Nosocomial pneumonia, a continuing challenge in hospitals, is associated with substantial morbidity, mortality, and cost.Reference Magill, Edwards and Bamberg1 The delivery of timely, appropriate antibiotic therapy represents the most critical and modifiable factor that drives these outcomes.Reference Freire, Melnyk and Kim2–Reference Wunderink, Rello, Cammarata, Croos-Dabrera and Kollef9 The ever-shifting landscape of antimicrobial resistance, however, complicates decision making regarding empiric treatment in severe infections. In nosocomial pneumonia, which includes hospital-acquired (HAP) and ventilator-associated (VAP) pneumonias, clinicians face a wide variety of potential pathogens and unpredictable resistance patterns, pitting the clinical mandate not to undertreat a potentially fatal infection against the public health reality that unnecessarily broad antibiotic administration promotes more resistance.
One tool available to clinicians to help them balance these competing risks is evidence-based clinical practice guidelines that provide recommendations in the context of current microbiological trends. However, clinical practice guideline updates lag behind the evolution of these trends. Additionally, because they generally represent an international or a national consensus, clinical practice guidelines are unable to consider regional differences in the distributions of specific microorganisms. For all these reasons, it is vital for clinicians to have access to more contemporary epidemiology to facilitate risk stratification to optimize prescription of antimicrobial regimens.
Unfortunately, much of the timelier microbiology data in HAP/VAP relies on single-center or small multicenter evaluations. This necessarily puts into question the results’ generalizability. Therefore, to balance the need to provide both a broader and more contemporary picture of the current microbiology of nosocomial pneumonia, we developed an algorithm to identify bacterial nosocomial pneumonia (ie, HABP/VABP) in an administrative database enriched with microbiology results.Reference Zilberberg, Nathanson, Sulham, Fan and Shorr10 We applied this algorithm to explore the microbiology of HABP/VABP, the empiric treatment of these infections, as well as the impact of inappropriate empiric therapy on hospital outcomes. Because emerging literature suggests that ventilated HABP (vHABP) is distinct from nonventilated (nvHABP), we further stratified our analyses by this factor.Reference Esperatti, Ferrer and Theessen11,Reference Vallecoccia, Dominedo, Cutuli, Martin-Loeches, Torres and De Pascale12
Methods
Study design and patient population
We conducted a multicenter, retrospective, cohort study of hospitalized patients with culture-positive nvHABP, vHABP, or VABP to explore their microbiology, empiric treatment patterns, and impact of receiving inappropriate empiric treatment on hospital outcomes. The case identification approach relied on a slight modification of a previously published algorithm.Reference Zilberberg, Nathanson, Sulham, Fan and Shorr10 The details of the study methods can be found in Zilberberg et al,Reference Zilberberg, Nathanson, Puzniak and Shorr14 an analysis conducted in the same cohort.Reference Zilberberg, Nathanson, Puzniak and Shorr14 Briefly, patients were included (1) if they were adults (aged >18 years) whose pneumonia diagnosis appeared in a secondary position, (2) it they had an index respiratory and/or blood culture obtained on hospital day 3 or later for HABP, or (3) if they were on mechanical ventilation day 3 or later for VABP, and (4) if they were treated with an antibiotic on the day of the index culture and for the next >3 consecutive days. We excluded patients who fit the definition for either a complicated urinary tract infection or a complicated intra-abdominal infection to reduce misclassification.Reference Zilberberg, Nathanson, Sulham, Fan and Shorr15,Reference Zilberberg, Ditch, Lawrence, Olesky and Shorr16 Because this study used existing fully deidentified data, it was exempt from ethics review under US 45 CFR 46.101(b)4.13
Data source
We obtained data from the Premier Research database, an electronic laboratory, pharmacy and billing data repository, for 2012 through the third quarter of 2019. The database has been described in detail previously.Reference Zilberberg, Nathanson, Sulham, Fan and Shorr10,Reference Zilberberg, Nathanson, Puzniak and Shorr14–Reference Haessler, Lindenauer and Zilberberg19 During the study period, ∼200 US institutions submitted microbiology data. The details regarding this cohort are provided in the study by Zilberberg et al.Reference Zilberberg, Nathanson, Puzniak and Shorr14
Pneumonia classification
Pneumonia was defined as HABP if, at the time of the index culture, the patient was not on mechanical ventilation and was defined as VABP if, at the time the index culture, the patient had been on mechanical ventilation for ≥3 days. HAPB was further subdivided into vHABP and nvHABP. Specifically, vHABP designation was given for patients who needed mechanical ventilation <5 days following the onset of index HABP episode and nvHABP if mechanical ventilation was not required.
