1. Introduction
The Causses Basin in southern France is known for its abundant Early Jurassic vertebrate footprints (Demathieu et al. Reference Demathieu, Gand, Sciau and Freytet2002; Gand et al. Reference Gand, Demathieu and Montenat2007). More than 60 archosaur tracksites have been reported in the Hettangian–Sinemurian sediments in this area. The Hettangian Dolomitic Formation from the Causses Basin mainly contains theropod trackways (Ellenberger, Reference Ellenberger1988; Demathieu, Reference Demathieu1990; Demathieu & Sciau, Reference Demathieu and Sciau1992, Reference Demathieu and Sciau1999; Demathieu et al. Reference Demathieu, Gand, Sciau and Freytet2002; Sciau, Reference Sciau2003, Reference Sciau2019; Gand et al. Reference Gand, Demathieu and Montenat2007; Moreau et al. Reference Moreau, Baret, Trincal and André2012a, Reference Moreau, Trincal, Gand, Néraudeau, Bessière and Bourel2014) and rarely crocodylomorph trackways (Sciau, Reference Sciau1998; Demathieu et al. Reference Demathieu, Gand, Sciau and Freytet2002; Moreau et al. Reference Moreau, Fara, Néraudeau and Gand2019a). In all these Hettangian tracksites, ichnofossils are preserved in homogeneous dolomudstone beds or laminites showing abundant microbial mats (Demathieu et al. Reference Demathieu, Gand, Sciau and Freytet2002; Moreau et al. Reference Moreau, Trincal, André, Baret, Jacquet and Wienin2018, Reference Moreau, Fara, Néraudeau and Gand2019a). Such sedimentological facies, corresponding to low-energy depositional palaeoenvironments, are usually interpreted as tidal flats.
In the area of Mongisty in the Causses Basin (Aveyron, southern France), Demathieu et al. (Reference Demathieu, Gand, Sciau and Freytet2002) and Sciau (Reference Sciau2003) reported several small outcrops with rare Hettangian dinosaur footprints (Grallator variabilis Lapparent & Montenat, Reference Lapparent and Montenat1967 and Eubrontes giganteus Hitchcock, Reference Hitchcock1845). We excavated a new outcrop recently at Mongisty. This paper provides a detailed description of the newly discovered dinosaur tracks. The excavated surface consists of a high density of well-preserved and large tridactyl footprints. In contrast to other tracksites of the Dolomitic Formation, the tracks are preserved in a coarse-grained substrate that constitutes an uncommon track-bearing facies. Here, we characterize the tracks using morphometry and 3D imaging photogrammetry, in association with sedimentological and ichnotaphonomic analyses. The track assemblage is compared with other coeval tracks, and a depositional environment model is proposed.
2. Geographical and geological setting
The dinosaur footprints were discovered in the Dolomitic Formation on the western edge of the Causses Basin, south of the Massif Central (southern France; Fig. 1). The tracksite is located in Aveyron, 10 km west of Millau, near the hamlet of Jouvenet and the ruin of the Mongisty farm. In the area of Millau, the Dolomitic Formation is 70 to 200 m thick (Mennessier et al. Reference Mennessier, Collomb, Astruc, Roche, Bambier and Galharague1984, Reference Mennessier, Collomb, Astruc, Roche, Vautrelle and Galharague1986). It mainly consists of grey dolomite organized in centimetric to metric layers, as well as dolomitic laminites alternating with green and black argillites or marls. Locally, the base of the Dolomitic Formation is exposed as conglomerates, sandstones and sandy dolomites (Mennessier et al. Reference Mennessier, Collomb, Astruc, Roche, Bambier and Galharague1984). The top of the Dolomitic Formation bears plant-bearing beds yielding the conifers Brachyphyllum paparelii Saporta, 1884 and Pagiophyllum peregrinum (Lindley & Hutton) A. Schenk emend. Kendall, 1948, as well as the pteridosperm Thinnfeldia rhomboidalis Ettingsh, 1852 (Roquefort, Reference Roquefort1934; Mennessier et al. Reference Mennessier, Collomb, Astruc, Roche, Bambier and Galharague1984; Thévenard, Reference Thévenard1993; Moreau et al. Reference Moreau, Philippe and Thévenard2019b). The Dolomitic Formation has already yielded numerous archosaur tracksites in all the Causses Basin (Ellenberger, Reference Ellenberger1988; Demathieu, Reference Demathieu1990; Demathieu & Sciau, Reference Demathieu and Sciau1992, Reference Demathieu and Sciau1999; Sciau, Reference Sciau1998, Reference Sciau2003, Reference Sciau2019; Demathieu et al. Reference Demathieu, Gand, Sciau and Freytet2002; Gand et al. Reference Gand, Demathieu and Montenat2007; Moreau, Reference Moreau2011; Moreau et al. Reference Moreau, Baret, Trincal and André2012a, Reference Moreau, Trincal, Gand, Néraudeau, Bessière and Bourel2014, Reference Moreau, Trincal, André, Baret, Jacquet and Wienin2018, Reference Moreau, Fara, Néraudeau and Gand2019a). With the exception of vertebrate footprints and terrestrial plants, body fossils are uncommon in the Dolomitic Formation. Some bivalves (e.g. Cardinia Agassiz, 1841, Liostrea Douvillé, 1904, Ostrea Linnaeus, 1758) and external natural casts of unidentified gastropods have been mentioned (Brouder et al. Reference Brouder, Gèze, Macquar and Paloc1977; Briand et al. Reference Briand, Couturié, Geffroy and Gèze1979; Gèze et al. Reference Gèze, Pellet, Paloc, Bambier, Roux and Senaud1980), as well as rare ammonites found at the base of the formation (e.g. Psiloceras planorbis Sowerby, 1824; Brouder et al. Reference Brouder, Gèze, Macquar and Paloc1977) that give a Hettangian age to the Dolomitic Formation. Demathieu et al. (Reference Demathieu, Gand, Sciau and Freytet2002) and Sciau (Reference Sciau2003) located the Mongisty tracksite in ‘Unit IIIA’ of Arrondeau (Reference Arrondeau1982) which is considered late Hettangian in age.

