Introduction
Milk fat lipolysis consists of enzymatic hydrolysis of the triglycerides of fat globules, which leads to an increase in the concentration of free fatty acids (FFAs) and, consequently, of the acidity of the fat. In raw milk, lipolysis is mainly caused by the action of lipoprotein lipase (LPL), a natural milk enzyme synthesized in secretory epithelial cells (Chen et al., Reference Chen, Daniel and Coolbear2003), although it may be partly haematic in origin (Chilliard et al., Reference Chilliard, Ferlay, Rouel and Lamberet2003). Other enzymes with significant lipolytic activity are lipases of microbial origin, mainly from psychotropic germs (Ouattara et al., Reference Ouattara, Jeon, Hart-Thakur and Schmidt2004), and somatic cell lipases (Gargouri et al., Reference Gargouri, Hamed and ElFeki2008).
Different physiological, genetic and nutritional factors have been identified that may influence the LPL activity in raw milk (Deeth, Reference Deeth2006; Chilliard et al., Reference Chilliard, Toral, Shingfield, Rouel, Leroux and Bernard2014). The good correlation between LPL activity and lipolysis could be due to a higher degree of association of LPL with the fat phase in goat's milk (Chilliard et al., Reference Chilliard, Selselet-Attou, Bas and Morand-Fehr1984), thus increasing the enzyme–substrate interactions, contrary to what occurs in cow's milk (Chilliard et al., Reference Chilliard, Ferlay, Rouel and Lamberet2003). On the other hand, it has also been shown that the factors that impair fat globule membrane integrity, such as excessive shaking or abrupt changes in storage temperature, can increase the lipolysis level in milk by exposing the triglycerides to lipase action (Meffe, Reference Meffe1994; Chen et al., Reference Chen, Daniel and Coolbear2003).
It should be noted that the hydrolytic release of FFA from triglycerides can have negative consequences for the dairy industry. First, it may affect the technological properties of milk, causing fat loss and delays in the growth of starter cultures used in the production of fermented products such as cheese or yoghurt (IDF 1991; Collomb and Spahni, Reference Collomb and Spahni1995). It may also give rise to the appearance of off-flavours, described as rancid, butyric, astringent or even bitter, in milk and its by-products (Le Mens et al., Reference Le Mens, Heuchel, Jubert, Bodin, Sauvageot and Humbert1997; Deeth Reference Deeth2006). For this reason, FFA concentration is often used as an indicator of the organoleptic quality of milk, which is occasionally included in inter-professional regulations for payment by quality (Pirisi et al., Reference Pirisi, Lauret and Dubeuf2007; Skeie Reference Skeie2014). Additionally, another possible negative effect of lipolysis is that it could affect the analytical results of milk composition obtained with infrared equipment (IDF 2000), as the release of FFA from triglycerides due to lipase action changes the readings (absorbances) from the equipment in certain wavelengths that affect the determination of fat (fat wavelength A, 5.7 µm) and protein (protein wavelength: 6.5 µm). Similarly, Robertson et al. (Reference Robertson, Dixon, Nowers and Brink1981) stated that in cow's milk an increase in FFA of 1 meq/l resulted in analyses with infrared equipment showing a decrease in fat (−0.033%) and an increase in crude protein content (+0.019%). These analytical changes are also reflected in the IDF standard for cow's milk analysis with mid-infrared based equipment (IDF 2000).
Although lipolysis progresses during milk storage, most of it occurs in the first 24 h of refrigeration (Wiking et al., Reference Wiking, Björk and Nielsen2003; Ouattara et al., Reference Ouattara, Jeon, Hart-Thakur and Schmidt2004), before it reaches the dairy industry. Therefore, the mechanical stress that affects milk in the milking systems becomes a crucial aspect to preserve milk supply and quality.
