Introduction
As postulated by many scientists (e.g. Schidlowski Reference Schidlowski2001; Javaux et al. Reference Javaux, Knoll and Walter2003; Westall Reference Westall and Tokano2005), the search for life in the Cosmos depends on our understanding of modern and ancient life forms as well as past and present factors that affect the distribution of life and its interaction with the planet. Of necessity, therefore, the success of astrobiology will depend greatly upon the capacity of paleontology to identify connections among simple forms of past life on Earth as possible clues for recognizing life elsewhere. The Precambrian (4.56–0.542 Ga) paleobiology is especially important in this respect, because during this time life originated and evolved by way of a surprisingly small number of benchmark innovations that provided the basis for the present biosphere. Knoll & Bambach (Reference Knoll and Bambach2000) suggested that life has followed six megatrajectories, each the result of a major biological breakthrough leading to a significant increase in the volume and complexity of ecospace over time. Of these steps, four occurred during the Precambrian: (i) the emergence of protolife certainly prior to 3.0–3.3 Ga (Brasier et al. Reference Brasier, McLoughlin, Green and Wacey2006) or even 3.85 Ga (Allwood et al. Reference Allwood, Walter, Kamber, Marshall and Burch2006; Mojzsis et al. Reference Mojzsis, Arrhenius, Mckeegan, Harrison, Nutman and Friend1996, and also Nutman Reference Nutman2007), within the first billion years of Earth history; (ii) the evolution and success of the prokaryotic cell as the archetype of life on Earth, also prior to or around 3.5 Ga (Brasier et al. Reference Brasier, McLoughlin, Green and Wacey2006; Schopf Reference Schopf2006; Wacey et al. Reference Wacey, Kilburn, Saunders, Cliff and Brasier2011); (iii) the development of the eukaryotic cell by a complex series of endosymbiotic and other events, underway at least by 1.8 Ga (Porter Reference Porter2004; Rasmussen et al. Reference Rasmussen, Fletcher, Brocks and Kilburn2008); or possibly even by 2.6 Ga (Waldbauer et al. Reference Waldbauer, Sherman, Summer and Summons2009); and (iv) the appearance of multicellular organisms in the seas, at least by 1.2 Ga (microscopic bangiomorph algae; Butterfield Reference Butterfield2000) and possibly as early as 2 Ga (See Han & Runnegar Reference Han and Runnegar1992; Bengtson et al. Reference Bengtson, Rasmussen and Krapez2007; Albani et al. Reference Albani2010). Each of these major evolutionary innovations allowed life to diversify morphologically, metabolically and ecologically, limited only by a new set of constraints on size and complexity inherent to the innovation. In this way, each megatrajectory was added to previous ones, resulting in ever-increasing ecospace. Determining the chronology of these events from the record of Precambrian fossils not only elucidates the paths of terrestrial evolution but also furnishes potential temporal–ecological bases for searching for extraterrestrial life, both living and fossil.
Given that around two million square kilometres (25%) of Brazil is occupied by Precambrian rocks varying in age between 3.6 and 0.54 Ga (Schobbenhaus & Brito Neves Reference Schobbenhaus, Brito-Neves, Bizzi, Schobbenhaus, Vidotti and Alves2003), data on fossils from these terrains (Figs 1 and 2) may provide valuable insights into biological and planetary evolution, applicable to the search for life in the Cosmos. Below, we review several key aspects of the Brazilian Precambrian fossil record that we judge of greatest potential interest to astrobiologists.

Fig. 1. Map of Brazil showing the location of the Precambrian stratigraphic units mentioned in this paper. Conselheiro da Mata Group based on a bore hole. Based on Mapa Geológico do Brasil, CPRM (2003).
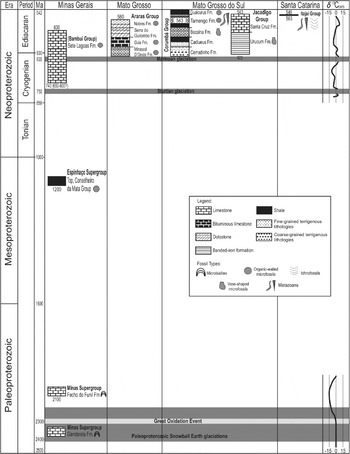
Fig. 2. Stratigraphic chart summarizing data on the fossiliferous stratigraphic units discussed in this paper. Age constraints indicated by numbers associated with schematic sections. The non-Snowball Gaskiers glaciation at 583 Ma is not indicated to avoid cluttering the image. Carbon isotope data based on Mills et al. (2011) for the Neoproterozoic and Bekker et al. (2001) for the Paleoproterozoic. Paleoproterozoic glaciations after Bekker et al. (2001). See text for details.
Nature of the Brazilian Precambrian fossil record
Although studies in Precambrian paleobiology in Brazil have essentially been descriptive, mostly dealing with new occurrences of fossils, morphological description and taxonomic identification, recent years have witnessed important initial advances in the application of these fossils to paleoenvironmental, biostratigraphical, paleoecological and evolutionary questions. In fact, with the exception of controversial phosphatized embryo-like microfossils, as in the Doushantuo Formation, China (Xiao et al. Reference Xiao, Yuan and Knoll2000; Chen et al. Reference Chen2009; but see also Butterfield Reference Butterfield2011; Huldtgren et al. Reference Huldtgren, Cunninghan, Yin, Stampanoni, Marone, Donoghue and Bengtson2011 for other interpretations) and rangeomorph fossils (Brasier & Antcliffe Reference Brasier and Antcliffe2009), all categories of Precambrian fossils known elsewhere have also been recognized in the Brazilian fossil record, from the lower Paleoproterozoic (2.4 Ga) practically to the limit with the Phanerozoic at 0.54 Ga. These fossils include microbialites, silicified microfossils, palynomorphs (acritarchs), vase-shaped microfossils (VSMs), biomarkers, macroalgae, metazoans and ichnofossils (Fig. 2).
As elsewhere, the commonest and most widespread categories of Precambrian fossils in Brazil are microbialites, especially stromatolites, but also including oncolites and rare thrombolites. Although microbialites of Early Paleoproterozoic age comprise the oldest known fossils in Brazil, they are much commoner in late Mesoproterozoic and Neoproterozoic limestones, dolostones, phosphate and chert, in which they may represent the full range of settings within the photic zone, from relatively deep water (below storm wave base) to the supratidal. The Paleoproterozoic stratiform and columnar stromatolites, and possible oncolites of the Minas Supergroup, in Minas Gerais (Fig. 3), will be discussed below because of their great age (2.4 and 2.1 Ga) close to the advent of oxygenic atmosphere and the oldest widespread glacial events on Earth.

Fig. 3. Paleoproterozoic Brazilian microbialites from the Minas Supergroup, Quadrilátero Ferrífero, Minas Gerais. (A) Stratiform to domical stromatolites of the Gandarela Formation (2.4 Ga). Scale, 1 cm. (B–D) Stromatolites of the Fecho do Funil Formation (2.1 Ga), Cumbi quarry, near Ouro Preto, MG, Brazil. Images: E.A.M. Sanchez. (B) Transverse to slightly oblique sections of deformed columnar stromatolites. Low-grade metamorphism has destroyed most of the internal lamination. Scale, 5 cm. (C) Longitudinal sections of columnar stromatolites. Note parallel branching (centre–right). Scale, 5 cm. (D) Deformed, laterally continuous domical stromatolites. Scale, 5 cm.
