1. Introduction
The Barents Shelf and adjacent Svalbard, Franz Josef Land and Novaya Zemlya archipelagos (Fig. 1) preserve a near-continuous succession of Triassic strata that were deposited in a long-lasting intracratonic basin (Sømme et al. Reference Sømme, Doré, Lundin and Tørudbakken2018). In contrast to arid-terrestrial and carbonate deposits typifying ‘classical’ Triassic localities of the Germanic and Alpine realms, the Triassic strata of the Barents Sea reflect a range of deltaic and shallow-marine palaeoenvironments from a warm-temperate palaeoclimatic belt along the northern coast of Pangaea (Boucot et al. Reference Boucot, Xu and Scotese2013).
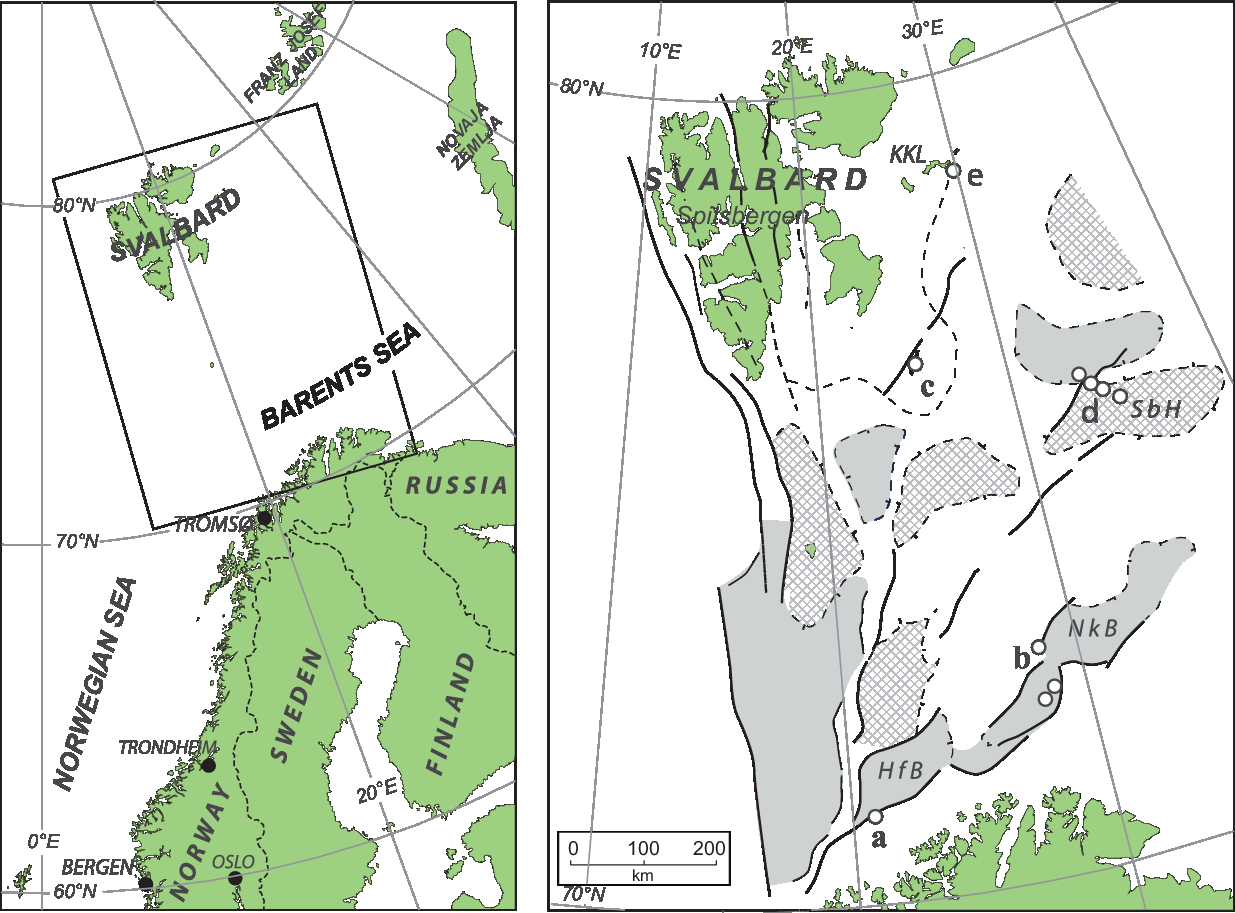
Fig. 1. Geological and structural map of Svalbard and the Barents Sea (after Vigran et al. Reference Vigran, Mangerud, Mørk, Worsley and Hochuli2014; simplified from Dallmann, Reference Dallmann1999). Reference localities: a, exploration wells 7120/12-2; b, 7228/2-1s, 7-1a and 9-1s; c, Hopen; d, stratigraphic boreholes 7533/2-U-1, 2-U-2 and 3-U-7, and 7534/4-U-1; e, boreholes 7830/3-U-1, 5-U-1, 6-U-1, and 7831/2-U-1 and 2-U-2. HfB – Hammerfest Basin; NkB – Nordkapp Basin; SbH – Sentralbanken High; KKL – Kong Karls Land.
Following the discovery of hydrocarbons in the Norwegian Barents Sea in the early 1980s, palynostratigraphy has become an essential tool for the dating of Triassic strata in the region, leading to the description of palynozonal schemes by Hochuli et al. (Reference Hochuli, Colin, Vigran and Collinson1989) and Vigran et al. (Reference Vigran, Mangerud, Mørk, Worsley and Hochuli2014). Nonetheless, Late Triassic zonal resolution remains relatively low, with only four zones established for an interval spanning approximately 36 Ma (Ogg et al. Reference Ogg, Ogg and Gradstein2016). The absence of a high-resolution Upper Triassic palynostratigraphy was the motivation for a series of studies focused on the Middle–Upper Triassic (Anisian–Rhaetian) stratigraphy of Svalbard and the Norwegian Barents Sea by the current authors (Paterson & Mangerud, Reference Paterson and Mangerud2015, Reference Paterson and Mangerud2017; Paterson et al. Reference Paterson, Mangerud, Cetean, Mørk, Lord, Klausen and Mørkved2016, Reference Paterson, Mangerud and Mørk2017, Reference Paterson, Morris and Mangerud2019a). Collectively, these form the basis of a refined palynozonal scheme that is presented in Section 3 (Revised zonal scheme).
The Late Triassic palaeoclimate was characterized by prevailing aridity in many regions, particularly in central Pangaean localities, but this was briefly interrupted by humid conditions during the Carnian Pluvial Episode (Simms & Ruffell, Reference Simms and Ruffell1989, Reference Simms and Ruffell2018; Simms et al. Reference Simms, Ruffell and Johnson1994; Ruffell et al. Reference Ruffell, Simms and Wignall2015). Intriguingly, the epoch was also important in terms of biotic development on land and in the ocean, with the origination and diversification of several new groups including dinosaurs, lizards, mammals, calcareous nannoplankton and dinoflagellate cysts (Furin et al. Reference Furin, Preto, Rigo, Roghi, Gianolla, Crowley and Bowring2006; Benton et al. Reference Benton, Bernardi and Kinsella2018; Bernardi et al. Reference Bernardi, Gianolla, Petti, Mietto and Benton2018; Mangerud et al. Reference Mangerud, Paterson and Riding2018). Since plant distribution is largely controlled by climate, a more detailed record of the palynofloral succession from the Barents Sea region contributes to our understanding of the impact of such climatic perturbations on the biotas of northern Pangaea.
Collectively, the data gathered by previous studies has revealed that substantial differences exist between the Late Triassic palynofloras in the Barents Sea region compared with those described from central and southern European localities. Such differences likely reflect palaeoclimatic control across a palaeolatitudinal gradient (Hochuli & Vigran, Reference Hochuli and Vigran2010; Vigran et al. Reference Vigran, Mangerud, Mørk, Worsley and Hochuli2014). Since independent age control indicates that many palynomorph index taxa have significantly earlier first occurrences in the Greater Barents Sea area (Smith, Reference Smith1982; Hochuli et al. Reference Hochuli, Colin, Vigran and Collinson1989; Hochuli & Vigran, Reference Hochuli and Vigran2010; Paterson & Mangerud, Reference Paterson and Mangerud2015; Paterson et al. Reference Paterson, Mangerud, Holen, Landa, Lundschien and Eide2019a), it appears the humid coastal region of northern Pangaea was a ‘cradle’ for floristic evolution during Late Triassic time. Refinement of existing palynozonation is therefore not only important for regional stratigraphic correlations, but is also critical for understanding the timing and drivers for the origination and dispersal of Late Triassic floras.
2. Geological background
2.a. Lithostratigraphy
The Triassic succession in the Barents Sea region primarily comprises siliciclastic deposits reflecting a suite of coastal plain, deltaic, prodeltaic and restricted marine palaeoenvironments. Seismic data show these as NW-prograding clinoform packages that define a series of seismic sequences (Riis et al. Reference Riis, Lundschien, Høy, Mørk and Mørk2008; Glørstad-Clark et al. Reference Glørstad-Clark, Faleide, Lundschien and Nystuen2010, Reference Glørstad-Clark, Birkeland, Nystuen, Faleide and Midtkandal2011; Høy & Lundschien, Reference Høy, Lundschien, Spencer, Embry, Gautier, Stoupakova and Sørensen2011; Klausen et al. Reference Klausen, Ryseth, Helland-Hansen, Gawthorpe and Laursen2015). Following the catastrophic destruction of long-lasting carbonate platform environments during the Permian–Triassic mass extinction, siliciclastic deposits derived principally from the Polar Urals and Fennoscandia rapidly filled the basin, prograding as far as the Svalbard Archipelago by the Carnian Age (Lundschien et al. Reference Lundschien, Høy and Mørk2014; Eide et al. Reference Eide, Klausen, Katkov, Suslova and Helland-Hansen2018; Sømme et al. Reference Sømme, Doré, Lundin and Tørudbakken2018). The progradation of deltaic systems during the Triassic Period likely played an important role in the floristic development of the region because, by the late Carnian Age, it had led to the establishment of a vast area of coastal plain and marginal marine habitats (Paterson et al. Reference Paterson, Mangerud, Cetean, Mørk, Lord, Klausen and Mørkved2016; Klausen et al. Reference Paterson, Mangerud, Holen, Landa, Lundschien and Eide2019).
