stem diameter (mm) was obtained using a digital caliper, for five plants per subplot, in the second internode above ground, at the maize physiological maturity. Plant height (cm) was measured at the time of physiological maturity of the maize crop, for five plants per subplot, as the distance from the soil surface to the flag leaf, with a graduated ruler. Main ear height (cm) was determined as the distance from the soil surface to the main ear, with a graduated ruler, for five plants per subplot at the physiological maturity of the maize crop.
At the maize harvest time, ten ears were randomly collected to determine the production components of maize: number of rows per ear (n°), number of grains per row (n°), number of grains per ear (n°), thousand-grain weight (g) and grain mass per ear. For the determination of thousand-grain weight and grain mass per ear, the grain moisture was corrected to 0.13 g/g.
Maize GY was obtained by manually harvesting the ears of the usable area in each subplot, which were mechanically threshed. The obtained grains were weighed and the value was corrected to a moisture content of 0.13 g/g.
To verify the effect of cropping systems and top-dressing N fertilization on grain production, the land equivalent ratio (LER) index was calculated according to Mead and Willey (Reference Mead and Willey1980) (Eqn (1)). This index allows assessing the area required by the sole cropping system to equal the total grain production of an intercropped system. Here, we considered the sole maize cropping system at a level of N equal to 0 as the control treatment. The average data of the two cropping seasons were used to calculate the LER:

where LER = land equivalent ratio; Y MM0 = sole maize GY at level 0 (kg/ha); Y CS = maize GY of the cropping system of interest (kg/ha) and Y CCM = sole cover crop dry mass yield (kg/ha).
The average production of the total dry mass by the sole cropping of C. spectabilis and U. ruziziensis for the two cropping seasons was 6.50 and 6.00 t/ha, respectively. These values were used to calculate the LER for each treatment.
Statistical analyses
For the study of the cropping systems, the data were subjected to analysis of variance by the F test, and the means were compared by the Tukey test at the 5% probability level. We use mixed models for statistical analysis, adopting treatments as fixed effects and blocks as random effects. We used Shapiro–Wilk test to assess the data normality (Royston, Reference Royston1995) and Levene's test to assess the homoscedasticity of variances (Gastwirth et al., Reference Gastwirth, Gel and Miao2009). Regarding the effect of N levels and their interaction with the cropping systems, polynomial regression analyses were performed. The statistical program Sisvar was used (Ferreira, Reference Ferreira2011).
In addition, due to the structure of dependence between the variables, multivariate statistics by principal components (PCs) was used to analyse the agronomic variables of maize, as a function of the factors cropping systems and N levels. All variables were standardized before analysis. The number of PCs was determined based on the Kaiser criterion, using those with eigenvalues greater than 1 (Kaiser, Reference Kaiser1958). For multivariate analysis, the statistical program used was Statistica v.7.
Results
Growth variables
Among the aspects that characterize nutritional diagnosis and growth of maize (Table 2), only main ear height was influenced by the cropping systems (first year: P < 0.001; second year: P < 0.05). In both years, the main ears of maize intercropped with C. spectabilis were located in the upper region of the stem. In the first year, leaf N content was affected (P < 0.01) differently between the cropping systems as a function of nitrogen fertilization (Fig. 2(a)). This variable increased quadratically up to the N level of 144 kg/ha in sole maize and linearly in the other cropping systems. Thus, the maximum value of leaf N content was not reached with the studied levels for the intercropping with C. spectabilis and U. ruziziensis, respectively increasing by 0.18 and 0.22 g/kg with the addition of 10 kg/ha of N. Stem diameter increased with the increment in N levels, and every 10 kg/ha of the nutrient added led to increment of approximately 0.09 mm in stem diameter (Fig. 2(b)).

Fig. 2. Leaf N content (a) and stem diameter (b) for maize as a function of nitrogen levels applied as top-dressing in the 2016/17 cropping season. ▴ Sole maize; ■ maize intercropped with C. spectabilis; • maize intercropped with U. ruziziensis.
