Introduction
The impact of grain particle size on feedlot performance has been studied extensively in diets containing dent maize, and despite advantages to starch digestion and milk yield from feeding dairy cows with fine ground maize (Moe and Tyrrell, Reference Moe and Tyrrell1977; Mitzner et al., Reference Mitzner, Owen and Grant1994; Knowlton et al., Reference Knowlton, Glenn and Erdman1998; Firkins et al., Reference Firkins, Eastridge, St-Pierre and Noftsger2001; Rémond et al., Reference Rémond, Cabrera-Estrada, Champion, Chauveau, Coudure and Poncet2004), few studies reported positive outcomes to fine grinding of grain on growth performance of Bos taurus beef cattle fed high-concentrate diets (Galyean et al., Reference Galyean, Wagner and Owens1979; Turgeon et al., Reference Turgeon, Brink and Britton1983; Secrist et al., Reference Secrist, Hill, Owens and Welty1995; Swanson et al., Reference Swanson, Islas, Carlson, Goulart, Gilbery and Bauer2014; Schwandt et al., Reference Schwandt, Wagner, Engle, Bartle, Thomson and Reinhardt2016). Nevertheless, McAllister et al. (Reference McAllister, Phillippe, Rode and Cheng1993) and Ramos et al. (Reference Ramos, Champion, Poncet, Mizubuti and Nozière2009) reported that starch digestion in vitro and in sacco were greater for smaller (0.25–1.3 mm) compared with larger (2.00–3.00 mm) maize particle sizes.
According to the survey conducted by Schwandt et al. (Reference Schwandt, Thomson, Bartie and Reinhard2015), average particle size of hammermill ground maize used in Midwestern United Stated feedlots was 1.81 mm with a range of 1.17–3.56 mm. Flint is the primary maize type fed in feedyards in South America, especially in Brazil, with processing methods varying from fine grinding (Millen et al., Reference Millen, Pacheco, Arrigoni, Galyean and Vasconcelos2009) to coarse cracking (Oliveira and Millen, Reference Oliveira and Millen2014). However, research investigating the impacts of grinding flint maize grain on nutrient digestibility and growth performance of finishing Bos indicus beef cattle are still limited (Carareto et al., Reference Carareto, Santos, Mourao, Pedroso, Sitta, Soares, Paula, Marques and Soares2011). In turn, steam flaking flint maize yields greater benefits to net energy content of the grain and cattle growth performance compared with flaking dent maize (Gouvêa et al., Reference Gouvêa, Batistel, Souza, Chagas, Sitta, Campanili, Galvani, Pires, Owens and Santos2016; Marques et al., Reference Marques, Chagas, Owens and Santos2016). Hence, it is expected that reducing grain particle size may further increase starch digestion and growth performance of finishing beef cattle fed flint maize. However, grinding maize too finely can result in rumen fermentation, leading to acidosis and decreased growth performance (Brown and Lawrence, Reference Brown and Lawrence2010; Lundy et al., Reference Lundy, Doran, Vermerr, Loy and Hansen2015).
Rumen fermentation characteristics and growth performance of feedlot cattle can also be enhanced through feeding certain additives such as monensin (Butaye et al., Reference Butaye, Devriese and Haesebrouck2003; Duffield et al., Reference Duffield, Merrill and Bagg2012) and more recently through feeding certain essential oils or their blends (Khiaosa-ard and Zebeli, Reference Khiaosa-ard and Zebeli2013; Khorrami et al., Reference Khorrami, Vakili, Mesgaran and Klevenhusen2015). Also, adding exogenous α-amylase has been proposed to optimize starch utilization in feedlot diets but results are inconsistent (Tricarico et al., Reference Tricarico, Abney, Galyean, Rivera, Hanson, McLeod and Harmon2007; DiLorenzo et al., Reference DiLorenzo, Smith, Quinn, May, Ponce, Steinberg, Engstrom and Galyean2011). According to Beauchemin et al. (Reference Beauchemin, Colombatto and Morgavi2004), the lack of interaction of enzymes with the substrate can explain the lack of response in some in vivo studies in which exogenous enzymes were added in cattle diets. The combination of a specific blend of essential oils and exogenous α-amylase resulted in greater average daily gain (ADG) and carcass weight compared with monensin, primarily by increasing energy intake and the amount of nutrient digested in the total tract of finishing Nellore bulls (Meschiatti et al., Reference Meschiatti, Gouvêa, Pellarin, Batalha, Biehl, Acedo, Dórea, Tamassia, Owens and Santos2019). The combination of these two additives could circumvent concerns regarding the use of antibiotics in commercial beef production (Jouany and Morgavi, Reference Jouany and Morgavi2007; de Souza et al., Reference de Souza, Cooke, Schubach, Brandão, Schumaher, Prado, Marques and Bohnert2018) and should be further evaluated.
Based on this information, it was hypothesized that reducing the particle size of flint ground maize could improve starch digestion and growth performance of finishing beef cattle, and that the extent of improvement on growth performance would be greater with the combination of essential oils and exogenous α-amylase due to greater interaction of exogenous enzyme with the substrate. Thus, the current study evaluated the growth performance and carcass characteristics of finishing Nellore bulls (Expt 1) and nutrient digestibility and ruminal fermentation characteristics of Nellore steers (Expt 2) fed flint maize ground to two different particle sizes (medium v. coarse), added with monensin or the combination of essential oils and exogenous α-amylase.
Materials and methods
Experiment 1. Growth performance and carcass characteristics
Animals, housing and experimental procedures
At the start of the study, 256 Nellore bulls [360 ± 11.7 kg initial body weight (BW)], sourced commercially, were weighed individually after 16 h of feed and water withdrawal, vaccinated against clostridiosis (3 ml s.c.; Sintoxan Polyvalente, Merial Saúde Animal Ltda, Paulínia, Brazil) and dewormed (3.15 g/100 ml ivermectin at 1 ml/50 kg BW; Ivomec Gold; Merial Saúde Animal Ltda). Thereafter, bulls were blocked by initial BW (12 weight blocks) and assigned to 48 pens (12 pens/treatment). Pens had different dimensions and characteristics so the number of bulls assigned to each pen varied as follows: 28 pens had concrete floors and partial roof cover (32 m2 and five bulls/pen; weight block 1 to 7), 12 pens had soil floors and no roof cover (84 m2 and six bulls/pen; weight block 8 to 10) and eight pens had soil floors and no roof cover [120 m2; four pens with five bulls/pen (weight block 11) and four pens with six bulls/pen (weight block 12)]. All bulls had free choice access to fresh water during the entire study.
Treatments were assigned randomly to pens within each weight block, in a 2 × 2 factorial arrangement, consisting of two maize particle sizes [medium ground maize (1.66 mm; MG) or coarse ground maize (2.12 mm; CG)] with added sodium monensin [26 mg/kg of dry matter (DM); MON] or the combination of a blend of essential oils (BEO) with an exogenous α-amylase (AM; 90 and 560 mg commercial product/kg of DM, respectively).