The lithostratigraphic scheme for the region outlined by Mørk et al. (Reference Mørk, Dallman, Dypvik, Johannessen, Larssen, Nagy, Nøttvedt, Olaussen, Pčhelina, Worsley and Dallman1999) divides the succession into two groups: the Sassendalen Group (Changhsingian/Induan–Anisian) and the Kapp Toscana Group (Ladinian – Middle Jurassic) (Fig. 2). The Sassendalen Group comprises the Havert (Changhsingian–Olenekian), Klappmyss (Olenekian) and Kobbe (Anisian) formations; organic-rich mudrocks of the Steinkobbe Formation are correlative (in part) to the latter. The Triassic portion of the Kapp Toscana Group includes the Snadd (Ladinian – upper Carnian), Fruholmen (upper Carnian – Rhaetian) and Tubåen (Rhaetian–Hettangian) formations. The sequence stratigraphy of the Triassic deposits on the Norwegian Barents Shelf has been described by a series of studies (including Riis et al. Reference Riis, Lundschien, Høy, Mørk and Mørk2008; Glørstad-Clark et al. Reference Glørstad-Clark, Faleide, Lundschien and Nystuen2010, Reference Glørstad-Clark, Birkeland, Nystuen, Faleide and Midtkandal2011; Høy & Lundschien, Reference Høy, Lundschien, Spencer, Embry, Gautier, Stoupakova and Sørensen2011; Klausen et al. Reference Klausen, Ryseth, Helland-Hansen, Gawthorpe and Laursen2015), with six second-order and eighteen third-order sequences delineated by regionally extensive maximum flooding surfaces (Fig. 3). Fluctuations in relative sea level during the Triassic Period likely influenced palynofloral development in the region as coastal habitats were periodically created and lost (Paterson et al. Reference Paterson, Mangerud, Cetean, Mørk, Lord, Klausen and Mørkved2016).

Fig. 2. Lithostratigraphical scheme for the Triassic and Lower–Middle Jurassic strata on Svalbard and in the subsurface Norwegian Barents Shelf. Modified from Mørk et al. (Reference Mørk, Dallman, Dypvik, Johannessen, Larssen, Nagy, Nøttvedt, Olaussen, Pčhelina, Worsley and Dallman1999).

Fig. 3. Spore-pollen zonal schemes for the Lower–Upper Triassic (Induan–Rhaetian) succession of Svalbard and the Barents Shelf: (1) Hochuli et al. (Reference Hochuli, Colin, Vigran and Collinson1989); (2) Vigran et al. (Reference Vigran, Mangerud, Mørk, Worsley and Hochuli2014); (3) this study; and (5) Fefilova (Reference Fefilova2001). (4) Dinoflagellate cyst zones, this study. Timescale after Ogg et al. (Reference Ogg, Ogg and Gradstein2016). Formations from (1) eastern Svalbard and (2) Norwegian Barents Shelf. Second- and third-order stratigraphic sequences from Glørstad-Clark et al. (Reference Glørstad-Clark, Faleide, Lundschien and Nystuen2010) and Klausen et al. (Reference Klausen, Ryseth, Helland-Hansen, Gawthorpe and Laursen2015).
As on the Barents Shelf, the Triassic succession on Svalbard is assigned to the Sassendalen and Kapp Toscana groups (Fig. 2). However, due to diachroneity resulting from the SE–NW progradation of the strata, the boundary between the groups is younger than in the subsurface Barents Sea, and corresponds approximately to the Middle–Upper Triassic (Ladinian–Carnian) boundary. On Svalbard, the groups are subdivided into an alternative series of local formations, which in the eastern parts of the archipelago comprise the Vikinghøgda and Botneheia formations (Sassendalen Group) and the Tschermakfjellet, De Geerdalen, Flatsalen and Svenskøya formations (Kapp Toscana Group) (Fig. 2).
2.b. Previous palynological schemes
The initial palynostratigraphic studies of Triassic strata in the region focused on outcrops of the Kapp Toscana Group on Hopen, Kong Karls Land and central Spitsbergen (e.g. Smith, Reference Smith1974; Smith et al. Reference Smith, Harland and Hughes1975; Bjærke, Reference Bjærke1977; Bjærke & Dypvik, Reference Bjærke and Dypvik1977; Bjærke & Manum, Reference Bjærke and Manum1977). The first of these (Smith, Reference Smith1974) described assemblages from the Iversenfjellet Formation (now De Geerdalen Formation) on Hopen that were tentatively dated as Carnian–Rhaetian. A subsequent study of the island by Smith et al. (Reference Smith, Harland and Hughes1975) and Bjærke & Manum (Reference Bjærke and Manum1977) considered the assemblages as being of Carnian – Early Jurassic and Rhaetian age, respectively, based on the limited independent dating evidence available at that time, and by comparison with the ranges of key taxa from central and southern Europe. However, an early Norian ammonoid fauna (kerri Zone) later described from the Flatsalen Formation (Korčinskaya, Reference Korčinskaya and Semevskij1980) prompted a reconsideration of the age of the palynofloras. The implications of the ammonoid dating were discussed by Smith (Reference Smith1982) who recognized that many typically ‘Rhaetian’ taxa had surprisingly early first occurrences in the Barents Sea region.
Consequently, recent studies in the region have relied less on interregional correlation, seeking instead to establish a regional zonation, calibrated to the global timescale by independent age constraints. The palynozonation of Hochuli et al. (Reference Hochuli, Colin, Vigran and Collinson1989) comprises 15 ‘assemblages’ (named A–P) spanning the Triassic Period (Fig. 3). This includes four Early Triassic assemblages: P (Griesbachian, ? early Griesbachian), O (Dienerian–Griesbachian), N (Smithian) and M (Spathian); five Middle Triassic assemblages: L (early Anisian), K (late Anisian), I (early Ladinian), H (‘middle’ – late Ladinian) and G (late Ladinian, now redated as early Carnian); and six Late Triassic assemblages: F (early Carnian), E and D (‘middle’ Carnian), C (late Carnian), B-1 and B-2 (Norian and Norian–Rhaetian, respectively), and A (Rhaetian).
The palynozonation of Vigran et al. (Reference Vigran, Mangerud, Mørk, Worsley and Hochuli2014) defined 15 formalized composite assemblage zones ranging from the Changhsingian to Rhaetian (Fig. 3). This zonation has particularly high resolution in the uppermost Permian and Lower Triassic strata, where seven ammonoid calibrated zones are defined: the Uvaesporites imperialis (Changhsingian), Reduviasporonites chalastus (uppermost Changhsingian – lower Griesbachian), Proprisporites pocockii (upper Griesbachian), Maculatasporites spp. (Dienerian), Naumovaspora striata (Smithian), Pechorosporites disertus (lower Spathian) and Jerseyiaspora punctispinosa (upper Spathian) zones. The Middle and the Upper Triassic strata are both represented by four zones each: the Anapiculatisporites spiniger (lower Anisian), Triadispora obscura (middle Anisian), Protodiploxypinus decus (upper Anisian) and Echinitosporites iliacoides (Ladinian) zones; and the Aulisporites astigmosus (lower–‘middle’ Carnian), Rhaetogonyaulax spp. (upper Carnian), Limbosporites lundbladii (Norian) and Ricciisporites tuberculatus (Rhaetian) zones. However, despite the improved Lower Triassic resolution, a significant limitation of the zonation is the relatively low Upper Triassic resolution.
In order to increase the Upper Triassic zonal resolution, we outlined a series of palynological assemblages for the Upper Triassic strata on Svalbard and in stratigraphical cores and exploration wells from the southern Barents Sea (Paterson & Mangerud, Reference Paterson and Mangerud2015, Reference Paterson and Mangerud2017; Paterson et al. Reference Paterson, Mangerud, Cetean, Mørk, Lord, Klausen and Mørkved2016, Reference Paterson, Mangerud and Mørk2017, Reference Paterson, Morris and Mangerud2019a, b). These assemblages were originally defined as informal biostratigraphic subdivisions since it was suspected that their temporal and geographical distribution might be facies dependent. However, since comparable assemblages have now been consistently recognized in correlative deposits throughout the region by us and other authors (e.g. Mueller et al. Reference Mueller, Hounslow and Kürschner2016; Rismyhr et al. Reference Simms and Ruffell2018; Smelror et al. Reference Smelror, Larssen, Olaussen, Rømuld and Williams2019), they are now confidently elevated to full zonal status.
3. Revised zonal scheme
The present Anisian–Rhaetian spore-pollen zonation comprises 11 composite assemblage zones (Figs 3, 4a,b), referred to here as ‘zones’. Additionally, two dinoflagellate cyst zones are defined for the late Carnian (Tuvalian) – early Norian (Lacian) ages. Biostratigraphic events used in the recognition of the zones are defined in Table 1. Representative palynomorph taxa for the zones are illustrated in Figures 5–10.


Fig. 4. (a) Anisian–Norian and (b) Norian–Rhaetian biozonal events. Formations from (1) eastern Svalbard and (2) Norwegian Barents Shelf. Timescale after Ogg et al. (Reference Ogg, Ogg and Gradstein2016).
Table 1. Definition of the biostratigraphic event terms


Fig. 5. Palynomorph taxa from the Late Triassic (early Carnian) Semiretisporis hochulii Zone. Palaeontological Museum Oslo (PMO) number followed by England Finder Coordinates. Scale bars = 20 μm. (a) Camarozonosporites rudis, PMO 234.369 B, R48-4; (b) Leschikisporis aduncus, PMO 234.372 D, R40; (c) Dictyophyllidites mortonii, PMO 234.374 A, G52; (d) Semiretisporis hochulii sp. nov., holotype, PMO 234.369 A, K54; (e) Lycopodiacidites rugulatus, PMO 234.373 A, U52-4; (f) Striatella parva, PMO 234.373 C, X49; (g) Kraeuselisporites sp. tetrad, PMO 234.375 A, G52-1; (h) Podosporites amicus, PMO 234.373 B, N39-3; (i) Striatoabieites balmei, PMO 234.372 A, K45-1; (j) Triadispora verrucata, PMO 234.370 B, T45-3; (k) Podosporites vigraniae sp. nov., holotype, PMO 234.374 B, S42-2; (l) Dyupetalum vicentinense, PMO 234.371 C, M49-1; (m) Voltziaceaesporites heteromorpha, PMO 234.371 A, H46-2; (n) Institisporites crispus, PMO 234.371 B, M44-1; (o) Staurosaccites quadrifidus, PMO 234.372 C, Q47-4; and (p) Illinites chitonoides, PMO 234.370 A, S45-3.
