Introduction
Ichnology of deposits exposed subaerially started its theoretical development about 50 years later than ichnology from subaquatic environments (e.g. Ekdale et al., Reference Ekdale, Bromley and Pemberton1984; Genise, Reference Genise2016). In contrast to trace fossils from marine environments, paleosol trace fossils involving new ichnotaxa and architectural designs have been mostly discovered in recent years and are still found in large numbers (e.g. Genise, Reference Genise2016; Genise and Harrison, Reference Genise and Harrison2018). How is this related to Mars? Although some Martian paleosols have been described (e.g. Retallack, Reference Retallack2014), the probability of finding trace fossils there is almost null. The relationship in which this contribution is based is more methodological. Paleosol ichnology and ichnologists usually deal with structures that can be barely recognized as trace fossils or separated from inorganic structures. The relatively yet underexplored field of paleosol trace fossils involves the analysis of a large array of ‘strange things’ found in terrestrial rocks that need to be tested for ichnogenicity, i.e. if the objects under study are morphologically recurrent structures resulting from the life activity of an organism modifying the substrate; if they agree with the current definition of trace fossils (Bertling et al., Reference Bertling, Braddy, Bromley, Demathieu, Genise, Mikuláš, Nielsen, Nielsen, Rindsberg, Schlirf and Uchman2006). In some cases, ichnogenicity has to be analysed with the same (or less) means than those of the exploration of Mars offers: unique pictures from almost inaccessible localities. As paleosol ichnologists, we usually deal with many inquiries of ‘strange things’ in terrestrial deposits that cannot be classified. Thus, ichnogenicity tests are performed on a regular basis. This usual practice places paleosol ichnology in an advantageous position to search for and to analyse trace-like structures in Mars images.
As an example of this methodology, this contribution will focus on one of the most discussed evidence of putative trace fossils until now, recovered from the NASA's Martian Science Laboratory (MSL) rover Curiosity: the so dubbed ‘stick-like structures’ (David, Reference David2018; DiGregorio, Reference DiGregorio2018; Howell, Reference Howell2018a, Reference Howellb; Baucon et al., Reference Baucon, Neto De Carvalho, Felletti and Cabella2020) (Fig. 1). A detailed analysis of these structures by Baucon et al. (Reference Baucon, Neto De Carvalho, Felletti and Cabella2020) concluded that ‘ichnofossils are among the closest morphological analogues of these features [stick-like structures]’. Also, these authors claimed that there is a need to establish other criteria for demonstrating the biogenic origin of trace-like structures. The protocol presented herein, involving some data and aspects not considered in previous discussions, contributes to these further analyses.
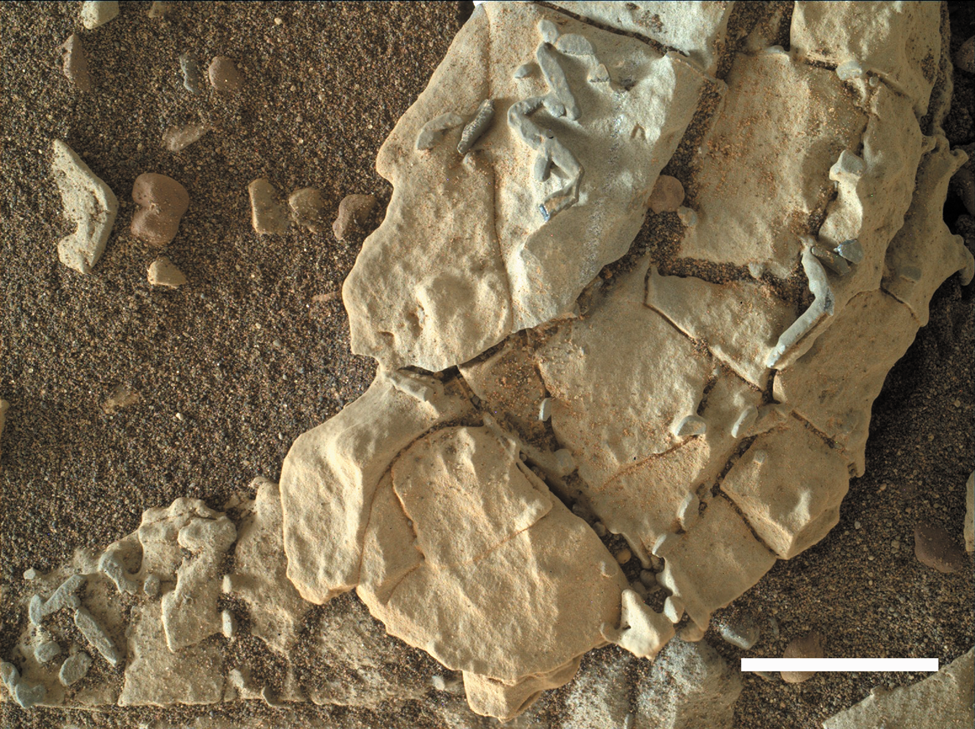
Fig. 1. Stick-like structures from the Murray Formation at Vera Rubin Ridge in Gale Crater. Mars. Image 1923MH0007280000703207R00_DXXX from the Curiosity rover using MAHLI. Scale bar: 1 cm.
For this reason, the objective of this contribution is to analyse the ‘stick-like structures’ in light of several criteria synthesized from paleosol ichnology for the first time as a protocol (i.e. a series of complementary analysis) for conducting an ichnogenicity test. Another objective is to call attention to the potential usefulness of this protocol to test structures in the search for trace fossils, and consequently life, on Mars. Even when this protocol is synthesized from paleosol ichnology and the examples used here come from this field, it may also be used in trace-like structures from any paleoenvironment on Earth or Mars since it is based exclusively on morphology and context. This protocol has a different approach from those of biogenicity (e.g. McLoughlin et al., Reference McLoughlin, Brasier, Wacey, Green and Perry2007; Brasier and Wacey, Reference Brasier and Wacey2012), since it is based almost exclusively on morphology lacking the chemical approach of the biogenicity tests. In a way, it may be seen as a preliminary and simpler test completely focussed on demonstrating that the structures under study fulfil all the requirements to be considered trace fossils, to proceed eventually with more complex analyses of biogenicity.