The basal diet (Table 1) was formulated to meet the requirements of finishing Nellore bulls as specified by NRC (1996). Sodium monensin (Poulcox), the positive control in the current trial, was obtained from Biovet Joint Stock Company, Sofia, Bulgaria. The blend of essential oils (CRINA Ruminants) containing thymol, eugenol, limonene and vanillin on an organic carrier (McIntoch et al., Reference McIntoch, Williams, Losa, Wallace, Beever and Newbold2003) and the exogenous α-amylase produced by Bacillus licheniformis (Ronozyme RumiStar) were provided by DSM Nutritional Products, Basel, Switzerland. The dose level employed for BEO was based on previous studies (Kung et al., Reference Kung, Williams, Schmidt and Hu2008; Meyer et al., Reference Meyer, Erickson, Klopfenstein, Greenquist, Luebbe, Williams and Engstrom2009). The exogenous enzyme α-amylase, a granular amylase formulation with amylase activity of 600 Kilo Novo units (KNU) per g was added to the appropriate total mixed rations (TMR) to achieve 336 KNU of amylase activity per kg of TMR DM (DiLorenzo et al., Reference DiLorenzo, Smith, Quinn, May, Ponce, Steinberg, Engstrom and Galyean2011; Meschiatti et al., Reference Meschiatti, Gouvêa, Pellarin, Batalha, Biehl, Acedo, Dórea, Tamassia, Owens and Santos2019). One KNU is defined as the amount of enzyme released in a two-step reaction 6 µmol of p-nitrophenol per min from 1.86 mm 4.6-ethylidene-G7-nitrophenyl-maltoheptaoside at pH 7.0 and 37 °C (DiLorenzo et al., Reference DiLorenzo, Smith, Quinn, May, Ponce, Steinberg, Engstrom and Galyean2011; Meschiatti et al., Reference Meschiatti, Gouvêa, Pellarin, Batalha, Biehl, Acedo, Dórea, Tamassia, Owens and Santos2019). The inclusion concentration of α-amylase was selected based on previous studies (Klingerman et al., Reference Klingerman, Hu, McDonell, DerBedrosian and Kung2009; Gencoglu et al., Reference Gencoglu, Shaver, Steinberg, Ensink, Ferraretto, Bertics, Lopes and Akins2010; Andreazzi et al., Reference Andreazzi, Pereira, Reis, Pereira, Morais Júnior, Acedo, Hermes and Cortinhas2018; Meschiatti et al., Reference Meschiatti, Gouvêa, Pellarin, Batalha, Biehl, Acedo, Dórea, Tamassia, Owens and Santos2019).
Table 1. Ingredients and chemical composition of the diet used (Expt 1 and 2)
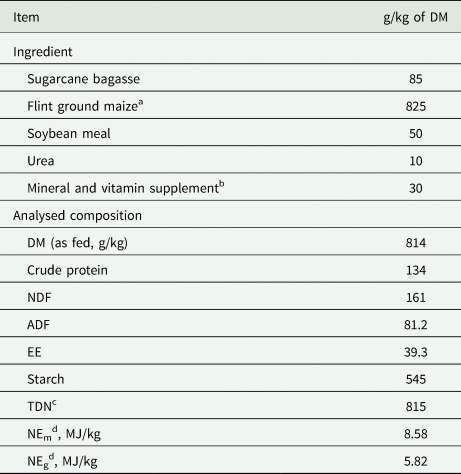
a Flint maize grain was processed through a hammer mill (ML 100-A, Lucato, Indústria e Comercial Lucato, Limeira, SP, Brazil) to medium ground (MG; 1.66 mm) or coarse ground (CG; 2.12 mm).
b Feed additives were incorporated into the mineral and vitamin supplement to reach the treatments: MON = sodium monensin (26 mg/kg of DM); BEO + AM = blend of essential oils + exogenous α-amylase (90 and 560 mg commercial product/kg of DM respectively). Sodium monensin (Poulcox) was from Biovet Joint Stock Company, Sofia, Bulgaria. The blend of essential oils (CRINA Ruminants) and the exogenous α-amylase (Ronozyme RumiStar) were provided by DSM Nutritional Products, Basel, Switzerland. Mineral and vitamin supplement was composed (DM basis) of 140 g/kg Ca, 16 g/kg P, 36 g/kg S, 20 g/kg Mg, 34 g/kg K, 56 g/kg Na, 8 mg/kg Co, 540 mg/kg Cu, 6.7 mg/kg Cr, 27.5 mg/kg I, 1.070 mg/kg Mn, 6.7 mg/kg Se, 2000 mg/kg Zn, 168 000 IU/kg vitamin A, 17 000 IU/kg vitamin D3, 1740 IUI/kg vitamin E, 90 mg/kg biotin, 2.7 × 109 CFU/kg Saccharomyces cerevisiae. Manufactured by DSM Nutritional Products, São Paulo, Brazil.
c TDN were estimated from the equations of Weiss et al. (Reference Weiss, Conrad and St-Pierre1992) using the analysed ingredient composition and assuming processing adjustment factor of 1.00 for flint ground maize (NRC, 2001).
d The net energy for maintenance (NEm) and gain (NEg) were estimated with the equations proposed by the NASEM (2016; empirical solution type) with addition of ionophore and using the TDN values from Weiss et al. (Reference Weiss, Conrad and St-Pierre1992) obtained by NRC (2001).
The flint maize grain was processed through a hammer mill (ML 100-A, Lucato, Indústria e Comercial Lucato, Limeira, SP, Brazil) at a commercial feed mill (Coplacana, Cooperativa dos Plantadores de Cana do Estado de São Paulo, Piracicaba, SP, Brazil) and delivered every 7 days to the experimental feedlot. Maize samples were taken from every load (one sample/load and a total of 14 samples) and particle distribution was analysed according to Yu et al. (Reference Yu, Huber, Santos, Simas and Theurer1998) using sieves with 6.0, 3.5, 2.0, 1.25 mm2 pores (Produtest T Model; Telastem Peneiras para Análises Ltda, São Paulo, SP, Brazil). The residue retained in the bottom (<1.25 mm) was assumed to represent a sieve with pores of 0.625 mm2. Average particle size, reported in terms of geometric mean of the diameter (d gw), log-normal standard deviation (S gw), surface area (cm2/g) and particles/g were calculated using the equations of Baker and Herrman (Reference Baker and Herrman2002) (Table 2 and Fig. 1).

Fig. 1. Cumulative mass distribution of ground maize grain. Flint maize grain was processed through a hammer mill (ML 100-A, Lucato, Indústria e Comercial Lucato, Limeira, SP, Brazil). Maize grain particle distribution was determined according to Yu et al. (Reference Yu, Huber, Santos, Simas and Theurer1998) using sieves with 6.0, 3.5, 2.0, 1.25 mm square pores (Produtest T Model; Telastem Peneiras para Análises Ltda, São Paulo, SP, Brazil). Particles <1.25 mm was assumed to represent a 0.625 mm square pores sieve. Geometric mean of the diameter (d gw) was calculated using the equations of Baker and Herrman (Reference Baker and Herrman2002).