Several studies in cattle have demonstrated the relationship between certain milking conditions and the increase in lipolysis in milk (Pillay et al., Reference Pillay, Myhr, Gray and Biggs1980; Escobar and Bradley, Reference Escobar and Bradley1990; Abeni et al., Reference Abeni, Degano, Calza, Giangiacomo and Pirlo2005). One of these factors is milking with a milk line located above the animals’ standing level, i.e. in mid-line (ML) or high-line (HL), with elevations lower or higher than 1.25 m, respectively (ISO, 2007a). In this type of setup, the milk is mixed with air so that it rises through the long milk tube, forming bubbles and, therefore, being submitted to more turbulent agitation. In cattle, it has been shown that HL milking, in comparison with low-line milking (LL), increases milk lipolysis (Gudding and Lorentzen, Reference Gudding and Lorentzen1982; Mikulová, Reference Mikulová2011); the higher the milkline height or the air intake caused by the milking cluster, the greater the increase (Judge et al., Reference Judge, Fleming, O'Shea and Raftery1977; Meffe, Reference Meffe1994; Rasmussen et al., Reference Rasmussen, Wiking, Bjerring and Larsen2006). However, information about this is scarce in small ruminants, even though the use of ML milking has become increasingly popular in recent years. This is because, with an equal number of milking stalls, the installation of an ML usually cuts initial investment by around 25–35% (Díaz et al., Reference Díaz, Peris, Rodríguez, Molina and Fernández2004) compared with LL. To the best of our knowledge, there are only two studies: one comparing ML v. LL in sheep (Díaz et al., Reference Díaz, Peris, Rodríguez, Molina and Fernández2004) and another comparing HL v. LL in goats (Morand-Fehr et al., Reference Morand-Fehr, Selselet Attou, Bas and Chilliard1983), neither of which found statistically significant differences in FFA concentrations in milk. Further studies along these lines would be warranted to determine whether the use of ML or HL milking can negatively affect the quality of goat's milk; a crucial aspect when considering that goat's milk is mainly used to manufacture cheese, whose sensory characteristics might be affected by an increase in the FFA concentration as a result of excessive lipolysis in the milk. In this sense, Morgan et al. (Reference Morgan, Bodin and Gaborit2001) noted a high risk of obtaining lactic coagulation cheeses with unacceptable sensory characteristics when the FFA concentration is ⩾1 g oleic acid/100 g milkfat (3.5 meq/100 g milkfat) in goat's milk. However, there is no information on the effect of lipolysis on the sensory quality of cheeses made by enzymatic coagulation, a processing technique widely used in traditional goat cheese-making of Mediterranean countries. There are no studies evaluating the effect of lipolysis in goat's milk on the results of analyses performed with infrared equipment by milk quality laboratories. To this end, the aim of the current study was to assess the influence of ML milking system on lipolysis and components of goat's milk that are determined routinely with infrared equipment, taking milk from LL milking as a reference. The effect of ML milking on the sensory features of enzymatic coagulation goat cheeses was also evaluated.
Material and methods
Experimental procedure
To meet the aforementioned objectives, two experiments were carried out: the first at the dairy goat experimental farm of the Universitat Politècnica de València (UPV, Valencia, Spain) and the second on commercial dairy goat farms, whose bulk milk was routinely analysed at the Interprofessional Dairy Laboratory of the Valencian Community Region (LILCOVAL, Valencia, Spain).
First experiment
This experiment was carried out in triplicate on the Universitat Politècnica de València (UPV) experimental farm. Each replicate experiment was designed as follows: 62 Murciano-Granadina breed goats, halfway through the lactation period (4 ± 1.0 month of lactation), were used. The animals were divided into two groups of 28 goats each, according to production level and lactation number, with each group randomly assigned to ML or LL milking for an initial 4-day period. Then, the treatments (ML and LL) were switched between the two groups for a second experimental 4-day period.
The goats were milked once on a daily basis (08:30 h) following a routine which included machine stripping, manual teat-cup removal and iodine post-dipping solution.
The milking parlour (2 × 12) had two milking pipelines installed with six clusters in ML and 12 clusters in LL. The ML milkline, dead in type, had an internal diameter of 52 mm and length of 520 cm and was located at 112 cm above the goat standing level. The LL milkline, looped type, was 52 mm in diameter, 1500 cm in length and located at 40 cm below the standing level.