Fig. 1. Simplified geological map of the Causses Basin (modified from Moreau et al. Reference Moreau, Trincal, André, Baret, Jacquet and Wienin2018) and location of the Mongisty tracksite indicated by the black star.
The tracksite at Mongisty was discovered in 1998. It was first mentioned briefly by Demathieu et al. (Reference Demathieu, Gand, Sciau and Freytet2002) in an inventory of the Hettangian–Sinemurian dinosaur tracksites from the Causses Basin. At Mongisty, tracks are dispersed in several small outcrops separated from each other by several metres. Demathieu et al. (Reference Demathieu, Gand, Sciau and Freytet2002) mentioned a total of six tridactyl footprints located on two outcrops called Mongisty A and Mongisty B. The main track-bearing surface (Mongisty A) was limited to very small dolomitic slabs located along a dirt road and measuring less than 2 m2. This surface yielded four in situ footprints referred to Eubrontes giganteus (Demathieu et al. Reference Demathieu, Gand, Sciau and Freytet2002; Sciau, Reference Sciau2003). Mongisty B yielded two traces referred to Grallator variabilis (Demathieu et al. Reference Demathieu, Gand, Sciau and Freytet2002; Sciau, Reference Sciau2003). In a recent paper, Sciau (Reference Sciau2019) described a third outcrop with two in situ tracks referred to Eubrontes giganteus (Mongisty C).
In 2018, a fourth outcrop yielding tridactyl tracks was discovered by one of us (J.S.). Here, we describe this new fossiliferous surface which is located 100 m south of the main surface (Mongisty A) described by Demathieu et al. (Reference Demathieu, Gand, Sciau and Freytet2002) and then by Sciau (Reference Sciau2003). We call this new outcrop ‘Mongisty D’ (Fig. 2). When the tracksite of Mongisty D was discovered in 2018, only two tracks were visible on a surface less than 1 m2. After an excavation conducted in 2018–19 and supervised by one of us (J.S.), an area up to 7 m wide and 11 m long was cleaned, representing a surface of 53 m2. Today, Mongisty D bears 118 in situ tridactyl footprints (Figs 2 and 3).

Fig. 2. Illustration of the Mongisty D tracksite (a–b) showing abundant tridactyl dinosaur footprints (c–d).

Fig. 3. Mongisty D tracksite showing abundant tridactyl dinosaur tracks preferentially oriented towards the north. (a) Photograph. (b) Interpretative sketch.
In addition to the four outcrops with tridactyl footprints (Mongisty A to D), Sciau (Reference Sciau2019) mentioned two other outcrops that contain tetradactyl pes impressions and pentadactyl manus impressions which he identified as the first prosauropod trackways from the Lower Jurassic of the Causses Basin. Two of us (J.-D.M and G.G.) have recently reinvestigated these structures that are most probably surfaces bearing microbial crust and mud cracks showing reticular patterns.
3. Material and methods
The descriptive terminology and morphometric parameters are taken from Leonardi (Reference Leonardi1987), Demathieu (Reference Demathieu1990), Demathieu et al. (Reference Demathieu, Gand, Sciau and Freytet2002) and Marty (Reference Marty2008). We used the following standard abbreviations (Fig. 4): L, length of the footprint; W, width of the footprint; LII, LIII, LIV, lengths of the digits; II–IV, divarication angle between digit II and digit IV. We also used D, which corresponds to the length of the free part of digit III sensu Demathieu et al. (Reference Demathieu, Gand, Sciau and Freytet2002). In Section 4.b below, we describe these morphometric parameters by giving three values: the minimum, the average (in brackets) and the maximum. None of the specimens were collected. The orientations of tracks were measured on site. The morphometric values of the tracks from Mongisty D were compared with a large dataset including measurements of 431 tridactyl tracks from the Early Jurassic of the Causses Basin (based on data from Demathieu et al. Reference Demathieu, Gand, Sciau and Freytet2002 and Moreau et al. Reference Moreau, Baret, Trincal and André2012a, Reference Moreau, Trincal, Gand, Néraudeau, Bessière and Bourel2014, Reference Moreau, Trincal, André, Baret, Jacquet and Wienin2018) and of 80 tridactyl tracks from the Early Jurassic of Connecticut and Massachusetts, USA (data from Weems, Reference Weems2019). Following the diagnosis of Eubrontes giganteus, proposed by Olsen et al. (Reference Olsen, Smith and McDonald1998), we only used tracks that were more than 30 cm long, thus selecting 80 footprints out of the 83 E. giganteus footprints in the dataset of Weems (Reference Weems2019). Locomotion speed and height of the hip were estimated based on the formulas of Alexander (Reference Alexander1976) and Thulborn (Reference Thulborn1990). In order to produce 3D-photogrammetric reconstructions of the tracksite (Mallison & Wings, Reference Mallison and Wings2014; Matthews et al. Reference Matthews, Noble, Breithaupt, Falkingham, Marty, Richter, Falkingham, Marty and Richter2016), the software Agisoft PhotoScan Professional 1.2.4 was used to align and combine multiple digital photographs taken with a Nikon D5200 camera coupled with an AF-S NIKKOR 18–105 mm f/3.5–5.6G ED camera lens. The same software was used to produce 3D-photogrammetric textured meshes and digital elevation models (DEMs) in false colours. The 3D meshes are available online at https://figshare.com.

Fig. 4. Morphometric measurements taken on tridactyl tracks. L, length; W, width; D, length of the free part of digit III; LII, LIII and LIV, digit lengths; T: angle between the two most external digits.
4. Results
4.a. Sedimentology and track preservation
At Mongisty D, deposits of the Dolomitic Formation are exposed along a 4 m high outcrop. Based on the location of outcrops, we can estimate that the track-bearing surfaces of Mongisty A to C are all in stratigraphic layers located some metres above Mongisty D. Based on the main lithological variations along the stratigraphic section at Mongisty D, we distinguish eight levels (Level 1 to Level 8), and four facies (F1 to F4; Table 1; Figs 5 and 6). At the base of the section, Facies F1 is a yellowish, thinly laminated dolomite forming decimetre-thick beds with abundant hemispherical stromatolites (Fig. 6a–c). Stromatolitic domes are up to 40 cm in diameter (Fig. 6a–b). F2 consists of a grey to yellowish homogeneous dolomudstone forming centimetre- to decimetre-thick layers bearing mud cracks and microbial mats (Fig. 6d–e). F3 is a grey-blue to yellow clayey cryptalgal dolomudstone showing abundant millimetre-thick laminites with mud cracks and microbial mats. The microbial laminae are parallel to the bedding planes and are wavy to planar. F4 corresponds to yellowish matrix- to clast-supported breccia organized in lenticular, 0 to 15 cm thick, successive, and partially overlapping prograded lobes. Clasts consist of angular to sub-rounded, millimetric to centimetric (up to 2 cm), poorly sorted, randomly oriented, homogeneous dolostone intraclasts with a mudstone texture and floating in a dolomudstone matrix (Fig. 7). Clast arrangement is chaotic. Abundance of intraclasts is variable and the latter can be lacking in lobes locally. Thin-sections of samples from Facies F4 show irregular, alveolar, nodular structures similar to those described by Arrondeau et al. (Reference Arrondeau, Bodeur, Cussey, Fajerwerg and Yapaudjian1985) in the Hettangian Dolomitic Formation of Aveyron. Arrondeau et al. (Reference Arrondeau, Bodeur, Cussey, Fajerwerg and Yapaudjian1985) interpreted such structures as rhizoconcretions. The thin-sections do not reveal the presence of microbial mats in Facies F4.
Table 1. Microfacies