The Middle Triassic (Anisian and Ladinian) zonation is essentially unmodified from Vigran et al. (Reference Vigran, Mangerud, Mørk, Worsley and Hochuli2014). However, the early Anisian Anapiculatisporites spiniger Zone is renamed here as the Carnisporites spiniger Zone to reflect the revised taxonomy of Morbey (Reference Morbey1975). Each of the zones is described in the following subsections and correlated with previous palynozonal schemes (Fig. 3). The early Carnian Semiretisporis hochulii and the Podosporites vigraniae zones are named after newly defined taxa, which are described in Section 5 (Systematic palynology) together with a third taxon, Kyrtomisporis moerki sp. nov. The new species are illustrated in Figures 5, 6 and 11. All palynomorph specimens shown in Figures 5–11 are curated in the palaeontological collections of the Natural History Museum, Oslo (except for some of those illustrated in Fig. 10, which belong to Applied Petroleum Technology, Oslo).

Fig. 6. Palynomorph taxa from the Late Triassic (early Carnian) Podosporites vigraniae Zone. Palaeontological Museum Oslo (PMO) number followed by England Finder Coordinates. Scale bars = 20μm. (a) Leschikisporis aduncus, PMO 234.381 A, H56; (b) Kyrtomisporis moerki sp. nov., holotype, PMO 234.376 B, M44-1; (c) Kraeuselisporites ? dentatus, PMO 234.378 B, N45; (d) Lycopodiacidites rugulatus, PMO 234.380 B, G54-2; (e) Kraeuselisporites cooksonae, PMO 234.380 A, E54; (f) Kraeuselisporites cooksonae tetrad, PMO 234.378 A, D56-1; (g) Triadispora plicata, PMO 234.383 F, J56-3; (h) Carnisporites spiniger, PMO 234.383 D, E55-3; (i) Camarozonosporites laevigatus, PMO 234.384 A, J45-2; (j) Triadispora verrucata, PMO 234.380 C, G49-4; (k) Striatoabieites balmei, PMO 234.383 B, C51-2; (l) Schizaeoisporites worsleyi, PMO 234.383 C, D51-2; (m) Illinites chitonoides, PMO 234.377 A, R54-4; (n) Podosporites vigraniae sp. nov., PMO 234.379 A, G47-1; (o) Staurosaccites quadrifidus, PMO 234.383 A, C48-2; and (p) Vitreisporites pallidus, PMO 234.382 A, J45-1.
3.a. Anisian
3.a.1. Carnisporites spiniger Zone
Definition: The base of the Carnisporites spiniger Zone is defined by the consistent first occurrences of Carnisporites spiniger, Illinites chitonoides and Podosporites amicus (Vigran et al. Reference Vigran, Mangerud, Mørk, Worsley and Hochuli2014, p. 70) and the first occurrences of Falcisporites stabilis, Kuglerina meieri and Staurosaccites quadrifidus (Fig. 4a). The base of the zone also coincides with the last occurrence of Crustaesporites globosus.
Biozonal assemblages: The zone is characterized by the common to abundant occurrence of Aratrisporites spp., together with the common occurrence of Jerseyiaspora punctispinosa and various species of Triadispora.
Occurrence: On Svalbard, assemblages assigned to the Carnisporites spiniger Zone have been recorded in the Bravaisberget Formation (Passhatten Member) and in the equivalent Botneheia Formation (Muen Member) (Fig. 2), in association with ammonoids of the lower Anisian Karangites evolutus Zone (Vigran et al. Reference Vigran, Mangerud, Mørk, Worsley and Hochuli2014). The zone is also recorded in the correlative Steinkobbe Formation in shallow stratigraphical core 7323/7-U-4 from the Svalis Dome, and in the Kobbe Formation in several exploration wells from the Hammerfest, Maud and Nordkapp basins (Vigran et al. Reference Vigran, Mangerud, Mørk, Worsley and Hochuli2014; Paterson & Mangerud, Reference Paterson and Mangerud2017).
Biostratigraphic correlation: According to Vigran et al. (Reference Vigran, Mangerud, Mørk, Worsley and Hochuli2014, fig. 3a), the lower part of the Carnisporites spiniger Zone corresponds to the Striatella seebergensis – Accinctisporites circumdatus – Anapiculatisporites spiniger – Pretricolpipollenites spp. Concurrent Range Zone (‘Svalis-5’) of Vigran et al. (Reference Vigran, Mangerud, Mørk, Bugge and Weitschat1998) described from the Steinkobbe Formation on the Svalis Dome. The zone correlates with ‘Assemblage L’ of Hochuli et al. (Reference Hochuli, Colin, Vigran and Collinson1989), and to ‘floral phases 3 and 4’ of Hochuli & Vigran (Reference Hochuli and Vigran2010). Comparable assemblages rich in Aratrisporites spp. have also been recorded from the Anisian ‘T2a palynocomplex’ of the Russian Barents Sea (Pavlov et al. Reference Pavlov, Fefilova and Lodkina1985; Mørk et al. Reference Mørk, Vigran, Korchinskaya, Pchelina, Fefilova, Vavilov and Weitschat1993; Fefilova, Reference Fefilova and Nekhorosheva2013) (Fig. 3).
Age: The zone is dated as early Anisian by the co-occurrence of an ammonoid fauna assigned to the Karangatites evolutus Zone on Svalbard and in shallow stratigraphic core 7323/07-U-04 from the Svalis Dome (Weitschat & Dagys, Reference Weitschat and Dagys1989; Vigran et al. Reference Vigran, Mangerud, Mørk, Bugge and Weitschat1998, Reference Vigran, Mangerud, Mørk, Worsley and Hochuli2014).
3.a.2. Triadispora obscura Zone
Definition: The Triadispora obscura Zone was defined by Vigran et al. (Reference Vigran, Mangerud, Mørk, Worsley and Hochuli2014, p. 71) with the zone base coinciding with the last occurrence of Densoisporites nejburgii, and by the first occurrences of Camarozonosporites laevigatus, Protodiploxypinus minor, P. ornatus and Pseudoenzonalasporites summus. The top of the zone is defined by the last consistent occurrence of Jerseyiaspora punctispinosa, the consistent occurrence of Podosporites amicus and the increased diversity of Triadispora spp. (Fig. 4a).
Biozonal assemblages: The zone is characterized by diverse assemblages with common specimens of Angustisulcites spp., Aratrisporites spp., Illinites chitonoides, Striatoabieites spp. and Triadispora spp. (Vigran et al. Reference Vigran, Mangerud, Mørk, Worsley and Hochuli2014, p. 71).
Occurrence: The zone has been recorded in the Botneheia Formation (Muen Member) from sections on Svalbard (Vigran et al. Reference Vigran, Mangerud, Mørk, Worsley and Hochuli2014). In the Barents Sea, the zone is recognized in stratigraphical cores and exploration wells penetrating the Steinkobbe and Kobbe formations on the Svalis Dome and Sentralbanken High, and in the Hammerfest, Maud and Nordkapp basins (Vigran et al. Reference Vigran, Mangerud, Mørk, Worsley and Hochuli2014; Paterson & Mangerud, Reference Paterson and Mangerud2017).
Biostratigraphic correlation: The Triadispora obscura Zone correlates with the Aratrisporites macrocavatus – Triadispora plicata – Jerseyiaspora punctispinosa – Kraeuselisporites apiculatus Concurrent Range Zone (‘Svalis-6’) of Vigran et al. (Reference Vigran, Mangerud, Mørk, Bugge and Weitschat1998). The zone is also equivalent to the lower part of ‘Assemblage K’ of Hochuli et al. (Reference Hochuli, Colin, Vigran and Collinson1989), and partially equivalent to ‘Floral Phase 4’ of Hochuli & Vigran (Reference Hochuli and Vigran2010). In the Russian Barents Sea, the last occurrence Densoisporites nejburgii occurs in the ‘T2a palynocomplex’ (Mørk et al. Reference Mørk, Vigran, Korchinskaya, Pchelina, Fefilova, Vavilov and Weitschat1993), the upper part of which likely correlates with the Triadispora obscura Zone (Fig. 3).
Age: The zone is dated as middle Anisian by a rich ammonoid fauna belonging to the Anagymnotoceras varium Zone which was recorded in Svalis Dome core 7323/07-U-01 (Vigran et al. Reference Vigran, Mangerud, Mørk, Bugge and Weitschat1998, Reference Vigran, Mangerud, Mørk, Worsley and Hochuli2014, p. 71).
3.a.3. Protodiploxypinus decus Zone
Definition: Vigran et al. (Reference Vigran, Mangerud, Mørk, Worsley and Hochuli2014, p. 71) defines the base of the Protodiploxypinus decus Zone on the first common occurrence of Chasmatosporites spp. and Illinites chitonoides (Fig. 4a). Other taxa with first occurrences include Camarozonosporites rudis and Retisulcites perforatus. According to range data presented by Vigran et al. (Reference Vigran, Mangerud, Mørk, Worsley and Hochuli2014), the upper limit of the zone is defined by the last occurrences of Densoisporites playfordii, Falcisporites stabilis, Kraeuselisporites apiculatus, Jerseyiaspora punctispinosa, Protodiploxypinus decus and P. ornatus.
Biozonal assemblages: The zone is characterized by abundant striate pollen, including Striatoabieites spp. and Lunatisporites spp., together with non-striate pollen such as Illinites chitonoides (Vigran et al. Reference Vigran, Mangerud, Mørk, Worsley and Hochuli2014, p. 71). Aratrisporites spp. remains a common to abundant element of the zone. Marine palynomorphs are also relatively common, as is the fresh- to brackish-water alga Plaesiodictyon mosellanum (Vigran et al. Reference Vigran, Mangerud, Mørk, Worsley and Hochuli2014, p. 71).
Occurrence: The zone has been recorded in the Bravaisberget (Passhatten Member) and correlative Botneheia (Muen Member) formations on Svalbard (Vigran et al. Reference Vigran, Mangerud, Mørk, Worsley and Hochuli2014). It is also observed in core material from the Steinkobbe Formation on the Svalis Dome, and in the upper Klappmyss and Kobbe formations in the Hammerfest, Maud and Nordkapp basins and on the Sentralbanken High (Vigran et al. Reference Vigran, Mangerud, Mørk, Worsley and Hochuli2014; Paterson & Mangerud, Reference Paterson and Mangerud2017).
Comments: While Vigran et al. (Reference Vigran, Mangerud, Mørk, Worsley and Hochuli2014) defined the top of the zone by the last occurrence of Kraeuselisporites apiculatus, Jerseyiaspora punctispinosa, Protodiploxypinus decus and P. ornatus, subsequent studies by the current authors have observed these taxa to have considerably longer stratigraphic ranges (Paterson & Mangerud, Reference Paterson and Mangerud2017; Paterson et al. Reference Paterson, Mangerud and Mørk2017, Reference Paterson, Morris and Mangerud2019a). However, the possibility that these taxa are reworked into younger deposits cannot be excluded.