Table 2. Maize grain particle size distribution (g/kg; Expt 1 and 2)

a Flint maize grain was processed through a hammer mill (ML 100-A, Lucato, Indústria e Comercial Lucato, Limeira, SP, Brazil) to medium (MG) or coarse ground (CG).
b Maize grain particle distribution was determined according to Yu et al. (Reference Yu, Huber, Santos, Simas and Theurer1998) using sieves with 6.0, 3.5, 2.0, 1.25 mm square pores (Produtest T Model; Telastem Peneiras para Análises Ltda, São Paulo, SP, Brazil). Particles <1.25 mm were assumed to represent a 0.625 mm square pores sieve. Geometric mean diameter (d gw), log-normal standard deviation (S gw), surface area (cm2/g) and particles/g were calculated using the equations of Baker and Herrman (Reference Baker and Herrman2002).
Bulls were fed an adaptation TMR diet during the first 15 days of the experiment and finishing TMR from day 16 to 99. During the adaptation period the dietary concentration of the sugarcane bagasse was reduced from 250 to 150 g/kg (50 g/kg every 5 days; DM basis) and replaced with ground maize accordingly. From day 16 to 99, all bulls received the finishing diet [85 g/kg sugarcane bagasse plus 915 g/kg concentrate (DM basis; Table 1)]. Treatments were added into diets during the entire experiment (99 days).
Feed additives and enzymes were incorporated into the mineral–vitamin supplement that was included as 30 g/kg of the dietary DM (Table 1). These mineral–vitamin supplements with the appropriate additives and enzymes were produced at a commercial feed mill following all the manufacturing standards for quality and guaranteed levels (DSM Nutritional Products Brazil S.A., Mairinque, SP, Brazil).
Each treatment diet was mixed individually using a feed wagon (Siltomac S-2.3; Indústria de Implementos Agrícolas Siltomac Ltda, São Carlos, SP, Brazil) and delivered to each pen once daily at 08.00 h. The feed wagon was cleaned carefully after delivering each ration to avoid cross-contamination. Feed bunks were evaluated visually each day and managed for a maximum of 30 g/kg of orts that were removed weekly, weighed and sampled. Feed and ort samples from each pen were dried at 105 °C for 24 h to determine DM content and to calculate dry matter intake (DMI).
On day 70, individual fresh faecal samples were collected from the pen floor (three randomly bulls/pen) before feeding. Approximately 50 g of faeces were collected from the inside of each faecal pat, using sterile cups. Faeces were pooled by pen and immediately frozen (−20 °C) for further faecal starch analyses.
At the end of the 99-day feeding trial, bulls were weighed following 16 h of feed and water deprivation and transported (8.4 km; approximately 20 min) in a commercial cattle truck (11 trucks with 22 bulls/truck and 1 truck with 14 bulls) to a commercial slaughterhouse (Friuna Alimentos Ltda, Piracicaba, Brazil). ADG was calculated using the initial and final shrunk BW. Feed efficiency (G : F) was calculated as the ratio of ADG to DMI. After slaughtering, hot carcass weights (HCWs) were collected and dressing percent calculated (HCW to final shrunk BW ratio). Subcutaneous fat thickness and longissimus muscle (LM) area were measured at the 12th rib after a 24 h chill (2 °C), using digital calliper and a numbered grid, respectively.
Feed analysis and calculations
Samples of ingredients collected every 10 days were dried in a forced-air oven at 55 °C for 72 h, and ground through a 1-mm screen using a Wiley-type mill (MA-680; Marconi Ltda, Piracicaba, SP, Brazil). Samples were analysed for DM at 105 °C (method 930.15; AOAC, 1986), ash (method 942.05; AOAC, 1986), ether extract [(EE); method 920.85; AOAC, 1986], ash-corrected neutral detergent fibre [(aNDF); Van Soest et al., Reference Van Soest, Robertson and Lewis1991] using sodium sulphite and heat-stable α-amylase, acid detergent fibre [(ADF); Goering and Van Soest, Reference Goering and Van Soest1970], and nitrogen [(N); Leco FP-528; Leco Corp., St Joseph, MI, USA]. Maize and faecal samples were analysed for starch using a Total Starch K-TSTA kit (Megazyme, Chicago, IL, USA; method 996.11; AOAC, 1986 and method 76-13.01; AACC, 1976). The total digestible nutrients (TDN) values for each diet were estimated according to Weiss et al. (Reference Weiss, Conrad and St-Pierre1992) using a processing factor of 1.00 for ground maize (NRC, 2001). Maize net energy content for maintenance (NEm) and gain (NEg) were estimated from faecal starch concentration (Zinn et al., Reference Zinn, Calderon, Corona, Plascencia, Montaño and Torrentera2007). Total tract starch digestion (TSD) was calculated from faecal starch content according to Zinn et al. (Reference Zinn, Owens and Ware2002). Observed net energy (NE) concentrations of each diet were calculated according to Zinn and Shen (Reference Zinn and Shen1998) using mean values for shrunk BW, DMI and ADG of the bulls in each pen. These calculated NE concentrations were compared with those predicted using the Weiss et al. (Reference Weiss, Conrad and St-Pierre1992) equations for TDN that were converted to NE concentrations using equations from the NASEM (2016) empirical model. Specific details related to feeding procedures, sampling, laboratory analysis and calculations were described by Meschiatti et al. (Reference Meschiatti, Gouvêa, Pellarin, Batalha, Biehl, Acedo, Dórea, Tamassia, Owens and Santos2019).
Experiment 2. Digestibility and ruminal fermentation
Four ruminally cannulated Nellore steers (BW = 594 ± 22.4 kg) were used in a 4 × 4 Latin Square design to evaluate intake, apparent total tract digestibility of nutrients, ruminal parameters and rumen microbial protein synthesis of diets used in Expt 1. Detailed information related to feeding procedures, sample collection, laboratory analysis and calculations for Expt 2 were described by Meschiatti et al. (Reference Meschiatti, Gouvêa, Pellarin, Batalha, Biehl, Acedo, Dórea, Tamassia, Owens and Santos2019). Briefly, steers were housed in individual pens (32 m2; concrete floor and solid roof) and given free access to water during the experiment. Four consecutive periods were conducted, each consisting of 15 days for adaptation to diets and 5 days for sample collection. From day 16 to 20 of each period, steers were monitored every 3 h and total faeces were collected from the concrete floor and analysed for DM content to estimate total faecal production. On day 18 (at 08.00, 14.00, 20.00 and 02.00 h), day 19 (at 10.00, 16.00, 22.00 and 04.00 h) and day 20 (at 12.00, 18.00, 24.00 and 06.00 h), approximately 50 g of faecal samples were also collected directly from the rectum of each steer in a manner that all collections represented one full day. These last samples were composited by period and steer, and analysed for DM, CP, EE, aNDF, ADF, ash and faecal starch as described for Expt 1. Non-fibrous carbohydrate (NFC, g/kg DM) was calculated as: NFC = 1000 − (CP, g/kg + aNDF, g/kg + Ash, g/kg + EE, g/kg). Total apparent digestibility was calculated using the equation: Nutrient digestibility, g/kg DM = ([Nutrient intake, kg − Faecal nutrient output, kg]/Nutrient intake, kg).