Material and methods
The studied material is rocks photographed by the NASA's Mars Science Laboratory (MSL) Curiosity rover during Sols 1905, 1910, 1911, 1912 and 1922 in lacustrine deposits of the Jura Member from the Murray Formation at Vera Rubin Ridge in Gale Crater (Heydari et al., Reference Heydari, Parker, Calef, Schroeder, Van Beek, Rowland and Fairen2018; Frydenvang et al., Reference Frydenvang, Mangold, Wiens, Fraeman, Edgar, Fedo, L'Haridon, Bedford, Gupta, Grotzinger, Bridges, Clark, Rampe, Forni, Gasda, Lanza, Olilla, Meslin, Payré, Calef, Salvatore and House2019a, Reference Frydenvang, Mangold, Wiens, Fraeman, Edgar, Fedo, L'Haridon, Bedford, Gupta, Grotzinger, Bedford, Bridges, Clark, Rampe, Forni, Gasda, Lanza, Olilla, Meslin, Payré, Calef and Salvatoreb; Edgar et al., Reference Edgar, Fedo, Gupta, Banham, Fraeman, Grotzinger, Stack, Stein, Bennett, Rivera-Hernandez, Sun, Edgett, Rubin, House and Van Beek2020; L'Haridon et al., Reference L'Haridon, Mangold, Fraeman, Johnson, Cousin, Rapin, David, Dehouck, Sun, Frydenvang, Gasnault, Gasda, Lanza, Forni, Meslin, Schwenzer, Bridges, Horgan, House, Salvatore, Maurice and Wiens2020; Bennett et al., Reference Bennett, Rivera-Hernández, Tinker, Horgan, Fey, Edwards, Edgar, Kronyak, Edgett, Fraeman, Kah, Henderson, Stein, Dehouck and Williams2021). The stick-like structures occur on the rock target named Haroldswick. Complementary images of loose structures on Mars surface, just for morphological comparisons, also come from the rover Curiosity on Sol 1278 at the Naukluft Plateau, and from the NASA's Mars Exploration Rover (MER) Opportunity taken in the Endeavour Crater during Sol 3415 at Solander Point from the surface target ‘Dibbler’, and during Sol 4194 at Marathon Valley, from the surface target ‘Pvt. Ebenezer Tuttle’. All Martian images were downloaded from the NASA's official site and some of them were enhanced and cropped for illustrative purposes.
The protocol applied was to check different aspects of the structures shown in photographs following a series of complementary analysis and to evaluate the Martian geological context following the synthesis of criteria used in ichnology (Genise, Reference Genise2016). This set of criteria includes morphological regularity, morphological completeness, morphological dispersion, morphological recurrence, morphological complexity, surface morphology and context. This protocol is proposed exclusively for trace fossils sensu stricto as defined by Bertling et al. (Reference Bertling, Braddy, Bromley, Demathieu, Genise, Mikuláš, Nielsen, Nielsen, Rindsberg, Schlirf and Uchman2006) that excludes microbially induced sedimentary structures, stromatolites and other biogenic sedimentary structures.
Results and discussion
Morphological regularity
The regularity of shape is probably the principal prerequisite of a structure in a rock to suggest its organic origin. Trace fossils, as remains of life, are usually associated with regular shapes (Fig. 2(A)). However, some inorganic structures also show regular shapes and may be confused with trace fossils or biological entities on Earth (Seilacher, Reference Seilacher2001; Brasier and Wacey, Reference Brasier and Wacey2012; Genise, Reference Genise2016). Its regular shape is not enough if we consider the wide array of inorganic structures that also show regular shapes. Although recognized as one of the most important ichnotaxobases, and the basal stone of the ichnotaxonomical system (Bertling et al., Reference Bertling, Braddy, Bromley, Demathieu, Genise, Mikuláš, Nielsen, Nielsen, Rindsberg, Schlirf and Uchman2006) alone as a character may be misleading in ichnology (Genise, Reference Genise2016). On Mars, ‘blueberries’ have been a paradigmatic study case of how a regular shape has encouraged a lot of discussions about the origin, unsolved yet, of a Martian structure (e.g. Catling, Reference Catling2004; Chan et al., Reference Chan, Beitler, Parry, Ormö and Komatsu2004; Squyres et al., Reference Squyres, Arvidson, Bell III, Brückner, Cabrol, Calvin, Carr, Christensen, Clark, Crumpler, Des Marais, d'Uston, Economou, Farmer, Farrand, Folkner, Golombek, Gorevan, Grant, Greeley, Grotzinger, Haskin, Herkenhoff, Hviid, Johnson, Klingelhöfer, Landis, Lemmon, Li, Madsen, Malin, McLennan, McSween, Ming, Moersch, Morris, Parker, Rice, Richter, Rieder, Sims, Smith, Smith, Soderblom, Sullivan, Wänke, Wdowiak, Wolff and Yen2004; Grotzinger et al., Reference Grotzinger, Arvidson, Bell III, Calvin, Clark, Fike, Golombek, Greeley, Haldemann, Herkenhoff, Jolliff, Knoll, Malin, McLennan, Parker, Soderblom, Sohl-Dickstein, Squyres, Tosca and Watters2005; McLennan et al., Reference McLennan, Bell III, Calvin, Christensen, Clark, de Souza, Farmer, Farrand, Fike, Gellert, Ghosh, Glotch, Grotzinger, Hahn, Herkenhoff, Hurowitz, Johnson, Johnson, Jolliff, Klingelhöfer, Knoll, Learner, Malin, McSween, Pocock, Ruff, Soderblom, Squyres, Tosca, Watters, Wyatt and Yen2005; Calvin et al., Reference Calvin, Shoffner, Johnson, Knoll, Pocock, Squyres, Weitz, Arvidson, Bell, Christensen, de Souza, Farrand, Glotch, Herkenhoff, Jolliff, Knudson, McLennan, Rogers and Thompson2008; Marion et al., Reference Marion, Kargel and Catling2008; Rizzo and Cantasano, Reference Rizzo and Cantasano2009; Stack et al., Reference Stack, Grotzinger, Kah, Schmidt, Mangold, Edgett, Summer, Siebach, Nachon, Lee, Blaney, Deflores, Edgar, Fairén, Leshin, Maurice, Oehler, Rice and Wiens2014; McMahon et al., Reference McMahon, Bosak, Grotzinger, Milliken, Summons, Daye, Newman, Fraeman, Williford and Briggs2018; Sun et al., Reference Sun, Stack, Kah, Thompson, Fischer, Williams, Johnson, Wiens, Kronyak, Nachon, House and VanBommel2018; Misra and Acosta-Maeda, Reference Misra, Acosta-Maeda and Al-Juboury2018).