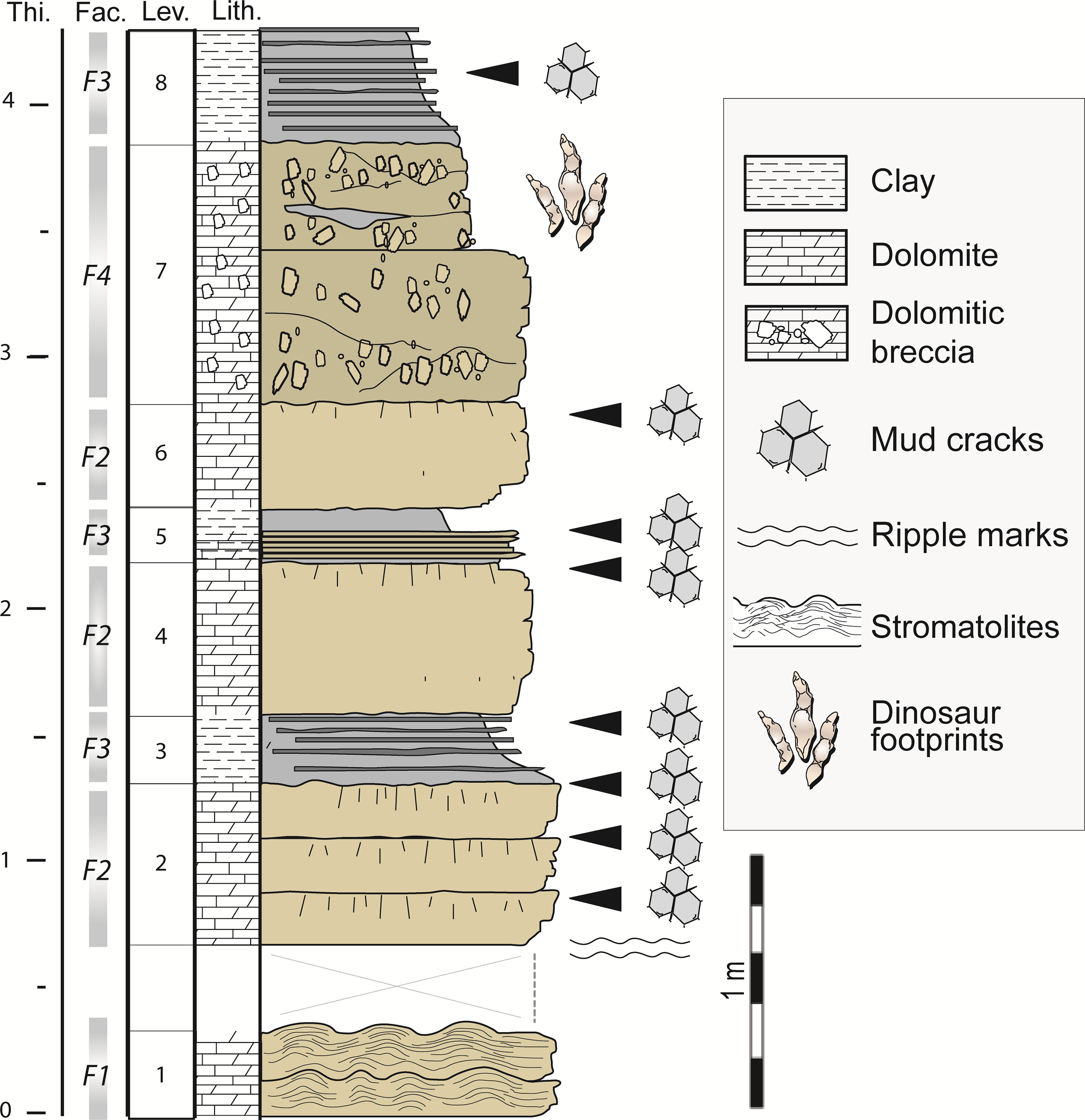
Fig. 5. Stratigraphic section of Mongisty D and location of the track-bearing level (Level 7).

Fig. 6. Facies. (a–b) Hemispherical stromatolites (Facies F1), upper view of Level 1, photograph (a) and interpretative sketch (b). (c) Transversal section of a thinly laminated hemispherical stromatolite (Facies F1) from Level 1, photograph and interpretative sketch. (d) Interface between Levels 4 and 5 with dolomudstone showing vertical mud cracks (white arrows; Facies F2) and dolomudstone laminites (Facies F3). (e) Surface of a dolomudstone slab from Level 2 with mud cracks (Facies F2). (f) Interface between Levels 7 and 8 showing matrix- to clast-supported breccia (Facies F4) and dolomudstone laminites (Facies F3).

Fig. 7. Details of the matrix- to clast-supported breccia (Facies F4) composing the track-bearing surfaces. (a–c) Tridactyl track Mon-57 (a) and detail of the sediment in an imprint of digit IV (b–c) showing abundant angular to sub-rounded millimetric to centimetric, poorly sorted, randomly oriented, homogeneous dolostone intraclasts floating in a dolomudstone matrix, photograph (b) and interpretative sketch (c). (d–e) Detail of the matrix- to clast-supported breccia, photograph (d) and interpretative sketch (e). (f–g) Microfacies of the track-bearing surfaces showing poorly sorted, randomly oriented, homogeneous dolostone intraclasts (In.) floating in a dolomudstone matrix (Mx.).
Only Level 7 (within Facies F4) yielded tracks. In the upper part of Level 7, six 0–15 cm thick and consecutive lobes (L1 to L6) bear footprints (Fig. 8a–b). All footprints from Mongisty D are concave epireliefs. The lithology is similar for the six lobes (matrix- to clast-supported breccia). The lateral extension of lobe L3 is limited to the northern part of the outcrop (Fig. 8a–b). To the south, L3 is missing but a clay lens (C1), up to 13 cm thick, is present between L2 and L4 (Fig. 8a–f). The lobes L4 to L6 overlap each other towards the south or the southeast, suggesting a progradation in that direction (Fig. 8c–d).