On day 16, rumen fluid samples (50 ml) were obtained from each steer via ruminal cannula at 0, 2, 4, 6, 8, 10, 12, 14, 16, 18, 20, 22 and 24 h after feeding as described by Danes et al. (Reference Danes, Chagas, Pedroso and Santos2013). Ruminal fluid samples were squeezed through four layers of cheesecloth and the pH was immediately recorded using a portable pH meter (Digimed Model DM22, Digicrom Analítica Ltda, São Paulo, SP, Brazil). Sub-samples were later analysed for volatile fatty acids (VFA) according to Palmquist and Conrad (Reference Palmquist and Conrad1971) and ammonia nitrogen (NH3-N) according to Chaney and Marbach (Reference Chaney and Marbach1962).
Spot samples of urine were spontaneously collected into sterile plastic cups on day 17 of each period, 4 h after feeding. The concentrations of creatinine and uric acid in urine were determined using commercial kits (Bioclin – Belo Horizonte, MG, Brazil and CELM – Compania Equipadora de Laboratórios Modernos, São Caetano do Sul, SP, Brazil) and readings were performed using an automatic biochemistry analyser (Automatic System of Biochemistry SBA-200 – CELM). Allantoin concentrations were determined as described by Chen and Gomes (Reference Chen and Gomes1992). The absorbed purines were calculated from excretion of purine derivatives according to Verbic et al. (Reference Verbic, Chen, Macleod and Ørskov1990). Urine volume was estimated from the concentration of creatinine in the urine (Chizzotti et al., Reference Chizzotti, Valadares Filho, Valadares, Chizzotti and Tedeschi2008) and ruminal synthesis of nitrogenous compounds (N mic, g/day) was calculated based on the absorbed microbial purine, according to the equation proposed by Barbosa et al. (Reference Barbosa, Valadares, Valadares Filho, Pina, Detmann and Leão2011). Microbial nitrogen efficiency was calculated as the ratio of g microbial nitrogen to kg of TDN ingested.
Statistical analysis
Data in Expt 1 were analysed using the MIXED procedure of SAS (version 9.3, SAS Inst. Inc., Cary, NC, USA) as a randomized complete block design with a 2 × 2 factorial arrangement of treatments. Pen was the experimental unit and the Satterthwaite approximation method was used to determine the correct denominator degrees of freedom for the test of fixed effects. The statistical model included the fixed effects of maize particle size, feed additive and particle size × feed additive interaction. Pen (maize particle size × feed additive interaction), bull (pen), weight of block and pen type were considered random variables for IBW, FBW, ADG and carcass characteristics. Bull (pen) was not included as random effect for statistical analysis of intake, feed efficiency, faecal starch, TSD and maize and diet net energy concentrations.
All data in Expt 2 were analysed as a 4 × 4 Latin Square design with a 2 × 2 factorial arrangement of treatments, using the MIXED procedure of SAS (version 9.3, SAS Inst. Inc., Cary, NC, USA). The statistical model for intake and total apparent digestibility of nutrients, nitrogen metabolism and microbial protein synthesis included the fixed effect of maize particle size, feed additive and maize particle size × feed additive interaction. The steers and periods of Latin Square were included as random term. Steer within period was the experimental unit. Ruminal fermentation characteristics (VFA, N-NH3 and pH) were analysed as repeated measures over time. The statistical model included fixed effect of maize particle size, feed additive, time, maize particle size × feed additive and maize particle size × feed additive × time interactions. Time was the repeated measure and the subject was animal (maize particle size × feed additive). The covariance structure type used was compound symmetry because it had the smallest value for Akaike's information criterion. The Kenward–Roger approximation was used to determine the denominator degrees of freedom that was used for testing fixed effects. When no interaction between maize particle size × feed additive × time were observed, this statement and time were dropped off the model and averages across 24 h period were used for statistical analysis to simplify data interpretation and reporting.
Results from Expt 1 and 2 were all reported as least-squares means. When no significant interactions were detected, main effects of maize particle size and feed additives were examined. Differences between each factor were tested by the F-test. When significant interactions (P < 0.05) were observed for a trait, means were separated using PDIFF option. Differences were declared significant when P ⩽ 0.05, whereas trends were discussed when 0.05 < P ⩽ 0.10.
Results
Experiment 1. Growth performance and carcass characteristics
Effects of maize particle size × feed additive interactions were not detected (P ⩾ 0.157) for final BW, DMI, ADG and G : F (Table 3). Feed additive effect was detected for DMI, which was 7.4% greater for bulls fed BEO + AM v. MON (P = 0.001). A tendency for maize particle size effect was detected for final BW and ADG (P ⩽ 0.096), which was greater (9 kg and 100 g/day, respectively) for bulls fed CG than bulls fed MG maize. Neither maize particle size nor feed additive impacted feed efficiency (P ⩾ 0.207).
Table 3. Performance and carcass characteristics of Nellore bulls finished in feedlot and provided, in a 2 × 2 factorial arrangement, diets containing flint maize ground to medium (1.66 mm; MG) or coarse (2.12 mm; CG) particle sizes, added with sodium monensin (26 mg/kg of DM; MON) or a blend of essential oils + exogenous α-amylase (90 and 560 mg commercial product/kg of DM respectively; BEO + AM) in Expt 1a

BW, body weight; DMI, dry matter intake; ADG, average daily gain; G : F, feed efficiency; LM, longissimus muscle.
a Randomized complete block design; 12 pens/treatment; five or six bulls/pen. Feeding period lasted 99 days.
b Flint maize grain was processed through a hammer mill (ML 100-A, Lucato, Indústria e Comercial Lucato, Limeira, SP, Brazil). Geometric mean diameter (mm) was calculated using the equations of Baker and Herrman (Reference Baker and Herrman2002).
c Sodium monensin (Poulcox) was from Biovet Joint Stock Company, Sofia, Bulgaria. The blend of essential oils (CRINA Ruminants) and the exogenous enzyme [α-amylase (Ronozyme RumiStar)] were provided by DSM Nutritional Products, Basel, Switzerland.
d Dressing percentage/100.
A maize particle size × feed additive interaction was detected for HCW (P = 0.013; Table 4). The HCW was 11 kg heavier for bulls fed BEO + AM v. MON in diets containing CG (P = 0.007), but not MG particle size (P = 0.420). Bulls fed BEO + AM in diets containing CG particle size had 14 kg heavier carcass weight compared with bulls fed the same feed additive (BEO + AM) and 11 kg heavier than bulls fed MON in diets containing MG particle size (P ⩽ 0.011). Maize particle size effect was detected for LM area, which was 4.1% greater for bulls fed CG than MG maize (P = 0.023).
Table 4. HCW of Nellore bulls finished in feedlot and provided, in a 2 × 2 factorial arrangement, diets containing flint maize ground to medium (1.66 mm; MG) or coarse (2.12 mm; CG) particle sizes, added with sodium monensin (26 mg/kg of DM; MON) or a blend of essential oils + exogenous α-amylase (90 and 560 mg commercial product/kg of DM respectively; BEO + AM) in Expt 1a

a Randomized complete block design; 12 pens/treatment; five or six bulls/pen. Feeding period lasted 99 days.
b Flint maize grain was processed through a hammer mill (ML 100-A, Lucato, Indústria e Comercial Lucato, Limeira, SP, Brazil). Geometric mean diameter (mm) was calculated using the equations of Baker and Herrman (Reference Baker and Herrman2002).
c Sodium monensin (Poulcox) was from Biovet Joint Stock Company, Sofia, Bulgaria. The blend of essential oils (CRINA Ruminants) and the exogenous enzyme [α-amylase (Ronozyme RumiStar)] were provided by DSM Nutritional Products, Basel, Switzerland.