Table 2. Leaf nitrogen content (LNC), stem diameter (SD), plant height (PH) and main ear height (EH) of maize in different cropping systems and subjected to top-dressing nitrogen levels in 2016/17 (year 1) and 2017/18 (year 2) cropping seasons

a Maize intercropped with U. ruziziensis.
b Different letters correspond to the significant difference at 5% probability level (P ≤ 0.05) by Tukey test.
c Maize intercropped with C. spectabilis.
d Sole maize.
**Differs by F test at 1% probability level; *differs by F test at 5% probability level; nsnot significant.
In the second year, for leaf N content, based on the interaction between the factors cropping systems and nitrogen fertilization (P < 0.001), there was a quadratic increase up to the N levels of 144, 125 and 198 kg/ha in sole maize, intercropped with C. spectabilis and intercropped with U. ruziziensis, respectively (Fig. 3(a)). In addition, when there was no supply of N as top-dressing (level 0), sole maize had a higher value of leaf N content (35 g/kg) compared to maize intercropped with C. spectabilis (27 g/kg) and U. ruziziensis (23 g/kg).

Fig. 3. Leaf N content (a), stem diameter (b) and main ear height (c) for maize crop as a function of nitrogen levels applied as top-dressing in the 2017/18 cropping season. ▴ Sole maize; ■ maize intercropped with C. spectabilis; • maize intercropped with U. ruziziensis.
Stem diameter and main ear height increased linearly as a function of nitrogen fertilization, at rates of 0.05 mm and 0.08 cm, respectively, for every 10 kg/ha of the nutrient added (Figs 3(b) and (c)).
Yield components and GY
Regarding the variables related to ear and GY, the cropping systems affected (P < 0.01) only in GY in the first year (Table 3). Thus, sole maize produced approximately 1200 kg/ha (15%) more than maize intercropped with U. ruziziensis (Table 3).
Table 3. Number of rows per ear (NRE), number of grains per row (NGR), number of grains per ear (NGE), thousand-grain weight (1000GW), grain mass per ear (GME) and GY of maize in different cropping systems and subjected to top-dressing nitrogen levels in 2016/17 (year 1) and 2017/18 (year 2) cropping seasons

a Maize intercropped with U. ruziziensis.
b Different letters correspond to significant difference at 5% probability level (P ≤ 0.05) by Tukey test.
c Maize intercropped with C. spectabilis.
d Sole maize.
**Differs by F test at 1% probability level; *differs by F test at 5% probability level; nsnot significant.
Regarding the increment of N in the top-dressing fertilization for the first year, there was a quadratic increase in the number of grains per row (Fig. 4(a)) and thousand-grain weight (Fig. 4(c)) up to the N levels of 204 and 169 kg/ha, respectively. As for the number of grains per ear, the increase was linear, and every 10 kg/ha of N led to an increment of 1.7 grains on the ear (Fig. 4(b)).

Fig. 4. Number of grains per row (a), number of grains per ear (b), thousand-grain weight (c) and GY (d) for maize crop as a function of nitrogen levels applied as top-dressing in the 2016/17 cropping season. ▴ Sole maize; ■ maize intercropped with C. spectabilis; • maize intercropped with U. ruziziensis.
For GY, different responses were observed between the cropping systems and N levels (first year: P < 0.001; second year: P < 0.01) (Fig. 4(d)). There was a quadratic increase up to 95 and 201 kg/ha of N for sole maize and intercropped with C. spectabilis, respectively. For maize intercropped with U. ruziziensis, the increment was linear, equal to 150 kg/ha for every 10 kg/ha of N added.
In the second year, the treatments did not affect the grain and ear variables (Table 3). As for nitrogen fertilization, every 10 kg/ha of N added led to an increases of 0.07 grains per row (Fig. 5(a)), 0.8 g in thousand-grain weight (Fig. 5(c)) and 0.7 g in the grain mass per ear (Fig. 5(d)). The number of grains per ear (Fig. 5(b)), however, increased quadratically up to the N level of 164 kg/ha. Based on the interaction between the factors cropping systems and N levels, GY increased up to the N level of 169 kg/ha for sole maize (Fig. 5(e)). On the other hand, in the intercropping with C. spectabilis there was no increase in GY as a function of N levels. For maize intercropped with U. ruziziensis, every 10 kg/ha of N applied led to an increment of approximately 90 kg/ha of grains, not reaching the maximum of the variable with the tested levels. In addition, without nitrogen fertilization, the yields were equal to 5.58, 6.83 and 5.84 t/ha, for sole maize, maize intercropped with C. spectabilis and maize intercropped with U. ruziziensis, respectively.