Biostratigraphic correlation: The Protodiploxypinus decus Zone was defined by Vigran et al. (Reference Vigran, Mangerud, Mørk, Worsley and Hochuli2014, p. 71) from the Steinkobbe Formation in Svalis Dome cores 7323/7-U-7 and 7323/7-U-9. The zone incorporates the Protodiploxypinus decus – P. gracilis – Chasmatosporites sp. A – Kraeuselisporites apiculatus Concurrent Range Zone (‘Svalis-7’ of Vigran et al. Reference Vigran, Mangerud, Mørk, Bugge and Weitschat1998). The Protodiploxypinus decus Zone is also correlative of ‘floral phases 5–7’ of Hochuli & Vigran (Reference Hochuli and Vigran2010). The zone likely correlates with the ‘T2a–l palynocomplex’ of the Russian Barents Sea (Fig. 3).
Age: The zone is dated as late Anisian based on an abundant ammonoid fauna comprising Frechites sp. and Parapopanoceras sp., recorded in Svalis Dome core 7323/07-U-09, and assigned to the Frechites laqueatus Zone (Vigran et al. Reference Vigran, Mangerud, Mørk, Bugge and Weitschat1998, Reference Vigran, Mangerud, Mørk, Worsley and Hochuli2014, p. 71). However, Rhenium–Osmium (Re-Os) geochronology from Svalbard and the Svalis Dome more broadly constrains the age as late Anisian – Ladinian (Xu et al. Reference Xu, Hannah, Stein, Bingen, Yang, Zimmermann, Weitschat, Mørk and Weiss2009).
3.b. Ladinian
3.b.1. Echinitosporites iliacoides Zone
Definition: The Echinitosporites iliacoides Zone was defined by Vigran et al. (Reference Vigran, Mangerud, Mørk, Worsley and Hochuli2014, p. 72) based on the first occurrence of Echinitosporites iliacoides. According to our observations and range data presented by Vigran et al. (Reference Vigran, Mangerud, Mørk, Worsley and Hochuli2014), other taxa with first occurrences at the base of the zone include Annulispora folliculosa, Institisporites crispus, Lagenella martini, Lycopodiacidites rugulatus, Rogalskaisporites cicatricosus, Thomsonisporites toralis, T. undulatus, Zebrasporites laevigatus and Z. interscriptus. The top of the zone is defined by the last common occurrence of Echinitosporites iliacoides and Lagenella martini.
Biozonal assemblages: The zone is characterized by a diverse taxonomic association dominated by bisaccate pollen, including the common occurrence of non-taeniate pollen (Vigran et al. Reference Vigran, Mangerud, Mørk, Worsley and Hochuli2014, p. 72). The zone also contains abundant and diverse Aratrisporites spp.
Occurrence: On Svalbard, the Echinitosporites iliacoides Zone has been recorded in samples from the Bravaisberget Formation (Somovbreen and Van Keulenfjorden members) and the correlative upper Muen – Blanknuten members of the Botneheia Formation equivalent (Vigran et al. Reference Vigran, Mangerud, Mørk, Worsley and Hochuli2014). In the Barents Sea, the zone is recorded in the subsurface Botneheia Formation east of Kong Karls Land, in the upper Kobbe Formation on the Bjarmeland Platform, Loppa High and Nordkapp Basin, and in the lower Snadd Formation on the Loppa High and in the Maud Basin (Vigran et al. Reference Vigran, Mangerud, Mørk, Worsley and Hochuli2014; Paterson & Mangerud Reference Paterson and Mangerud2017).
Comments: According to Vigran et al. (Reference Vigran, Mangerud, Mørk, Worsley and Hochuli2014, p. 72) the taxa Staurosaccites quadrifidus and Triadispora verrucata have their first occurrences in the lower part of the zone. However, the present authors have observed rare occurrences beginning in the lower Anisian Kobbe Formation in the Nordkapp Basin (Paterson & Mangerud Reference Paterson and Mangerud2017; Rossi et al. Reference Rossi, Paterson, Helland-Hansen, Klausen and Eide2019). Despite being a marker for the base of the zone, the taxon A. astigmosus has only ever been observed to be a sporadic element among our Carnian assemblages. This issue was highlighted in Paterson et al. (Reference Paterson, Mangerud and Mørk2017), who suggested that the taxon Podosporites cf. amicus (now P. vigraniae sp. nov.) may have been misidentified as A. astigmosus by previous authors, leading to an overestimation of A. astigmosus in the Carnian strata of the Barents Sea region. This speculation was based on the similarity between the specimen of A. astigmosus illustrated by Klaus (Reference Klaus1960, pl. 28, 2) and specimens of P. vigraniae with weakly developed sacci observed in our material. Since this matter remains unresolved, in this paper we define the top of the Echinitosporites iliacoides Zone by the base of the Semiretisporis hochulii Zone, which is the oldest of our subdivisions of the former Aulisporites astigmosus Zone.
Biostratigraphic correlation: The Echinitosporites iliacoides Zone correlates with assemblages ‘H and I’ of Hochuli et al. (Reference Hochuli, Colin, Vigran and Collinson1989), and incorporates the Ovalipollis pseudoalatus – Echinitosporites iliacoides – Cordaitina gunyalensis Concurrent Range Zone of Vigran et al. (Reference Vigran, Mangerud, Mørk, Bugge and Weitschat1998). The zone likely corresponds, at least in part, to the ‘T2l palynocomplex’ of the Russian Barents Sea. However, in contrast to the Norwegian Barents Shelf, the highest abundance of Echinitosporites iliacoides there is recorded in the slightly younger ‘T2–T3 palynocomplex’ (Fefilova, Reference Fefilova and Gramberg1988; Mørk et al. Reference Mørk, Vigran, Korchinskaya, Pchelina, Fefilova, Vavilov and Weitschat1993).
Age: The Echinitosporites iliacoides Zone is dated as Ladinian based on the sparse occurrence of bivalves assigned to Aparimella spp. (Vigran et al. Reference Vigran, Mangerud, Mørk, Bugge and Weitschat1998, p. 118).
3.c. Carnian
3.c.1. Semiretisporis hochulii Zone
Definition: The Semiretisporis hochulii Zone was first described from the lowermost Snadd Formation in the Nordkapp Basin (Paterson & Mangerud Reference Paterson and Mangerud2017) and from the Tschermakfjellet Formation in the Sentralbanken area (Paterson et al. Reference Paterson, Mangerud, Holen, Landa, Lundschien and Eide2019a). The base of the zone is defined by the first common occurrence of Semiretisporis hochulii sp. nov., and the first occurrences of Aratrisporites laevigatus, Patinasporites densus and Vallasporites ignacii (Fig. 4a). The top of the zone is marked by the last common occurrences of Dyupetalum vicentinense, Patinasporites summus and S. hochulii sp. nov.
Biozonal assemblages: Characteristic taxa include Aratrisporites fischeri, Araucariacites australis, Calamospora tener, Conbaculatisporites spp., Deltoidospora spp., Echinitosporites iliacoides (rare/ sporadic), Illinites chitonoides, Leschikisporis aduncus, Podosporites vigraniae sp. nov., Semiretisporis hochulii sp. nov., Striatella seebergensis, Striatoabieites balmei, S. multistriatus and Triadispora verrucata. A representative association of species present in the zone are illustrated in Figure 5.
Occurrence: The Semiretisporis Zone was first recorded informally as ‘assemblage SBS-VII’ from the basal Snadd Formation in Nordkapp Basin wells 7228/2-1s and 7228/9-1s (Paterson & Mangerud Reference Paterson and Mangerud2017). Comparable assemblages were subsequently recorded as ‘assemblage SBH-I’ in preparations from the ‘Tschermakfjellet’ and Snadd formations in shallow stratigraphical cores 7533/3-U-7 and 7534/4-U-1 from the Sentralbanken High area (Paterson et al. Reference Paterson, Mangerud, Holen, Landa, Lundschien and Eide2019a). The zone is given formal status here.
Biostratigraphic correlation: The Semiretisporis hochulii Zone is likely equivalent to ‘Assemblage G’ of Hochuli et al. (Reference Hochuli, Colin, Vigran and Collinson1989, p. 143) and the lowermost part of the Aulisporites astigmosus Zone of Vigran et al. (Reference Vigran, Mangerud, Mørk, Worsley and Hochuli2014, p. 73) (Fig. 3). The common occurrence of Semiretisporis is a feature noted in the lower De Geerdalen Formation on central Spitsbergen by Mueller et al. (Reference Mueller, Hounslow and Kürschner2016). The Semiretisporis hochulii Zone likely corresponds to the low-productivity assemblages with consistent Semiretisporis ‘barentsi’ (=S. hochulii sp. nov.) tentatively assigned by van Veen (Reference van Veen1985) to his informal ‘palynozone VIII’ from well 7120/9-2. At the time, van Veen (Reference van Veen1985) noted that the assemblages were restricted to this one well; however, recovery of comparable assemblages on Spitsbergen (Mueller et al. Reference Mueller, Hounslow and Kürschner2016) and elsewhere in the Barents Sea (Paterson & Mangerud Reference Paterson and Mangerud2017; Paterson et al. Reference Paterson, Mangerud, Holen, Landa, Lundschien and Eide2019a) suggests it has a regional distribution.
The Semiretisporis hochulii Zone likely corresponds to the transitional ‘T2–T3 palynocomplex’ of the Russian Barents Sea (Fig. 3) based on its relative stratigraphic position and the composition of over- and underlying zones. However, as noted by Mørk et al. (Reference Mørk, Vigran, Korchinskaya, Pchelina, Fefilova, Vavilov and Weitschat1993) the latter zone contains abundant Echinitosporites iliacoides, which is comparatively rare in age-equivalent assemblages in the Norwegian Arctic. This disparity may be the result of reworking, or due to the parent plant taxon possibly having a longer range in the more proximal areas to the east.
Age: The Semiretisporis hochulii Zone is dated as earliest Carnian (Julian) based on the last consistent occurrence of Echinitosporites iliacoides, which on Bjørnøya (Mørk et al. Reference Mørk, Vigran and Hochuli1990) occurs in strata containing the ammonoid Daxatina cf. canadensis (e.g. Vigran et al. Reference Vigran, Mangerud, Mørk, Worsley and Hochuli2014, p. 72–73). Re-Os geochronology of the underlying Echinitosporites iliacoides Zone in core material from offshore Kong Karls Land (Xu et al. Reference Xu, Hannah, Stein, Mørk, Vigran, Bingen, Schutt and Lundschien2014) provides an age of 236.6 ± 0.4 Ma, which dates the base of Semiretisporis hochulii Zone as early Carnian.