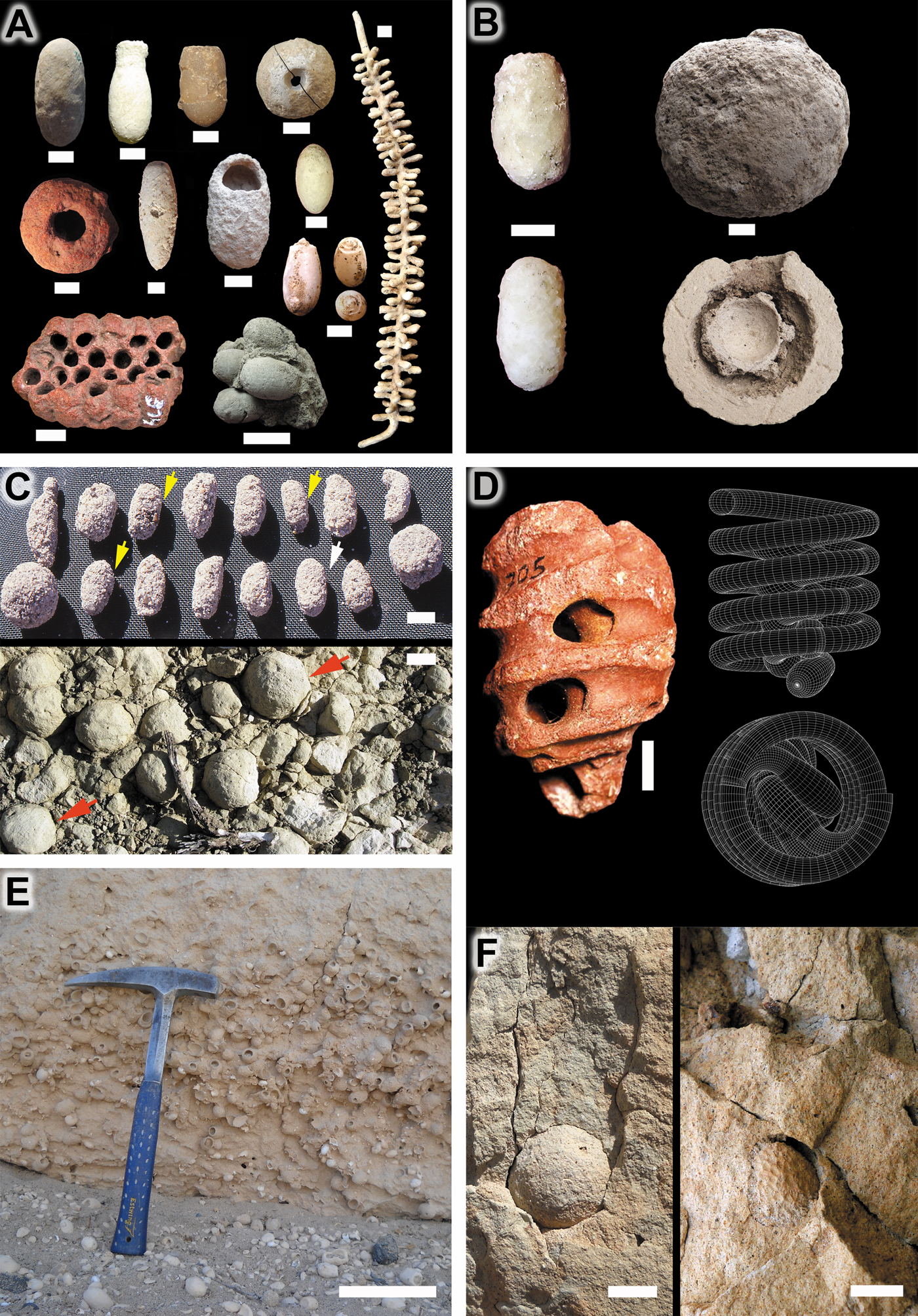
Fig. 2. Features of paleosol trace fossils and comparable structures. (A) Morphological regularity of some trace fossils from paleosols. (B) Completeness. Left column, on top an incomplete, truncated, specimen of Fictovichnus (chafer pupation chamber) that may be confused (below) with a specimen of Celliforma (bee cell). Right column, on top the external, little informative, aspect of a specimen of Coprinisphaera contrasting with its internal complex morphology (below). (C) Dispersion. On top, a collection of armoured clay nodules. Arrows indicate the most regular ones that may be confused with the trace fossil Rebuffoichnus. Below, a group of inorganic concretions, where arrows indicate two more regular spheroidal ones that may be confused with Coprinisphaera. (D) Complexity. Elipsoideichnus meyeri from the Eocene of Uruguay shows an extremely complex morphology. (E) Recurrence. A high concentration of Rebuffoichnus from the Pleistocene of Canary Islands. (F) Surface morphology. On the left a spheroidal concretion showing a smooth surface, on the right, a specimen of Pallisphaera puertai showing a pelletal surface morphology, both from the early Cretaceous of Argentina. All bar scales: 1 cm except for (E): 10 cm.