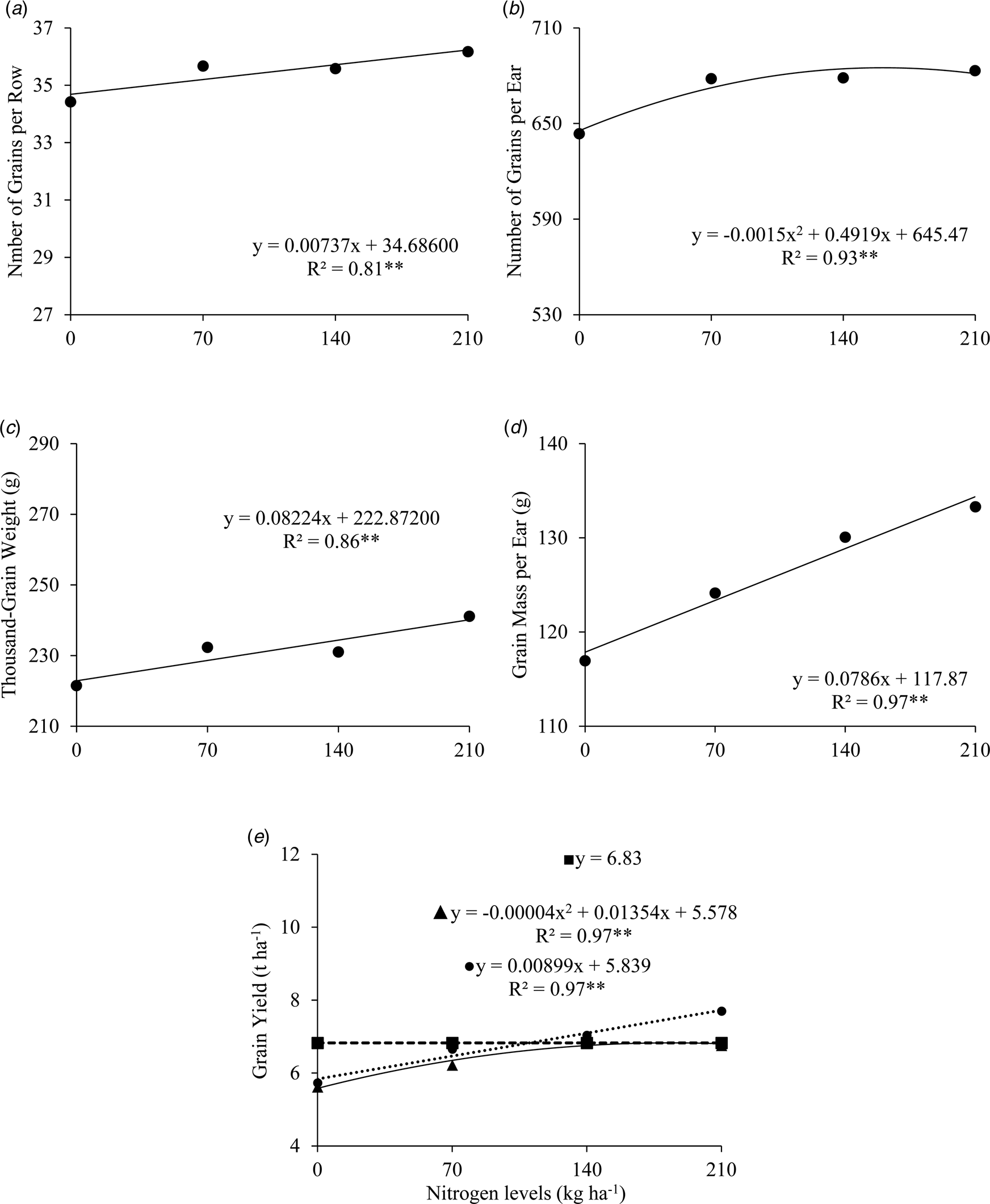
Fig. 5. Number of grains per row (a), number of grains per ear (b), thousand-grain weight (c), grain mass per ear (d) and GY (e) for maize crop as a function of nitrogen levels applied as top-dressing in the 2017/18 cropping season. ▴ Sole maize; ■ maize intercropped with C. spectabilis; ● maize intercropped with U. ruziziensis.