Microbiology and empiric treatment
We examined both gram-positive and gram-negative pathogens that cause bacterial nosocomial pneumonia. Namely, the gram-negative organisms of interest included Pseudomonas aeruginosa, Acinetobacter baumannii, Stenotrophomonas maltophilia, Haemophilus spp, Moraxella catarrhalis, and Enterobacterales. The gram-positive pathogens of interest included Staphylococcus aureus (both methicillin-susceptible [MSSA] and MRSA), Streptococcus pneumoniae, and other Streptococcus spp.
Organisms were classified as S (susceptible), I (intermediate) or R (resistant), and I and R were grouped together as resistant. Carbapenem resistance was present when a gram-negative organism had an I or R in the susceptibility category to 1 of the 4 carbapenems: imipenem, meropenem, ertapenem, or doripenem. P. aeruginosa and A. baumannii were considered carbapenem resistant if they had an I or R in the susceptibility category to antipseudomonal carbapenems: imipenem, meropenem, or doripenem. All S. maltophilia isolates were assumed to be carbapenem resistant. The organism was carbapenem susceptible if it was susceptible to either a carbapenem or to a third-generation cephalosporin. Third-generation cephalosporin resistance (a phenotypic surrogate for extended-spectrum β-lactamase–containing organisms, ESBL) was defined as nonsusceptibility among Enterobaceriales to any of the agents in that class. Resistance was identified to the following noncarbapenem antipseudomonal β-lactams: cefepime, ceftazidime, ceftazidime/avibactam, ceftolozane/tazobactam, and piperacillin/tazobactam.
Antimicrobial coverage was considered appropriate if a drug administered within 2 days of the index culture being obtained covered the recovered organism. All other treatment was defined as inappropriate empiric treatment. All microbiology results were based on the local testing done by participating hospitals using Clinical and Laboratory Standards Institute (CLSI) break points to determine susceptibilities.
Outcome variables
The primary outcome of interest was hospital mortality. Secondary outcomes included postinfection-onset duration of mechanical ventilation; postinfection intensive care unit (ICU) length of stay (LOS); hospital LOS (total and postinfection onset); hospital costs; and 30-day readmission rates among survivors.
Statistical analyses
We report descriptive statistics to compare nvHABP, vHABP, and VABP groups across their infection characteristics, microbiology, and empirical treatment regimens. Continuous variables are reported as means with standard deviations and as medians with interquartile ranges (IQR). Differences between mean values were tested using a 1-way ANOVA test and between medians using the Kruskal-Wallis test. Categorical data are summarized as proportions, and the χReference Freire, Melnyk and Kim2 test used to examine intergroup differences unless a cell count was <5, wherein the Fisher exact test was used.
To examine the impact of inappropriate empiric treatment compared to appropriate treatment on hospital outcomes, we employed multivariable logistic regression for modeling the binary outcomes of mortality and 30-day readmission endpoints and generalized linear regression models for the continuous outcomes. All models derived robust standard errors based on clustering at the hospital level. The model included the following variables: demographic characteristics; comorbidity burden; acute illness severity as measured by the need for ICU admission, dialysis, and vasopressor use; whether the admission was due to medical or surgical diagnosis; diagnosis of acute trauma or neurologic insult; duration of hospital stay from admission to index date, a variety of common treatments provided to patients in the ICU (eg, nutritional support, inotropes), and hospital structural characteristics (census region, size, teaching status, urbanicity).Reference Zilberberg, Nathanson, Puzniak and Shorr14 Interaction effects between empiric treatment status (ie, appropriate or inappropriate) and pneumonia type were explored in each model and as subanalyses, and separate regression models were rerun for each outcome in each of the 3 patient groups. P < .05 was considered statistically significant. Notably, however, because of the large sample size, statistical significance may not indicate clinical significance.
Results
Among 17,819 patients who met enrollment criteria, 26.5% had an nvHABP, 25.6% had a vHAPB, and 47.9% had a VABP. VABP accounted for the highest proportion of pneumonias in the northeastern United States (54.0%) and the lowest in the West census region (44.3%) (Table 1).