Fig. 8. Sedimentary structures of the tracksite and location of the trampled surfaces. (a) Map of the tracksite showing the location of the six lobes (L1–L6). (b) Three transversal sections (S1–S3) showing the structure of the six lobes (L1–L6) and location of the four trampled surfaces (TS1–TS4); footprints are indicated by blue vertical arrows; trampled surfaces are indicated by dotted blue lines and black arrows; Fac., facies; Lev., level. (c–d) View from the south showing the successive and overlapping decimetre-thick progradation lobes L4–L6; photograph (c) and interpretative sketch (d). (e–f) View from the east showing successive decimetre-thick progradation lobes (L1–L2 and L4–L5) alternating with a thin clay lens (C1); photograph (e) and interpretative sketch (f).
The surfaces of the six lobes L1 to L6 (Facies 4) bear footprints, whereas no tracks have been observed in the clay lens C1. At least four trampled surfaces (TS) can be distinguished. TS-1 to TS-3 correspond to the surface of lobes L1 to L3 respectively, whereas TS-4 corresponds to the surface of the three consecutive and prograded lobes L4 to L6 (Fig. 8a–d).
The trampled surface TS-4 is directly covered by Level 8 which is a clayey cryptalgal dolomudstone showing abundant thin laminites (Facies 3). Level 8 being not deformed by tracks, the tracks from the surface TS-4 are interpreted as true tracks. Most of the tracks deform a single lobe and did not propagate into the underlying lobe. However, some tracks are impressed over the limit of two overlapping lobes (where the overlying lobe is the thinnest) and deform both of them (e.g. limits between lobes L1/L2 and L4/L5). The trampled surface TS-1 bears true tracks and under-tracks. True tracks from TS-1 are directly covered by lobe L2 (Fig. 8e–f), whereas under-tracks from TS-1 correspond to tracks made on the surface of lobe L2 and that propagated into lobe L1 (Fig. 8e–f).
Diverse kinds of preservation are observable. Despite the relative coarseness of the sediment, tracks show a good anatomical fidelity and completeness (Fig. 9). Others are poorly marked and limited to isolated marks of digits (Fig. 9). The tracks exhibit a good physical preservation and a variable morphological preservation sensu Marchetti et al. (Reference Marchetti, Belvedere, Voigt, Klein, Castanera, Díaz-Martínez, Marty, Xing, Feola, Melchor and Farlow2019). Footprints could get a score of 0.5 to 2.5 according to the morphological preservation scale of Marchetti et al. (Reference Marchetti, Belvedere, Voigt, Klein, Castanera, Díaz-Martínez, Marty, Xing, Feola, Melchor and Farlow2019).

Fig. 9. Influence of water saturation on preservation of tracks in tidal breccia. (a) Five grades of water saturation (grade 1 to grade 5) influencing plasticity of substrate; grade 1, track Mon-34 (lobe L4); grade 2, track Mon-3 (lobe L5); grade 3, track Mon-7 (lobe L5); grade 4, track Mon-29 (lobe L4); grade 5, track Mon-91 (lobe L1). (b) Five kinds of preservation of tracks related to the physical properties of substrate. (c) Sketches of tracks. (d) Sketches showing the deformation of substrate in a transversal plan. (e) Characteristics of the different kinds of preservation.
4.b. Palaeoichnology
Due to the partial preservation of many traces, only 60 of them (located on the surface of the six lobes L1 to L6) can be ascribed to an ichnotaxon, of which 58 are Eubrontes giganteus Hitchcock and 2 are Grallator cf. lescurei Demathieu, Reference Demathieu1990. Due to the high number of overlapping footprints, trackways cannot be easily identified. Although several alignments of two consecutive tracks are observed, a single short trackway composed of three consecutive E. giganteus footprints is identified (Mon-1–Mon-3).
Eubrontes giganteus Hitchcock, Reference Hitchcock1845
Description. The trackway is 290 cm long (Mon-1–Mon-3; Fig. 10). Pace length is 122–(124)–126 cm and the stride is 252 cm long. Pes tracks are aligned with the trackway midline (Fig. 10). Footprints are tridactyl, longer than wide (L/W = 1.1–(1.3)–1.4), 30.0–(36.5)–47.0 cm long and 24.5–(29.5)–36.0 cm wide (Fig. 11; Tables 2 and 3). The divarication angle between digits II and IV is quite large (II–IV = 31.0°–(42.4°)–55.0°) and D is short (D = 9.0–(14.0)–17.5 and L/D = 2.1–(2.6)–3.3). The position of the digito-metatarsal pad of digit IV is commonly more proximal than that of digit II. Some footprints display wide impressions of digits with well-marked pads. When preserved, claw marks are elongated and pointed.

Fig. 10. Eubrontes giganteus trackway (Mon-1–Mon-3). (a–d) 3D-photogrammetric mesh with texture (a), 3D-photogrammetric mesh (b), DEM in false-colour depth map (c) and interpretative sketch (d).

Fig. 11. Eubrontes giganteus. (a) Mon-1. (b) Mon-3. (c) Mon-5. (d) Mon-7. (e) Mon-21. (f) Mon-22. (g) Mon-29. (h) Mon-66. (i) Mon-83. (j) Mon-106. For each footprint, photograph, DEMs in false-colour depth map and interpretative sketch are given (from left to right).
Table 2. Values measured on Hettangian tridactyl tracks from Mongisty D. L: length of the trace; W: width of the trace; D: length of the free part of digit III; LII, LIII, LIV: lengths of the digits; II–IV, divarication angle between digit II and digit IV (all in cm except II–IV in degrees); and ratio L/W, L/D and (L − D)/D

Table 3. Eubrontes giganteus. Minimum, maximum, average and standard deviation values for each morphometric parameter (L: length of the trace; W: width of the trace; D: length of the free part of digit III; LII, LIII, LIV: lengths of the digits; II–IV: divarication angle between digit II and digit IV; all in cm except II–IV in degrees) and ratio L/W, L/D and (L − D)/D; n = number of measured specimens