3.c.2. Podosporites vigraniae Zone
Definition: The Podosporites vigraniae Zone was originally described as the ‘Podosporites cf. amicus Assemblage’ from the Snadd Formation in the Kong Karls Land area by Paterson et al. (Reference Paterson, Mangerud, Cetean, Mørk, Lord, Klausen and Mørkved2016). It is elevated to full zonal status here. The zone is characterized by a diverse taxonomic association containing common to abundant specimens of Podosporites vigraniae sp. nov., without common Semiretisporis hochulii sp. nov. or dominant Leschikisporis aduncus, which typify assemblages from the underlying and overlying zones, respectively (Fig. 4a). The top of the zone is defined by the last common occurrence of P. vigraniae, the last occurrences of Cordaitina gunyalensis, Institisporites crispus and Retisulcites perforatus, and the first occurrence of dominant Leschikisporis aduncus, marking the base of the Leschikisporis aduncus Zone.
Biozonal assemblages: The zone contains a highly diverse palynological assemblage characterized by an association of fern, bryophyte, lycopsid and sphenopsid spores, together with conifer, cycad-ginkgophyte and pteridosperm pollen. A representative selection of species present in the zone is illustrated in Figure 6. Spore taxa recorded include Annulispora folliculosa, Aratrisporites laevigatus, A. paenulatus, Calamospora tener, Camarozonosporites rudis, Carnisporites spiniger, Conbaculatisporites spp., Deltoidospora spp., Densosporites fissus, Dictyophyllidites mortonii, Kraeuselisporites cooksonae, Kyrtomisporis moerki sp. nov., Leschikisporis aduncus, Limatulasporites limatulus, Lycopodiacidites rugulatus, Lycopodiumsporites semimuris, Neoraistrickia spp., Reticulatisporites sp., Semiretisporis hochulii sp. nov. (sporadic), Striatella parva, S. seebergensis, Thomsonisporites toralis, Tigrisporites halleinis and Zebrasporites spp.
Pollen taxa present in the assemblage include Alisporitesspp., Angustisulcites klausii, Araucariacites australis, Aulisporites astigmosus, Brachysaccus spp., Camerosporites secatus, Chasmatosporites spp., Chordasporites singulichorda, Dyupetalum vicentinense, Enzonalasporites vigens, Eucommiidites intrareticulatus, Illinites chitonoides, Infernopollenites sp., Institisporites crispus, Kuglerina meieri, Lunatisporites acutus, L. noviaulensis, Ovalipollis ovalis, Partitisporites sp., Patinasporites densus, Podosporites amicus, P. vigraniae sp. nov., Protodiploxypinus spp., Pseudoenzonalasporites summus, Pseudoquadrisaccus irregularis, Retisulcites spp., Schizaeoisporites worsleyi, Striatoabieites spp., Staurosaccites quadrifidus, Triadispora spp. and Vitreisporites pallidus.
Occurrence: The Podosporites vigraniae Zone has been observed in the ‘Tschermakfjellet’ and Snadd formations from offshore Kong Karls Land (Paterson et al. Reference Paterson, Mangerud and Mørk2017). The zone has also been recorded in the Snadd Formation in Hammerfest Basin well 7120/12-2 (2425–2645 m), and in Nordkapp Basin wells 7228/2-1s (1911–2215 m) and 7228/9-1s (1373.5–1557 m) (Paterson & Mangerud Reference Paterson and Mangerud2017).
Biostratigraphic correlation: The Podosporites vigraniae Zone correlates with ‘Assemblage F’ of Hochuli et al. (Reference Hochuli, Colin, Vigran and Collinson1989) and the lower part of the Aulisporites astigmosus Zone of Vigran et al. (Reference Vigran, Mangerud, Mørk, Worsley and Hochuli2014). The zone probably corresponds to the undifferentiated Carnian ‘T2k palynocomplex’ of the Russian Barents Sea, which also contains abundant Leschikisporis aduncus and Podosporites amicus (Mørk et al. Reference Mørk, Vigran, Korchinskaya, Pchelina, Fefilova, Vavilov and Weitschat1993; Fefilova, Reference Fefilova and Nekhorosheva2013) (Fig. 3).
Age: Re-Os geochronology of the underlying Echinitosporites iliacoides Zone in core material from offshore Kong Karls Land (Xu et al. Reference Xu, Hannah, Stein, Mørk, Vigran, Bingen, Schutt and Lundschien2014) provides an age of 236.6 ± 0.4 Ma, which constrains the ages of the Semiretisporis hochulii and Podosporites vigraniae zones as no older than early Carnian (Paterson et al. Reference Paterson, Mangerud and Mørk2017). Furthermore, dating of the succeeding Leschikisporis aduncus Zone on Hopen indicates that the top of the Podosporites vigraniae is no younger than late Carnian (Tuvalian 2) (Lord et al. Reference Lord, Solvi, Ask, Mørk, Hounslow and Paterson2014; Paterson & Mangerud, Reference Paterson and Mangerud2015).
3.c.3. Leschikisporis aduncus Zone
Definition: The Leschikisporis aduncus Zone was described as the ‘Leschikisporis aduncus Assemblage’ in preparations from the lower exposures of the De Geerdalen Formation (= middle De Geerdalen Formation) on Hopen by Paterson & Mangerud (Reference Paterson and Mangerud2015). It is elevated to full zonal status here. The base of the zone is defined by the first occurrence of dominant Leschikisporis aduncus, typically in association with Apiculatasporites hirsutus, A. lativerrucosus, Apiculatisporis parvispinosus, Calamospora tener and Clathroidites papulosus (Fig. 4a). The zone is characterized by a low-diversity taxonomic association comprising mainly palynomorphs of hygrophytic affinity such as bryophyte, fern, lycopsid and sphenopsid spores (Fig. 7) (see Paterson et al. Reference Paterson, Mangerud, Cetean, Mørk, Lord, Klausen and Mørkved2016, table 1: Paterson et al. Reference Paterson, Mangerud and Mørk2017, table 4). The top of the zone is defined by the base of the overlying Protodiploxypinus spp. Zone and the last occurrence of dominant L. aduncus.
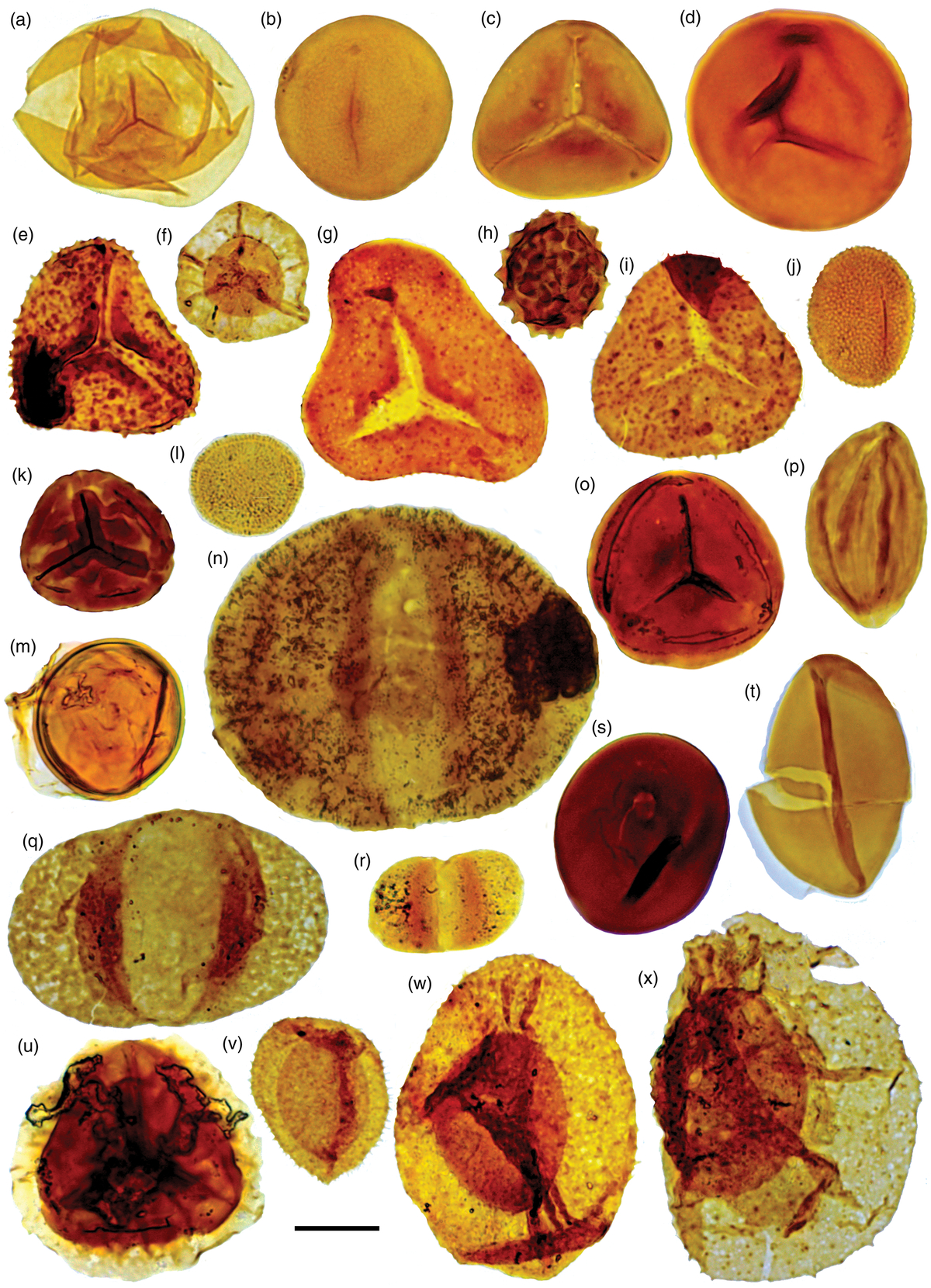
Fig. 7. Palynomorph taxa from the Late Triassic (late Carnian) Leschikisporis aduncus Zones. Palaeontological Museum Oslo (PMO) number followed by England Finder Coordinates. Scale bars = 20μm. (a) Calamospora tener, PMO 234.391 A, C44-4; (b) Leschikisporis aduncus, PMO 234.391 D, J44-4; (c) Deltoidospora sp., PMO 234.391 B, C47; (d) Punctatisporites fungosus, PMO 234.387 D, W59; (e) Clathroidites papulosus, PMO 234.387 A, K40; (f) Thomsonisporites toralis, PMO 234.394 A, N64; (g) Concavisporites scabratus, PMO 234.387 C, U54-4; (h) Anapiculatisporites lativerrucosus, PMO 234.392 C, H60-4; (i) Conbaculatisporites hopensis, PMO 234.388 A, M50-1; (j) Apiculatisporis parvispinosus, PMO 234.391 C, C55-2; (k) Camarozonosporites rudis, PMO 234.392 D, J44-2; (l) Vallasporites ignacii, PMO 234.391 E, K41; (m) Cymatiosphaera sp. 1 sensu Hochuli et al. (Reference Hochuli, Colin, Vigran and Collinson1989), PMO 234.390 A, K45-1; (n) Illinites chitonoides, PMO 234.392 F, M61-1; (o) Camarozonosporites laevigatus, PMO 234.393 A, D61-4; (p) Ephedripites steevesii, PMO 234.392 B, G61; (q) Alisporites sp., PMO 234.387 B, T44; (r) Vitreisporites pallidus, PMO 234.394 B, O65; (s) Aulisporites canalis, PMO 234.389 B, E39-4; (t) Aratrisporites laevigatus, PMO 234.395 A, U50-4; (u) Zebrasporites kahleri, PMO 234.393 B, Y64-1; (v) Aratrisporites scabratus, PMO 234.387 E, X49-3; (w) Aratrisporites macrocavatus, PMO 234.389 A, D52; and (x) Aratrisporites fischeri, PMO 234.392 A, F47-4.