PCs analysis
Multivariate analysis of PCs for the agronomic variables of maize in the first year showed that the two first PCs were responsible for 68.84% of the total variability (Fig. 6(a)). The variables that most discriminated PC1 and PC2 were related to the production performance and vegetative growth of maize, respectively. Grain mass per ear (−0.94), stem diameter (−0.91), 1000-grain weight (−0.80), number of grains per ear (−0.85), GY (−0.65), number of grains per row (−0.86), leaf N content (−0.79) were correlated with PC1, called agronomic performance. On the other hand, PC2, called plant growth, was characterized by ear height (−0.92) and plant height (−0.92). The number of rows per ear was not part of any component this year.

Fig. 6. Biplot of the PCs for the distribution of cropping systems under nitrogen levels as a function of the agronomic variables of maize in experiments I (a) and II (b) in Jaboticabal, SP, Brazil. The cropping systems are indicated by M: sole maize, M + C: maize intercropped with C. spectabilis and M + U: maize intercropped with U. ruziziensis. The numbers accompanying the cropping system indicate the N level (kg/ha). The agronomic variables are: NRE = number of rows per ear; GME = grain mass per ear; SD = stem diameter; 1000GW = 1000-grain weight; NGE = number of grains per ear; GY = grain yield; NGR = number of grains per row; LNC = leaf N content; EH = ear height; PH = plant height.
Analysis of the biplot showed that the cropping systems with sole maize at N levels 70, 140 and 210 kg/ha, maize intercropped with C. spectabilis at the N level of 210 kg/ha and maize intercropped with U. ruziziensis at N levels of 140 and 210 kg/ha were the ones with the highest performance. This fact confirms that the requirement for nitrogen fertilization is lower for sole maize.
In the second year, principal components analysis (PCA) showed for the agronomic variables of maize (Fig. 6(b)), that the first two PCs were responsible for 73.14% of the total variability and that the variables that most discriminated PC1 and PC2 were related, respectively, to the agronomic performance and vegetative growth of maize, as in the first year. Thus, the variables that most discriminated PC1 were stem diameter (−0.93), number of grains per row (−0.83), number of grains per ear (−0.86), thousand-grain weight (−0.90), grain mass per ear (−0.94) and GY (−0.73). The variables most correlated with PC2 were leaf N content (0.60), plant height (0.87) and main ear (0.70). The number of rows per ear was not part of any component this year.
Based on the direct correlations between the variables and PC1, it was observed that the higher the agronomic performance of maize, the higher the GY, stem diameter, grain mass per ear, thousand-grain weight, number of grains per ear, number of grains per row and leaf N content. Furthermore, the biplot analysis showed that the highest agronomic performance occurred for sole maize at the N level of 210 kg/ha, maize intercropped with C. spectabilis at the N levels of 70, 140 and 210 kg/ha and maize intercropped with U. ruziziensis at N levels of 70, 140 and 210 kg/ha.
Land equivalent ratio
The intercropping systems, either with C. spectabilis or U. ruziziensis, showed a higher LER than the sole maize cropping system (Fig. 7). Between the two intercropping systems, the maize + U. ruziziensis intercropping system showed a higher LER with increasing N levels in the top-dressing. Top-dressing N fertilization increased the LERs of the sole maize cropping and maize + U. ruziziensis intercropping systems. The maximum LER value for the former system (1.14) was obtained at the 128 kg/ha N level, whereas linear increments of 0.012 for every 10 kg/ha of N added to the top-dressing fertilization were observed in the latter. In the intercropping system with C. spectabilis, top-dressing N fertilization did not affect the LER, which showed an average value of 1.36.

Fig. 7. LER of intercropped systems as a function of nitrogen levels applied as top-dressing in the average of the two years. M: sole maize; M + Cs: maize intercropped with C. spectabilis; M + Ur: maize intercropped with U. ruziziensis. *P < 0.05; **P < 0.01.
