Introduction
The world population has been parallel to ammonia synthesis from atmospheric paired nitrogen fixed by the Haber-Bosch process in the early 20th century (Fig. 1). According to a quantitative estimation, approximately half of the global population seems to depend on synthetic ammonia fertilizers (Erisman, et al., Reference Erisman, Galloway, Klimont and Winiwarter2008). Hence, it follows that currently 3.9 billion population depend on the synthetic nitrogen fertilizer. However, the deposition of nitrogen from the atmosphere on terrestrial surfaces was estimated at 125 Tg/year in the early 21st century (Gruber & Galloway, Reference Gruber and Galloway2008). Most (0.8) of the deposition is emitted from industrially fixed nitrogen and the remaining 0.2 is emitted from the combustion of fossil fuels. Once the stable paired nitrogen (N2) in the atmosphere is chemically transformed to ammonia and urea as nitrogen fertilizers, the reactive nitrogen compounds are no longer under control owing to nitrification and denitrification in the soil, hydrosphere or atmosphere. Thus, these chemical transformations in reactive nitrogen must be controlled during the ammonia stage. Thus, it is important to reduce the amount of ammonium nitrogen (NH4+-N) in the environment, reduce the influx of NH4+-N into the environment, and promote the recycling of NH4+-N.

Fig. 1. Parallel increase in the world population to the global increase in nitrogen input (FAOSTAT, 2021).
Some harmful nitrogenous intermediates are formed and emitted through biochemical reactions, including NO3− and nitrite (NO2−) in the soil and then the hydrosphere due to leaching (Takahashi, Reference Takahashi, Currie and Hanley2006). High concentrations of NO3− absorbed as a nutrient by grasses and other plants often cause NO3−-NO2− poisoning and methemoglobinemia caused by nitrate-reducing bacteria in the rumen of ruminants (Takahashi et al., Reference Takahashi, Johch and Fujita1989). However, the reduction of nitrate in the rumen inhibits methane production by rumen methanogens, another greenhouse gas, owing to hydrogen uptake (Takahashi and Young, Reference Takahashi and Young1991). Nitrogen oxides (NOx) are derived from the excess amount of nitrogen fertilizers and manures such as nitric oxide (NO), which contributes to the acidity of rainwater, and nitrous oxide (N2O), which is an ozone depletion substance in the stratosphere and is a powerful greenhouse gas, although both gases play important roles in medical physiology (Mennerick, et al., Reference Mennerick, Jevtovic-Todorovic, Todorovic, Shen, Olney and Zorumski1998; Rosselli, et al., Reference Rosselli, Keller and Dubey1998).
Improper management of livestock wastewater causes eutrophication in the hydrosphere due to NO3− and N2O emissions in the atmosphere, which is attributed to the excess amount of NH4+-N. It is a common issue in Asian and African developing and emerging countries, where abrupt population expansion and urbanization have progressed along with economic development (Nyenje, et al., Reference Nyenje, Foppen, Uhlenbrook, Kulabako and Muwanga2010; Lin, et al., Reference Lin, Shen, Zhou and Lyu2021). Thus, excessively fixed reactive NH4+-N should eventually return to atmospheric N2 through complete nitrification and denitrification without the deposition and emission of any pollutant nitrogenous intermediates. To achieve this, it is fundamentally necessary to reduce the excessive input of nitrogen into the environment. NH4+-N, which is a pollutant and a burden on the environment, must be removed by efficient nitrification and denitrification. Most biological approaches to ammonia removal from livestock wastewater have conventionally been implemented by aerobic nitrification and anaerobic denitrification using heterotrophs and autotrophs (Carrera et al., Reference Carrera, Baeza, Vicent and Laufuente2003). However, autotrophic bacteria are presumably unsuitable for livestock wastewater treatment because of the high concentrations of ammonium and organic matter (Ruiz et al., Reference Ruiz, Jeison and Chamy2003). Furthermore, the long retention time of autotrophic nitrification has been attributed to the slow proliferation rate of bacteria (Richardson and Watmouth, Reference Richardson and Watmouth1999). In an attempt to determine the biological ammonia removal ability, Joo et al. (Reference Joo, Hirai and Shoda2005a, Reference Joo, Hirai and Shoda2005b) isolated heterotrophic bacteria, A. faecalis strain No.4, from sewage sludge, which has heterotrophic nitrification and aerobic denitrification abilities. They demonstrated that A. faecalis strain No.4 could achieve prompt removal of ammonia from piggery wastewater and efficient denitrification from the removed ammonia under high-strength NH4+-N and chemical oxygen demand (COD) (Joo et al., Reference Joo, Hirai and Shoda2006).
The present study deals with a feasibility study in A. faecalis strain No.4 removes NH4+-N from piggery effluent water according to Joo et al. (Reference Joo, Hirai and Shoda2006) and consequently could improve river water quality polluted by flowing piggery effluent into the river.
Materials and methods
First, the properties of pH, dissolved oxygen (DO) and liquid temperature were surveyed in wastewater containing effluent from a piggery beside a tributary of the Yangtze River located in the suburb of Shanghai, China (Fig. 2). Within a 20 km radius from east to west of this river basin, there is a concentration of 107 piggeries, including the piggery surveyed in this study. There are several factory complexes in the river basin, including textile factories, but industrial wastewater does not flow into the river. Thus, annual changes in water quality (temperature, pH, DO and EC) in the downstream most reaches of the study area were monitored.