Biozonal assemblages: The zone is typified by an association of spore taxa including Annulispora folliculosa, Apiculatasporites hirsutus, A. lativerrucosus, Apiculatisporis parvispinosus, Aratrisporites fischeri, A. granulatus, A. scabratus, Calamospora tener, Camarozonosporites rudis, Clathroidites papulosus, Conbaculatisporites hopensis, Deltoidospora spp., Dictyophyllidites mortonii, Kraeuselisporites cooksonae, Porcellispora longdonensis, Striatella parva, Zebrasporites interscriptus and Z. laevigatus. Pollen grains comprise a relatively minor component of this assemblage: taxa include Araucariacites australis, Aulisporites astigmosus, Chasmatosporites spp., Cycadopites spp., Eucommiidites sp., Illinites chitonoides, Ovalipollis ovalis, Steevesipollenites sp., Triadispora verrucata and Vesicaspora fuscus.
Occurrence: The Leschikisporis aduncus Zone has been observed in preparations from the De Geerdalen (below the Hopen Member) on Hopen (Paterson & Mangerud, Reference Paterson and Mangerud2015), Spitsbergen (Rismyhr et al. Reference Simms and Ruffell2018) and in shallow stratigraphical cores from the Sentralbanken High (Paterson et al. Reference Mangerud, Paterson and Riding2018). The zone has also been recorded in the middle part of the Snadd Formation in Hammerfest Basin 7228/2-1s (1766.5–1891 m) and 7228/9-1s (sample 1358 m) (Paterson & Mangerud Reference Paterson and Mangerud2017).
Biostratigraphic correlation: The Leschikisporis aduncus Zone corresponds to the informal ‘middle’ Carnian ‘assemblages D and E’ of Hochuli et al. (Reference Hochuli, Colin, Vigran and Collinson1989), ‘Floral Phase 12’ (Hochuli & Vigran, Reference Hochuli and Vigran2010, Fig. 2) and to the upper part of the Aulisporites astigmosus Zone of Vigran et al. (Reference Vigran, Mangerud, Mørk, Worsley and Hochuli2014). On Hopen, the L. aduncus Zone correlates with an interval rich in lycopsid megaspores, recently defined as the Dijkstraisporites beutleri Zone (Paterson et al. Reference Paterson, Morris and Mangerud2019b). Together with the preceding Podosporites vigraniae Zone, the Leschikisporis aduncus Zone likely correlates with the undifferentiated Carnian ‘T2k palynocomplex’ of the Russian Barents Sea (Mørk et al. Reference Mørk, Vigran, Korchinskaya, Pchelina, Fefilova, Vavilov and Weitschat1993; Fefilova, Reference Fefilova and Nekhorosheva2013) (Fig. 3).
Age: The zone is dated as late Carnian (Tuvalian 2–3) based on magnetostratigraphy (Lord et al. Reference Lord, Solvi, Ask, Mørk, Hounslow and Paterson2014; Paterson & Mangerud, Reference Paterson and Mangerud2015), and constrained with ammonoid data from the overlying Flatsalen Formation (Korčinskaya, Reference Korčinskaya and Semevskij1980; Smith, Reference Smith1982).
3.c.4. Protodiploxypinus spp. Zone
Definition: The Protodiploxypinus spp. Zone was originally defined from the upper De Geerdalen Formation (Hopen Member) as the ‘Protodiploxypinus spp. Assemblage’ by Paterson & Mangerud (Reference Paterson and Mangerud2015). It is elevated to full zonal status here. The base of the zone is marked by an increased relative abundance of Protodiploxypinus spp., together with the first occurrence of several distinctive spore taxa including Cingulizonates rhaeticus, Kyrtomisporis gracilis, K. laevigatus, K. speciosus, Limbosporites lundbladiae, Retitriletes austroclavatidites and Velosporites cavatus (Fig. 4a). On Hopen, the Protodiploxypinus spp. Zone is also rich in fresh- to brackish-water algae, particularly Plaesiodictyon mosellanum. The top of the zone is defined by the decreased abundance of Protodiploxypinus spp., and the first common occurrence of Classopollis torosus, which delineates the base of the overlying Classopollis torosus Zone.
The assemblage is distinguished from the Classopollis torosus Zone by the high abundance of Protodiploxypinus spp. and Plaesiodictyon mosellanum, and the absence of Classopollis spp. The assemblage is distinguished from the underlying Leschikisporis aduncus Zone based on the relatively low abundance or absence of Leschikisporis aduncus.
Biozonal assemblages: Araucariacites australis, Chasmatosporites apertus, C. hians, Cingulizonates rhaeticus, Concavisporites crassexinus, Cycadopites spp., Eucommiidites spp., Illinites chitonoides, Kyrtomisporis gracilis, K. laevigatus, K. speciosus, Limbosporites lundbladiae (rare), Ovalipollis ovalis, Plaesiodictyon mosellanum, Protodiploxypinus spp., Quadraeculina anellaeformis (rare), Retitriletes austroclavatidites, Ricciisporites umbonatus, Triadispora verrucata, Velosporites cavatus, Vesicaspora fuscus, Zebrasporites interscriptus and Z. laevigatus. A representative selection of taxa from the zone is illustrated in Fig. 8.

Fig. 8. Palynomorph taxa from the Late Triassic (late Carnian) Protodiploxypinus spp. Zone. Palaeontological Museum Oslo (PMO) number followed by England Finder Coordinates. Scale bars = 20μm. (a) Velosporites cavatus, PMO 234.399 A, M37-2; (b) Kyrtomisporis laevigatus, PMO 234.403 A, X32; (c) Protodiploxypinus minor, PMO 234.398 C, O58; (d) Protodiploxypinus decus, PMO 234.405 A, D58-1; (e) Striatella parva, PMO 234.403 B, P31-4; (f) Kyrtomisporis speciosus, PMO 234.401 C, T33-4; (g) Concavisporites crassexinus, PMO 234.405 F, S60; (h) Retitriletes austroclavatidites, PMO 234.405 C, H40-4; (i) Concavisporites hopensis, PMO 234.405 B, H38-4; (j) Anapiculatisporites lativerrucosus, PMO 234.399 B, M44; (k) Dictyophyllidites mortonii, PMO 234.401 D, W33-4; (l) Striatella seebergensis PMO 234.405 D, N32-2; (m) Aratrisporites laevigatus, PMO 234.405 E, N55-3; (n) Annulispora folliculosa, PMO 234.400 A, C34-2; (o) Alisporites sp., PMO 234.398 D, Y56-1; (p) Araucariacites australis, PMO 234.398 E, Y50; (q) Plaesiodictyon mosellanum, PMO 234.402 A, O56-2; (r) Chasmatosporites hians, PMO 234.398 B, H36-1; (s) Cingulizonates rhaeticus, PMO 234.401 A, S29-3; (t) Chasmatosporites apertus, PMO 234.398 A, G47-4; and (u) Botryococcus sp., PMO 234.401 B, T30-1.
Occurrence: The Protodiploxypinus spp. Zone has been recorded in preparations from the uppermost De Geerdalen Formation (Hopen Member) on Hopen (Paterson & Mangerud, Reference Paterson and Mangerud2015), Spitsbergen (Rismyhr et al. Reference Simms and Ruffell2018) and in cores from the Sentralbanken High (Paterson et al. Reference Mangerud, Paterson and Riding2018). The zone has also been observed in Nordkapp Basin wells 7228/2-1s and 7228/9-1s (Paterson & Mangerud Reference Paterson and Mangerud2017).
Biostratigraphic correlation: The Protodiploxypinus spp. Zone is equivalent to the late Carnian ‘Assemblage C’ (Hochuli et al. Reference Hochuli, Colin, Vigran and Collinson1989), ‘Floral Phase 15’ (Hochuli & Vigran, Reference Hochuli and Vigran2010, Fig. 2), the Rhaetogonyaulax spp. Zone (Vigran et al. Reference Vigran, Mangerud, Mørk, Worsley and Hochuli2014) and the Protodiploxypinus ornatus Composite Assemblage Zone (Rismyhr et al. Reference Simms and Ruffell2018), all of which are characterized by the increased relative abundance of Protodiploxypinus spp. The Protodiploxypinus spp. Zone probably correlates with the upper part of the undifferentiated Carnian ‘T2k palynocomplex’ of the Russian Barents Sea (Fig. 3). However, to the best of our knowledge, an increased abundance of Protodiploxypinus in the upper Carnian Russian deposits has not been recognized.
Age: The zone is dated as late Carnian (Tuvalian 3) based on magnetostratigraphic data from the De Geerdalen Formation on Hopen (Lord et al. Reference Lord, Solvi, Ask, Mørk, Hounslow and Paterson2014; Paterson & Mangerud, Reference Paterson and Mangerud2015), which is further constrained by an early Norian ammonoid fauna from the overlying Flatsalen Formation (Korčinskaya, Reference Korčinskaya and Semevskij1980; Smith, Reference Smith1982).