The sticks probably meet this prerequisite of showing a regular and singular morphology if we consider the interest that they aroused. However, the degree of subjectivity in this interest is contrasted by the lack of interest in other Martian structures pictured by the Opportunity MI and the Curiosity MAHLI that are comparable in shape with terrestrial trace fossils and received no attention. Figure 3(A) and (C) shows apparently cylindrical, or somewhat flattened, linear structures from Mars that are compatible with rhizoliths or Planolites (Fig. 3(B) and (D)). The first images from Perseverance Sherloc-Watson camera show comparable linear structures mixed with rounded clasts on Martian surface. Figure 3(E) shows a drop-like structure compatible with Celliforma (Fig. 3(F)). Figure 3(G) shows a structure composed of a bulbous end prolonged in an irregular cylinder, similar to a specimen of Fictovichnus showing an emergence tunnel (Fig. 3(H)). In contrast to the ‘stick-like structures’, these and other regular structures scattered among the images taken by the Curiosity and the Opportunity rovers did not attract any attention or discussions, demonstrating a high degree of subjectivity in the interest to analyse possible astrobiological targets.
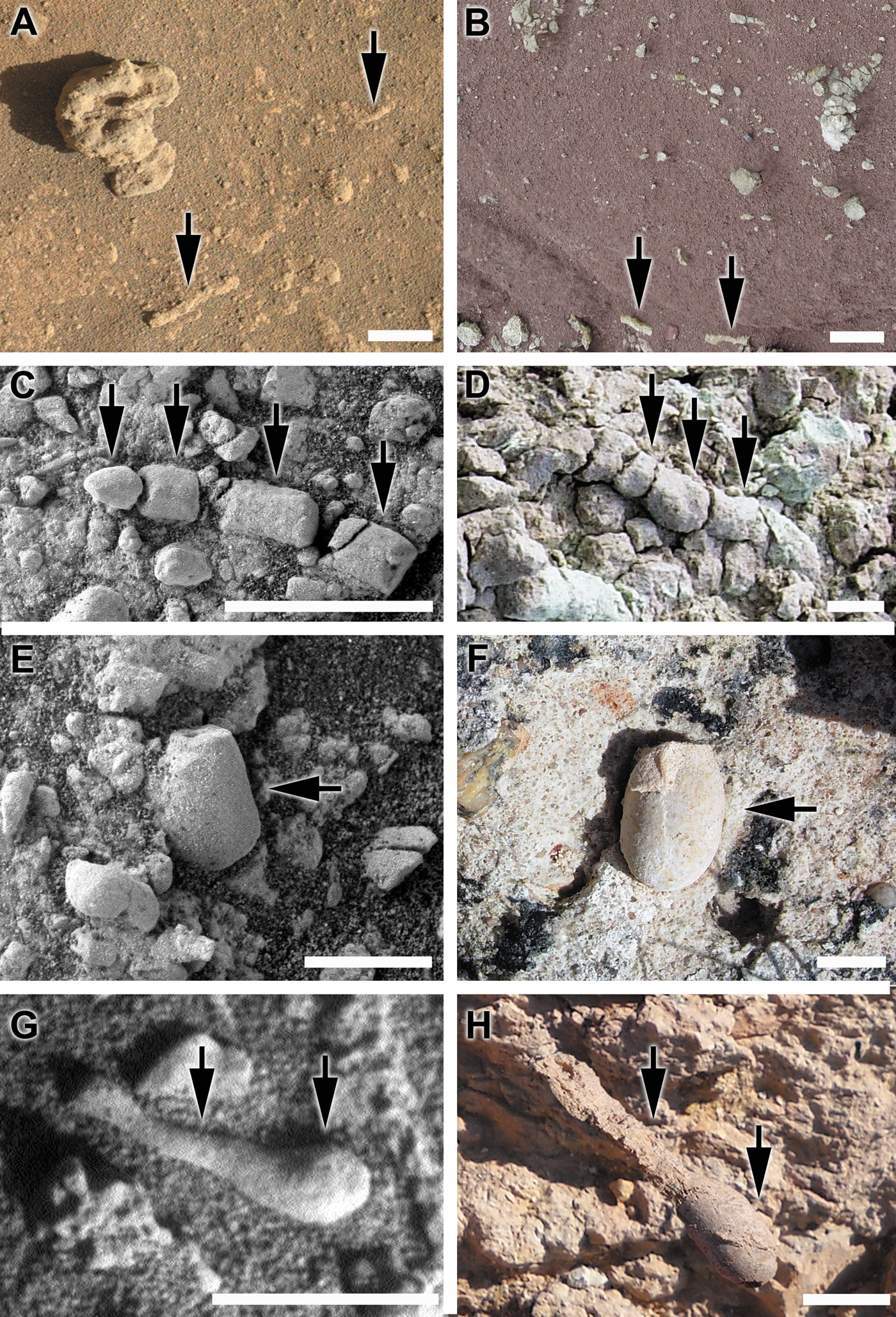
Fig. 3. Structures imaged on Mars compared to Earth trace fossils. (A) Linear structures (arrows) scattered on Mars surface at the Naukluft Plateau. Image 1278MH0001900010404672C00_DXXX from MAHLI of Curiosity rover. Scale bar: 1 cm. (B) Loose and broken rhizoliths (arrows) from the Oligocene Chilapa Formation of Mexico. Scale bar: 5 cm. (C) Cracked tubular structure (arrows) from the surface target ‘Pvt. Ebenezer Tuttle’ at Marathon Valley, Mars. Image 1M500516263EFFCONIP2935M2M1 from the MI of the Opportunity rover. Scale bar: 1 cm. (D) Cracked invertebrate burrow (arrows) from the Miocene Pinturas Formation of Argentina. Scale bar: 1 cm. (E) Structure showing a rounded and a flat extreme (arrow) from the surface target ‘Pvt. Ebenezer Tuttle’ at Marathon Valley, Mars. Image 1M500516482EFFCONIP2936M2M1 from the MI of the Opportunity rover. Scale bar: 5 mm. (F) Celliforma (arrow) from the Cretaceous Mercedes Formation of Uruguay. Scale bar: 1 cm. (G) Structure showing a bulbous end (right arrow) followed by an irregular cylinder (left arrow) from the surface target ‘Dibbler’ at Solander Point, Mars. Image 1M431365143EFFC7KDP2955M2M1 from the MI of the Opportunity rover. Scale bar: 5 mm. (H) Irregular tunnel (left arrow) ended in a specimen of Fictovichnus (right arrow) from the Miocene Calatayud-Daroca Basin of Spain. Scale bar: 1 cm.