Table 1. Infection Characteristics
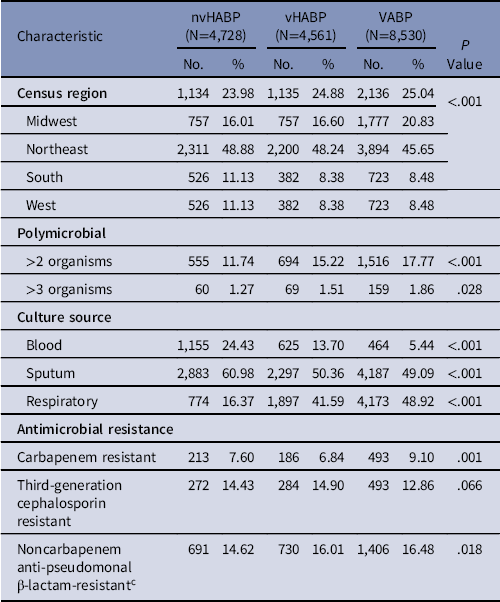
Note. nvHABP, nonventilated HABP; vHABP, ventilated HABP; VABP, ventilator-associated bacterial pneumonia.
a Denominator consists of patients with gram-negative pathogens only.
b Denominator consists of patients with Enterobacteriales only.
c Includes cefepime, ceftazidime, ceftazidime/avibactam, ceftolozane/tazobactam, and piperacillin/tazobactam.
Mean time to infection was shortest in the VABP and longest in the nvHABP groups (Table 1). Although most infections were monomicrobial, a substantial proportion harbored 2 or more offending organisms, with VABP having the highest prevalence (17.8%). Having 3 or more organisms was rare. More than three-quarters of all isolates came from a respiratory source, with the remainder from blood and a small minority from both (Table 1). Among 521 patients with both respiratory and blood isolates, 384 (73.7%) were concordant. Bacteremia was most common in the setting of nvHABP (24.4%) and least in VABP (5.4%), and 46.2% of all blood cultures grew S. aureus.
The single most common organism across all 3 pneumonia groups was S. aureus, occurring in nearly 40% of each pneumonia type (Fig. 1). MSSA was slightly more prevalent than MRSA in each group. MRSA was most common and MSSA was least common in VABP, and the reverse was true in nvHABP. P. aeruginosa, the next most prevalent pathogen, was present in 16.8% of VABP patients and 19.2% of vHABP patients, with 18.5% of the nvHABP group harboring P. aeruginosa. E. coli and K. pneumoniae accounted for 12%–13% of all infections, except for a lower prevalence of E. coli in VABP patients (8.7%) (Fig. 1). A. baumannii, though rare across all pneumonia types, was more than twice as likely in the setting of VABP (3.2%) as in nvHABP (1.5%) or vHABP (1.5%). Similarly, S. marcescens and E. aerogenes were both substantially more frequent in VABP than in either nvHABP or vHABP. Among the gram-negative organisms, the rates of carbapenem resistance ranged from 6.8% in vHABP to 9.1% in VABP. Across all pneumonia types, 4 organisms accounted for 80% of all carbapenem-resistant pathogens: S. maltophilia, A. baumannii, P. aeruginosa, and P. mirabilis. Third-generation cephalosporin resistance was more prevalent than carbapenem resistance, present among Enterobacteriales in 14.4% in nvHABP, 14.9% in vHABP, and 12.6% in VABP (Table 1).

Fig. 1. Distribution of pathogens. Note. SA, S. aureus; MRSA, methicillin-resistant SA; MSSA, methicillin-susceptible SA.
During the index admissions and prior to the onset of their pneumonia, >70% of each group had received antibiotics. Within the 90 days prior to the index admission date, the prevalence of antimicrobial treatment had ranged from 8.9% in VABP to 16.0% in nvHABP (Table 2). Vancomycin and antipseudomonal penicillins with β-lactamase inhibitor were the most common empiric regimens across all 3 pneumonia types. Inappropriate empiric treatment was administered in 8.5% of nvHABP, 5.6% of vHABP, and 7.2% of VABP patients.