Discussion
Cover crops enhance the sustainability of cropping systems owing to the various ecological benefits generated by their cultivation, including producing straw for soil cover thus mitigate evaporation from the soil and run-off, improve nutrient cycling and weed control (Pacheco et al., Reference Pacheco, Barbosa, Leandro, de Machado, de Assis, Madari and Petter2013; Schipanski et al., Reference Schipanski, Barbercheck, Douglas, Finney, Haider, Kaye, Kemanian, Mortensen, Ryan, Tooker and White2014). Although sustainability in cropping systems is one of the main agricultural goals in many countries (United Nations, 2021), these benefits alone do not ensure the implementation of intercropped systems by farmers. This is because cover crops do not provide direct economic benefits. Thus, intercropping systems involving cover crops are a better alternative to the sole cultivation of cover crops since they combine the ecosystem benefits of cover crops and the economic benefits of grain production in the same area. However, an increased acceptance of cover crop cultivation should not affect the GY.
Our results showed that, although the cover crops do not interfere with the growth of maize, in the presence of C. spectabilis the ears were located higher up on the stem, in the two years. Thus, the fact that maize intercropped with C. spectabilis has ears in a higher region of the stem without necessarily having excessive growth of the plant is beneficial, because it avoids lodging and facilitates the mechanized harvesting operation, especially in intercropping systems, allowing the harvest of only the ear and not of parts of cover plants, which could lead to problems in the harvesters.
The increase in the variables related to plant growth as a function of nitrogen fertilization is justified by the N soil solution availability increase, favouring the uptake by plants, which use this element in their metabolism. Thus, the increase in leaf N content in the first year in an intercropping system agrees with that found in the literature (Souza et al., Reference Souza, Buzetti, Teixeira Filho, Andreotti, Sá and Arf2011; dos Santos et al., Reference dos Santos, Aquino, Nunes and Xavier2013; Pivetta et al., Reference Pivetta, Jordão, Larini, Luchese, Gasparin, Debuss, Carvalho and Silva2019).
In the second year, the results of leaf N content showed higher requirement for nitrogen fertilization by maize intercropped with U. ruziziensis, and in the absence of the nutrient as top-dressing, the only value below the range considered adequate in the diagnostic leaf of maize, from 27 to 35 g/kg (Cantarella and Raij, Reference Cantarella, Raij, Raij, Cantarella, Quaggio and Furlani1997), occurred in this intercropping. Therefore, for the leaf N content of maize intercropped with U. ruziziensis to reach the recommended level, N fertilization of at least 36 kg/ha needs to be applied.
The increase in stem diameter as a function of N levels in both years is consistent with that observed by Gonçalves et al. (Reference Gonçalves, Silva and Brandão2016) and Marini et al. (Reference Marini, Guimarães, Dartora, do Lana and Pinto Júnior2015). For being an organ with structural and reserve function, a larger stem diameter is advantageous to avoid lodging of plants and contribute with translocated photoassimilates to grain filling (da Silva Brum et al. Reference da Silva Brum, dos Cunha, Stecca, Grando and Martin2016).
The results of PCA confirmed the direct relationship between stem diameter and the variables of grains and ear, confirming the capacity of the stem to translocate reserves for grain filling. In addition, especially in the first year, it was possible to observe the greater dependence of nitrogen fertilization for maize intercropped with U. ruziziensis in comparison with other cropping systems.
The negative loadings of all variables in PC1 indicate direct correlations between the variables, showing a relationship between stem diameter and the variables of ear and grains, since thicker stems indicate greater reserve of photoassimilates and, consequently, provide better development of grains and ear. Therefore, the higher the agronomic performance of maize, the higher the GY, stem diameter, grain mass per ear, thousand-grain weight, number of grains per ear, number of grains per row and leaf N content.
A similar result regarding the lower GY for maize intercropped with U. ruziziensis compared to sole maize was verified by Arf et al. (Reference Arf, Meirelles, Portugal, Buzetti, Sá and Rodrigues2018). Souza et al. (Reference Souza, Couto Júnior, de Flôres, Mingotte and Lemos2019), on the other hand, observed no differences between sole maize and intercropped with U. ruziziensis and C. spectabilis for GY, as occurred in the second year of our study.