To avoid such subjectivity in the process of target selection, it would be advisable that after the first impression that any trace-like structure on Mars surface may produce, the simple protocol presented herein is carried out before initiating more complicated research or cataloguing a structure as a target for astrobiological research.
Morphological completeness
Shape analyses should be based on the full structure of the most complete specimens and also on all the available and complementary evidence that other incomplete specimens can yield. Figure 2(B) shows two examples of trace fossils from paleosols in which the completeness of the structure is critical to identify and understand them. Fictovichnus are ellipsoid structures representing fossil beetle pupation chambers, whereas Celliforma are fossil bee cells having a rounded rear and a flat top. In outcrops, many specimens of Fictovichnus show a truncated end, produced by the beetle emergence, resembling Celliforma. The only way to identify the correct ichnotaxon and its affinities is studying as many specimens as possible, to look for the most complete specimens of Fictovichnus and to base the conclusions on them. Also, there are trace fossils that need to be studied internally to understand them. The external aspect of Coprinisphaera in many cases is just a spheroid as many inorganic concretions. The only way to be sure of its identification and to correctly describe specimens is to section them longitudinally to evaluate their complete morphology (Fig. 2(B)).
Figure 4 shows three remains (arrows), which were probably part of one of the most complete stick-like structures at right and top. They are vertically oriented and attached to one side of a step in the rock that is probably one side of a former crack. The complete crack that is connected to the step is also observable below the fragments and to the right and up, below the stick-like structure. The hypothesis of these remains being simply fallen loose fragments of sticks is unlikely because of their vertical orientation that would not be very resistant to weathering, particularly in the case of the upper fragment whose base is mostly unsupported. These fragments are then in their original position and they are probably remains of a former crack fill. This suggests two possibilities, either these fragments are also vertical ‘sticks’, most probably crack fills, or they were part of a former stick showing a T-shaped cross-section that lost its upper horizontal component. Both possibilities are unlikely for trace fossils. This analysis is in agreement with the interpretation of the stick-like structures of Haroldswick as the outward growth of prismatic forms from fractures (Bennett et al., Reference Bennett, Rivera-Hernández, Tinker, Horgan, Fey, Edwards, Edgar, Kronyak, Edgett, Fraeman, Kah, Henderson, Stein, Dehouck and Williams2021).

Fig. 4. Three remains (arrows) vertically oriented, attached to one side of a step that is probably one side of a former crack observable below the fragments and more to the right and up below the stick-like structure. Image 1923MH0007280000703211R00_DXXX from the Curiosity rover using MAHLI. Scale bar: 1 cm.
These results indicate that the squarish cross-section proposed by Baucon et al. (Reference Baucon, Neto De Carvalho, Felletti and Cabella2020) results from a partial selection of fragments. In addition, the squarish cross-section can be hardly compared with that of few cases of very specialized trace fossils of marine environments on Earth as these authors recognized.
Morphological dispersion
The surroundings and stratigraphic level of any structure tested for ichnogenicity should be studied to know its morphological dispersion as far as possible. The objective is to look for possible unshaped forms that may indicate that the regular forms under study are just only subjectively chosen ones among a wide array of unshaped structures. Some classical examples from paleosol ichnology (Fig. 2(C)) involve the trace fossil Rebuffoichnus, which can be confused with armoured clay nodules that range from ellipsoidal to more unshaped ones or Coprinisphaera, which may be confused at first glance with spheroidal inorganic concretions (Genise, Reference Genise2016). The subjective selection of the most regular specimens may result in misleading conclusions.