3.d. Norian
3.d.1. Classopollis torosus Zone
Definition: The Classopollis torosus Zone was first described as the ‘Classopollis torosus Assemblage’ by Paterson & Mangerud (Reference Paterson and Mangerud2015) based on assemblages from the lowermost Flatsalen Formation on Hopen. It is elevated to full zonal status here. The zone is defined by the first common occurrence of the pollen taxa Classopollis torosus (Fig. 4a, b). On Hopen, the zone is also characterized by the presence of microforaminiferal test-linings and the fresh- to brackish-water alga Plaesiodictyon mosellanum. Paterson & Mangerud (Reference Paterson and Mangerud2015, fig. 13) suggested that the first occurrences of Cavatoretisporites obviusand Retitriletes austroclavatidites occurred within the zone; however, these taxa have been subsequently documented within the preceding Protodiploxypinus spp. Zone in the Sentralbanken area (e.g. Paterson et al. Reference Paterson, Mangerud, Holen, Landa, Lundschien and Eide2019a). Based on a compilation of available published data, the zone also contains the last occurrences of several long-ranging taxa including Cordaitina minor, Illinites chitonoides, Kraeuselisporites cooksonae, Patinasporites densus, Staurosaccites quadrifidus, Triadispora verrucata, Voltziaceaesporites heteromorpha and Vallasporites ignacii.
Biozonal assemblages: Annulispora folliculosa, Cavatoretisporites obvius, Chasmatosporites apertus, C. hians, Cingulizonates rhaeticus, Classopollis torosus, Clathroidites papulosus, Deltoidospora spp., Kyrtomisporis gracilis, K. laevigatus, K. speciosus, Limbosporites lundbladiae (rare/sporadic), Polycingulatisporites bicollateralis, Protodiploxypinus spp., Quadraeculina anellaeformis (rare/sporadic), Retitriletes austroclavatidites, Rogalskaisporites cicatricosus, Zebrasporites interscriptus and Z. laevigatus. A representative selection of taxa from the Classopollis torosus zone is illustrated in Figure 9.

Fig. 9. Palynomorph taxa from the Late Triassic (latest Carnian – early Norian) Classopollis torosus, Rhaetogonyaulax arctica and R. rhaetica zones. Palaeontological Museum Oslo (PMO) number followed by England Finder Coordinates. Scale bars = 20μm. (a) Dictyophyllidites mortonii, PMO 234.410 B, D45-4; (b) Concavisporites crassexinus, PMO 234.386 D, S36-4; (c) Annulispora folliculosa, PMO 234.404 A, Q59-1; (d) Zebrasporites interscriptus, PMO 234.414 A, C41-4; (e) Polycingulatisporites bicollateralis, PMO 234.414 E, O50; (f) Camarozonosporites laevigatus, PMO 234.413 A, Y55; (g) Kyrtomisporis speciosus, PMO 234.412 A, K53; (h) Velosporites cavatus, PMO 234.414 F, O32-2; (i) Retitriletes semimuris, PMO 234.414 B, C54; (j) Araucariacites australis, PMO 234.414 C, K33; (k) Quadraeculina anellaeformis, PMO 234.411 E, N42-1; (l) Classopollis torosus tetrad, PMO 234.386 C, N47-2; (m) Classopollis torosus, PMO 234.386 A, D34-3; (n) Vesicaspora fuscus, PMO 234.410 C, F36-3; (o) Retitriletes austroclavatidites, PMO 234.386 E, S39; (p) Kraeuselisporites reissingerii, PMO 234.386 B, K55-2; (q) Carnisporites spiniger, PMO 234.410 E, G51-3; (r) Alisporites sp., PMO 234.410 D, F37-4; (s) Triadispora boelchii, PMO 234.410 F, M50; (t) Baltisphaeridium sp., PMO 234.411 D, J49-4; (u) Pterospermella sp., PMO 234.414 D, L33; (v) Crassosphaera sp., PMO 234.410 G, O37-1; (x) Rhaetogonyaulax rhaetica, PMO 234.411 F, R58-2; (y) Lophotriletes novicus, PMO 234.411 B, C42; (z) microforaminiferal test-lining, PMO 234.410 A, B46-4; (aa) Veryhachium sp., PMO 234.411 A, C34; (ab) scolecodont gen. et. sp. indet., PMO 234.411 C, E60-4; and (ac) ?Cymatiosphaera sp., PMO 234.412 B, L53-2.
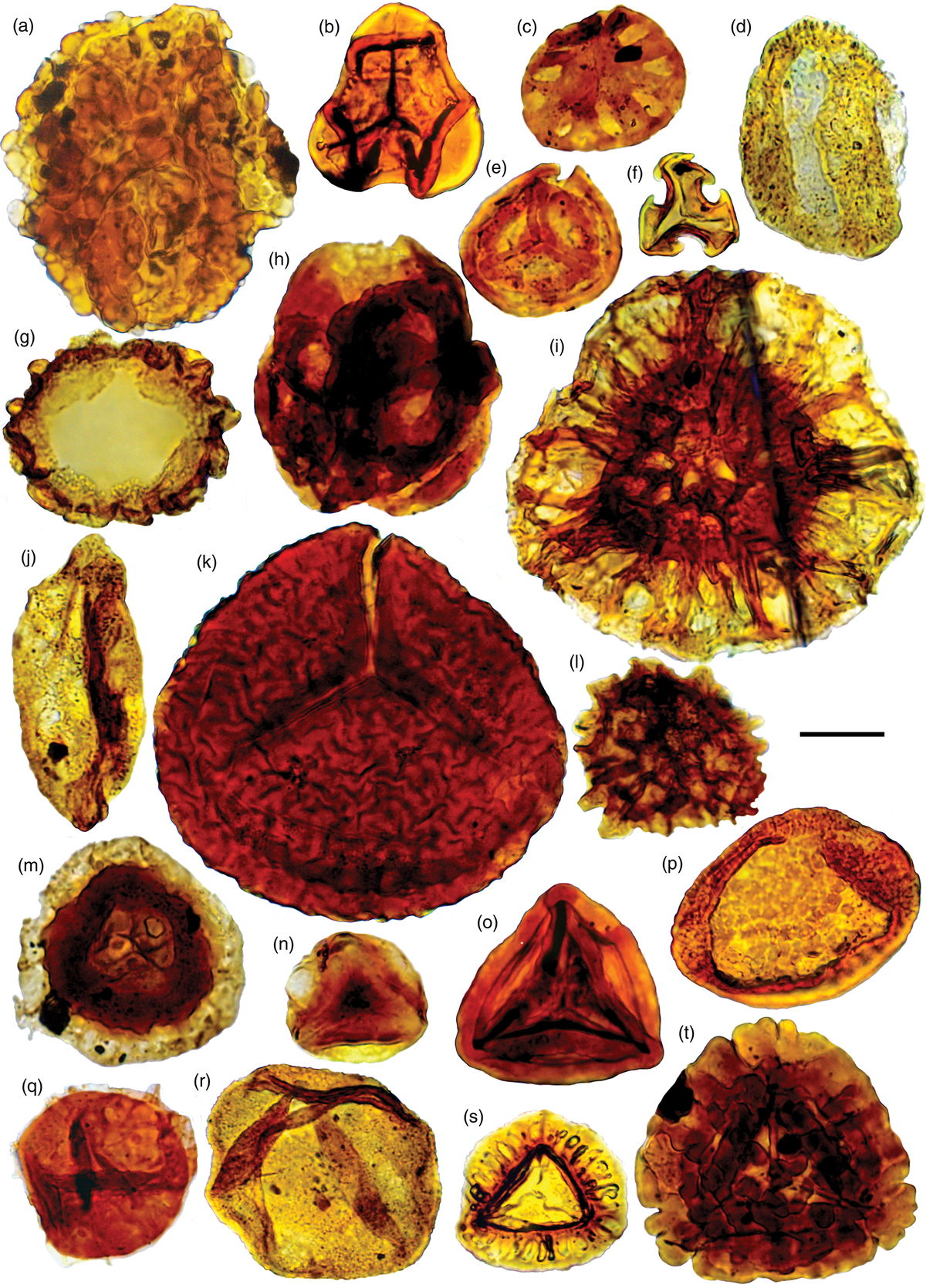
Fig. 10. Palynomorph taxa from the Late Triassic (Norian–Rhaetian) Quadraeculina anellaeformis and Ricciisporites spp. zones. Palaeontological Museum Oslo (PMO) number followed by England Finder Coordinates. Slides from well 7228/7-1A belong to Applied Petroleum Technology, Oslo. Scale bars = 20μm. (a) Ricciisporites tuberculatus, well 7228/7-1A 1506 m, F49-3; (b) Cibotiumspora jurienensis, well 7228/7-1A 1548 m, E44-2; (c) Rogalskaisporites ambientis, PMO 234.408 E, X52; (d) Quadraeculina anellaeformis, well 7228/7-1A 1506 m, W50-4; (e) Polycingulatisporites mooniensis, well 7228/7-1A 1506 m, B44-3; (f) ?Tripartites sp., well 7228/7-1A 1506 m, L48-4; (g) Cerebropollenites macroverrucosus, PMO 234.397 A, U37-2; (h) Ricciisporites annulatus, PMO 234.408 B, G35; (i) Semiretisporis gothae, well 7228/7-1A 1506 m, H29-1; (j) Chasmatosporites hians, PMO 234.396 A, K40-2; (k) Lycopodiacidites rugulatus, well 7228/7-1A 1506 m, R51-1; (l) Retitriletes austroclavatidites, PMO 234.408, G54-3; (m) Cingulizonates rhaeticus, PMO 234.409 A, G30-1; (n) Zebrasporites laevigatus, PMO 234.408 A, C39; (o) Kyrtomisporis laevigatus, PMO 234.408 D, O33-1; (p) Chasmatosporites apertus, well 7228/7-1A 1548 m, W37; (q) Perinopollenites elatoides, PMO 234.406 A, G35-3; (r) Araucariacites australis, well 7228/7-1A 1542 m, O34-3; (s) Limbosporites lundbladiae, well 7228/7-1A 1548 m, S51; and (t) Kyrtomisporis gracilis, PMO 234.407 A, X58-1.