No effects of maize particle size × feed additive interactions or maize particle size (P ⩾ 0.205), but effects of feed additive (P ⩽ 0.009) were detected for starch intake, faecal starch, TSD and maize net energy concentration (Table 5). Bulls fed the combination of BEO + AM had 7.4% greater starch intake than bulls fed MON (P = 0.001). Faecal starch concentrations averaged 24% greater (P = 0.009) leading to lower total tract starch digestibility (3.4%; P = 0.009) for bulls fed BEO + AM compared with MON. Based on faecal starch alone, feeding MON increased estimated maize NEm and maize NEg by 5.4 and 6.5%, respectively, compared with BEO + AM (P = 0.009).
Table 5. Starch intake, faecal starch and total TSD of Nellore bulls finished in feedlot and provided, in a 2 × 2 factorial arrangement, diets containing flint maize ground to medium (1.66 mm; MG) or coarse (2.12 mm; CG) particle sizes, added with sodium monensin (26 mg/kg of DM; MON) or a blend of essential oils + exogenous α-amylase (90 and 560 mg commercial product/kg of DM respectively; BEO + AM) in Expt 1a

a Randomized complete block design; 12 pens/treatment; five or six bulls/pen. Feeding period lasted 99 days.
b Flint maize grain was processed through a hammer mill (ML 100-A, Lucato, Indústria e Comercial Lucato, Limeira, SP, Brazil). Geometric mean diameter (mm) was calculated using the equations of Baker and Herrman (Reference Baker and Herrman2002).
c Sodium monensin (Poulcox) was from Biovet Joint Stock Company, Sofia, Bulgaria. The blend of essential oils (CRINA Ruminants) and the exogenous enzyme [α-amylase (Ronozyme RumiStar)] were provided by DSM Nutritional Products, Basel, Switzerland.
d Calculated using the starch content in the diet and DMI data of each pen.
e TSD (coefficient) = total tract starch digestion was estimated according to Zinn et al. (Reference Zinn, Owens and Ware2002).
f Maize net energy for maintenance (NEm) and gain (NEg) were estimated according to Zinn et al. (Reference Zinn, Calderon, Corona, Plascencia, Montaño and Torrentera2007).
Effects of maize particle size × feed additive interactions, maize particle size and feed additive were not detected (P ⩾ 0.120) for observed dietary NE values estimated based on intake and growth performance (Table 6).
Table 6. Observed dietary net energy (NE) concentration of Nellore bulls finished in feedlot and provided, in a 2 × 2 factorial arrangement, diets containing flint maize ground to medium (1.66 mm; MG) or coarse (2.12 mm; CG) particle sizes, added with sodium monensin (26 mg/kg of DM; MON) or a blend of essential oils + exogenous α-amylase (90 and 560 mg commercial product/kg of DM respectively; BEO + AM) in Expt 1a

a Randomized complete block design; 12 pens/treatment; five or six bulls/pen. Feeding period lasted 99 days.
b Flint maize grain was processed through a hammer mill (ML 100-A, Lucato, Indústria e Comercial Lucato, Limeira, SP, Brazil). Geometric mean diameter (mm) was calculated using the equations of Baker and Herrman (Reference Baker and Herrman2002).
c Sodium monensin (Poulcox) was from Biovet Joint Stock Company, Sofia, Bulgaria. The blend of essential oils (CRINA Ruminants) and the exogenous enzyme [α-amylase (Ronozyme RumiStar)] were provided by DSM Nutritional Products, Basel, Switzerland.
d Observed NE was calculated using cattle performance data based on the equation proposed by Zinn and Shen (Reference Zinn and Shen1998).
e Expected values were calculated using the NASEM (2016) – empirical solution type – based on the TDN values (Weiss et al., Reference Weiss, Conrad and St-Pierre1992).
Experiment 2. Digestibility and ruminal fermentation
Effect of the maize particle size × feed additive interaction was not significant (P ⩾ 0.227; Table 7) for the intake of most nutrients, except for EE intake (P = 0.070; Table 8). Steers fed BEO + AM presented greater EE intake (P = 0.002) than steers fed MON in diets containing CG maize, but not MG maize (P = 0.130). Steers fed BEO + AM in diets containing CG particle size also had greater EE intake compared with bulls BEO + AM (P = 0.028) and MON (P < 0.001) in diets containing MG particle size.
Table 7. Nutrient intake and total apparent digestibility of Nellore feedlot steers provided, in a 2 × 2 factorial arrangement, diets containing flint maize ground to medium (1.66 mm; MG) or coarse (2.12 mm; CG) particle sizes, added with sodium monensin (26 mg/kg of DM; MON) or a blend of essential oils + exogenous α-amylase (90 and 560 mg commercial product/kg of DM respectively; BEO + AM) in Expt 2a

DM, dry matter; CP, crude protein; EE, ether extract; NDF, neutral detergent fibre corrected by ash; NFC, non-fibrous carbohydrate; TDN, total digestible nutrients.
a 4 × 4 Latin Square design [four steers (BW = 594 ± 22 kg); four periods (15 days for adaptation to diets and 5 days for sample collection); four treatments].
b Flint maize grain was processed through a hammer mill (ML 100-A, Lucato, Indústria e Comercial Lucato, Limeira, SP, Brazil). Geometric mean diameter (mm) was calculated using the equations of Baker and Herrman (Reference Baker and Herrman2002).
c Sodium monensin (Poulcox) was from Biovet Joint Stock Company, Sofia, Bulgaria. The blend of essential oils (CRINA Ruminants) and the exogenous enzyme [α-amylase (Ronozyme RumiStar)] were provided by DSM Nutritional Products, Basel, Switzerland.
Table 8. Intake of EE and total apparent digestibility of Nellore feedlot steers provided, in a 2 × 2 factorial arrangement, diets containing flint maize ground to medium (1.66 mm; MG) or coarse (2.12 mm; CG) particle sizes, added with sodium monensin (26 mg/kg of DM; MON) or a blend of essential oils + exogenous α-amylase (90 and 560 mg commercial product/kg of DM respectively; BEO + AM) in Expt 2a

EE, ether extract; NFC, non-fibrous carbohydrate; TDN, total digestible nutrients.
a 4 × 4 Latin Square design [four steers (BW = 594 ± 22 kg); four periods (15 days for adaptation to diets and 5 days for sample collection); four treatments].
b Flint maize grain was processed through a hammer mill (ML 100-A, Lucato, Indústria e Comercial Lucato, Limeira, SP, Brazil). Geometric mean diameter (mm) was calculated using the equations of Baker and Herrman (Reference Baker and Herrman2002).
c Sodium monensin (Poulcox) was from Biovet Joint Stock Company, Sofia, Bulgaria. The blend of essential oils (CRINA Ruminants) and the exogenous enzyme [α-amylase (Ronozyme RumiStar)] were provided by DSM Nutritional Products, Basel, Switzerland.