This is the case of the stick-like structures. Far from being distinct structures they are part of the spectrum of other dark-toned diagenetic features typical of the Facies 3 of the Jura Member (Edgar et al., Reference Edgar, Fedo, Gupta, Banham, Fraeman, Grotzinger, Stack, Stein, Bennett, Rivera-Hernandez, Sun, Edgett, Rubin, House and Van Beek2020) and particularly from the grey outcrops (L'Haridon et al., Reference L'Haridon, Mangold, Fraeman, Johnson, Cousin, Rapin, David, Dehouck, Sun, Frydenvang, Gasnault, Gasda, Lanza, Forni, Meslin, Schwenzer, Bridges, Horgan, House, Salvatore, Maurice and Wiens2020; Bennett et al., Reference Bennett, Rivera-Hernández, Tinker, Horgan, Fey, Edwards, Edgar, Kronyak, Edgett, Fraeman, Kah, Henderson, Stein, Dehouck and Williams2021). Figure 5 shows a wide array of shapes of these dark-toned features, in images captured during Sols 1905–1922, when Curiosity travelled across an area ca 20 m long and 7.5 m wide where the same level of the sticks was exposed. The wide array of dark-toned, erosion-resistant, diagenetic features of the Vera Rubin Ridge includes angular crystal casts, nodules, crystals, fracture fills, and polygonal and elongated features (L'Haridon et al., Reference L'Haridon, Mangold, Fraeman, Johnson, Cousin, Rapin, David, Dehouck, Sun, Frydenvang, Gasnault, Gasda, Lanza, Forni, Meslin, Schwenzer, Bridges, Horgan, House, Salvatore, Maurice and Wiens2020; Bennett et al., Reference Bennett, Rivera-Hernández, Tinker, Horgan, Fey, Edwards, Edgar, Kronyak, Edgett, Fraeman, Kah, Henderson, Stein, Dehouck and Williams2021). The stick-like structures were defined as dark-toned polygonal and elongated features that protrude in positive relief along fracture planes (L'Haridon et al., Reference L'Haridon, Mangold, Fraeman, Johnson, Cousin, Rapin, David, Dehouck, Sun, Frydenvang, Gasnault, Gasda, Lanza, Forni, Meslin, Schwenzer, Bridges, Horgan, House, Salvatore, Maurice and Wiens2020, Fig. 7) or as prismatic features formed in association with fluids in the fine-scale fractures that may represent poikilotopic crystal growth (Bennett et al., Reference Bennett, Rivera-Hernández, Tinker, Horgan, Fey, Edwards, Edgar, Kronyak, Edgett, Fraeman, Kah, Henderson, Stein, Dehouck and Williams2021, Fig. 4g). In all cases, the dark tone of all these features is interpreted as the result of the high concentration of Fe in their composition, compatible with crystalline hematite and linked to postdepositional processes involving iron mobility (L'Haridon et al., Reference L'Haridon, Mangold, Fraeman, Johnson, Cousin, Rapin, David, Dehouck, Sun, Frydenvang, Gasnault, Gasda, Lanza, Forni, Meslin, Schwenzer, Bridges, Horgan, House, Salvatore, Maurice and Wiens2020; Bennett et al., Reference Bennett, Rivera-Hernández, Tinker, Horgan, Fey, Edwards, Edgar, Kronyak, Edgett, Fraeman, Kah, Henderson, Stein, Dehouck and Williams2021).
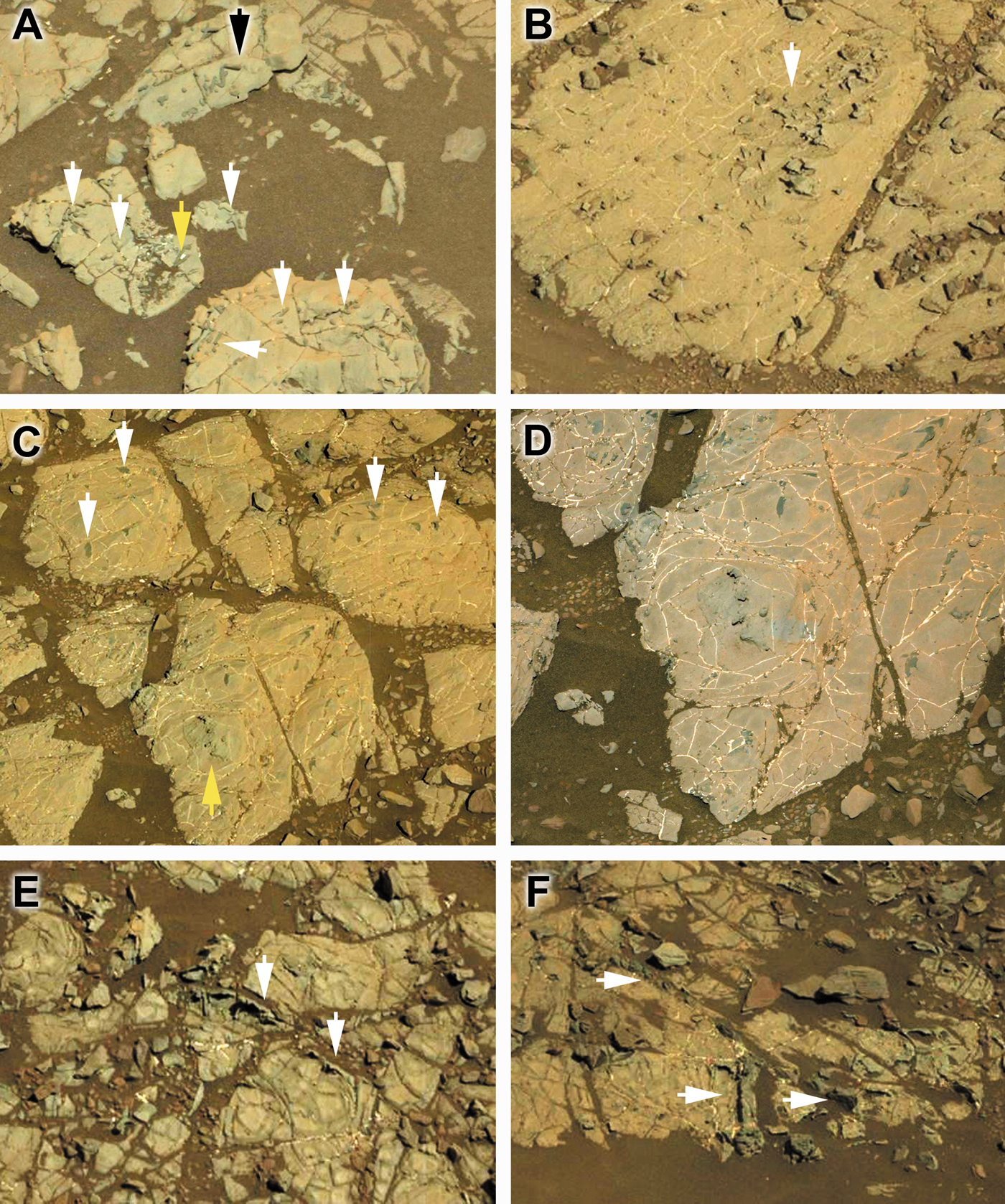
Fig. 5. Panoramic views of neighbouring rocks of Haroldswick showing dark-toned, positive-relief features similar to stick-like structures. Images obtained by the Mastcam of the Curiosity rover during Sols 1905–1922. (A) Rocks near the stick-like structures (black arrow) showing from elongated to unshaped dark-toned structures, some of them showing sharp contacts with the rock. Only some of them are indicated with white arrows, and also a Y-V shaped form (yellow arrow). Image 1905MR0099940220900105E01_DXXX. (B) A rock about 20 m from Haroldswick showing dark-toned structures with unshaped to nodular structures (white arrow) and loose fragments in the surroundings. Image 1910ML0100170050704064E01_DXXX. (C) Several rocks about 15 m from Haroldswick showing a full relief dark-toned spot (yellow arrow) and several unshaped to V-shaped forms in the surroundings, some of them indicated with white arrows. Image 1910ML0100170040704063E01_DXXX. (D) A close up to the rock depicted in (C). Image 1911MR0100210000900178E01_DXXX. (E) A rock about 8 m from Haroldswick showing a straight to gently curved fracture fill preserved in full relief (white arrow). Image 1922ML0100350120704450E01_DXXX. (F) Close to (E) several dark-toned fracture fills preserved as full relief structures. Image 1922ML0100350130704451E01_DXXX.