The second commonest category of Precambrian fossils in Brazil are microfossils (Fig. 4), presently known from 12 Mesoproterozoic to Neoproterozoic stratigraphic units in six tectonic domains. Most studies till now have dealt with silicified benthic, prokaryote-dominated, mat-forming microbiotas associated with stratiform, domical and columnar stromatolites. Fewer studies have focused on planktonic prokaryotes and eukaryotes (microalgae and acritarchs) in siliciclastic sequences, which have greater potential in paleoenvironmental and stratigraphic studies, including biostratigraphy. The importance of these microfossils and of vase-shaped microfossils is dealt with in greater detail below.
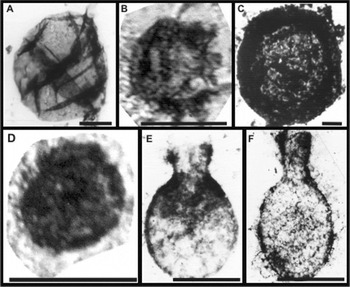
Fig. 4. Organic-walled microfossils. (A) Leiosphaeridia sp. from drill core sample, Conselheiro da Mata Group, Minas Gerais. Scale, 10 µm. Image from Simonetti, 1994. (B) Trachyhystrichosphaera aimica, Sete Lagoas Formation, Bambuí Group, Minas Gerais. Scale, 200 µm. (C) Leiosphaeridia crassa, Guia Formation, Araras Group, Mato Grosso. Scale, 25 µm. (D) Unidentified acritarch, Nobres Formation, Araras Group, Mato Grosso. Scale, 25 µm. (B–D) Images from Hidalgo (2007). (E–F) Vase-shaped microfossils, Urucum Formation, Jacadigo Group, Mato Grosso do Sul. Images from Zaine (1991). Scale, 50 µm in both figures.
Perhaps the group of Brazilian Precambrian fossils of greatest paleobiological importance are the metazoan fossils Corumbella and Cloudina (Fig. 5(b)–(f)), which attest to the advent of skeletogenesis in latest Neoproterozoic metazoans. These fossils occur in the uppermost Ediacaran Tamengo Formation, Corumbá Group, Mato Grosso do Sul, and are associated with vendotaenids in both this formation and the overlying Guaicurus formation (Zaine Reference Zaine1991; Gaucher et al. Reference Gaucher, Boggiani, Sprechman, Sial and Fairchild2003). The significance of the fossils of the Corumbá Group is discussed extensively further on.

Fig. 5. Ediacaran macrofossils. (A) Discoidal impression (Itajaí Group, Santa Catarina) described as Cyclomedusa by Zucatti da Rosa (2005). Scale, 15 mm. Image from Zucatti da Rosa (2005). (B–F) Metazoan fossils, Tamengo Formation, Corumbá Group, Corumbá, Mato Grosso do Sul. (B–C) Corumbella werneri. (B) Internal cast, holotype (DGM-5601-I). Scale, 5 mm. (C) Corumbella werneri. Internal cast. Scale, 1 mm. (B–C) M.L.A.F. Pacheco. (D–F) Cloudina lucianoi. (D) Surface view of specimens, showing typical aspect of the wall. Scale 2.5 cm. (E) Longitudinal sections of C. lucianoi shells in petrographic thin section. Note nested truncated conical structures in central specimen. Scale, 1 cm. (F) Transverse section of C. lucianoi shells in petrographic thin section. Scale, 1 cm. Images (D–F) from Meira (2011).
Ichnofossils have been identified in siliciclastic units of the Ediacaran to Lower Cambrian Itajaí Basin, state of Santa Catarina, Camaquã and Santa Bárbara basins, in the state of Rio Grande do Sul, and Camarinha Formation, in the state of Paraná. In the Itajaí Basin, Netto & Zucatti da Rosa (Reference Netto and Zucatti Da Rosa1997) originally identified the ichnogenera Bifungites, Gordia and Oldhamia in a turbidite setting, and Zucatti da Rosa (Reference Zucatti Da Rosa2005) later added the ichnogenus Helminthoidichnites. The ichnofauna of the Camaquã and Santa Bárbara basins includes nine ichnogenera preserved in two different assemblages, the older in marine and deltaic sediments and the younger in deltaic to eolian lithologies (Netto et al. Reference Netto, Paim and Da Rosa1992). In the Camarinha formation, the ichnogenera, Gordia, Planolites and Skolithos have been reported from turbidites (Ciguel et al. Reference Ciguel, Góis and Aenolaza1992). The presence of these trace fossils suggests the interesting possibility that the Precambrian–Cambrian transition may be present in these successions (e.g. Brasier et al. Reference Brasier, Cowie and Taylor1994).
Biomarkers were identified in Brazilian Proterozoic rocks in the Vazante Group, Minas Gerais, by Olcott et al. (Reference Olcott, Sessions, Corsetti, Kaufman and Oliveira2005) and interpreted within the context of Snowball Earth glaciation (Hoffman et al. Reference Hoffman, Kaufman, Halverson and Schrag1998; Hoffman & Schrag Reference Hoffman and Schrag2002). Thinly laminated, organic-rich black shales overlying diamictite, rhythmic marls and carbonates in the Poço Verde Formation contain indigenous organic matter, which included 2-a-methylhopanes derived from cyanobacteria, alkylated 2,3,6-trimethylbenzenes derived from green sulfur bacteria, 3-b-methylhopanes derived from aerobic methanotrophs, gammacerane derived from protists, and steranes non-methylated at carbon 4 indicative of aerobic eukaryotes. If the Snowball Earth setting for this formation is correct, then these results would indicate that life was able to persist even under the harsh glacial conditions proposed in this hypothesis. Within the same snowball scenario, organic matter concentrated in bituminous limestone and shale overlying a Marinoan cap dolostone in the Araras Group in Mirassol d'Oeste, Mato Grosso, has yielded hydrocarbons that were deposited in a post-glacial, deep anoxic platform to slope setting (Nogueira et al. Reference Nogueira, Riccomini, Sial, Moura, Trindade and Fairchild2007). The nature and composition of these hydrocarbons, however, are still under investigation, and the results are anxiously awaited for comparison with the biogeochemical data of the apparently synglacial Poço Verde Formation.
For geological and paleobiological studies and of greatest relevance for astrobiology, the following four categories of Brazilian Precambrian fossils are of special interest: the ancient microbialites of the Minas Supergroup in Minas Gerais; vase-shaped protistan microfossils of the Jacadigo Group in Mato Grosso do Sul; the organic-walled microfossils of the Bambuí and Araras groups in Minas Gerais and Mato Grosso, respectively; and the early metazoans and associated fossils of the Corumbá Group in Mato Grosso do Sul (Figs 1 and 2).
Selected Brazilian Precambrian fossils of special interest
Paleoproterozoic microbialites of the Minas Supergroup
Although known especially for its huge deposits of banded iron formation (BIF), the Paleoproterozoic Minas Supergroup in the Quadrilátero Ferrífero of eastern Minas Gerais also includes microbialitic carbonates in the Gandarela and Fecho do Funil formations (Fig. 2), which comprise the oldest reliable record of Precambrian fossils in Brazil, dated at ca. 2.4 and ca. 2.1 Ga, respectively (Pb/Pb, Babinski et al. Reference Babinski, Chemale and Van Schmus1995). These fossils crop out in only four localities and have undergone low- to medium-grade metamorphism.