Fig. 2. Colour online. Location of a surveyed piggery and water qualities in the river basin.
In general, piggeries in this area manage manure without solid-liquid separation. The liquid waste mixed with cleaning water from livestock barns is dumped into the river through a drainage ditch, leading to a tributary stream, and the solid part that settles in the drainage ditch is used as a fertilizer after it dries naturally. Water quality in terms of pH, DO, liquid temperature, and electrical conductivity (EC) were measured upstream and downstream of the piggery.
Subsequently, NH4+-N removal from piggery wastewater contaminated with swine effluent was performed using A. faecalis strain 4. (Shoda & Hirai, Reference Shoda and Hirai2006). Table 1 shows the culture medium used for the growth and cultivation of A. faecalis strain 4. Table 2 shows the composition of trace elements added to the medium. The cultured cells of A. faecalis strain No.4 were mixed with 50% glycerol solution and stored at −84°C. The preparation and characteristics of A. faecalis strain No.4 were determined according to the procedure described by Joo et al. (Reference Joo, Hirai and Shoda2006).
Table 1. Culture medium for Alcaligenes faecalis strain No.4

Table 2. Culture medium for Alcaligenes faecalis strain No.4

Figure 3 shows a small-scale (working volume 300 ml) jar fermenter (BMJ-01PI, Able Corp., Tokyo) and the sensors used. To determine the optimal incubation conditions to remove NH4+-N derived from piggery effluent, the DO concentration and pH were continuously monitored using a DO sensor (SDOC-12F, Able Corp., Tokyo) and pH sensor (Easyferm Plus 225, Hamilton Bonaduz AG, Bonaduz). NH4+-N concentration was monitored using an ammonium sensor (SNH-10, Able Corp., Tokyo). The aeration rate was set at 300 ml/min and the temperature was maintained at 30°C. The agitation speed was set at either 400 rpm (rotation per minute) or 700 rpm. Ammonia removal experiments were carried out by the addition of 45 ml A. faecalis strain No. 4 and 2 ml defoaming agent with citric acid (denoted as S in figures) or without citric acid (denoted as C in figures (Control) as a carbon source to 255 ml piggery sample fluid. When vigorous foaming occurred, the defoaming sensor deformed the culture.