The stick-like structures are just the result of the hazardous preservation of one of these diagenetic features resembling a curious zigzagging pattern that subjectively captured some attention among a spectrum of more unshaped remains.
Morphological recurrence and complexity
A very significant aspect to be analysed for considering a structure as a trace fossil is its recurrence, unless its morphology is so complex or comparable with extant traces that leave no doubt on its affinities (Genise, Reference Genise2016). The latter case may be illustrated by Elipsoideichnus meyeri, which was known only from its holotype for many years. However, its helical architecture showing two opposite cells in each whorl is so complex that nobody has ever doubted its biogenic origin (Fig. 2(D)). The sticks and many trace fossils do not enjoy the same privilege; on the contrary, many trace fossils were confirmed as such when their recurrence was proved. The same criterion of recurrent morphologies has been recommended by Minter and Braddy (Reference Minter and Braddy2006) and Minter et al. (Reference Minter, Braddy and Davis2007) for creating new ichnotaxa. These authors proposed that the new ichnotaxa should be based on more than ten specimens occurring at a locality or one that occurs at localities from different regions, ages and settings. Many trace fossils from paleosols are extremely abundant in some localities (Fig. 2(E)) and they are also found in different other localities worldwide and from different ages, leaving no doubt of its condition.
Martian stick-like structures do not fulfil this requirement. Images from different localities of the Jura Member, some of them illustrated in Fig. 5, show that the shape of sticks is not repeated, and on the contrary, the morphological dispersion is very high.
Surface morphology
Many trace fossils show particular surface morphologies as a result of the work of the organisms to produce them. Such features are traditionally called bioglyphs (e.g. Ekdale and De Gibert, Reference Ekdale and De Gibert2010; Genise, Reference Genise2016) and in many cases are as important, or even more relevant, than the general morphology to identify a structure as a trace fossil and its producer as well as how it was produced. Complex and diagnostic morphologies may be present either in the external or internal surfaces of walls. An example of how the presence of distinct surface morphology is useful to identify a trace fossil and its producer is represented by the trace fossil Pallisphaera puertai (Genise et al., Reference Genise, Bellosi, Sarzetti, Krause, Dinghi, Sánchez, Umazano, Puerta, Cantil and Jicha2020). Its shape is a simple spheroid, as many concretions of the early Cretaceous Castillo Formation of Argentina, where this trace fossil occurs (Fig. 2(F)). However, P. puertai shows a distinct surface morphology composed of rounded to rhomboid pellets that indicate that it represents a chamber having a distinct wall and by comparison with extant traces that it was constructed for aestivation or moulting purposes, probably by beetles or millipedes (Fig. 2(F)).
The Martian stick-like structures show no bioglyphs but a rough or granular surface texture, which may reflect that these structures are crystals undergoing weathering, or the growth of a finely crystalline secondary phase, or the incorporation of substrate grains (Bennett et al., Reference Bennett, Rivera-Hernández, Tinker, Horgan, Fey, Edwards, Edgar, Kronyak, Edgett, Fraeman, Kah, Henderson, Stein, Dehouck and Williams2021). The absence of bioglyphs when combined with the other features analysed herein supports the inorganic origin of these structures.
Context
The context is also important when making a decision. Brasier and Wacey (Reference Brasier and Wacey2012) proposed a protocol for demonstrating ancient or extra-terrestrial life by separating biologic from inorganic structures. Such protocol includes analyses on cell morphospace, biological and metabolic behaviour, and context. The latter is shared by the methodology presented herein.