The older microbialites, in the Gandarela Formation, include laterally linked domical stromatolites with alternating dark and light grey laminae (Fig. 3(a)) and columnar stromatolites, in addition to stratiform mats and micritic oncoids (Souza & Müller Reference Souza and Müller1984; Bertolino & Pires Reference Bertolino and Pires1995). Bekker et al. (Reference Bekker, Sial, Karhu, Ferreira, Noce, Kaufman, Romano and Pimentel2003) interpreted them as having formed in high-energy intertidal to subtidal settings. However, only the stratiform mats have been adequately described and illustrated.
Although considerably deformed and recrystallized, the younger microbialites of the Fecho do Funil Formation, on the other hand, are much better known, because they were mined from a single quarry near Ouro Preto (Minas Gerais) that furnished flagstone for buildings throughout Brazil, including shopping malls (Sallun-Filho & Fairchild Reference Sallun-Filho and Fairchild2005), for more than 60 years. Within the now inactive quarry, large bioherms and biostromes of reddish to nearly white, densely packed branched columnar stromatolites (Fig. 3(b) and (c)) as well as subordinate laterally continuous domes (Fig. 3(d)) and oncolites are preserved in dolomitic lenses among highly folded phyllites (Dardenne & Campos Neto Reference Dardenne and Campos Neto1975). Lamination in these stromatolites has been nearly obliterated by metamorphism, but the tuberous to slender, parallel to divergently branching columnar form of these structures comprises convincing evidence that they are indeed stromatolites. The depositional setting of the Fecho do Funil Formation was interpreted as subtidal by Bekker et al. (Reference Bekker, Sial, Karhu, Ferreira, Noce, Kaufman, Romano and Pimentel2003).
In 2003, Bekker et al. studied the geochemistry and isotopic content of Minas Supergroup carbonates with the dual purpose of establishing their correlation with other units and establishing their significance with respect to key events, including glaciation, of the first-half of the Paleoproterozoic (Fig. 2). Values of δ13C and δ18O in the carbonates of the Gandarela Formation varied, in general, between −1.6 and +0.4‰ and −13.5 to −6‰, respectively, but between −1 and −1.3‰ for the δ13C signal in carbonate levels with microbialites. Total organic carbon showed values between 0.03 and 0.16 mg C g−1 and an isotopic record of δ13C between +18.6 and +23.8‰. By comparison with similar results from other Paleoproterozoic units older than 2.32 Ga. The data for the carbonates of the Gandarela Formation were considered representative of the composition of Paleoproterozoic seawater, slightly altered by diagenesis and metamorphism.
Geochemical analysis of the Fecho do Funil Formation revealed values of +5.6 to +7.4‰ for δ13C and −12.3 to −9.1‰ for δ18O in the carbonates. Total organic carbon varied from 0.04 to 0.18 mg C g−1 sample and δ13C for organic matter ranged from −24.8 to −14.4‰. The carbon isotopic results presented higher values than expected for Paleoproterozoic units of similar age, but the authors concluded that the values were, in fact, representative of seawater at that time.
What is missing in the Brazilian record of this period is evidence of glacial evidence of a Paleoproterozoic Snowball Earth scenario (Kirschvink et al. Reference Kirschvink, Gaidos, Bertani, Beukes, Gutzmer, Maepa and Steinberger2000; Bekker et al. Reference Bekker, Sial, Karhu, Ferreira, Noce, Kaufman, Romano and Pimentel2003) as well as any evidence in the Gandarela Formation microbialites, for the methane atmosphere prior to the first glacial event, as proposed by Pavlov et al. (Reference Pavlov, Kasting, Brown, Rages and Freedman2000) and Kasting et al. (Reference Kasting, Pavlov and Siefert2001). However, the importance of the carbonates of the Gandarela and Fecho do Funil formations is that they register seawater conditions at two different moments of the Paleoproterozoic, first, when the putative supercontinent of that time was undergoing fragmentation which provoked changes in the dynamics of weathering, carbonate sedimentation and geochemical recycling of carbon, and second, when all these events, coupled with the rise of aerobic photosynthesis, resulted in an oxygenated atmosphere and a new biosphere (Kirschvink et al. Reference Kirschvink, Gaidos, Bertani, Beukes, Gutzmer, Maepa and Steinberger2000; Bekker et al. Reference Bekker, Kaufman, Karhu, Beukes, Swart, Coetzee and Eriksson2001, Reference Bekker, Sial, Karhu, Ferreira, Noce, Kaufman, Romano and Pimentel2003; Bekker & Eriksson Reference Bekker and Eriksson2003; Knoll Reference Knoll2003).
Organic-walled microfossils
Organic-walled microfossils are the most abundant direct evidence of life in the Precambrian. Differences in their size, ornamentation and complexity have been employed in attempts to distinguish eukaryotic from prokaryotic microfossils and thereby identify the most ancient forms in the Precambrian record of microfossils. However, at the present time, steranes in rocks 2.4–2.6 Ga old of the Transvaal Supergroup (South Africa) are the oldest candidates for the earliest eukaryotic biomarkers in the geological record (Waldbauer et al. Reference Waldbauer, Sherman, Summer and Summons2009), but it is wise to remember that similar, equally old biomarkers from the Pilbara Craton of Australia (Brocks et al. Reference Brocks, Logan, Buick and Summons1999) were later shown to be younger contaminants (Rasmussen et al. Reference Rasmussen, Fletcher, Brocks and Kilburn2008). At present, then, the oldest reliable direct evidence of eukaryotes are compressed, circular, elliptical and lanceolate organic-walled microfossils (acritarchs), from Paleoproterozoic rocks about 1.8 Ga old in the Changcheng Group, China, whose relatively large size and complexity exclude prokaryotic affinities (Hofmann & Chen Reference Hofmann and Chen1981; Zhang Reference Zhang1986; Yan Reference Yan1991; Huntley et al. Reference Huntley, Xiao and Kowalewski2006; Lamb et al. Reference Lamb, Awramik, Chapman and Zhu2009; Peng et al. Reference Peng, Bao and Yuan2009).
Over the time span of the Proterozoic, and especially in the Neoproterozoic, diversification and extinction among the eukaryotes (see Javaux et al. Reference Javaux, Knoll and Walter2003; Huntley et al. Reference Huntley, Xiao and Kowalewski2006; Knoll et al. Reference Knoll, Javaux, Hewitt and Cohen2006) introduced sufficient diversity among unicellular micro-organisms as to permit the use of fossil microalgae and acritarchs in biostratigraphic correlation schemes in Australia (Zang Reference Zang1995; Grey Reference Grey2005; Willman & Moczydlowska Reference Willman and Moczydlowska2008), Africa (Gaucher & Germs Reference Gaucher and Germs2006; Couëffé & Vecoli Reference Couëffé and Vecoli2011), Europe (Moczydlowska Reference Moczydlowska2008a), China (Zhou et al. Reference Zhou, Xie, McFadden, Xiao and Yuan2007; McFadden et al. Reference McFadden, Xiao, Zhou and Kowalewski2009) and South America (Gaucher et al. Reference Gaucher, Boggiani, Sprechman, Sial and Fairchild2003).
In Brazil, however, only Simonetti & Fairchild (Reference Simonetti and Fairchild2000), Gaucher et al. (Reference Gaucher, Boggiani, Sprechman, Sial and Fairchild2003) and Hidalgo (Reference Hidalgo2007) have attempted to use Brazilian Precambrian microfossils for biostratigraphy. In the first case, Simonetti & Fairchild (Reference Simonetti and Fairchild2000) interpreted the low morphological diversity and small size (<200 μm) of the assemblage of organic-walled microfossils, dominated by Leiosphaeridia spp. (Fig. 3(a)) recovered from drill cores of siliciclastic rocks from the upper Conselheiro da Mata Group, Espinhaço Supergroup, Minas Gerais (Fig. 4(a)), as being typical of late Mesoproterozoic assemblages, a finding consistent with the ca. 1.2 Ga radiometric age for the group. These microfossils are still the oldest reported from Brazil.