Fig. 3. Colour online. Fermenter (Type: BMJ-1L, ABLE Corp. Tokyo Japan) and sensors attached to the reactor.
Results
The wastewater quality in the piggery was indicated such as pH 8.44, DO 0.28 mg/l, fluid temperature 9.5°C, chemical oxygen demand Cr (CODcr) 2160 mg, biochemical oxygen demand (BOD) 1020 mg/l, and NH4+-N 1100 mg/l. The total content of the observed acids (oxalic acid, citric acid, lactic acid, formic acid, acetic acid, propionic acid, iso-butyric acid, and butyric acid) was approximately 1000 mg/l. These organic acids were the main carbon sources for A. faecalis strain No. 4. For the upstream water quality, pH 6.85, EC 472 μS/cm, DO 5.12 mg/l and fluid temperature 9.5°C were observed. In downstream water, pH 6.83, EC 470 μS/cm, DO 4.32 mg/l and fluid temperature 9.5°C were quantified. The EC values in the upstream and downstream areas were slightly above the surface water standard limit (400 μS/cm). The DO concentrations were below the WHO (2011) standard limit for drinking water and below the surface water standard limit (6 mg/l); however, the lower values in the downstream area indicated slightly higher contamination (Mahadevan et al., Reference Mahadevan, Krishnan, Pillai and Sudhakaran2020).
Table 3 shows annual changes in water quality (temperature, pH, DO and EC) in the downstream most reaches of the study area. Water temperature in rivers is affected by temperature and has large seasonal variations, but DO also fluctuates widely. A negative correlation (−0.61, P < 0.05) was found between water temperature and DO (Table A1 Appendix). The average river DO throughout the year was 4.82 mg/l, below the lower limit of the guideline. The annual mean value of EC was 527 μS/cm, well above the standard value.
Table 3. Annual changes in water quality (temperature, pH, DO and EC) in the downstream most reaches of the study area

Figure 4 shows the effect of 20 g/l sodium citrate addition on ammonia removal by A. faecalis strain No.4 at an aeration rate of 300 ml/min, temperature of 30°C, agitation speed of 400 rpm and initial pH of 8.9. There was no significant difference in the change in ammonia concentration between the experimental (S) and control (C) incubations. However, DO in C levelled off in 20 h, but in S, the gradual decline in DO indicated continuous consumption of oxygen by A. faecalis strain No.4 using citrate as a carbon source, which reflected a constant decrease in ammonia concentration to 0 in 70 h. The initial pH value of 8.9 in the sample fluid was relatively high and inhibited the activity of A. faecalis strain No.4, and the pH increased to 9.0. This led to decreased activity of A. faecalis strain No.4, especially for A. faecalis strain No.4 in C. Total organic acid content in the original wastewater of approximately 10 g/l was presumably consumed in 20 h.

Fig. 4. Changes in ammonia removal by A. faecalis strain NO. 4 and DO at 300 ml/min of aeration rate, at 30°C and 400 rpm of agitation speed (Initial pH8.9) with or without 20 mM citrate.
Figure 5 shows the effect of the initial pH 8.0 and 10 g citrate addition on ammonia removal by A. faecalis strain No.4. The other operational conditions are the same as those shown in Fig. 4. The progressive removal of ammonia by active A. faecalis strain No.4 in S medium was confirmed because oxygen deficiency was observed after 10 h of incubation. At 20 h, an increase in DO corresponded to almost complete exhaustion of the carbon sources. Foaming is not a problem. To prevent oxygen deficiency in the bacteria, the agitation speed was set at 700 rpm, and the initial pH was adjusted to 8.0 in the next experiment.

Fig. 5. Changes in ammonia removal and DO at 300 ml/min of aeration rate, at 30°C, and 400 rpm of agitation speed (Initial pH8.0) with or without 10 mM citrate.
Figure 6 shows the effect of adding 10 g/l citrate on the ammonia removal effect of A. faecalis strain No.4 at an aeration rate of 700 ml/min, temperature of 30°C, agitation speed of 300 rpm and initial pH of 8.0. There was no significant improvement in the removal rate of ammonia due to heavy foaming.