The Almatic™ cluster G50 from DeLaval (Tumba, Sweden) was used in the current study. However, in the case of ML milking, a claw from DeLaval cluster SG-TF80 (claw volume 100 ml) was incorporated.
A different nominal vacuum was set (40 kPa in ML and 37 kPa in LL) so that the average teat-end vacuum, in the absence of milk flow during milking, was similar in both types of milking systems. The effective reserve (ML: 750 litres/min; LL: 950 litres/min) complied with international recommendations (higher than 512 and 804 litres/min in ML and LL, respectively; extra air for automatic teat-cup valves: 32 litres/min; ISO, 2007b). The pulsation rate (90 cycles/min) and ratio (60:40) were the same in ML and LL. The air intakes in the milking cluster (7.5 litres/min) were produced in the inlets at the base of the teat-cup liners.
The milk from the two groups of animals was stored separately in tanks that were empty at the start of each experimental period and which accumulated the milk obtained during each 4-day set. Bulk milk samples (250 ml) were taken on a daily basis from each batch of animals immediately after milking (0 h) and 24 h later, just before starting the next milking, to determine the following variables: FFA content, main milk components (fat, protein, lactose and dry matter), pH, freezing point, somatic cell count (SCC) and total bacterial count (TBC). For the FFA analysis, a 30 ml aliquot was separated after sampling, adding hydrogen peroxide (0.02%) following IDF recommendations (1991). In addition, the bulk milk accumulated during each 4-day period was used to prepare enzymatic coagulation cheeses, whose sensory characteristics were assessed by a test panel after 50 and 100 days’ maturation. The cheese-making could only be performed in the first experimental replicate.
Second experiment
Of the 200 commercial dairy goat farms whose milk is usually analysed by the Interprofessional Dairy Laboratory of the Valencian Community Region (LILCOVAL, Valencia, Spain), 55 were chosen at random, 25 with ML milking and 30 with LL. All these commercial exploitations produce milk from Murciano-Granadina goats and carry out a daily milking routine including machine stripping, manual teat-cup removal and some of them use an iodine post-dipping solution.
Milking parlours had one or two milking platforms (0.28 and 0.62, respectively), most of them having between 12 and 24 stalls per platform. In ML, there was usually a milking cluster for every 2–4 stalls, whereas the most frequent setup in LL was one milking cluster for every 1–2 stalls.
Bulk milk samples from commercial farms were taken in 50 ml flasks containing azidiol as a preservative (133 µl/40 ml milk), as stipulated by the Spanish legislation (Ministerio de la Presidencia, 2011). Milk sampling was performed weekly during three consecutive weeks, between April and May (one sample/week and farm) to determine the same variables as in the first experiment.
Cheese procedure
Four batches of cheese were prepared in a commercial artisan cheese factory, with milk collected by LL and ML milking from each of the two periods considered in the first replicate of the experiment.
Pasteurized goat's milk (74 °C, 15 s) inoculated with starter cultures and spiked with calcium chloride was coagulated by rennet at 32 ± 1.0 °C. After coagulation (40 min), the curd was cut and gently shaken for 20–30 min while the temperature was steadily increased until reaching a maximum of 38 °C. After moulding, the cheeses (900–1000 g) were pressed for 2 h under increasing pressure until a pH value of 5.3–5.4 was reached. Next, cheeses were salted immersed in brine (22 Bè) for 4 h and then placed in an airing chamber (4 °C, 75% RH) for 48 h. Finally, the cheeses were kept in a ripening chamber (10–12 °C, 80–85% RH) for a 100-day period.
Analytical procedure
FFA quantification was performed, in duplicate, at the Interprofessional Dairy Laboratory of Cantabria Region (LILC, Santander, Spain) using the copper soap method (IDF, 1991). The SCC was analysed with Fossomatic 5000 equipment (Foss, Hillerød, Denmark) and the chemical composition of the milk (fat, protein, lactose and dry matter) was analysed using Milko Scan FT 6000 infrared equipment (Foss). The freezing point and the pH of goat's milk were determined by reference methods using a thermistor cryoscope (Cryostar, Funger-Gerber, Germany) and a conventional pH meter (Crison Instruments, Barcelona, Spain), respectively. The TBC of the milk samples was determined from the standard plate count at 30 °C (ISO, 2013).