Hidalgo (Reference Hidalgo2007) analysed microfossils from the much younger Araras (<635 Ma) and Bambuí (<740 Ma) groups, in Mato Grosso and Minas Gerais, respectively, in an attempt to establish a biostratigraphic framework for the Neoproterozoic of Brazil and to identify possible effects of Snowball Earth glaciations upon the Brazilian fossil record. Despite the generally poor preservation of the microfossils, she identified nearly 20 taxa of Neoproterozoic acritarchs (Fig. 4(b)–(d)), including such biostratigraphically important forms as Cymatiosphaeroides, Trachyhystrichosphaera (Fig. 4(b)), Leiosphaeridia minutissima, Leiosphaeridia crassa (Fig. 4(c)) and Tanarium. Furthermore, she distinguished three different associations of acritarchs similar to those in biostratigraphic biozones proposed for the Neoproterozoic by Grey (Reference Grey2005) and Sergeev (Reference Sergeev2006), specifically, an older association in the Sete Lagoas Formation of the Bambuí Group and two younger ones in the Mirassol D´Oeste and Guia formations, and the overlying Nobres Formation (Fig. 4(d)), respectively, of the Araras Group. The results are consistent with the biostratigraphic proposal of Grey (Reference Grey2005) for the Neoproterozoic, based on morphological changes representative of evolutionary innovations.
The most ambitious correlation proposal for the Brazilian Neoproterozoic was made by Gaucher et al. (Reference Gaucher, Boggiani, Sprechman, Sial and Fairchild2003), who correlated the post-Marinoan Corumbá Group of Brazil with the Arroyo del Soldado Group of Uruguay on the combined basis of organic-walled microfossils, metazoans, stable isotopes and lithology. Three species of organic-walled microfossils, including cyanobacteria and acritarchs, occur in common in both units, but only two were considered biostratigraphically significant: Leiosphaeridia tenuissima and Soldadophycus bossii.
The studies by Gaucher et al. (Reference Gaucher, Boggiani, Sprechman, Sial and Fairchild2003) and Hidalgo (Reference Hidalgo2007) have thus revealed the potential value of Brazilian Neoproterozoic microfossils for biostratigraphic correlation, both regionally and globally. With respect to important evolutionary issues, acritarchs from the Araras Group have provided tentatively significant data. Hidalgo (Reference Hidalgo2007) found unornamented acritarchs at the bottom of the succession and, near the top, spiny forms, suggestive of a rapid change in composition (taxonomic replacement) after the Marinoan glaciation, possibly related to the appearance of metazoans (Peterson & Butterfield Reference Peterson and Butterfield2005; Willman & Moczydlowska Reference Willman and Moczydlowska2008; Cohen et al. Reference Cohen, Knoll and Kodner2009).
Vase-shaped microfossils
The Jacadigo Group near Corumbá (MS) is well known for its commercially exploitable, Neoproterozoic Rapitan-like iron (and manganese) formation, which is much younger (<623 Ma) than the Archean to Paleoproterozoic-banded iron formations that make up the world's major iron ores. However, near the base of this group, in the Urucum Formation, carbonate clasts within a massive diamictite deposited in a continental setting (Freitas et al. Reference Freitas, Warren, Boggiani, De Almeida and Piacentini2011) contain abundant vase-shaped microfossils, first identified by Fairchild et al. (Reference Fairchild, Barbour and Haralyi1978) and later likened to Melanocyrillium sp. (Zaine Reference Zaine1991; Zaine et al. Reference Zaine, Simonetti and Fairchild1989) (Fig. 4(e) and (f)). These fossils consist of thin-walled, subspherical to ellipsoidal bodies, up to 108 μm in width and up to 143 μm in length, with a single, simple opening at one pole or at the end of a short to long neck-like extension, giving them their vase-shaped form. Walls are black, unornamented and carbonaceous. The thin wall, long neck and absence of a pylome differentiate these microfossils from the melanocyrillids of the Chuar Group (USA), with which they were compared by Zaine (Reference Zaine1991).
Porter & Knoll (Reference Porter and Knoll2000) and Porter et al. (Reference Porter, Meisterfeld and Knoll2003) have demonstrated that Neoproterozoic VSMs are the oldest direct fossil evidence for heterotrophic protists. Since the original description in the Chuar Group (Bloeser et al. Reference Bloeser, Schopf, Horodyski and Breed1977), a variety of VSMs have been described, including agglutinated forms (Bosak et al. Reference Bosak, Lahr, Pruss, Msdonald, Dalton and Matys2011) from Namibia and Mongolia that are similar in size but morphologically distinct from, and somewhat older than, the organic-walled Brazilian VSMs. In fact, the Urucum VSMs are seemingly different from practically all other described Proterozoic VSMs. Possible VSMs similar to thecamoebans present in phosphorites at the top of the stratigraphically younger Bocaina Formation of the Corumbá Group (Fontaneta Reference Fontaneta2012) considerably broaden the paleontological perspectives for this region and research on early protistan heterotrophs. All of these fossils are distinctly different from the supposedly agglutinated fossil foraminiferan test Titanotheca coimbrae, made up exclusively of rutile crystals, registered in these same phosphorites by Gaucher et al. (Reference Gaucher, Boggiani, Sprechman, Sial and Fairchild2003).
Metazoans
Two regions stand out in Brazil for their fossil evidence of complex megascopic organisms of Ediacaran age: the Itajaí Group in Santa Catarina and the Corumbá Group in Mato Grosso do Sul.
Zircon crystals from volcanic tuffs have provided U/Pb (SHRIMP) ages of ca. 559–584 Ma for the Itajaí Group (Drukas & Basei Reference Drukas and Basei2009), and Guadagnin et al. (2010) reported U/Pb (LA-MC-ICP-MS) ages of 549 ± 4 Ma to 563 ± 3 Ma as the limits for the Itajaí Basin. The upper portion of the Itajaí Group, deposited in relatively calm waters below storm wave base in a prodeltaic marine setting has yielded enigmatic fossils interpreted as part of the benthic epifauna. These fossils were attributed to the sponge-like genus Choia and to possible Chancelloriida (Silva & Dias Reference Silva and Dias1981; Da Rosa et al. Reference Da Rosa, Paim, Chemale, Zucatti Da Rosa and Girardi1997; Leipnitz et al. Reference Leipnitz, Paim, Da Rosa, Zucatti Da Rosa and Nowatzki1997; Paim et al. Reference Paim, Leipnitz, Netto, Da Rosa and Zucatti Da Rosa1997), taxa previously considered to be exclusively Cambrian in age (Conway Morris Reference Conway Morris1992). Suspect medusoids, possibly Cyclomedusa (Fig. 5(a)) and Charniodiscus and rounded impressions or moulds of Aspidella, as well as faint, horseshoe-shaped impressions attributed to Parvancorina sp. (arguably related to the arthropods) have also been described (Zucatti da Rosa Reference Zucatti Da Rosa2005), along with the ichnofossils Bifungites, Gordia, Oldhamia and Helminthoidichnites (Netto & Zucatti da Rosa Reference Netto and Zucatti Da Rosa1997; Zucatti da Rosa Reference Zucatti Da Rosa2005).