Effect of maize particle size was detected for intake of DM, CP, NDF, NFC, starch and TDN (P ⩽ 0.009), which were greater for steers fed CG than steers fed MG maize (Table 7). Feed additive affected intake of CP (P = 0.036) and tended to affect intakes of DM, starch and TDN (0.053 ⩽ P ⩽ 0.098), and in each case steers fed BEO + AM had greater intakes than MON.
Maize particle size × feed additive interaction was not significant (P ⩾ 0.174; Table 7) for the total apparent digestibility of most nutrients, except for total apparent digestibility of EE (P = 0.002), TDN (P = 0.049) and for a tendency for NFC apparent digestibility (P = 0.062; Table 8). Apparent total digestibility of EE was less for steers fed BEO + AM v. MON in diets containing MG maize (P = 0.010) but was greater than MON in diets containing CG maize (P = 0.020). Apparent total digestibility of EE was less for steers fed MON in diets containing CG v. MG maize (P = 0.001). Total apparent digestibility of NFC (P = 0.101) and the TDN (P = 0.060) tended to be greater for MON v. BEO + AM in diets containing MG maize. Total apparent digestibility of NFC tended to be greater for steers fed BEO + AM in diets containing CG maize compared with steers fed BEO + AM in diets containing MG maize (P = 0.041). No other differences between treatments were observed (P ⩾ 0.200) for total apparent digestibility of NFC and TDN.
Effect of maize particle size was not detected for total apparent digestibility of all nutrients (P ⩾ 0.327; Tables 7 and 8). Effect of feed additive was detected only for total apparent digestibility of NDF (P = 0.015), which was greater for steers fed MON than steers fed BEO + AM (Table 7).
Effects of maize particle size × feed additive (P ⩾ 0.110) and maize particle size × feed additive × time interactions (P ⩾ 0.126; data no shown) were not detected for any ruminal fermentation characteristics evaluated (Table 9). A tendency (P = 0.082) for maize particle size effect was detected for total VFA concentration in the rumen, which was greater for steers fed CG v. MG maize. Feed additive affected (P = 0.011) ruminal NH3-N concentration, which was less for steers fed BEO + AM compared with MON (Table 9).
Table 9. Ruminal fermentation characteristics of Nellore feedlot steers provided, in a 2 × 2 factorial arrangement, diets containing flint maize ground to medium (1.66 mm; MG) or coarse (2.12 mm; CG) particle sizes, added with sodium monensin (26 mg/kg of DM; MON) or a blend of essential oils + exogenous α-amylase (90 and 560 mg commercial product/kg of DM respectively; BEO + AM) in Expt 2a

VFA, volatile fatty acids; NH3-N, ammonia nitrogen.
a 4 × 4 Latin Square design [four steers (BW = 594 ± 22 kg); four periods (15 days for adaptation to diets and 5 days for sample collection); four treatments].
b Flint maize grain was processed through a hammer mill (ML 100-A, Lucato, Indústria e Comercial Lucato, Limeira, SP, Brazil). Geometric mean diameter (mm) was calculated using the equations of Baker and Herrman (Reference Baker and Herrman2002).
c Sodium monensin (Poulcox) was from Biovet Joint Stock Company, Sofia, Bulgaria. The blend of essential oils (CRINA Ruminants) and the exogenous enzyme [α-amylase (Ronozyme RumiStar)] were provided by DSM Nutritional Products, Basel, Switzerland.
Effect (P = 0.006) or a tendency (P = 0.071) for maize particle size effect were detected for nitrogen intake and faecal nitrogen excretion (Table 10), which were greater for steers fed CG than MG maize (28 and 25% respectively). Also, feeding BEO + AM increased nitrogen intake (P = 0.036) and tended to increase faecal nitrogen excretion (P = 0.089) by 17 and 22%, respectively, compared with MON (Table 10).
Table 10. Nitrogen metabolism and microbial protein synthesis of Nellore feedlot steers provided, in a 2 × 2 factorial arrangement, diets containing flint maize ground to medium (1.66 mm; MG) or coarse (2.12 mm; CG) particle sizes, added with sodium monensin (26 mg/kg of DM; MON) or a blend of essential oils + exogenous α-amylase (90 and 560 mg commercial product/kg of DM respectively; BEO + AM) in Expt 2a

Emic, microbial nitrogen efficiency; TDN, total digestible nutrients.
a 4 × 4 Latin Square design [four steers (BW = 594 ± 22 kg); four periods (15 days for adaptation to diets and 5 days for sample collection); four treatments].
b Flint maize grain was processed through a hammer mill (ML 100-A, Lucato, Indústria e Comercial Lucato, Limeira, SP, Brazil). Geometric mean diameter (mm) was calculated using the equations of Baker and Herrman (Reference Baker and Herrman2002).
c Sodium monensin (Poulcox) was from Biovet Joint Stock Company, Sofia, Bulgaria. The blend of essential oils (CRINA Ruminants) and the exogenous enzyme [α-amylase (Ronozyme RumiStar)] were provided by DSM Nutritional Products, Basel, Switzerland.
A tendency for a maize particle size × feed additive interaction effect (P ⩾ 0.081) was detected for nitrogen absorbed (Table 11). In diets containing CG maize, steers fed BEO + AM absorbed more nitrogen than steers fed MON (P = 0.010), but not in diets containing MG maize (P = 0.640). Steers fed BEO + AM in diets containing CG maize had greater absorbed nitrogen than steers fed BEO + AM in diets containing MG maize (P = 0.002) and also MON in diets containing MG maize (P = 0.002).
Table 11. Nitrogen absorbed (g/steer/day) of Nellore feedlot steers provided, in a 2 × 2 factorial arrangement, diets containing flint maize ground to medium (1.66 mm; MG) or coarse (2.12 mm; CG) particle sizes, added with sodium monensin (26 mg/kg of DM; MON) or a blend of essential oils + exogenous α-amylase (90 and 560 mg commercial product/kg of DM respectively; BEO + AM) in Expt 2a

a 4 × 4 Latin Square design [four steers (BW = 594 ± 22 kg); four periods (15 days for adaptation to diets and 5 days for sample collection); four treatments].
b Flint maize grain was processed through a hammer mill (ML 100-A, Lucato, Indústria e Comercial Lucato, Limeira, SP, Brazil). Geometric mean diameter (mm) was calculated using the equations of Baker and Herrman (Reference Baker and Herrman2002).
c Sodium monensin (Poulcox) was from Biovet Joint Stock Company, Sofia, Bulgaria. The blend of essential oils (CRINA Ruminants) and the exogenous enzyme [α-amylase (Ronozyme RumiStar)] were provided by DSM Nutritional Products, Basel, Switzerland.