Table 2. Antimicrobial Treatments and Outcomes
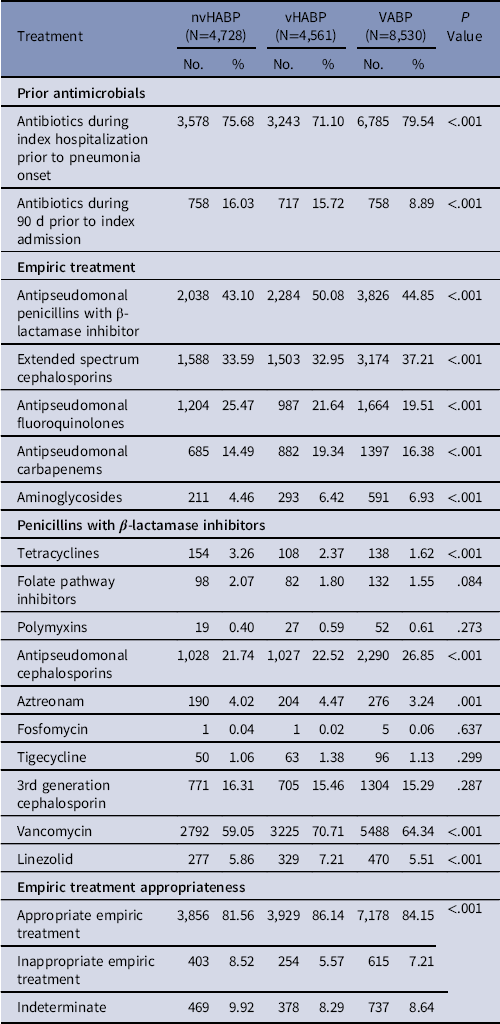
Note. nvHABP, nonventilated hospital-acquired bacterial pneumonia; vHABP, ventilated hospital-acquired bacterial pneumonia; VABP, ventilator-associated bacterial pneumonia.
Table 3 shows the impact of inappropriate empiric treatment on the adjusted outcomes. Although inappropriate empiric treatment was not associated with a significant increase in hospital mortality or the risk of a 30-day readmission among survivors, there were substantial increases in the LOS across the continuum of care, ranging from 1.1 (95% confidence interval [CI], 0.4–1.8) extra days in the ICU to 4.8 (95% CI, 2.5–7.1) additional days in the hospital. Inappropriate empiric treatment was also associated with the attributable cost of $12,142 (95% CI, $3,272–$21,013). Whether the pathogen was gram-positive or gram-negative did not alter this relationship significantly (data not shown). The association of inappropriate empiric treatment with several components of resource use varied by pneumonia type (Table 4). Total hospital LOS (8.7 days; 95% CI 3.3, 14.1), postinfection onset LOS (4.9 days; 95% CI, 3.0–6.9), and costs ($13,147; 95% CI, $3,009–$23,284) were much greater in the nvHABP group than in the other 2 pneumonia categories. Among the remaining outcomes examined, the impact of inappropriate empiric treatment was of similar magnitude across the groups.
Table 3. Adjusted Contribution of Inappropriate Empiric Treatment to Outcomes

Note. OR, odds ratio; CI, confidence interval; LOS, length of stay; ICU, intensive care unit; MV, mechanical ventilation.
a Ventilated hospital-acquired bacterial pneumonia and ventilator-associated bacterial pneumonia only.
Table 4. Differential Impact of Inappropriate Empiric Treatment by Pneumonia Type

Note. nvHABP, nonventilated hospital-acquired bacterial pneumonia; vHABP, ventilated hospital-acquired bacterial pneumonia; VABP, ventilator-associated bacterial pneumonia; CI, confidence interval; LOS, length of stay.
a vHABP and VABP only.
Discussion
Across groups of patients with nvHABP, vHABP, and VABP, the general prevalence of various pathogens was mostly similar, with the exception of several organisms. Namely, E. coli was less prevalent, whereas S. marcescens, E. aerogenes, and A. baumannii were more common in VABP than in the other 2 pneumonia types. Similarly, MRSA, while common in all forms of nosocomial pneumonia, was less prevalent in VABP than in nvHABP. The case was reversed for MSSA. The prevalences of carbapenem resistance and third-generation cephalosporin resistance were quite high, and carbapenem resistance was most common in the setting of VABP in patients with positive cultures. We observed that inappropriate empiric treatment in was infrequent during this period. Although it does not appear to be significantly associated with increased hospital mortality and 30-day readmission, it independently adds substantially to postinfection-onset duration of mechanical ventilation, ICU and hospital LOS, and overall costs. Interestingly, the impact of inappropriate empiric treatment appears to vary by pneumonia type, being most resource intensive in patients with nvHABP, where it adds 4.9 days to the postinfection-onset LOS and >$13,000 to the total hospital bill.