The Corumbá Basin in the southern part of the Paraguay Belt is made up of the Cadiueus and Cerradinho formations, at its base, reflecting deposition in an initial continental rift basin, and the Bocaina, Tamengo and Guaicurus formations, deposited in a stable marginal basin (Almeida Reference Almeida, Almeida and Hasuy1984; Boggiani Reference Boggiani1998; Alvarenga et al. Reference Alvarenga, Moura, Gorayeb, Abreu, Cordani, Milani, Thomaz Filho and Campos2000). Of these, the Tamengo Formation (543 ± 3 Ma U-Pb−1; Babinski et al. Reference Babinski, Trindade, Alvarenga, Boggiani, Liu, Santos and Brito Neves2006, Reference Babinski, Boggiani, Fanning, Simon and Sial2008) is the most noteworthy in terms of its fossil content. Until recently, it was the only formation in the world containing abundant fossils of the early skeletal metazoans Corumbella werneri and Cloudina lucianoi (Zaine & Fairchild, Reference Zaine and Fairchild1987; Zaine Reference Zaine1991; Boggiani Reference Boggiani1998; Nogueira et al. Reference Nogueira1998; Babcock et al. Reference Babcock, Grunow, Sadowski and Leslie2005) (Fig. 5(b)–(f)), which are coeval with late members of the well-known Ediacaran soft-bodied biota (Hahn et al. Reference Hahn, Hahn, Leonardos, Pflug and Walde1982; Walde et al. Reference Walde, Leonardos, Hahn, Hahn and Pflug1982; Hahn & Pflug Reference Hahn and Pflug1985; Zaine Reference Zaine1991; Babcock et al. Reference Babcock, Grunow, Sadowski and Leslie2005; Simon Reference Simon2007; Pacheco et al. Reference Pacheco, Leme and Fairchild2010a, Reference Pacheco, Leme and Fairchildb).
Corumbella werneri (Fig. 5(b) and (c)) was discovered in marls and shales of the Tamengo Formation in Ladário, just outside Corumbá, (Hahn et al. Reference Hahn, Hahn, Leonardos, Pflug and Walde1982). Later, two specimens of Corumbella sp. were described in sandstone from the similarly aged lower Wood Canyon Formation, in the Great Basin, USA (Hagadorn & Waggoner Reference Hagadorn and Waggoner2000), and very recently, parautochthonous fragments of Corumbella were found together with in situ specimens of Cloudina in calcareous grainstones and mudstones of the Itapucumi Group, in Paraguay, 360 km south of Corumbá (Warren et al. Reference Warren, Fairchild, Gaucher, Boggiani, Poiré, Anelli and Inchausti2011).
C. werneri was originally reconstructed as a bipartite scyphozoan made up of a uniseriate primary polyp and a biseriate polypar, and placed within its own new subclass (Corumbellata) by Hahn et al. (Reference Hahn, Hahn, Leonardos, Pflug and Walde1982). Zaine (Reference Zaine1991) suggested rather that it may have been a vendobiont, an extinct group of megascopic organisms of uncertain biological affinity (Seilacher Reference Seilacher1989; Buss & Seilacher Reference Buss and Seilacher1994), possibly more closely related to the Protista than to the Metazoa (Seilacher et al. Reference Seilacher, Grazhdankin and Legouta2003; Seilacher Reference Seilacher, Vickers-Rich and Komarower2007). Recent investigations, however, have revealed polarization and symmetry in C. werneri that permit its reconstruction as a quadrate tube made up of ring-like segments, similar to some modern coronate scyphozoans and possibly to the extinct conulariids as well, thereby assuring its place within the kingdom Metazoa (Babcock et al. Reference Babcock, Grunow, Sadowski and Leslie2005; Pacheco et al. Reference Pacheco, Leme and Fairchild2010a, Reference Pacheco, Leme and Fairchildb, Reference Pacheco, Leme and Fairchild2011a, b, Pacheco Reference Pacheco2011).
The genus Cloudina was created by Germs (Reference Germs1972) for small, straight to sinuous, tubular calcareous shelly fossils (up to 6.5 mm in diameter and 35 mm long), open at the apex and closed at the base, found in limestones of the late Neoproterozoic Nama Group, Namibia (Germs Reference Germs1972; Grant Reference Grant1990). Characteristic of Cloudina are its short, partly overlapping segments having the shape of open truncated cones (Germs Reference Germs1972; Zaine & Fairchild Reference Zaine and Fairchild1987; Grant Reference Grant1990; Chen et al. Reference Chen, Bengtson, Zhou, Hua and Yue2008; Meira Reference Meira2011) (Fig. 5(d) and (e)). Its walls are uniformly thick yet extremely thin (3–50 μm) (Fig. 5(f)), but early cementation between walls of successive segments appears to have strengthened the original shell (Grant Reference Grant1990; Hua et al. Reference Hua, Pratt and Zhang2003, Reference Hua, Chen, Yuan, Zhang and Xiao2005). Cloudina was immediately recognized as latest Neoproterozoic in age, thereby giving it the status of oldest known shelly metazoan fossil. Grant (Reference Grant1990) demonstrated that because of its widespread occurrence in rocks of identical age, it deserved to be considered an index fossil for the uppermost Ediacaran.
Cloudina is known from practically all the quarries and major outcrops of the Tamengo Formation in the Corumbá área (Zaine & Fairchild Reference Zaine and Fairchild1987; Meira Reference Meira2011) and has been reported from Uruguay and Argentina (Gaucher et al. Reference Gaucher, Boggiani, Sprechman, Sial and Fairchild2003, Reference Gaucher, Frimmel and Germs2005) and, most recently, from Paraguay (Boggiani & Gaucher Reference Boggiani and Gaucher2004; Warren et al. Reference Warren, Fairchild, Gaucher, Boggiani, Poiré, Anelli and Inchausti2011). Outside South America, it occurs in Namibia (Germs Reference Germs1972), Oman (Conway Morris et al. Reference Conway Morris, Mattes and Menge1990), China (Conway Morris et al. Reference Conway Morris, Mattes and Menge1990; Bengtson & Zhao Reference Bengtson and Zhao1992), Canada (Hofmann & Mountjoy Reference Hofmann and Mountjoy2001), Nevada (Hagadorn & Waggoner Reference Hagadorn and Waggoner2000), Spain (Palacios Reference Palacios1989) and Russia (Kontorovich et al. Reference Kontorovich2008).
Perspectives
The importance of the Brazilian fossils discussed above resides in their association with paradigmatic transitions and benchmark innovations in the evolution of the Proterozoic environment and biosphere.