Remarks. The ichnogenus Eubrontes Hitchcock, Reference Hitchcock1845 is well known in diverse Late Triassic – Early Jurassic formations throughout the world (e.g. Lucas et al. Reference Lucas, Klein, Lockley, Spielmann, Gierliński, Hunt and Tanner2006 a and references therein; Dalman, Reference Dalman2012; Lagnaoui et al. Reference Lagnaoui, Klein, Voigt, Hminna, Saber, Schneider and Werneburg2012; Xing et al. Reference Xing, Peng, Ye, Lockley, McCrea, Currie, Zhang and Burns2014; Getty & Fox, Reference Getty and Fox2015; Wagensommer et al. Reference Wagensommer, Latiano, Mocke and D’Orazi2016; Sciscio et al. Reference Sciscio, Bordy, Abrahams, Knoll and McPhee2017). In the Early Jurassic of France, the ichnogenus Eubrontes was reported from Aveyron (Demathieu & Sciau, Reference Demathieu and Sciau1999; Demathieu et al. Reference Demathieu, Gand, Sciau and Freytet2002; Moreau et al. Reference Moreau, Gand, Fara and Michelin2012 b), Gard (Sciau, Reference Sciau2003), Lot (Lange-Badré & Lafon, Reference Lange-Badré and Lafon2000), Lozère (Moreau et al. Reference Moreau, Trincal, Gand, Néraudeau, Bessière and Bourel2014, Reference Moreau, Trincal, André, Baret, Jacquet and Wienin2018) and Vendée (Lapparent & Montenat, Reference Lapparent and Montenat1967). Amongst these Hettangian–Sinemurian tracks, the ichnospecies E. giganteus was only reported from Aveyron and Gard in the Causses Basin (Demathieu et al. Reference Demathieu, Gand, Sciau and Freytet2002; Sciau, Reference Sciau2003). Based on ten large tracks from the site of Peyre, Demathieu & Sciau (Reference Demathieu and Sciau1999) were the first to identify the ichnogenus Eubrontes in the Hettangian Dolomitic Formation of the Causses Basin. They initially ascribed the tracks to Eubrontes divaricatus Hitchcock, 1865. Later, based on the revision of the ichnogenus by Olsen et al. (Reference Olsen, Smith and McDonald1998), Demathieu et al. (Reference Demathieu, Gand, Sciau and Freytet2002) renamed the tracks from Peyre Eubrontes giganteus. Using the formula of Alexander (Reference Alexander1976) and Thulborn (Reference Thulborn1990), and based on the trackway Mon-1–Mon-3, we estimate the hip height of the track maker between 1.71 and 1.86 m and their speed between 8.47 and 9.28 km h−1, suggesting a walking gait.
Grallator cf. lescurei Demathieu, Reference Demathieu1990
Description. Tracks are tridactyl, longer than wide (L/W = 1.4), 26 cm long and 18.0 cm wide (Fig. 12; Tables 2 and 4). The angle between digits II and IV is 39°–(40°)–41°. Impressions of digits are separated, well-defined and elongated. The traces of digits III and IV are similar in length. D is quite long (D = 11.0–(11.5)–12.0). Impressions of digital pads are well preserved and are circular to oval. The position of the digito-metatarsal pad of digit IV is more proximal than that of digit II. There is no plantar impression.

Fig. 12. Grallator cf. lescurei. (a) Mon-104. (b) Mon-112. For each track, photograph, DEMs in false-colour depth map and interpretative sketch are given (from left to right).
Table 4. Grallator cf. lescurei. Minimum, maximum, average and standard deviation values for each morphometric parameter (L: length of the trace; W: width of the trace; D: length of the free part of digit III; LII, LIII, LIV: lengths of the digits; II–IV: divarication angle between digit II and digit IV; all in cm except II–IV in degrees) and ratio L/W, L/D and (L − D)/D; n = number of measured specimens

Remarks. Grallator lescurei was erected by Demathieu (Reference Demathieu1990) based on tracks from Saint-Léons in Aveyron (southern France). In the Early Jurassic of France, G. lescurei was only reported from Aveyron, Gard and Hérault (Demathieu, Reference Demathieu1990; Demathieu et al. Reference Demathieu, Gand, Sciau and Freytet2002; Sciau, Reference Sciau2003). Grallatoroid footprints showing strong similarity with G. lescurei were also reported from the Hettangian Dolomitic Formation of Lozère (Moreau et al. Reference Moreau, Trincal, Gand, Néraudeau, Bessière and Bourel2014).
5. Discussion
5.a. Comparison with tridactyl tracks from the Causses Basin
Amongst Hettangian–Sinemurian tridactyl footprints from the Causses Basin, Demathieu et al. (Reference Demathieu, Gand, Sciau and Freytet2002) described six ichnospecies as currently identified: Dilophosauripus williamsi Welles, 1971, Eubrontes giganteus, Grallator variabilis, Grallator lescurei, Grallator minusculus Hitchcock, 1868 and Grallator sauclierensis Demathieu & Sciau, Reference Demathieu and Sciau1992. It is interesting to note that some authors consider Dilophosauripus Welles, 1971 as a junior synonym of Eubrontes (see references in Lucas et al. Reference Lucas, Lockley, Hunt, Milner and Tanner2006 b; Lockley et al. Reference Lockley, Gierliński and Lucas2011). Recently, based on morphometrical comparisons, Gand et al. (Reference Gand, Fara, Durlet, Caravaca, Moreau, Baret, André, Lefillatre, Passet, Wiénin and Gély2018) recommended using Kayentapus Welles, 1971 rather than Dilophosauripus for the lowermost Jurassic tracks of the Causses Basin.
Based on the parameters L, L/W and (L − D)/D, Figure 13 shows bivariate diagrams for the diverse ichnospecies from the Lower Jurassic of the Causses Basin. The two grallatoroid tracks from Mongisty D (Mon-104 and Mon-112) are much longer than the small Grallator ichnospecies from the Causses Basin (Grallator sauclierensis, Grallator variabilis; Fig. 13). They mainly differ from G. minusculus by being a longer length of the free part of digit III ((L − D)/D = 1.2–(1.3)–1.4). Kayentapus tracks from the Causses Basin differ from G. cf. lescurei tracks from Mongisty D in being as long as wide (L/W = 0.8–(1.0)–1.3 and 1.4, respectively) and in having higher II–IV values (35–(68)–98° and 39–(40)–41°, respectively). Demathieu (Reference Demathieu1990) described G. lescurei as: ‘Digits slender, well specified and separated in the proximal part. Projection of digit III beyond the other toes of moderate size (ratio III/D = 1.70 +/− 0.14); divarication between digits II and IV rather great for this ichnogenus, about 50.9° +/− 4.3° (instead of 24.9 +/− 3.4°, as exhibited by the type species). Differences between digits II and IV higher than G. sillimani. Length/width ratio about 1.2 +/− 0.06.’ Although Demathieu (Reference Demathieu1990) proposed in the diagnosis of G. lescurei a smaller L/W ratio than G. cf. lescurei from Mongisty D (1.4 on average; Table 4), the data set used to describe this ichnotaxon in Demathieu et al. (Reference Demathieu, Gand, Sciau and Freytet2002) included values of L/W ratio that range from 1.0 to 1.9. Although Demathieu (Reference Demathieu1990) diagnosed G. lescurei with a divarication angle higher than that observed in G. cf. lescurei from Mongisty D (40° on average), the data set used in Demathieu et al. (Reference Demathieu, Gand, Sciau and Freytet2002) included values of II–IV that range from 30° to 80°. This demonstrates the broad variability of the divarication angle II–IV, probably depending on the nature and the hydration of substratum, as well as on the speed of the animal (Milàn, Reference Milàn2003; Milàn & Falkingham, Reference Milàn, Falkingham, Falkingham, Marty and Richter2016).