Sensory analysis of cheeses made with milk from ML and LL milking was performed by a panel of 62 tasters (balanced 0.50 by gender and aged from 20 to 55 years) through a triangular test (ISO, 2004). At each tasting session, the judges analysed two successive triads with cheeses from both experimental periods. These tests were performed repeatedly at 50 and 100 days of maturation, obtaining a total of 248 evaluations.
Statistical analysis
In the first experiment, the milk quality variables (FFA content, main milk components (fat, protein, lactose and dry matter), pH, freezing point, SCC and TBC) were analysed using PROC GLM procedures in SAS 9.2, with a model that included the following fixed effects: milkline (ML and LL), post-milking time (0 and 24 h), replication of the trial (1–3), day of the period (1–4), their respective interactions and the effect of the group of animals within each replicate.
The variables of the second experiment were analysed using PROC MIXED procedures in SAS 9.2, as per Littell et al. (Reference Littell, Henry and Ammerman1998), using a model that considered the fixed effects of the milkline (ML and LL), the week of sampling (1–3) and their interaction and the random effect of the farm (1–55). In both analyses, when an interaction was non-significant, the corresponding interaction term was pooled with the error.
The data obtained from the sensorial analysis of the cheeses (frequency of hits in the triangular test) were analysed statistically based on the binomial distribution of the parameter P = 1/3 with n responses (ISO, 2004).
Results
Goat's milk quality parameters
In the first experiment it was observed that ML milking caused a significant increase (P < 0.001) in the FFA concentration in goat's milk compared with LL milking (Table 1). This was the case in milk samples taken immediately after milking (ML: 0.64 ± 0.020 mmol/l; LL: 0.35 ± 0.020 mmol/l) as well as in those taken after 24 h of refrigerated storage (ML: 0.77 ± 0.020 mmol/l; LL: 0.45 ± 0.020 mmol/l). A higher level of lipolysis in ML than in LL was also found in each of the three experimental replicates, although in the last replicate a smaller difference was observed (milkline × replicate interaction significant, P < 0.05; Fig. 1).

Fig. 1. FFA concentrations (average value ± s.e.m., expressed as mmol/l) in the bulk milk of Murciano-Granadina breed goats according to the type of milking (ML: mid-line; LL: low-line) in each replicate of the study conducted under experimental conditions.
Table 1. Average values of parameters in bulk milk samples of Murciano-Granadina breed goats according to the type of milking used (ML: mid-line; LL: low-line) and refrigerated storage time (0 and 24 h post-milking) obtained under experimental farm conditions

FFA, free fatty acids (mmol/l); SCC log, somatic cell count (cell/ml) logarithm; TBC log, total bacterial count (cfu/ml) logarithm. s.e.m., standard error of the mean.
*P < 0.05 and ***P < 0.001. Degrees of freedom for milking type and refrigerated storage time are respectively, 1 and 1.
The time elapsed since milking also significantly affected the FFA concentration in goat's milk, in such a way that the milk samples taken after 24 h in refrigerated tank storage presented higher values (P < 0.001) than those taken immediately after milking (Table 1). Concerning the evolution of lipolysis with storage days, Fig. 2 shows how the release of FFA in bulk milk tended to increase during the 4-day study period, although the differences were only significant (P < 0.05) when the values of the first day were compared with those of the following days. All the interactions considered in the model (except the day × milkline interaction, described above) were non-significant.

Fig. 2. Evolution of FFA concentration (mmol/l) in bulk milk of Murciano-Granadina breed goats during a 4-day storage period (mean values of milk from ML and LL milking).
The rest of the milk variables analysed (gross composition, pH-value, freezing point, SCC and TBC) was unaffected by the milking pipeline height (Table 1). The time elapsed since milking only significantly affected (P < 0.05) the pH-value, which was higher (0.03) in milk samples taken immediately after milking than in those taken at 24 h post-milking. The storage day factor and all the interactions included in the statistical model did not significantly affect the aforementioned variables.