For instance, the microbialites in the Paleoproterozoic Gandarela and Fecho do Funil formations of the Minas Supergroup are not just the oldest Precambrian fossils in Brazil: they were deposited, respectively, at about the same time as and not long after the ‘Great Oxygenation Event’ (GOE) (Holland Reference Holland2002; Ohmoto Reference Ohmoto2003) around 2.3 Ga (Fig. 2), when free oxygen produced by aerobic photosynthesis finally began to accumulate in the atmosphere (Kirschvink et al. Reference Kirschvink, Gaidos, Bertani, Beukes, Gutzmer, Maepa and Steinberger2000; Bekker et al. Reference Bekker, Kaufman, Karhu, Beukes, Swart, Coetzee and Eriksson2001, Reference Bekker, Sial, Karhu, Ferreira, Noce, Kaufman, Romano and Pimentel2003; Knoll Reference Knoll2003; Catling & Claire Reference Catling and Claire2005). This event, coupled with continental breakup of the supercontinent Kernorland (Heaman Reference Heaman1997; Bekker & Eriksson Reference Bekker and Eriksson2003), led to profound changes in weathering, burial of organic carbon and geochemical cycling in general, resulting in major changes in climate (Paleoproterozoic Snowball Earth) and dominant metabolism in the biosphere (Kirschvink et al. Reference Kirschvink, Gaidos, Bertani, Beukes, Gutzmer, Maepa and Steinberger2000; Bekker et al. Reference Bekker, Kaufman, Karhu, Beukes, Swart, Coetzee and Eriksson2001, Reference Bekker, Sial, Karhu, Ferreira, Noce, Kaufman, Romano and Pimentel2003; Anbar & Knoll Reference Anbar and Knoll2002; Knoll Reference Knoll2003; Barley et al. Reference Barley, Bekker and Krapez2005; Catling & Claire Reference Catling and Claire2005; Kopp et al. Reference Kopp, Kirschvink, Hilburn and Nash2005). Within this context, the microbialites of the older Gandarela Formation and the younger Fecho do Funil Formation bracket this period (Fig. 2), thereby providing an opportunity to explore possible changes in the relationships among paleoenvironmental and paleobiological factors within the atmosphere, hydrosphere and biosphere in response to the oxidation of atmosphere at this crucial time. The paper by Bekker et al. (Reference Bekker, Sial, Karhu, Ferreira, Noce, Kaufman, Romano and Pimentel2003) exploits this question, but hardly exhausts the subject.
A second field in which Brazilian fossils will certainly be useful is Neoproterozoic biostratigraphy. In the absence of megascopic index-fossils (animals, vendobionts and macroalgae) prior to about 580 Ma (Narbonne Reference Narbonne2005; Yuan et al. Reference Yuan, Chen, Xiao, Zhou and Hua2011), recent Neoproterozoic biostratigraphic proposals have been based primarily on microfossils – acritarchs, microalgae and occasional other microfossils (such as VSMs, for example), whose abundance, complexity and diversity evolved in response to biological and environmental factors, once again related to continental breakup (Rodinia) and Snowball Earth scenarios and their consequences (Hoffman et al. Reference Hoffman, Kaufman, Halverson and Schrag1998; Hoffman & Schrag Reference Hoffman and Schrag2002; Knoll Reference Knoll2003). Several authors (Moczydlowska Reference Moczydlowska2005, Reference Moczydlowska2008a, Reference Moczydlowskab; Huntley et al. Reference Huntley, Xiao and Kowalewski2006) allege that changes, especially in paleoclimate, were responsible for both an increase in diversity and extinction among eukaryotes. The palynological study by Hidalgo (Reference Hidalgo2007) in post-glacial Neoproterozoic successions in Brazil is consistent with this interpretation.
Recent geochronological and sedimentological analyses, however, suggest alternative interpretations for the age of a supposed cap carbonate at the base of the Bambuí Group (Sete Lagoas Formation), which Hidalgo studied, and for the glacial origin of diamictites in the Corumbá region. In the former case, Pimentel et al. (Reference Pimentel, Rodrigues, Giustina and Junges2009) suggested a maximum age for deposition between 650 and 600 Ma based on U/Pb (SHRIMP) ages obtained for detrital grains of zircon at the top the Sete Lagoas Formation. This significantly challenges the long-standing idea that deposition of the Bambuí Group began after 740 Ma, following the older (Sturtian) of the Neoproterozoic snowball glaciations (Vieira et al. Reference Vieira, Trindade, Nogueira and Ader2007), rather than the younger Neoproterozoic (Marinoan) glacial event at ca. 635 (Fig. 2). Nevertheless, based on the biostratigraphic considerations of Sergeev (Reference Sergeev2006), the acritarch assemblage identified from this formation by Hidalgo (Reference Hidalgo2007) favours the older, traditional view.
The vase-shaped microfossils in the Jacadigo Group comprise a third group of Precambrian fossils from Brazil that merit special attention, in this case because of their potential paleoecological/paleoenvironmental importance. This is because they occur in limestone clasts of apparently non-marine origin within a diamictite deposited prior to the Rapitan-like iron formation of the same group that has been associated with Neoproterozoic glacial conditions (see Gaucher et al. Reference Gaucher, Boggiani, Sprechman, Sial and Fairchild2003) (Fig. 2). In their recent detailed sedimentological study of the Jacadigo Group, however, Freitas et al. (Reference Freitas, Warren, Boggiani, De Almeida and Piacentini2011) found no convincing evidence of glacial conditions during any phase of deposition this group. Hence, these VSMs may offer a rare view of non-marine Ediacaran life outside the context of Snowball Earth. They are also important because they differ from other VSMs, as summarized by Porter (Reference Porter2011), and thus add to a growing body of evidence for considerable morphological variety among Neoproterozoic heterotrophic protists (Porter et al. Reference Porter, Meisterfeld and Knoll2003; Porter Reference Porter2004; Bosak et al. Reference Bosak, Lahr, Pruss, Msdonald, Dalton and Matys2011) related to changes in trophic complexity (Karlstrom et al. Reference Karlstrom2000).
The fourth important group of Precambrian fossils are the late Ediacaran Brazilian metazoans (and associated fossils), which represent a critical phase of one of the most profound events in the evolution of the biosphere – the emergence of animal life. Metazoans arose much earlier than the Early Cambrian explosion of life (Fedonkin Reference Fedonkin2003), probably before the first records of the soft-bodied Ediacara biota (Shen et al. Reference Shen, Zhang and Hoffman2008; Yuan et al. Reference Yuan, Chen, Xiao, Zhou and Hua2011), and even earlier than the extreme climatic changes and increase in oxygen levels of the latter half of the Neoproterozoic (Brain et al. Reference Brain, Prave, Hoffmann, Fallick, Botha, Herd, Sturrock, Young, Condon and Allison2012). Molecular clock evidence points to an origin for crown group demosponges and cnidarians at about 700 Ma (Conway Morris Reference Conway Morris2000; Erwin et al. Reference Erwin, Laflamme, Tweedt, Sperling, Pisani and Peterson2011). In face of the sparse, problematical record of early metazoan fossils worldwide, e.g., controversial 600 Ma-old phosphatized ‘metazoan embryos’ from the Doushantuo Formation, China (Xiao & Knoll Reference Xiao and Knoll2000; Chen et al. Reference Chen, Bottjer, Oliveri, Dornbos, Gao, Ruffins, Chi, Li and Davidson2004; Huldtgren et al. Reference Huldtgren, Cunninghan, Yin, Stampanoni, Marone, Donoghue and Bengtson2011), 635 Ma-old sponge biomarkers in the Huqf Supergroup in Oman (Love et al. Reference Love2009), and 760–550 Ma-old Ma sponge-like fossils from the Otavi and Nama Groups in Namibia (Brain et al. Reference Brain, Prave, Hoffmann, Fallick, Botha, Herd, Sturrock, Young, Condon and Allison2012), the Brazilian (and South American) Precambrian fossil record represents a largely untapped, potentially major source of significant new finds regarding early metazoan evolution.