To our knowledge, ours is the first study in approximately a decade to elucidate the microbiology in bacterial nosocomial pneumonia, particularly in the context of stratifying epidemiology as a function of the pneumonia types and the subtypes of HABP (ventilated vs nonventilated). Esperatti et alReference Esperatti, Ferrer and Theessen11 studied the similarities and differences between VABP and what they termed nonventilated ICU-acquired pneumonia (NV-ICUAP). In this single-center cohort from Spain, the investigators enrolled 315 patients who met their criteria for VABP and NV-ICUAP. Among the 151 (47.9%) patients who had an organism isolated, the pathogen distribution was largely similar to our findings. That is, P. aeruginosa, MRSA, and MSSA were most common organisms, and their relative prevalence across pneumonia types was similar to our findings. In their study, as in ours, MSSA was more often associated with VABP and MRSA was more often associated with NV-ICUAP. The prevalence of P. aeruginosa, though higher in VABP than in NV-ICUAP in the Spanish study and lower in ours, was not substantively different across the pneumonia types. Overall, the enteric gram-negative pathogen distribution we observed was similar to that reported by Esperatti et al.Reference Esperatti, Ferrer and Theessen11
Our data comport with other US-based estimates. For example, an analysis from the National Healthcare Safety Network (NHSN) in the years 2011–2012 revealed a similar distribution of common organisms in VAP.Reference Weiner, Webb and Limbago20 In this study, S. aureus was most common, followed by P. aeruginosa and K. pneumoniae, a pattern also reported by Quartin et alReference Quartin, Scerpella, Puttagunta and Kett21 in a multinational study with the data collected from 2004 to 2010.Reference Weiner, Webb and Limbago20,Reference Quartin, Scerpella, Puttagunta and Kett21 Moreover, the latter investigators reported a similar pathogen distribution to ours in HABP, although the prevalence of MRSA far exceeded that of MSSA.Reference Quartin, Scerpella, Puttagunta and Kett21 The high prevalence of these organisms along with their likelihoods of carrying carbapenem resistance and ESBL, respectively, suggest that broad empiric coverage continues to be a necessity in HABP and VABP.
One other US-based study delved into the contemporary microbiology of nosocomial pneumonia with the specific focus on carbapenem resistance.Reference Cai, Echols and Corvino22 Using the Premier database between the years 2010 and 2015, the investigators derived a cohort of patients with HAP or VAP and a positive respiratory culture for a gram-negative organism. Of the 6,483 respiratory cultures included in the analysis, 70% were in the setting of HAP and the remainder in VAP; the corresponding carbapenem resistance rates were 9.9% and 27.6%, respectively. Although they diverged from these rates somewhat, our estimates are likely to be more accurate for a number of reasons. First, by applying a case-finding algorithm that maximized specificity (eg, excluding other infection types), we were less likely to misclassify patients. Second, we did not limit the culture source to respiratory, but included positive blood cultures as well. This is an important distinction for 2 reasons. First, respiratory cultures tend to overrepresent colonization with resistant organisms when compared to blood cultures.Reference Haessler, Lindenauer and Zilberberg19 Second, because the vast majority of cultures in the study by Cai et alReference Cai, Echols and Corvino22 were from patients with HAP, they were unlikely to have originated from the lower airway, thus setting up an even greater bias for identifying colonization rather than infection. The overrepresentation of HAP by a factor of more than 2:1 in their study also suggests a high degree of misclassification. Specifically, in other studies the distribution is either more balanced or, as in ours, essentially reversed.Reference Esperatti, Ferrer and Theessen11,Reference Quartin, Scerpella, Puttagunta and Kett21
The prevalence of bacteremia in our study also parallels that observed by Quartin et al.Reference Quartin, Scerpella, Puttagunta and Kett21 More importantly, it is consistent with the literature contained within the recent clinical practice guidelines.Reference Kalil, Metersky and Klompas23 Notably, the proportion of all S. aureus isolates that were MRSA was lower in our study than in the previous analyses.Reference Weiner, Webb and Limbago20,Reference Quartin, Scerpella, Puttagunta and Kett21 Such an encouraging pattern mirrors that reported in the setting of hospital-onset bloodstream infections in the United States. Namely, evidence from both NHSN and CDC-funded Emerging Infections Program (EPI) suggests a decrease in MRSA over time.24–Reference Kourtis, Hatfield and Baggs26
Another potentially encouraging finding in the current study was the rate of inappropriate empiric treatment, which was lower than we had reported in a prior contemporary cohort of patients with HABP/VABP.Reference Zilberberg, Nathanson, Sulham, Fan and Shorr10 However, this small reduction may have been due to differences in the patients and organism mix. Nonetheless, compared to reports from 20 years ago when the issue and impact of inappropriate empiric treatment in nosocomial pneumonia initially received attention, it appears that rates of inappropriate empiric treatment have fallen substantially, which is encouraging. This reduction in the rate of inappropriate empiric treatment potentially explains our failure to detect its impact on mortality. The rate of inappropriate empiric treatment may have been sufficiently low enough to no longer alter mortality measurably but large enough to continue an adverse impact on morbidity and cost. It is also possible that we undercounted cases of inappropriate empiric treatment, given that we required patients have antimicrobial treatment on the day of the index culture as an inclusion criterion, thus leaving out those who failed to receive timely treatment.