There are some ichnological examples of this use of context. After long controversies (e.g. Bromley et al., Reference Bromley, Buatois, Genise, Labandeira, Mángano, Melchor, Schlirf and Uchman2007) it has been proposed that the body fossil record of the putative producers of trace fossils should be taken into account to add reliability to the attribution of trace fossils to extant taxa (Genise, Reference Genise2016). In other words, a supposed fossil bee cell if found in Paleozoic/Triassic rocks, when bees were very far from been present, should be discarded as such. The certainty of attributions increases with high taxonomic ranks, congruence with the body fossil record, and morphological complexity of the trace fossil (Genise, Reference Genise2016). Another case may occur when a complex trace fossil certainly produced by a taxon restricted to a continent is said to be discovered in a different one where that taxon is not recorded and not expectable according to its phylogeny. Although not completely impossible, the claims of trace fossils out of the expected context should be revised exhaustively and accompanied by extraordinary proofs.
These are examples of how context may be applied to make a final decision on the origin of structures and to avoid unsustainable conclusions due to the lack of perspective. On Mars, the claim for the discovery of life should be based on a very sound and extraordinary evidence and could be invoked only after discarding all abiotic hypotheses (Brasier and Wacey, Reference Brasier and Wacey2012; Grotzinger et al., Reference Grotzinger, Sumner, Kah, Stack, Gupta, Edgar, Rubin, Lewis, Schieber, Mangold, Milliken, Conrad, DesMarais, Farmer, Siebach, Calef III, Hurowitz, McLennan, Ming, Vaniman, Crisp, Vasavada, Edgett, Malin, Blake, Gellert, Mahaffy, Wiens, Maurice, Grant, Wilson, Anderson, Beegle, Arvidson, Hallet, Sletten, Rice, Bell III, Griffes, Ehlmann, Anderson, Bristow, Dietrich, Dromart, Eigenbrode, Fraeman, Hardgrove, Herkenhoff, Jandura, Kocurek, Lee, Leshin, Leveille, Limonadi, Maki, McCloskey, Meyer, Minitti, Newsom, Oehler, Okon, Palucis, Parker, Rowland, Schmidt, Squyres, Steele, Stolper, Summons, Treiman, Williams and Yingst2014). The scarcity of free oxygen in the Martian atmosphere, as well as the availability of surface water 1–2 billion years before the origin of eukaryotes on Earth currently suggest that potential life on Mars may involve anaerobic microbial models (McMahon et al., Reference McMahon, Bosak, Grotzinger, Milliken, Summons, Daye, Newman, Fraeman, Williford and Briggs2018). The possibility of finding trace fossils on Mars is real but low if we consider these current models for Martian environments and the record of trace fossils on Earth. The great majority of trace fossils on Earth are produced by metazoans or plants. In rough numbers, and leaving aside vertebrate trace fossils, only 2% of the rest of the trace fossils of animals are produced by microorganisms such as bacteria and fungi (Knaust, Reference Knaust, Knaust and Bromley2012; Wisshak et al., Reference Wisshak, Knaust and Bertling2019). These organisms produce microborings less than 100 μm in diameter (Glaub et al., Reference Glaub, Golubic, Gektidis, Radtke, Vogel and Miller2007). Deep sea anaerobic environments on Earth lack evidence of bioturbation, they are almost devoid of metazoan life. There is a single case of bioturbation in an almost anoxic environment (0.02–0.03 ml O2 per litre H2O), whereas dysaerobic ones (1–0.1 ml O2 per litre H2O) show mostly a shallow microbioturbation (Buatois and Mángano, Reference Buatois and Mángano2011). In sum, on Earth traces produced by microorganisms not only are very few and from oxygenated environments, but also they are very small to be found without a very specific search. As a single exception to these small sizes, El Albani et al. (Reference Albani A, Mángano, Buatois, Bengtson, Riboulleau, Bekker, Konhauser, Lyons, Rollion-Bard, Bankole, Baghekema, Meunier, Trentesaux, Mazurier, Aubineau, Laforest, Fontaine, Recourt, Fru, Macchiarelli, Reynaud, Gauthier-Lafaye and Canfield2018) described larger trace fossils probably produced by syncytial microorganisms. The present knowledge suggests that one of the possibilities of finding true trace fossils on Mars, by the definition of trace fossil by Bertling et al. (Reference Bertling, Braddy, Bromley, Demathieu, Genise, Mikuláš, Nielsen, Nielsen, Rindsberg, Schlirf and Uchman2006), would be related to the possible past existence of colonies of homospecific anaerobic microorganisms producing common structures with a recurrent morphology.
Considering this scenario, the finding of Martian trace fossils is possible but also exceptional, and due to this exceptional condition and its significance as a proof of Martian life, any potential record should be proved unequivocally not only following a protocol like that presented herein and others for biogenicity, but also by extraordinary proofs.
Conclusions
Isolated criteria used for recognizing trace fossils include analysis of morphological regularity, morphological completeness, morphological dispersion, morphological recurrence, morphological complexity, surface morphology and context. Synthesis of criteria as a single protocol (i.e. a series of complementary analyses) useful for testing the ichnogenicity of trace-like structures is presented herein for the first time. The so-called ‘stick-like structures’ from Mars, taken here as a study case, do not fulfil any of the requirements posed by this protocol to be postulated as trace fossils. Such protocol, particularly based on morphology and independent of biogenicity tests, may be useful in the future with other potential cases of Martian trace-like structures before carrying on more complex analyses or before cataloguing them as of astrobiological interest.
Acknowledgements
The author thanks Nadia Garrido for her assistance with the language and Ana Vadnjal for her assistance with the processing of Martian images. Two anonymous reviewers improved the manuscript. This contribution was supported by grant PICT 17-0779 from the FONCYT of the Agencia Nacional de Promoción Científica y Tecnológica from Argentina.
Conflict of interest
None.