Fig. 13. Footprints from Mongisty D compared to tridactyl tracks from the Early Jurassic of the Causses Basin (based on data from Demathieu et al. Reference Demathieu, Gand, Sciau and Freytet2002; Moreau et al. Reference Moreau, Baret, Trincal and André2012a, Reference Moreau, Trincal, Gand, Néraudeau, Bessière and Bourel2014, Reference Moreau, Trincal, André, Baret, Jacquet and Wienin2018) and the Early Jurassic of the eastern USA (based on data from Weems, Reference Weems2019), in a bivariate diagram L vs (L− D)/D (a) and in a bivariate diagram L vs L/W (b). L in metres.
The morphometric space of the largest Grallator Hitchcock, 1858 ichnospecies (G. lescurei and G. minusculus) and that of E. giganteus from Mongisty D are distinct (Fig. 13). Track lengths of G. lescurei and G. minusculus are shorter (12.5–(25.5)–36.0 and 24.0–(30.1)–35.0 cm, respectively) than the well-preserved tracks of E. giganteus from Mongisty D. G. lescurei and G. minusculus display digit imprints that seem more slender than E. giganteus from Mongisty D, although quantitative values are not available for a formal comparison. G. minusculus also shows a higher L/W ratio than E. giganteus from Mongisty D (0.9–(1.6)–1.9 and 1.1–(1.3)–1.4, respectively). Kayentapus from the Causses Basin differs from E. giganteus at Mongisty D by shorter tracks that are as long as wide, and higher II–IV values (35–(68)–98° and 31.0–(42.4)–55°, respectively).
Based on material from the Lower Jurassic of the USA, Olsen et al. (Reference Olsen, Smith and McDonald1998) described Eubrontes giganteus as: ‘Large (>30 cm long), functionally tridactyl ichnite … and the length to width ratio is about 1.4 to 1.5; projection of digits II and IV along the axis of digit III about equal; and divarication of outer digits 30°–40°.’ Even if they used the ichnospecies E. giganteus, Demathieu et al. (Reference Demathieu, Gand, Sciau and Freytet2002) indicated that the diagnosis of E. giganteus proposed by Olsen et al. (Reference Olsen, Smith and McDonald1998) was not entirely appropriate for the track assemblage from the Causses Basin. Demathieu et al. (Reference Demathieu, Gand, Sciau and Freytet2002) mentioned that E. giganteus tracks from this area differs from those described by Olsen et al. (Reference Olsen, Smith and McDonald1998) in showing: small claw marks on impressions of digits II, III and IV; pads not well marked or absent; smaller L/W ratio (1.0–1.4); and higher II–IV values (41–72°). The length and width of E. giganteus reported by Demathieu et al. (Reference Demathieu, Gand, Sciau and Freytet2002) and those from Mongisty D are very close (length: 39.0 cm and 36.5 cm respectively on average; width: 31.4 cm and 29.5 cm respectively on average; Fig. 13). However, E. giganteus from Mongisty D differs from those reported by Demathieu et al. (Reference Demathieu, Gand, Sciau and Freytet2002) in having smaller II–IV values (up to 55° and up to 72° respectively; Tables 2 and 3), well-marked phalangeal pads and elongated claw marks. Thus, tracks from Mongisty D fit the diagnosis of E. giganteus better than those described in Demathieu et al. (Reference Demathieu, Gand, Sciau and Freytet2002). In Figure 13, the morphometric space of E. giganteus from the Causses Basin and those from the USA largely overlap. However, E. giganteus from the USA tend to have shorter length and can have a slightly higher L/W ratio.
In the lowermost Jurassic deposits from France, another ichnospecies of Eubrontes was reported from the Hettangian of the Veillon tidal flat, in Vendée: E. veillonensis Lapparent & Montenat, Reference Lapparent and Montenat1967. We may notice that the length, width and L/W ratio of E. veillonensis (34 cm, 28 cm and 1.21 respectively) clearly fall within the values of E. giganteus from the Causses Basin (Fig. 13). Lapparent & Montenat (Reference Lapparent and Montenat1967) erected this new ichnospecies and explained that it is very close to E. giganteus from Connecticut (Hitchcock, Reference Hitchcock1845). They distinguished these two ichnospecies based on a rather subjective criterion, namely that their ‘outlines are slightly different’ (Lapparent & Montenat, Reference Lapparent and Montenat1967, p. 26). In the absence of a robust comparison, the authors failed to distinguish E. veillonensis from E. giganteus. Demathieu & Sciau (Reference Demathieu and Sciau1999) indicated that E. veillonensis mainly differs from E. giganteus by the values of the divarication angle formed by the lateral digits II and IV. However, as observed on specimens from Mongisty D and from other areas in the Causses Basin (Demathieu et al. Reference Demathieu, Gand, Sciau and Freytet2002), the divarication angle can be quite variable amongst footprints of E. giganteus. Without synonymizing these two ichnospecies, Demathieu (Reference Demathieu2003) and Gand et al. (Reference Gand, Demathieu and Montenat2007) further suggested that they are very close to each other. Pending a robust comparison, for the time being we agree with these authors.
5.b. Ichnotaphonomy
Vertebrate tracks are commonly impressed in sediments that range from ‘clay-mineral or lime muds to siliciclastic or bioclastic-ooid sands’ (Allen, Reference Allen1997, p. 485). They are mainly preserved in fine-grained sediments corresponding to low-energy depositional palaeoenvironments (e.g. Laporte & Behrensmeyer, Reference Laporte and Behrensmeyer1980; Allen, Reference Allen1997; Alcalá et al. Reference Alcalá, Pérez-Lorente, Luque, Cobos, Roya-Torres and Mampel2014). Regionally, in the dozens of tetrapod tracksites reported from the Dolomitic Formation of the Causses Basin so far, footprints are always preserved in homogeneous dolomudstone beds or laminites showing abundant microbial mats without coarse intraclasts or coarse detrital particles (e.g. Demathieu, Reference Demathieu1993; Demathieu & Sciau, Reference Demathieu and Sciau1999; Demathieu et al. Reference Demathieu, Gand, Sciau and Freytet2002; Sciau, Reference Sciau2003; Moreau et al. Reference Moreau, Trincal, Gand, Néraudeau, Bessière and Bourel2014, Reference Moreau, Trincal, André, Baret, Jacquet and Wienin2018, Reference Moreau, Fara, Néraudeau and Gand2019 a). These track-bearing surfaces were interpreted as tidal flat deposits. As shown by some taphonomic experiences in present-day tidal-flat environments, a track can be consolidated by desiccation or lithification of the mat, or by ongoing growth of the mat (Marty et al. Reference Marty, Strasser and Meyer2009). The abundant microbial mats in archosaur tracksites from the Dolomitic Formation of the Causses Basin were probably crucial in the preservation of the frequent vertebrate tracks.