The differences reported in the literature for GY are related to crop management, intercropping modalities used and climate and soil factors. Thus, the favourable conditions of soil fertility possibly met the nutritional needs of maize in the second year of our study, avoiding losses in GY when the cover plant was present, especially U. ruziziensis. The study plots were allocated to the same location during the two years of experimentation. Hence, while the maize crops did not benefit from the greater amounts of N from the cover crop residues during the first year, they did so during the second year. Deienno et al. (Reference Deienno, Souza, Coelho and Lemos2021) reported that the intercropping systems of maize with C. spectabilis and U. ruziziensis left up to 66 and 94% more N, respectively, in the soil than the N left by the sole maize cropping system. This suggests that the higher amounts of N left in the soil after the first year of the experiment helped mitigate the lower availability of N for maize intercropping systems during the second year. Duete et al. (Reference Duete, Muraoka, da Silva, Trivelin and Ambrosano2008) found that, although the use of 39% of nitrogen fertilizer in the form of N15 urea by maize, in an Oxisol, the main source of the nutrient for the crop was the soil.
The quadratic or linear increase as a function of the increase of N of the variables number of grains per row, number of grains per ear, thousand-grain weight and GY in the first year, as well as these variables plus the grain mass per ear in the second year, occurred due to the greater availability for absorption of the nutrient by the plants. This is because N acts on the synthesis of proteins, hormones, chlorophylls and other compounds important for plant development; therefore, vegetative and reproductive aspects are favoured by the increase in the nutrient (Costa et al., Reference Costa, Andreotti, Lopes, Santos and Pariz2014).
Regarding to GY in the first year, the top-dressing nitrogen fertilization in sole maize in the present study is consistent with the value recommended by Cantarella and Raij (Reference Cantarella, Raij, Raij, Cantarella, Quaggio and Furlani1997), close to 100 kg/ha of N. On the other hand, the intercropped systems showed greater demand for nitrogen to obtain similar yields compared to sole maize. This occurs because in intercropped systems, cover crops take advantage of the applied N for maize top-dressing fertilization. This is even more severe in intercropping systems where cover crops are sown simultaneously with maize. In this situation, at the time of top-dressing fertilization, cover crops have a high development and are able to compete with maize for the nutrient.
In addition, among the intercropping systems, the presence of U. ruziziensis requires N levels even higher than that of C. spectabilis to obtain maximum yields. This is because, in addition to the requirement for N of the grass being higher than that of the legume, it does not have a contribution of BNF for N supply. Thus, the intercropping of maize with U. ruziziensis, when sown simultaneously, must receive top-dressing nitrogen fertilization with higher N levels compared to sole maize, or even the splitting of N, increasing fertilization efficiency (Du et al., Reference Du, Xi and Kong2019). Almeida et al. (Reference Almeida, Favarin, Otto, Pierozan, Oliveira, Tezotto and Lago2017) observed in the intercropping of maize with U. ruziziensis, with simultaneous sowing, competition of the grass with maize up to the N level of 100 kg/ha. However, when the intercropping occurs simultaneously with top-dressing nitrogen fertilization in maize, there is no competition of the forage species with maize for abiotic factors, including N (Borghi et al., Reference Borghi, Crusciol, Trivelin, Nascente, Costa and Mateus2014).
Several studies have shown that there is a reduction in maize yield when it is intercropped (Pariz et al., Reference Pariz, Andreotti, Azenha, Bergamaschine, Mello and Lima2011; Crusciol et al., Reference Crusciol, Nascente, Mateus, Borghi, Leles and Santos2013; Cambaúva et al., Reference Cambaúva, Leal and Lemos2019). Cambaúva et al. (Reference Cambaúva, Leal and Lemos2019) observed that the intercropping of maize + Crotalaria juncea reduces maize GY. However, for C. spectabilis, the same used in the present study, the GY of maize was equal to that obtained with sole maize. According to the authors, the reduction in the yield of maize intercropped with C. juncea is due to the greater competition of this plant with maize compared to C. spectabilis, due to its greater plant height (Cambaúva et al., Reference Cambaúva, Leal, Coelho and Lemos2022).