Discussion
Effects of dent maize particle size on growth performance and nutrient digestibility have been investigated in previous trials (Galyean et al., Reference Galyean, Wagner and Owens1979; Turgeon et al., Reference Turgeon, Brink and Britton1983; Secrist et al., Reference Secrist, Hill, Owens and Welty1995, Reference Secrist, Hill, Owens and Welty1995; Swanson et al., Reference Swanson, Islas, Carlson, Goulart, Gilbery and Bauer2014; Lundy et al., Reference Lundy, Doran, Vermerr, Loy and Hansen2015). In summary, small or no differences in growth performance of finishing cattle were observed among maize particle sizes (n = 6 studies; comparisons = 14; average particle size = 2.52 mm; maximum particle size = 7.94 mm; minimum particle size = 0.5 mm; particle size s.e.m. = 0.52 mm), despite total TSD often being greater in more finely ground dent maize. One possible reason for the lack of response may be because decreasing particle size in dent maize increases the rate of fermentation in the rumen, which may increase the incidence of subacute acidosis (Owens et al., Reference Owens, Secrist, Hill and Gill1998). On the other hand, intestinal starch digestion also may compensate for less extensive ruminal starch digestion when large particle sizes were fed (Owens et al., Reference Owens, Zinn and Kim1986; Huntington et al., Reference Huntington, Harmon and Richards2006), whereas energy availability from starch digested in the small intestine (estimated at 97%) is greater than energy availability from starch fermented in the rumen (estimated at 80%) due to reduced energy loss as heat and methane (Huntington et al., Reference Huntington, Harmon and Richards2006). Hence, even if total TSD is greater for small particles, differences in site of digestion may counterbalance this effect so that no detrimental effects on growth performance are observed with more coarsely ground dent maize grain.
Flint maize is more vitreous than dent maize. The starch from maize grain that is more vitreous is less rapidly digested in the rumen based on in-situ measurements, and less digested in the total tract (Philippeau and Michalet-Doreau, Reference Philippeau and Michalet-Doreau1998). However, compared with ground dent grain, ground flint grain yields larger particles, and large particles are less rapidly and extensively digested than small particles, so particle size differences may partially explain the in-situ differences. Indeed, Ramos et al. (Reference Ramos, Champion, Poncet, Mizubuti and Nozière2009) illustrated that fine grinding can fully eliminate differences in in-situ starch degradation associated with vitreousness. The current authors hypothesized that grinding flint grain to a smaller particle size would increase nutrient digestion and consequently animal growth performance because finer grinding would increase the surface area for microbial attack (Richards and Hicks Reference Richards and Hicks2007). Reducing particle size from dry rolling (3.02 mm) to fine grinding (1.10 mm) flint maize increased feed efficiency by 12% and NEg by 14.4% in finishing cattle fed diets containing 120 or 200 g/kg of sugarcane bagasse as forage source (Carareto et al., Reference Carareto, Santos, Mourao, Pedroso, Sitta, Soares, Paula, Marques and Soares2011). Increases in the observed NEg value of flint maize have also been reported for more extensive maize processing methods such as steam flaking (Gouvêa et al., Reference Gouvêa, Batistel, Souza, Chagas, Sitta, Campanili, Galvani, Pires, Owens and Santos2016; Marques et al., Reference Marques, Chagas, Owens and Santos2016). However, no advantages on growth performance were observed in the current study where maize particle size was reduced from 2.12 to 1.66 mm and the surface area increased from 344 to 414 particles/g. On the contrary, higher particle sizes resulted in greater intake and growth performance in the current study.
McAllister et al. (Reference McAllister, Phillippe, Rode and Cheng1993) reported that although grinding dry maize ruptured the endosperm cells, starch granules remained embedded within a protein matrix. This could at least partially explain the lack of effect associated with maize particle size over the growth performance observed in the current trial. On the other hand, McAllister et al. (Reference McAllister, Phillippe, Rode and Cheng1993) observed that in-vitro starch digestion was greater for smaller particles (0.25–0.89 mm) than larger particles (2.00–3.00 mm), suggesting that at a similar ruminal residence time, an increase in surface area should increase the extent of starch degradation. Most of the difference in particle size for MG and CG maize used in the current trial came from changing maize distribution between the sieve of 1.25 and 2.00 mm in MG maize to particles between 3.5 and 6.0 mm in the CG maize. Grain particle distribution between 2.0 and 3.5 mm was very similar in both MG and CG and averaged 325 g/kg. Also, particle sizes lower than 1.25 mm averaged 220 g/kg between the two particle sizes evaluated. Therefore, in addition to the small difference between the two particle sizes evaluated in the current trial (1.66 v. 2.12 mm), the range in particle size distribution and surface area (30.4 v. 25.3 cm2/g) may have been too narrow to enhance microbial attachment and colonization in such a way as to affect growth performance. On the other hand, the narrow differences in maize particle size and its distribution in the sieves herein evaluated resulted in greater intake and growth performance when bulls were fed CG maize, suggesting positives outcomes from larger particle sizes.
An interaction between flint maize grain processing and the amount of dietary NDF from sugarcane silage was reported by Caetano et al. (Reference Caetano, Goulart, Silva, Drouillard, Leme and Lanna2015). The NDF level from sugarcane silage for maximum DMI was 137 g/kg of DM with high moisture flint maize (5.84 mm particle size) but 113 g/kg of DM for ground flint maize (1.30 mm particle size), presumably due to the greater availability of starch and ME of diets (12.4 and 11.3 MJ/kg for high moisture and ground maize respectively). Carareto et al. (Reference Carareto, Santos, Mourao, Pedroso, Sitta, Soares, Paula, Marques and Soares2011) showed that observed NEm and NEg for bulls fed flint ground maize (1.10 m particle size) was greater than bulls fed dry rolled flint maize (3.10 mm particle size) but no interaction between the forage level (120 v. 200 g/kg of sugarcane bagasse) and grain particle size was observed. In the current study, sugarcane bagasse was included at 85 g/kg (DM basis) corresponding to 69 g/kg of NDF from sugarcane bagasse. Mean ruminal pH of 5.66 and 5.58 for MG and CG maize diets, respectively, are indications of sub-acute ruminal acidosis (NASEM, 2016). Relative to the Carareto et al. (Reference Carareto, Santos, Mourao, Pedroso, Sitta, Soares, Paula, Marques and Soares2011) and Caetano et al. (Reference Caetano, Goulart, Silva, Drouillard, Leme and Lanna2015) studies, the amount of sugarcane bagasse included in the current trial was not enough to maximize DMI and growth performance in MG maize diets and contributed to the failure to detect a benefit from the finer particle size. One may speculate that cattle down-regulated DMI when MG maize was fed, in an attempt to avoid a drop in pH to 5.2 or 5.0 and the occurrence of acute acidosis (NASEM, 2016). Therefore, the benefits of CG maize over MG maize in the current study can be related to the greater DMI and greater concentration of VFA in the rumen. Overall, CG maize did not change the efficiency of energy use even though it increased nutrient intake compared with MG maize. This conflicts with the concept that decreasing maize particle size should increase starch digestibility and thereby increase energy density of the diet (Owens et al., Reference Owens, Secrist, Hill and Gill1997), resulting in DMI reduction (Krehbiel et al., Reference Krehbiel, Cranston and McCurdy2006). In fact, reducing maize particle size reduced DMI in the current study, however no advantages over maize digestibility and diet net energy were observed. The reasons for the lack of response in animal growth performance are not clear.