On the other hand, in the second experiment, carried out under commercial conditions, the level of lipolysis in the milk did not differ significantly between ML and LL milking (Table 2). Moreover, goat's milk from most commercial farms presented an FFA concentration between 0.2 and 0.8 mmol/l, regardless of the type of milking installation used (Fig. 3). Nor did the two groups of farms differ significantly in milk gross composition (fat, protein, lactose and total solids), pH-value, freezing point, SCC or TBC (Table 2). The milkline × week interaction was also non-significant in all cases.

Fig. 3. Frequency distribution of FFA concentration (mmol/l) in goat milk samples from commercial farms according to the type of milking used (ML: mid-line; LL: low-line).
Table 2. Quality parameters (average value ± s.e.m.) for goat's milk in commercial farm bulk samples according to the type of milking used (ML: mid-line; LL: low-line)
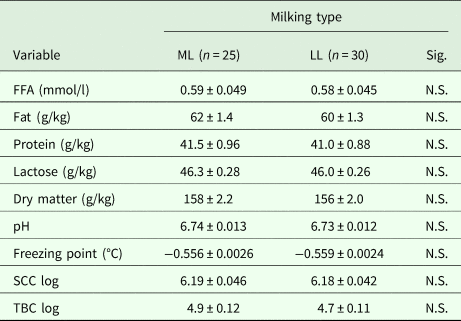
FFA, free fatty acid (mmol/l); SCC log, somatic cell count (cell/ml) logarithm; TBC log, total bacterial count (cfu/ml) logarithm; N.S., not significant.
Degrees of freedom for milking type are 53.
Sensory analysis of goat's milk cheeses
The characteristics of the goat's milk used in each of the cured cheese manufactures are presented in Table 3 and the results of sensory analysis of the cheese samples in Table 4.
Table 3. Quality parameters of goat's milk from ML and LL milking used for the production of cured cheese in each of the two 4-day periods of the first experiment

FFA, free fatty acids; SCC, somatic cell count; TBC, total bacterial count.
Table 4. Results of the sensorial analysis triangular test on cheeses made with milk obtained in the first experimental replicate from ML or LL milking, performed at 50 and 100 days of maturation

a Two triads per judge (one in the first experimental period, and another in the second); N.S., non-significant differences.
The outcomes show that the judges were not able to perceive significant differences between the two types of cheeses for either of the two maturing times considered (50 and 100 days). On the other hand, when tasters were successful in differentiating the two types of samples, 0.63 of the judgements considered that the cheeses from ML milking presented more intense flavour features (stronger, more acid or spicier) than the cheeses from LL milking, with the latter generally being the main reason for their choice.
Discussion
The average FFA concentration found in the current work for LL and ML milking in the first experiment (0.40 and 0.71 mmol/l, respectively; 0.88 and 1.5 meq/100 g milkfat), and in the second (0.58 and 0.59 mmol/l; 0.96 meq/100 g milkfat), falls within the range reported by other authors (Žan et al., Reference Žan, Stibilj and Rogelj2006; Strzalkowska et al., Reference Strzalkowska, Józwik, Bagnicka, Krzyżewski, Horbańczuk, Pyzel, Sloniewska and Horbańczuk2010; Chilliard et al., Reference Chilliard, Toral, Shingfield, Rouel, Leroux and Bernard2014) for goat's milk.
The three replicates performed under experimental conditions coincide in demonstrating that ML milking significantly increases the level of lipolysis in goat's raw milk, compared with LL milking, presenting an increase in the FFA concentration of between 62 and 92%. According to Meffe (Reference Meffe1994), the height of the milkline above the animals’ standing level can accentuate deterioration of the membrane of the fat globules through two mechanisms. The first is that the rise of the milk through the long milk tube, mixed with air and in a totally turbulent regime, causes an increase in the air–milk interface (the more the higher the air/milk ratio), giving rise to a greater deformation and risk of rupture of the membrane of the fat globules in the said interface. The second mechanism, less important than the previous one, derives from the friction of the milk against the walls of the pipes, subjecting the fat globule to shear forces that can break its membrane. Therefore, as in ML milking installations the length of the long milk tube is usually almost double that in LL (in the current work 215 and 100 cm, respectively), it can be assumed that the cited risk will increase. Moreover, it must be noted that in the three assays performed, an increase in the FFA concentration in the milk after 24 h in the refrigerated storage tank (between 10 and 42% depending on the type of line and assay) was observed, which agrees with the findings of other authors in cows’ milk (Wiking et al., Reference Wiking, Frøst, Larsen and Nielsen2002; Ouattara et al., Reference Ouattara, Jeon, Hart-Thakur and Schmidt2004).