Fig. 6. Changes in ammonia removal and DO at 300 ml/min of aeration rate, at 30°C, and 700 rpm of agitation speed (Initial pH8.0) with or without 10 mM citrate.
Discussion
A survey of annual changes in water quality (temperature, pH, DO and EC) collected in the downstream most reaches of the study area indicated a relatively higher value of EC and lower value of DO than the WHO standard (WHO, 2011). DO was shown to be negatively affected by water temperature, but the variability of these values appears to depend on the amount of piggeries effluent flowing into the river. DO was lower upstream than downstream of the drainage outlets of the piggery surveyed, but there was little difference in EC. This suggests that the entire river area under study is already being contaminated by piggeries effluent. Because the effluent polluted with swine manure from the piggeries adjacent to the river flows into the river, nitrogen compounds such as ammonia derived from livestock manure or its oxidation product, NO3−-N, are thought to have increased the concentration of dissolved ions, resulting in effluent with high EC. This suggests that ammonia in the effluent is one of the major factors for NO3−-N contributing to river eutrophication in rivers (Tedengren, Reference Tedengren2021). Furthermore, the emission of N2O into the atmosphere due to the reduction of NO3− produced from ammonia derived from livestock manure is considered to contribute to global warming as a powerful greenhouse gas along with ozone layer depletion (Torres, et al., Reference Torres, Simon, Rowley, Bedmar, Richardson, Gates and Delgado2016). Therefore, the removal of ammonia from swine effluent is an important issue for environmental health, not only in the hydrosphere but also in the atmosphere. Biological denitrification is an environmentally friendly method for treating wastewater containing livestock manure. Thus, ammonia removal from piggery wastewater using A. faecalis strain No.4 was conducted under three conditions. From the results of three different culture tests each removal rate of NH4+-N was calculated as follows, 1st culture test (Fig. 3): 0.35 kg N/m3/day, 2nd culture test (Fig. 4): 0.97 kg N/m3/day and 3rd culture test (Fig. 5): 0.70 kg N/m3/day. These values were similar to those obtained in Japanese piggery wastewater treatment (Joo et al., Reference Joo, Hirai and Shoda2006) despite the different qualities of the wastewater in different places. These values are more than 100 times higher than those of the conventional nitrification and denitrification methods. A. faecalis strain No.4 has the following mechanism (Joo et al., Reference Joo, Hirai and Shoda2005a).