Carbon and sulfur isotopic data suggest that Neoproterozoic oceans prior to a major oxygenation event near the end of the Ediacaran period (Canfield Reference Canfield1998; Holland Reference Holland2009; Shields-Zhou & Och Reference Shields-Zhou and Och2011), like those before the ‘Great Oxygenation Event’ at 2.32 Ga (Holland Reference Holland2002; Ohmoto Reference Ohmoto2003) (Fig. 2), may have been anoxic and probably iron- and sulfur-rich (Halverson et al. Reference Halverson, Hurtgen, Porter, Collins, Gaucher, Sial, Halverson and Frimmel2009), hardly suitable for the diversification of macroscopic metazoans (Catling et al. Reference Catling, Glein, Zahnle and McKay2005). Both of these global oxygenation events were accompanied by widespread deposition of banded iron formations, when anoxic deep waters with large amounts of ferrous iron in solution came into contact with oxygenated surface waters (Pierrehumbert et al. Reference Pierrehumbert, Abbot, Voigt and Knoll2011). The disappearance of these unusual deposits from the rock record, if not due to oxygenation of the oceans, may have occurred when the deep oceans became sulfidic, rather than oxic (Canfield Reference Canfield1998), producing deep seawater that may have become more reducing rather than more oxidizing, despite the rise in atmospheric oxygen. Yet it is possible, however, that even in the context of widespread Neoproterozoic oceanic anoxia, metabolic versatility in early stages of animal evolution may have been a key factor in the emergence and establishment of the group (Budd Reference Budd2008; Shields-Zhou & Och Reference Shields-Zhou and Och2011), while later oxygenation allowed significant increase in the size, complexity and mobility among the Metazoa (Catling et al. Reference Catling, Glein, Zahnle and McKay2005).
Diversity of eukaryotic plankton crashed during the breakup of Rodinia in the mid-Neoproterozoic (Nagy et al. Reference Nagy, Porter, Dehler and Shen2009). It is likely that in the Cryogenian glacial interval between ca. 720 and 635 Ma, the first animal lineages diverged from ancestral eukaryotic unicellular populations and/or communities (Peterson et al. Reference Peterson, Cotton, Gehling and Pisani2008), probably in close proximity with the anoxic, ferruginous, icy environments of the ocean (Canfield et al. Reference Canfield, Poulton, Knoll, Narbonne, Ross, Goldberg and Strauss2008). The oldest concrete evidence of the dawn of animal life is younger than this, represented in the fossil record by complex macroscopic multicellular organisms of the Mistaken Point (⩽575 Ma, Canada) and Lantian (>579 Ma, China) assemblages of the Ediacaran biota (Narbonne Reference Narbonne2005; Shen et al. Reference Shen, Zhang and Hoffman2008; Yuan et al. Reference Yuan, Chen, Xiao, Zhou and Hua2011). Thus, the macroscopic biosphere that continues to this day had its origin soon after the Marinoan glaciation (Xiao & Laflamme Reference Xiao and Laflamme2009; Yuan et al. Reference Yuan, Chen, Xiao, Zhou and Hua2011), and quite probably under anoxic conditions (Narbonne Reference Narbonne2011).
Within this new, macroscopic biosphere, a significant number of the classical ‘soft-bodied’ Ediacaran organisms may not have been metazoans at all, but rather members of the extinct Vendobionta (Seilacher Reference Seilacher1989; Buss & Seilacher Reference Buss and Seilacher1994), an extinct major group of organisms characterized by flexible bodies built up of hollow, tubular subunits in serial or fractal arrangements and having an organic cuticle (Buss & Seilacher Reference Buss and Seilacher1994; Seilacher Reference Seilacher, Vickers-Rich and Komarower2007). The varied frond-like and quilted benthic forms, typical of vendobionts, show no evidence of a mouth or gut (Fedonkin et al. Reference Fedonkin, Simonetta and Ivantsov2007) and were incapable of moving about. Some of them may have been osmotrophic, feeding off dissolved organic carbon in seawater (Laflamme et al. Reference Laflamme, Xiao and Kowalewski2009), while others may have digested the abundant microbial mats typical of the shallow sea bottom before the evolution of grazing and burrowing animals (Erwin & Tweedt Reference Erwin and Tweedt2011).
While the taxonomic status of the Vendobionta remains phylogenetically unresolved, even at the level of kingdom (Seilacher et al. Reference Seilacher, Grazhdankin and Legouta2003), the most recent and parsimonious explanation for the taxonomic composition of the other elements of the Ediacaran biota is that some of the fossils represent real metazoans – especially those with bilateral symmetry, such as Kimberella (Fedonkin & Waggoner Reference Fedonkin and Waggoner1997; Dzik Reference Dzik2003; Fedonkin et al. Reference Fedonkin, Simonetta and Ivantsov2007) and early skeletal taxa like Cloudina and Namacalathus (Grotzinger et al. Reference Grotzinger, Watters and Knoll2000). These early metazoan fossils are found together with soft-bodied Ediacaran fossils throughout the world but always in different, but often coeval facies (in carbonates and siliciclastic rocks, respectively) and never on the same bedding plane (Germs Reference Germs1972, Reference Germs1983; but see Warren et al. Reference Warren, Fairchild, Gaucher, Boggiani, Poiré, Anelli and Inchausti2011), clear evidence of a robust and varied late Ediacaran ecosystem.
At the same time that the vendobionts became extinct (Seilacher Reference Seilacher, Vickers-Rich and Komarower2007), metazoans diversified and began to dominate ecosystems near the Ediacaran–Cambrian boundary (Erwin & Tweedt Reference Erwin and Tweedt2011). This signal event may have been caused by great changes in environmental conditions related to supercontinent breakup (McKerrow et al. Reference McKerrow, Scotese and Brasier1992), variations in sea level (Hallam Reference Hallam1984), nutrient crises, fluctuations in atmospheric oxygen (Shields-Zhou & Och Reference Shields-Zhou and Och2011) and/or carbon dioxide levels (Brasier Reference Brasier1992), changes in ocean chemistry (Lowenstein et al. Reference Lowenstein, Timofeeff, Brennan, Hardie and Demicco2001), re-engineering of ecosystems, or, most likely, by a combination of these factors.
Within this context, the varied fossils in the Jacadigo and Corumbá groups comprise a practically unique assemblage within the Ediacaran period. Recent research (Meira Reference Meira2011; Pacheco Reference Pacheco2011; Pacheco et al. Reference Pacheco, Leme and Machado2011a; Warren et al. Reference Warren, Pacheco, Fairchild, Simões, Riccomini, Boggiani and Cáceresin press) and new studies will certainly broaden knowledge of the latter phases of eukaryotic evolution in the Ediacaran. The ichnofossils from the Itajai Basin (Zucatti da Rosa Reference Zucatti Da Rosa2005; Drukas & Basei Reference Drukas and Basei2009) will provide a further complementary view of this record. These studies, particularly those of the Jacadigo and Corumbá groups, will have global implications (Boggiani Reference Boggiani1998; Gaucher et al. Reference Gaucher, Boggiani, Sprechman, Sial and Fairchild2003), given the important occurrences of VSMs in the former group and, in the latter group, stromatolites, possible VSMs and phosphorite in the Bocaina Formation, and shelly fossils of Cloudina and organic carapaces of Corumbella in the Tamengo Formation (Fig. 2). The recent questioning (Freitas et al. Reference Freitas, Warren, Boggiani, De Almeida and Piacentini2011) of the glacial origin of the Rapitan-type iron formation in the Jacadigo Group and the diamictites of the Puga Formation, beneath the Corumbá Group near Corumbá (Urban et al. Reference Urban, Stribrny and Lippolt1992; Alvarenga et al. Reference Alvarenga, Boggiani, Babinski, Dardenne, Figueiredo, Santos, Dantas, Gaucher, Sial, Halverson and Frimmel2009; Boggiani et al. Reference Boggiani, Gaucher, Sial, Babinsky, Simon, Riccomini, Ferreira and Fairchild2010) will certainly force re-examination of basic aspects of the sedimentary dynamics and evolutionary implications of the Snowball Earth hypothesis.