However, the fact that commercial farms did not show significant differences in the FFA concentration in goat's milk depending on the type of milking installation (ML v. LL) suggests that the other factors able to influence lipolysis (physiological, genetic and other features of the milking machine, among others; Deeth Reference Deeth2006; Chilliard et al., Reference Chilliard, Toral, Shingfield, Rouel, Leroux and Bernard2014) are more important overall than the effect of the milk line height alone. In fact, some ML farms repeatedly had low FFA values (0.2–0.4 mmol/l), whereas other farms using LL always presented FFA concentrations higher than 0.8 mmol/l. Thus, it does not seem that the increase in lipolysis that can occur exclusively due to the effect of ML milking is a decisive argument to discourage this type of milking installation.
In any case, the FFA concentration in milk from most commercial farms considered in this study was lower than the threshold values applied in quality payment systems for goat's milk used in some regions of France (1.77 meq/100 g milkfat; Pirisi et al., Reference Pirisi, Lauret and Dubeuf2007), and Norway (1.33 meq/l; Skeie Reference Skeie2014), regardless of the type of milking system employed. Thus, 0.97 of goat's milk samples from farms using ML milking presented a FFA concentration lower than the French threshold, more restrictive, whereas for farms with LL milking, this proportion was 0.94.
On the other hand, ML milking had no relevant effect on the analytical results of the different milk components analysed by infrared equipment, in either experiment one or experiment two, as the differences found were quantitatively of low importance (⩽0.02%) and did not reach significance in any case. Similarly, in cows’ milk, Kaylegian et al. (Reference Kaylegian, Lynch, Fleming and Barbano2007) also found changes in fat and protein values no higher than 0.01% when increasing FFA up to 0.2 meq/kg milk.
Regarding cheeses, no information is currently available on the maximum thresholds of lipolysis (FFA) in the goat's milk of indigenous breeds to avoid the deterioration of the sensory characteristics of the flavour of the cheeses, in particular those made by enzymatic coagulation. The organoleptic analysis results suggest that FFA values in milk of 0.79 mmol/l compared with 0.45 mmol/l (1.73 meq/100 g milkfat v. 0.97 meq/100 g milkfat) do not alter significantly the sensory characteristics of the enzymatic coagulation cured cheeses. Nevertheless, these FFA levels are sufficient for some consumers to be able to detect some more intense flavour features (i.e. stronger, more acid or spicier) in the cheeses made with milk from ML milking. In any case, these values are far from those reported by other authors as causing off-flavours in goat's cheeses (3.5 meq/100 g milkfat, Morgan et al., Reference Morgan, Bodin and Gaborit2001). However, it seems prudent not to directly extrapolate these results to the environment of the current work, given the differences in milk composition and the cheese manufacturing process. This topic, therefore, remains open for future studies.
Conclusion
In experimental farm conditions, it was found that ML milking significantly increased the FFA concentration in raw goat's milk compared with LL milking system. However, the results obtained on commercial farms failed to confirm these differences, which points to the existence of other factors (related to the animals, feeding or other conditions of the machine and/or milking routine used) that may have a greater influence on the level of lipolysis of the milk than the mere fact of milking in ML or LL. No differences were found in other milk quality parameters, or were sensory defects in the enzymatic coagulation cheeses perceptible by consumers. There is, therefore, no reason to discourage farmers from this type of milking setup, provided that the correct functioning and management of the milking operation and milk storage on the farm is guaranteed.
Financial support
This research received no specific grant from any funding agency, commercial or not-for-profit sectors.
Conflicts of interest
None.
Ethical standards
Not applicable.