Our study had several limitations. To reduce the risk for selection bias, we defined the enrollment criteria prospectively. To mitigate misclassification, we used a previously published, though not clinically validated, algorithm. We also excluded other potential sources of infection, and included microbiology specimens from specific sources, pharmacy data, and dates of cultures and treatments. If present, however, such misclassification would drive the differences between groups toward null. The data did not allow us to differentiate between infection and colonization. If a large proportion of what we identified as culture-positive nosocomial pneumonia simply represent colonization, then our analyses underestimate the potential adverse impact of inappropriate empiric treatment on nosocomial pneumonia outcomes. Despite adjusting for a large number of confounders, the possibility of residual confounding remains. For example, our analysis focuses only on the period of empiric treatment. To examine potential confounding from the totality of the hospitalization would require more detailed longitudinal data than available in the current data set. Although generalizability of this large, multicenter geographically representative database is of minimal concern, our results capture only hospital-based events among patients who were culture-positive for common pathogens, and lack such data points as postdischarge death. As such, these findings may not generalize to nosocomial pneumonia with negative cultures. Despite these limitations, ours is the largest and most contemporary multicenter cohort study to examine the microbiology, treatment, and outcomes of nosocomial pneumonia in the United States.
In summary, we have demonstrated that there are substantial differences between populations who suffer from nvHABP, vHABP, and VABP. Although their microbiology varies, the general prevalence of such resistant organisms as MRSA appears to be lower currently than in the past. Despite having marginal impact on hospital mortality and the risk of readmission within 30 days, inappropriate empiric treatment remains an important modifiable risk factor that significantly worsens utilization outcomes, and this effect is most pronounced among patients with nvHABP. On the other hand, the rate of inappropriate empiric treatment may have also decreased, potentially highlighting the efficacy of adoption of clinical practice guidelines. Given the moderate rates of carbapenem resistance and third-generation cephalosporin resistance, our observations suggest that, depending on their clinical risk profile, patients require empiric antibiotic regimens that cover a range of bacteria, including those with a high likelihood of extended-spectrum β-lactamase and carbapenem resistance. This needs to be accompanied by meticulous attention to prompt de-escalation where appropriate to prevent further proliferation of antimicrobial resistance. Alternatively, if rapid diagnostics are proven to be both accurate and reliable, adopting an approach that utilized a rapid test that excluded the presence of resistance would allow one to start with narrower options. In the end, clinical trials and cost-effectiveness analyses will be required to delineate the tradeoffs and outcomes with these possible approaches.
Acknowledgments
Financial support
This study was supported by a grant from Merck Sharp & Dohme, a subsidiary of Merck & Co, Kenilworth, New Jersey.
Conflicts of interest
M.D.Z. is employed by EviMed Research Group, and she has received research grant support from Merck Sharp & Dohme, a subsidiary of Merck & Co. B.H.N. is employed by OptiStatim and has received support from EviMed Research Group. L.A.P. is an employee of and a stockholder in Merck Sharp & Dohme, a subsidiary of Merck & Co. A.F.S. is a consultant to and has received research grant support from Merck Sharp & Dohme, a subsidiary of Merck & Co. M.D.Z. and A.F.S. have received grant support and/or have served as consultants to Lungpacer, Melinta, Tetraphase, Pfizer, Astellas, Shionogi, The Medicines Company, Spero, and Theravance.