NH4+ → Hydroxylamine (NH2OH) → N2O → N2
The production of N2O was reported only by < 0.01 of used ammonia, and almost no NO2− and NO3− were produced in the process. Moreover, the growth rate of A. faecalis strain No.4 was more than a few hundred times higher than that of nitrification bacteria. Thus, a higher proliferation rate leads to a smaller treatment reactor and a higher treatment rate when A. faecalis strain No.4 was used. Furthermore, approximately 0.4 of NH4+-N is used as N2 gas, and the remaining 0.6 is used for microbial protein synthesis to form the cell mass of A. faecalis strain No.4 (Joo et al., Reference Joo, Hirai and Shoda2005c). This indicates that cell mass production is larger than that in the conventional biological denitrification process.
The conventional biological denitrification method consists of a nitrification process using aerobic nitrifying bacteria and a denitrification process using facultative anaerobic denitrifying bacteria. Therefore, an aeration-capable reaction tank for increasing DO in the nitrification process and an anaerobic tank for the denitrification process are required.
In contrast, the biological denitrification system using A. faecalis strain No. 4 requires only one aerobic reaction tank that can be aerated. Another property of A. faecalis strain No.4 has been reported to effectively inhibit the growth of plant pathogenic fungi (Honda et al., Reference Honda, Hirai, Ano and Shoda1998).
In consequence, an aerobic one-step denitrification system using A. faecalis strain No.4 can be proposed to remove ammonia and phytopathogens from piggery wastewater with high efficiency and prevent water pollution in adjacent rivers (Fig. 7).
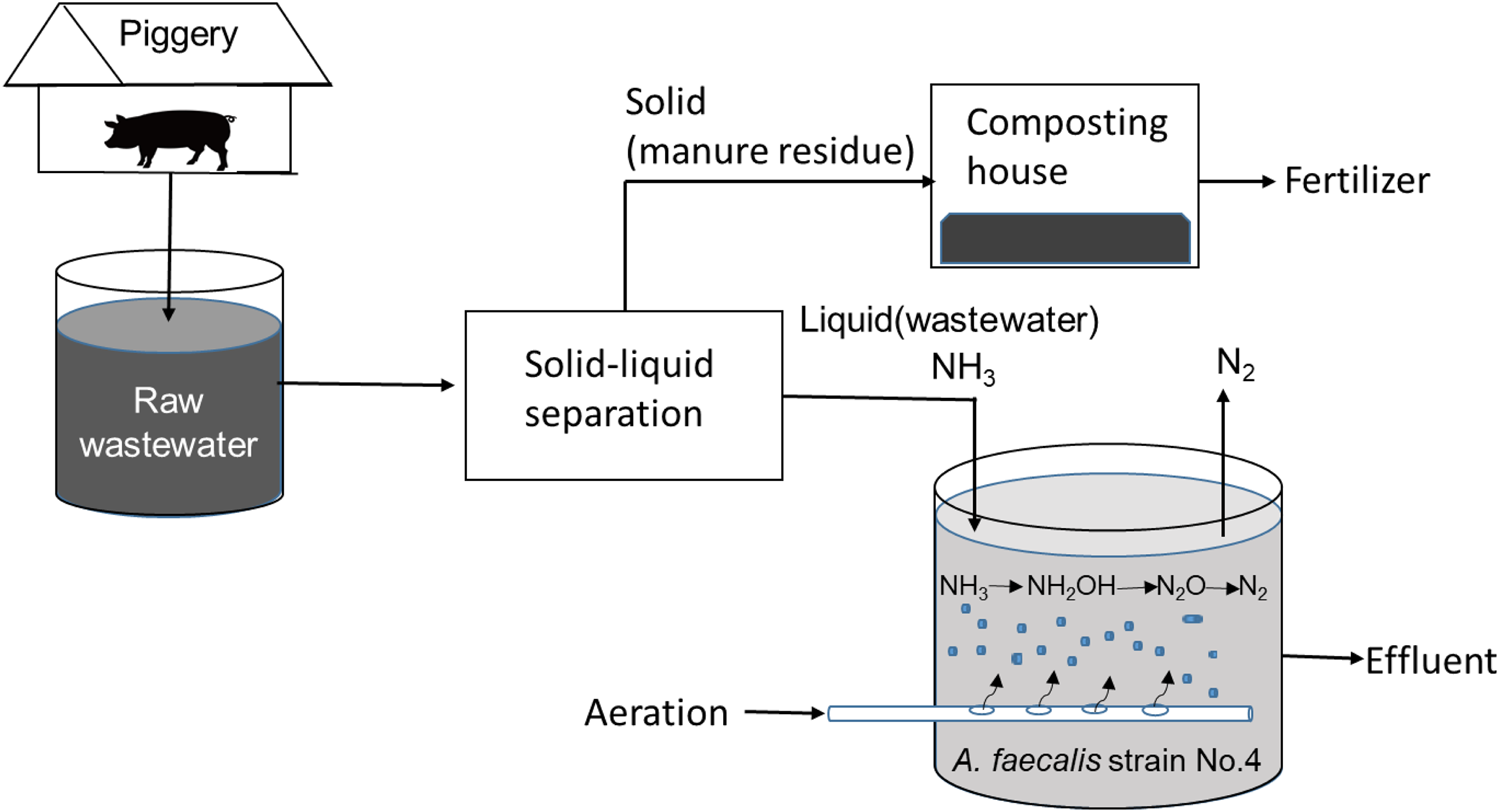
Fig. 7. Biological denitrification system with an only aerobic one-step process using A. faecalis No. 4 strain.
Acknowledgements
We acknowledge Able Corporation (Tokyo) for providing us with a reactor system.
Author contributions
J. Takahashi, M. Shoda, J. Li and N. Li conceived and designed the study. M. Shoda, J. Li and N. Li gathered the data. J. Takahashi and M. Shoda wrote the manuscript.
Financial support
This research received no specific grant from any funding agency, commercial or not-for-profit sectors.
Conflict of interest
The authors declare there are no conflicts of interest.
Ethical approval
Not applicable.
Appendix
Table A1. Correlation coefficients and their P values among the parameters in river water qualities