Intercropping systems can also promote competition for water because the leaf area index in intercropped systems is higher than that in the sole crop systems, increasing transpiration (Martin-Guay et al. Reference Martin-Guay, Paquette, Dupras and Rivest2018; Cambaúva et al., Reference Cambaúva, Leal and Lemos2019). While assessing the temporal variability of soil moisture in the sole maize and maize + C. spectabilis intercropping systems, Trevisan et al. (Reference Trevisan, Silva, Fontanetti, Souza Gallo and França Guimarães2021) observed lower soil moisture during some periods in the intercropped system, reducing water availability for maize. Silva et al. (Reference Silva, Andrade Júnior, Cardoso and Araújo Neto2020) found that soil moisture can be up to 10% lower in the maize + U. ruziziensis intercropping system than in the sole maize cropping system, especially during the grain-filling stage. It is noteworthy that in regions with high-intensity rainfall, a higher leaf area index may mitigate the impact of rain on the soil, reducing surface runoff and the potential for soil erosion, especially at the beginning of maize development.
Another benefit of intercropping is the higher total yield compared to sole cropping of maize and cover crops. This was verified by the LERs obtained in the present study, in which the intercropping systems presented LER values that were 35% higher than those of the sole cropping system, thereby indicating that for a total production similar to that of the intercropped systems, the sole cropping area should be increased by more than 35%. This value was similar to that observed by Martin-Guay et al. (Reference Martin-Guay, Paquette, Dupras and Rivest2018), who found that the LER of intercropping was 1.30, increasing the gross energy produced per area by 38%.
Depending on the cropping system, fertilization management is essential for increasing land yield. The LER of the sole maize increased by up to 14% and the LER of the maize + U. ruziziensis intercropping system increased at a rate of 0.023 for every 10 kg/ha of N. These results were similar to those observed by Salama et al. (Reference Salama, Nawar and Khalil2022) who evaluated the effect of intercropping maize with cowpea and N fertilization management on LER. They found that some intercropping configurations increased the LER by more than 30% and that better N fertilization management increased the LER by more than 25%. In the present study, the highest LER of the sole maize was obtained under 128 kg/ha N level, a value close to that recommended for maize in this region, which is 140 kg/ha of N (Cantarella and Raij, Reference Cantarella, Raij, Raij, Cantarella, Quaggio and Furlani1997).
The results obtained here suggest that the maize intercropping, associated with an adequate management of top-dressing nitrogen fertilization, may be a great strategy for farmers and technicians to increase sustainability in their crops. In addition to the ecosystem benefits of intercropping, our findings show that it is possible to associate the same land area with more production and more than one economic activity. This was evidenced by the 35% increase in the LER using intercropped systems and top-dressing fertilization, generating scientific information to prevent the opening of new cultivation areas in regions considered marginal, either due to climate or environmental issues.
Conclusion
Maize intercropping with U. ruziziensis and C. spectabilis increases the requirement for N fertilization to obtain an agronomic performance similar to that of the sole maize cropping system. This is especially true for intercropping with U. ruziziensis, where the increase in GY as a function of N levels is linear, with an average increase of 120 kg/ha for every 10 kg/ha of N applied. For other intercropping, N fertilization can either increase the GY quadratically or not interfere with this attribute. Compared to a sole maize crop, intercropping and N fertilization help increase land yield, with an increase in the LER by more than 35%. We determine that intercropping of maize with U. ruziziensis or C. spectabilis and with an adequate top-dressing N management is a potential strategy to assist farmers in using a more conservation-based cropping system. This will increase yields and promote a more efficient land use.
Acknowledgements
The present study builds on the PhD thesis of Stefany Silva de Souza published on 9 July 2020 (available from the São Paulo State University repository).
Author contributions
Conceptualization: SSS, JAF and LBL; data curation: SSS, JAF and APC; formal analysis: SSS, JAF and JMD; funding acquisition: SSS, LBL and GSR; investigation: SSS, LBL and GSR; methodology: SSS, JAF and LBL; project administration: LBL; resources: LBL; supervision: LBL and GSR; validation: APC and GSR; visualization: JMD and LBL; writing – original draft: SSS and APC; writing – review and editing: LBL and GSR
Financial support
This study was supported by Coordenação de Aperfeiçoamento de Pessoal de Nivel Superior (CAPES), finance code 001.
Conflict of interest
The authors declare there are no conflicts of interest.