In some tracksites throughout the world, footprints are preserved in coarser sediments that mainly consist of silts or sandstones deposited in alluvial/fluvial (e.g. Tucker & Burchette, Reference Tucker and Burchette1977; Courel & Demathieu, Reference Courel and Demathieu2000; Gand & Demathieu, Reference Gand and Demathieu2005) and aeolian context (e.g. Loope, Reference Loope2006; Milàn & Loope, Reference Milàn and Loope2007; Candia Halupczok et al. Reference Candia Halupczok, Sánchez, Veiga and Apesteguía2018). In most of these cases, tracks are reported from coarse silts to medium sands. Only very rare sites yield tracks preserved in coarse sands and microconglomerates (Szewczyk et al. Reference Szewczyk, Vennin, Moreau, Gand, Verolet, Klee and Fara2020). In contrast to all tracksites reported from the Lower Jurassic Dolomitic Formation of the Causses Basin, Mongisty D stands as an exceptional locality because footprints are preserved in a relatively coarse sediment (a dolomitic breccia) and in a facies apparently barren of microbial mats.
The morphology and dimensions of footprints depend on several biotic factors, such as the anatomy of the pes, the weight, the speed, the gait, the behaviour and the force applied on the sediment by the track maker (Allen, Reference Allen1997; Falkingham et al. Reference Falkingham, Bates, Margetts and Manning2011; Gatesy & Falkingham, Reference Gatesy and Falkingham2017). In addition, the formation and the resulting morphology of footprints also depend on substrate properties such as lithology (including the grain size of allochems/clasts), the morphology of the walking surface, its water saturation and the presence of microbial mats. Later erosional processes should also be added to this long list of parameters. At Mongisty D, the preservation of tracks varies a lot within the same trampled surface, and from one trampled surface to another (Fig. 9). As erosional processes alone are insufficient to explain such morphological contrasts, this indicates that tracks were made at different moments when the water saturation of sediment was not homogeneous. In Figure 9, we distinguish five grades of water saturation (grade 1 to grade 5) influencing the track preservation and classified from lower to higher water contents and linked with the solidity of substrate. These five grades were distinguished using true tracks only, and a single trampled surface can show several of these grades (for example, the trampled surface TS-4 combines grade 1 to grade 4). For grade 1, as footprints are poorly marked and digit impressions are only partially visible, tracks were made in a consistent/solid and dry sediment with a very low plasticity. For grade 2, as footprints are well marked, the plasticity of the sediment was higher than in grade 1. However, the relatively shallow tracks with well-defined walls revealing foot morphology suggest the water content was not so high. Only the tracks produced in grade 2 conditions exhibit all diagnostic characters of the ichnotaxa and all detailed marks of digits, pads and claws. The dolomitic breccia with coarse intraclasts was cohesive enough to prevent the disappearance of the tracks thanks to the presence of an abundant matrix between dolostone grains (Fig. 7). For grades 3 and 4, as footprints are very deep with narrow traces of digits without marks of pads and some deformation of the track walls (especially in grade 4, for which digit imprints are nearly ‘closed’), footprints were made in a sediment with high water saturation and high plasticity. In grade 5, as footprints consist of ovoid concavities and track walls are nearly totally collapsed (traces of digit barely visible), it appears that the sediment could not remain consistent after the kick-off phase of the track maker’s foot. In grade 5, footprints were made in sediment with very high water saturation and very low plasticity. The diverse types of preservation observed on this nearly monospecific track assemblage (E. giganteus) highlight the strong influence of the physical properties of the sediment upon track preservation (see also Gatesy & Falkingham, Reference Gatesy and Falkingham2017).
5.c. Depositional environment
The hemispherical stromatolites (Facies F1) present at the base in the Mongisty D section (Fig. 5) are characteristic of a subtidal to intertidal depositional environment (Jahnert & Collins, Reference Jahnert and Collins2012). Such laminated biosedimentary structures were reported from several Hettangian–Sinemurian archosaur tracksites of the Causses Basin (Demathieu et al. Reference Demathieu, Gand, Sciau and Freytet2002; Sciau, Reference Sciau2003; Moreau, Reference Moreau2011). The abundance of mud cracks in Facies F2 and F3 indicates that sediments were deposited in an environment which was periodically exposed or subaerial. The cryptalgal laminites (Facies F3) are characteristic of regularly flooded environments, such as the intertidal and supratidal zones of a tidal flat in which microbial mats are growing (e.g. Alsharhan & Kendall, Reference Alsharhan and Kendall2003; Hamon, Reference Hamon2004; Matysik, Reference Matysik2016). The lack of bioclasts and coarse particles in Facies F2 and F3 suggests limited storm-generated transport and a relatively long distance to the subtidal zone. Locally in the Causses Basin, some layers with halite pseudomorphs were reported from the Dolomitic Formation and suggest evaporitic conditions (Moreau, Reference Moreau2011). In contrast to Facies F1 to F3, the nature of Facies 4 (matrix-supported breccia) indicates high-energy events, such as the sudden reworking of material by floods or storms in a supratidal zone. The presence of rhizoconcretions and dinosaur tracks in Facies 4 attests to periods of subaerial exposure and pedogenesis. The dolostone intraclasts present in the matrix-supported breccia are remnants of dry mud beds (partially lithified) that were eroded further upstream and subsequently transported in mud flows. The origin of brecciation could be explained by a phase of dewatering during prolonged subaerial exposure or initial formation of palaeosols (Kendall & Warren, Reference Kendall and Warren1987). As observed in diverse sedimentological contexts, the trampling of a surface by large vertebrates may also contribute to brecciation (e.g. Dalla Vecchia et al. Reference Dalla Vecchia, Tarlao, Tentor, Tunis and Venturini2000; Loope, Reference Loope2006; Marty, Reference Marty2008; Candia Halupczok et al. Reference Candia Halupczok, Sánchez, Veiga and Apesteguía2018). Matrix- to clast-supported breccia organized in lenticular lobes (Facies F4) indicates that the mudflat of the Causses Basin was sporadically affected by large floods that reworked and redeposited intraclasts. The abundance and good preservation of footprints on the trampled surfaces (TS-1 to TS-4) demonstrate that erosion was not so intense during and after each flood (at least locally). The hardening of trampled surfaces after lobes were deposited and emerged was probably crucial in the preservation of tracks. The preservation of tetrapod tracks in such a depositional context remains exceptional. Based on sedimentological and ichnotaphonomical observations, Figure 14 shows a model to explain the formation of the Mongisty D tracksite. Such an occurrence of dinosaur tracks in tidal matrix- to clast-supported breccia remains extremely rare in the world (e.g. Marty, Reference Marty2008). Overall, all facies suggest that the depositional environments of Mongisty D varied from subtidal to intertidal/supratidal areas in a large and protected flat marsh. The absence of invertebrate skeletal fossils suggests unfavourable life conditions.