The failure of MG to increase total tract starch digestibility and increase NE value of these maize-rich diets conflict with the generally accepted dogma concerning starch digestion and utilization. In-vitro and in-situ measurements consistently indicate that rate and extent of ruminal digestion of starch is greater for smaller than larger maize grain particles. Increased rate of ruminal starch digestion should translate to increased extent of ruminal starch digestion if ruminal retention time remains unchanged. If ruminal starch digestion is increased, one might expect total tract starch digestibility to increase unless starch digestion in the large intestine is sufficiently high. According to Harmon et al. (Reference Harmon, Yamka and Elam2004) the amount of starch digested in the rumen is a linear function of intake, but at high levels of DMI, factors other than intake can affect starch digestion, such as ruminal kinetics variables, chemical properties (pH, osmolarity), rates and extents of particle passage (Huntington et al., Reference Huntington, Harmon and Richards2006).
According to Schwandt et al. (Reference Schwandt, Thomson, Bartie and Reinhard2015), different definitions of ‘fine’, ‘medium’ and ‘coarse’ ground maize is a likely source of variation of conflicting results. Furthermore, more important than the average particle size is the particle distribution across sieves. Future studies should evaluate the effects of maize particle distribution, within the same average particle size, on starch digestibility and growth performance.
Relative to feeding CG with MON, feeding CG with BEO + AM enhanced HCW despite having no effect on nutrient digestibility or on observed NE concentrations. The observed differences in intake and digestibility of EE and nitrogen absorbed must be involved in the observed performance. Since microbial yield was not affected by treatments, it could be speculated that lower rumen N-NH3 was caused by reduction of deamination, contributing to greater N supply and absorption in the small intestine of cattle fed BEO + AM compared with MON in CG maize diets. Similarly, Meschiatti et al. (Reference Meschiatti, Gouvêa, Pellarin, Batalha, Biehl, Acedo, Dórea, Tamassia, Owens and Santos2019) also observed 12 kg greater HCW for cattle fed the combination of BEO + AM compared with cattle fed MON in diets containing flint maize with a 2.04 mm particle size and also observed that MON decreased DMI compared with BEO + AM, despite no differences in feed efficiency between these two feed additives.
Yang and Russell (Reference Yang and Russell1993) reported that when monensin is fed, acetate typically decreases or does not change, but propionate concentration increases; this lowers the acetate : propionate ratio. In the current study, neither total nor proportions of individual VFA concentrations were significantly different between cattle given MON or BEO + AM, despite greater DMI of cattle fed the BEO + AM combination. It is uncertain whether the reduced DMI with the MON diets reflects a satiety signal resulting from propionate based on its hepatic effects, as proposed by Allen et al. (Reference Allen, Bradford and Oba2009), or some other factors. Meyer et al. (Reference Meyer, Erickson, Klopfenstein, Greenquist, Luebbe, Williams and Engstrom2009), using the same commercially available BEO as was used in the current trial, reported that total VFA concentration were greater compared with monensin even though no differences were detected in the molar proportion of propionate agreeing with findings of the current study. Reduced overall DMI and DMI fluctuation is usually observed in finishing steers fed diets containing sodium monensin, which is associated with greater growth performance (Stock et al., Reference Stock, Ludert, Stroup, Larson, Parrott and Britton1995; Pereira et al., Reference Pereira, Cruz, Arrigoni, Rigueiro, Silva, Carrara, Santos, Cursino and Millen2016). According to Duffield et al. (Reference Duffield, Merrill and Bagg2012), in high-energy finishing diets the increased propionate stimulated by monensin might have more effect on reducing DMI. However, compared with the combination of BEO + AM, the decreased DMI when bulls were fed MON did not result in greater feed efficiency. Therefore, the greater DMI observed in bulls fed BEO + AM was followed by a greater ADG, despite no statistical differences, leading to the same feed efficiency between the two feed additives evaluated. Similarly to Meschiatti et al. (Reference Meschiatti, Gouvêa, Pellarin, Batalha, Biehl, Acedo, Dórea, Tamassia, Owens and Santos2019), through the higher DMI and minimal differences over nutrient digestibility resulting in similar ruminal fermentation patterns, animals fed CG associated with BEO + AM sustained greater carcass weight compared with animals fed MON.
According to Callison et al. (Reference Callison, Firkins, Eastridge and Hull2001), ammonia nitrogen concentration in the rumen represents a net balance between ammonia production (degraded dietary protein), ammonia uptake by microbes (that varies with carbohydrate availability), ammonia absorption (which varies with ruminal pH) as well as recycling of urea to the rumen (via saliva and through the ruminal wall). In the current study, because rumen degradable protein was similar between diets and pH was not affected by treatment, recycling should not have been altered. Thereafter, despite the greater intake of DM, starch and TDN of cattle fed BEO + AM compared with cattle fed MON, microbial yield was not increased. Therefore, it is speculated that the combination of BEO + AM was effective at decreasing deamination of amino-acids and consequently decreasing rumen N-NH3. Consequently, benefits from the combination of BEO + AM and CG maize may be related to greater DMI and greater amount of nitrogen absorbed compared with monensin. For example, Andreazzi et al. (Reference Andreazzi, Pereira, Reis, Pereira, Morais Júnior, Acedo, Hermes and Cortinhas2018) proposed that for lactating cows, the increased ruminal starch degradation induced by feeding exogenous α-amylase was reflected not by increased total tract starch digestibility, but instead by increased milk yield and reduced DMI, improving feed efficiency in dairy cows. Also, according to Andreazzi et al. (Reference Andreazzi, Pereira, Reis, Pereira, Morais Júnior, Acedo, Hermes and Cortinhas2018), the post-ruminal digestion of starch escaping ruminal digestion compensated the total starch digestion. Others have suggested that increased starch digestion associated with amylase supplementation instead increases total tract digestibility of NDF because less starch reaches the large intestine to decrease the pH and NDF fermentation at that site (Klingerman et al., Reference Klingerman, Hu, McDonell, DerBedrosian and Kung2009; DiLorenzo et al., Reference DiLorenzo, Smith, Quinn, May, Ponce, Steinberg, Engstrom and Galyean2011). Further information about the site and extent of starch and NDF digestion is needed to fully understand the reasons why the combination of BEO + AM increased growth performance and carcass weight in diets contacting CG maize.
In summary, diets containing 85 g/kg of sugarcane bagasse, grinding flint maize to 2.12 mm particle size increases growth performance and carcass characteristics compared with 1.66 mm, especially due the increase of DMI. The combination of a blend of essential oils with exogenous α-amylase result in the heavier carcass weights compared with monensin supplementation when included in diets containing coarse ground maize because of greater intake of energy and greater absorption of N, with no negative effect on feed efficiency.
Financial support
This study was supported by DSM Nutritional Products (DSM, São Paulo, Brazil). The scholarship of the second author was supported by Coordenação de Aperfeiçoamento de Pessoal de Nível Superior (CAPES, Brazil) – Finance Code 001.
Conflict of interest
None.
Ethical standards
Animals were cared for according to the guidelines recommended by the Animal Care and Use Committee of the ‘Luiz de Queiroz’ College of Agriculture (ESALQ), University of São Paulo (USP) – protocol # 2015-29.