Temporal synchroneity of these units with other successions worldwide, which also present similar fossils and unusual lithologies may be established via chemostratigraphical correlation using C and Sr isotopes. For example, as observed in other carbonates in the latter part of the Ediacaran (after the Gaskiers glaciation at 583 Ma), two negative δ13C excursions associated with the Shuram–Wonoka anomaly of Oman and Australia at ca. 551 (Burns & Matter Reference Burns and Matter1993; Pell et al. Reference Pell, McKirdy, Jansyn and Jenkins1993), just below the disappearance of typical early Ediacaran large spiny acritarchs on a global scale and the first occurrence of Cloudina shells and the disappearance of typical early Ediacaran large spiny acritarchs on a global scale, also appear to be recorded in the Tamengo Formation (Fig. 2). This global δ13C variation has been attributed to the oxidation of vast amounts of dissolved organic carbon with extremely negative values in deep oceanic waters (Rothman et al. Reference Rothman, Hayes and Summons2003; Shields-Zhou & Och Reference Shields-Zhou and Och2011). A ubiquitous feature of the upper Tamengo Formation, on the other hand, is a positive δ13Ccarb plateau around +3 to +5‰, associated with the occurrence of Cloudina and Corumbella (Boggiani et al. Reference Boggiani, Gaucher, Sial, Babinsky, Simon, Riccomini, Ferreira and Fairchild2010). These values have been interpreted as indicating high rates of production and burial of organic carbon (with low δ13Corg) and subsequent deposition of carbonate with high δ13C values, coupled with increased release of oxygen to the atmosphere (Shields-Zhou & Och Reference Shields-Zhou and Och2011).
The positive δ13C plateau is thus consistent with the idea that favourable conditions for the origin or expansion of macroscopic metazoans existed around the time of deposition of the carbonates of the upper Tamengo Formation. Hence, it may not be merely coincidental, in this respect, that a firm organic or weakly mineralized skeleton in Corumbella (Pacheco Reference Pacheco2011; Pacheco et al. Reference Pacheco, Leme and Machado2011a; Warren et al. Reference Warren, Pacheco, Fairchild, Simões, Riccomini, Boggiani and Cáceres2012), and that the earliest evidence of possibly predatory borings in thin-walled, biomineralized shells of Cloudina (in China) (Bengtson & Zhao Reference Bengtson and Zhao1992; Bengtson Reference Bengtson and Bengtson1994; Hua et al. Reference Hua, Pratt and Zhang2003, Reference Hua, Chen, Yuan, Zhang and Xiao2005) first appear at this time, although other causes (protistans, worms and mineral grains) have been suggested (Bengtson & Zhao Reference Bengtson and Zhao1992). Therefore, of the four groups of Brazilian Precambrian fossils highlighted in this paper, it is the Corumbá Group that perhaps offers the best (and possibly a unique) opportunity to investigate a major issue in biospheric evolution, i.e., initial ecological relationships among early skeletal metazoans and the possible consequences of the introduction of competition and predation among macroscopic organisms upon trophic net complexity (Clapham & Narbonne Reference Clapham and Narbonne2002; Droser et al. Reference Droser, Gehling and Jensen2006; Wood Reference Wood2011; Warren et al. Reference Warren, Pacheco, Fairchild, Simões, Riccomini, Boggiani and Cáceresin press).
Final considerations
Despite the vast area occupied by Precambrian rocks in Brazil, the corresponding fossil record has been relatively little exploited. Traditionally, most papers dealing with this subject have focused on description and taxonomy rather than on paleobiological inference or biostratigraphical interpretation. Meanwhile, worldwide, in just the past few years, a series of new techniques has been applied to the study of very ancient fossils, especially microfossils and chemical fossils – Raman spectroscopy and imagery, confocal laser scanning microscopy (Schopf & Kudryavtsev Reference Schopf and Kudryavtsev2009), synchrontron X-ray microtomography (Chen et al. Reference Chen2009), nanoSIMS (Oehler et al. Reference Oehler, Robert, Walter, Sugitani, Allwood, Meibom, Mostefaoui, Selo, Thomen and Gibson2009), micro-FTIR spectroscopy (Igisu et al. Reference Igisu, Ueno, Shimojima, Nakashima, Awramik, Ohta and Maruyama2009), chemostratigraphy and others – that has elevated Precambrian paleobiological research to a new level of sophistication. Much of the technological development of many of these techniques have been directly stimulated by the concrete prospect of actually searching for fossil evidence of an ancient alien biosphere on Mars within a few decades. The needs of astrobiology in this endeavour require not only knowledge of Earth's most ancient life forms, but a well-founded understanding of paleontological practices and paleobiological concepts.
We have mentioned in this paper four different kinds of Brazilian Precambrian fossils of potential value for understanding biological evolution and the transformation of Earth's surface and atmosphere over time as well as for application to practical problems of geology, as in biostratigraphy. With respect to the Paleoproterozoic microbialites of the Quadrilátero Ferrífero, for instance, Bekker et al. (Reference Bekker, Sial, Karhu, Ferreira, Noce, Kaufman, Romano and Pimentel2003) conducted an initial geochemical investigation designed to test hypotheses regarding the atmosphere, oceanic chemistry and glacial events around the time of the Great Oxygenation Event at 2.3 Ga. Yet much remains to be done.
Few workers have investigated the organic-walled planktonic microfossils of the Brazilian Proterozoic, so that only the surface of their paleobiological and biostratigraphical significance has been touched. A first step forward in this regard would be the independent corroboration by other workers of the results that do exist and initiation of systematic formation-by-formation, basin-by-basin research programmes in Precambrian palynology.
Understanding of the paleobiology and global significance of the Ediacaran fossils in the Jacadigo (VSMs and microfossils) and Corumbá groups (microbialites, microfossils, macroalgae and metazoans) in the Corumbá region is already benefiting from recent detailed stratigraphic analyses (Oliveira Reference Oliveira2010; Freitas et al. Reference Freitas, Warren, Boggiani, De Almeida and Piacentini2011; Warren Reference Warren2011) and the application of such modern techniques as X-ray tomography (Pacheco and Leme in collaboration with Professor Cláudio Campi de Castro, Universidade de São Paulo), synchrontron X-ray microtomography (Pacheco and colleagues, in collaboration with the team of Professor Franz Pfeiffer, Technical University of Munich) and Raman spectroscopy (Sanchez & Fairchild Reference Sanchez and Fairchild2012, and other works in collaboration with Drs Douglas Galante, Fabio Rodrigues, Professor Dalva Faria, Universidade de São Paulo, and Professor Dr Airton Martin, UniVap, São José dos Campo, SP).
The application of these and other techniques to the Precambrian Brazilian fossils discussed here offers great promise for delving more deeply into the biological affinities and ecological aspects of Precambrian life (Fedonkin & Waggoner Reference Fedonkin and Waggoner1997; Seilacher Reference Seilacher1999; Narbonne Reference Narbonne2004), with implications for the astrobiological search for basic life forms outside the limits of the terrestrial biosphere.
Acknowledgements
The authors thank Dr Douglas Galante and Dr Fabio Rodrigues for the opportunity to present this paper based on the lecture given by TRF at SPASA 2011, and Msc Guilherme Raffaeli Romero for technical support. Research presented here has been supported by grants from the Brazilian funding agencies Conselho Nacional de Desenvolvimento Científico e Tecnológico (CNPq), Coordenação de Aperfeiçoamento de Pessoal de Nível Superior (CAPES) and Fundação de Amparo à Pesquisa do Estado de São Paulo (FAPESP).