Fig. 14. Ichnotaphonomic model of the formation of the six overlapping decimetre-thick progradation lobes (L1–L6) and the four trampled surfaces (TS1–TS4). (a–c) Progradation and emersion of lobe L1; deformation of its surface by dinosaur tracks (trampled surfaces TS-1). (d–f) Progradation and emersion of lobe L2; deformation of its surface by dinosaur tracks (trampled surfaces TS-2). (g–i) Progradation of lobe L3 followed by the deposition of the clay lens C1; emersion and deformation of the surface of L3 by dinosaur tracks (trampled surfaces TS-3). (j–l) Progradation of the three partially overlapping lobes L4 to L6 followed by an emersion; deformation of the three consecutive lobes L4 to L6 by dinosaur tracks (trampled surfaces TS-4). Blue dotted lines: trampled surfaces; vertical black wavy lines: drying of the substrate; vertical blue arrows: substrate deformed by dinosaur feet.
5.d. Track makers and their behaviour
Grallator and Eubrontes are tridactyl ichnogenera and show a similar phalangeal formula (type 3, 4 and 5 for toes II, III and IV). This osteological architecture corresponds to those of theropod dinosaurs (Baird, Reference Baird1957; Olsen et al. Reference Olsen, Smith and McDonald1998; Demathieu et al. Reference Demathieu, Gand, Sciau and Freytet2002). Worldwide, body fossils of Early Jurassic theropods are attributed to Coelophysoidea and Ceratosauria and reported from Africa, Antarctica, China, Europe and the USA (Weishampel et al. Reference Weishampel, Dodson and Osmolska2004; Smith et al. Reference Smith, Makovicky, Hammer and Currie2007; Xing et al. Reference Xing, Bell, Rothschild, Ran, Zhang, Dong, Zhang and Currie2013). In Europe, their body fossils are known from the Hettangian–Sinemurian deposits of England, France, Italy and Luxembourg (Larsonneur & Lapparent, Reference Larsonneur and Lapparent1966; Carrano & Sampson, Reference Carrano and Sampson2004; Delsate & Ezcurra, Reference Delsate and Ezcurra2014; Dal Sasso et al. Reference Dal Sasso, Maganuco and Cau2018). In France, Hettangian theropods are only represented by the Coelophysoidea Lophostropheus airelensis Cuny & Galton, Reference Cuny and Galton1993 from the Moon–Airel Formation in Normandie (northwestern France; Larsonneur & Lapparent, Reference Larsonneur and Lapparent1966; Cuny & Galton, Reference Cuny and Galton1993; Ezcurra & Cuny, Reference Ezcurra and Cuny2007) but they are currently unknown in the lowermost Jurassic of the Causses Basin. Although theropods are the most probable track makers of E. giganteus from the Hettangian of the Causses Basin, we notice that Weems (Reference Weems, Letourneau and Olsen2003, Reference Weems2019) suggested that the track makers of some E. giganteus from the Early Jurassic of the USA probably included basal sauropodomorphs.
Based on parallel trackways from the Early Jurassic of the USA, some authors (e.g. Hitchcock, Reference Hitchcock1848; Ostrom, Reference Ostrom1972) suggested that track makers of E. giganteus were gregarious. At the Mongisty D outcrop, the high density of tracks that are preferentially oriented to the north (Fig. 3) could be regarded as evidence in favour of such a hypothesis. However, the different types of preservation of true tracks observed among E. giganteus (Fig. 9) and the presence of four trampled surfaces (Fig. 14) indicate that the footprints were not made at the same time. There is no evidence for the passage of a large group. Based on material from the USA, the gregarious behaviour of the track makers of E. giganteus was debated by Getty et al. (Reference Getty, Hardy and Bush2015, Reference Getty, Aucoin, Fox, Judge, Hardy and Bush2017) who showed that such a parallel orientation of trackways can be mainly explained by physical barriers, suggesting that the track makers of E. giganteus were solitary. In a synthesis of many tracksites from the Hettangian–Sinemurian of the Causses Basin, Demathieu et al. (Reference Demathieu, Gand, Sciau and Freytet2002) observed that trackways show two preferential and perpendicular orientations at the scale of the basin. These authors proposed that dinosaurs from this area preferentially walked along the shoreline or were walking towards it.
6. Conclusion
Overall, the 118 large tridactyl tracks discovered at Mongisty D are significant for the following reasons:
-
1) They are preserved on the surface of lobes corresponding to matrix- to clast-supported breccia. This sharply contrasts with all other tracksites from the Dolomitic Formation in the Causses Basin, in which tracks are usually preserved in fine-grained sediments corresponding to low-energy depositional palaeoenvironments. The preservation of dinosaur tracks in such a coarse-grained sediment is also rare at the global scale.
-
2) Tracks from Mongisty D were formed in a subtidal to intertidal/supratidal setting in a large and protected flat marsh. This setting was sporadically affected by large mud flows that redeposited mudstone intraclasts.
-
3) Most of the tracks are ascribed to Eubrontes giganteus and they represent the highest abundance and density of this ichnospecies in the Early Jurassic of the Causses Basin. These footprints were not made at the same time, excluding the passage of a large group.
Acknowledgements
We address our sincere thanks to Jean Froment, the mayor of the municipality of Montjaux, who gave his agreement for palaeontological excavations. We thank Christophe Saint-Pierre, the mayor of the municipality of Millau, who lent an excavator during fieldwork sessions. We thank Alain Bernat, Jean-Marie Gineste, Guy Noirfalise, Guillaume Puech, Laurent Sciau as well as the association ‘Les randos à Lolo’ for their help during excavations. We thank the two anonymous reviewers for their constructive and thoughtful reviews of the manuscript. We express our gratitude to Guy Noirfalise for the photographs used in Figure 2.
Conflict of interest
None.