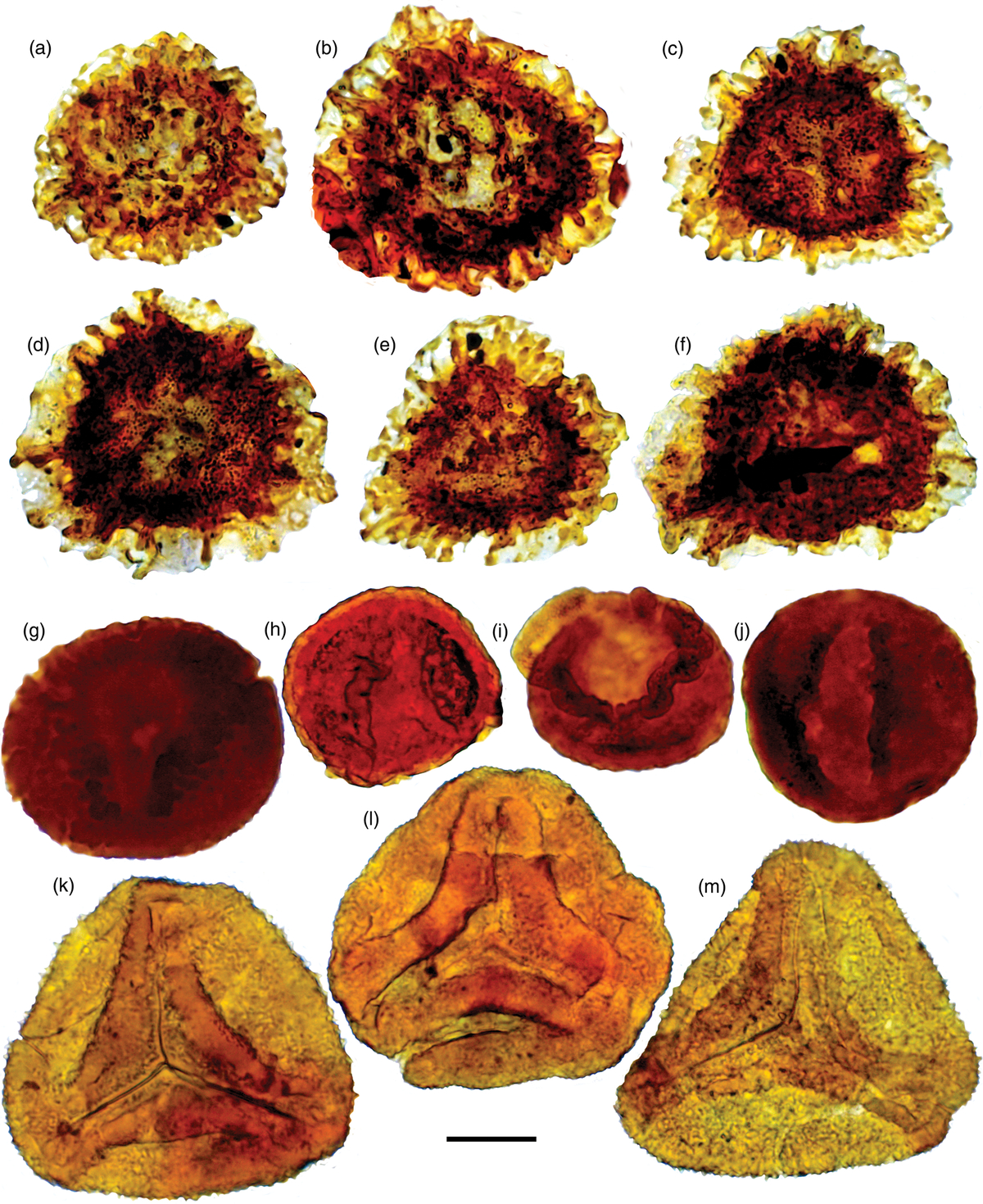
Fig. 11. Paratype specimens of new taxa described here. Palaeontological Museum Oslo (PMO) number followed by England Finder Coordinates. Scale bars = 20μm. (a–f) Semiretisporis hochulii sp. nov.: (a) PMO 234.367 A, C45-4; (b) PMO 234.367 B, K61; (c) PMO 234.372 E, U41-4; (d) PMO 234.368 B, G45; (e) PMO 234.368 A, F46-4; and (f) PMO 234.367 C, O49. (g–j) Podosporites vigraniae sp. nov.: (g) PMO 234.394 C, D49-2; (h) PMO 234.383 E, F44-1; (i) PMO 234.372 B, E37-4; and (j) PMO 234.392 E, L61-4. (k–m) Kyrtomisporis moerki sp. nov.: (k) PMO 234.385 A, V53-1; (l) PMO 234.376 C, Q40-1; and (m) PMO 234.376 A, K40-4.
Occurrence: Assemblages corresponding to the Classopollis torosus Zone have been recovered from outcrops of the Flatsalen Formation on Hopen (Paterson & Mangerud, Reference Paterson and Mangerud2015), Kong Karls Land (Smelror et al. Reference Smelror, Larssen, Olaussen, Rømuld and Williams2019) and cores from the Sentralbanken High (Paterson et al. Reference Paterson, Mangerud, Holen, Landa, Lundschien and Eide2019a). The zone has also been described from the lower Fruholmen Formation in the Hammerfest and Nordkapp basins (in the latter, undifferentiated from the overlying Rhaetogonyaulax rhaetica Zone) (Paterson & Mangerud, Reference Paterson and Mangerud2017).
Biostratigraphic correlation: The Classopollis torosus Zone is tentatively correlated with ‘Assemblage B-2’ of Hochuli et al. (Reference Hochuli, Colin, Vigran and Collinson1989) and the lowermost part of the Limbosporites lundbladii Zone (Vigran et al. Reference Vigran, Mangerud, Mørk, Worsley and Hochuli2014) (Fig. 3). Comparable assemblages rich in Classopollis torosus have been recorded in areas of the Russian Barents Shelf within Norian ‘T3k-n palynocomplex’ (Pavlov et al. Reference Pavlov, Fefilova and Lodkina1985; Fefilova, Reference Fefilova and Gramberg1988; Mørk et al. Reference Mørk, Vigran, Korchinskaya, Pchelina, Fefilova, Vavilov and Weitschat1993).
Age: The Classopollis torosus Zone is dated as early Norian (Lacian) based on an ammonoid fauna from the Flatsalen Formation on Hopen, which includes Argosirenites nelgechensis, a form typical of the Mojsisovicsites kerri Zone of British Columbia (Korčinskaya, Reference Korčinskaya and Semevskij1980; Smith, Reference Smith1982).
3.d.2. Rhaetogonyaulax arctica Dinoflagellate Cyst Zone
Definition: The Rhaetogonyaulax arctica Dinoflagellate Cyst Zone is defined by the first common occurrence of the dinoflagellate cyst Rhaetogonyaulax arctica, which occurs in association with the common occurrence of microforaminiferal test linings. This event was originally applied by Paterson & Mangerud (Reference Paterson and Mangerud2015) as a defining feature of their ‘Classopollis torosus Assemblage’. However, since the occurrence of dinoflagellate cysts is facies dependent and varies considerably across the region, separate spore-pollen and dinoflagellate cyst zones are now defined (Figs 3, 4a, b).
Biozonal assemblages: Rhaetogonyaulax arctica (common) and R. rhaetica (Fig. 9).
Occurrence: Assemblages belonging to this zone have been recorded from the transition between the De Geerdalen and lowermost Flatsalen formations on Spitsbergen, Hopen and in core material from the Sentralbanken High (Vigran et al. Reference Vigran, Mangerud, Mørk, Worsley and Hochuli2014; Paterson & Mangerud Reference Paterson and Mangerud2015; Paterson et al. Reference Paterson, Mangerud, Holen, Landa, Lundschien and Eide2019a; Rismyhr et al. Reference Simms and Ruffell2018). Comparable assemblages have also been recovered from the lower Fruholmen Formation in the Hammerfest and Nordkapp basins (in the latter, undifferentiated from the overlying Rhaetogonyaulax rhaetica Dinoflagellate Cyst Zone) (Paterson & Mangerud Reference Paterson and Mangerud2017).
Biostratigraphic correlation: The zone essentially correlates with the Rhaetogonyaulax arctica Zone defined by Rismyhr et al. (Reference Simms and Ruffell2018) on Spitsbergen, which in turn was based on the marine component present in Classopollis torosus Assemblage of Paterson & Mangerud (Reference Paterson and Mangerud2015).
Age: On Hopen, the zone is dated by an early Norian ammonoid fauna from the Flatsalen Formation, which includes Argosirenites nelgechensis, a taxon typical of the Mojsisovicsites kerri Zone of British Columbia (Korčinskaya, Reference Korčinskaya and Semevskij1980; Smith, Reference Smith1982). At the Festningen section on Spitsbergen, Vigran et al. (Reference Vigran, Mangerud, Mørk, Worsley and Hochuli2014) proposed a tentative late Carnian – early Norian age for comparable assemblages. Since the occurrence of dinoflagellate cysts is facies dependent, it is probable that the base of the zone is diachronous, younging in a southeasterly direction towards the palaeoshoreline.
3.d.3. Rhaetogonyaulax rhaetica Dinoflagellate Cyst Zone
Definition: The Rhaetogonyaulax rhaetica Dinoflagellate Cyst Zone was first described as the ‘Rhaetogonyaulax rhaetica Assemblage’ by Paterson & Mangerud (Reference Paterson and Mangerud2015). It is elevated to full zonal status here and differentiated from the correlative spore-pollen zone (Fig. 3). The base of the zone is defined based on the first common to abundant occurrence of R. rhaetica, with occurrences of Sverdrupiella spp. (Fig. 4b). The top of the zone is defined by the last occurence of R. rhaetica and Sverdrupiella spp.
Biozonal assemblages: Heibergella aculeata, H. asymmetrica, Noricysta fimbriata, N. varivallata, Rhaetogonyaulax arctica, R. rhaetica (dominant) and Sverdrupiella mutabilis. A representative selection of taxa from the Classopollis torosus, Rhaetogonyaulax arctica and R. rhaetica zones are illustrated in Figure 9.
Occurrence: Assemblages assigned to the Rhaetogonyaulax rhaetica Dinoflagellate Cyst Zone are well represented in outcrop samples from the Flatsalen Formation on Hopen (Paterson & Mangerud, Reference Paterson and Mangerud2015) and Kong Karls Land (Smelror et al. Reference Smelror, Larssen, Olaussen, Rømuld and Williams2019), and in core material from the Sentralbanken High (Paterson et al. Reference Mangerud, Paterson and Riding2018). However, in more proximal areas, such as in the Nordkapp Basin, it is more difficult to distinguish between the Rhaetogonyaulax arctica and R. rhaetica zones, due to the overall lower abundance of dinoflagellate cysts (Paterson & Mangerud Reference Paterson and Mangerud2017).
Biostratigraphic correlation: The Rhaetogonyaulax rhaetica Dinoflagellate Cyst Zone correlates with the marine components of ‘Assemblage B-2’ of Hochuli et al. (Reference Hochuli, Colin, Vigran and Collinson1989) and the lower part of the Limbosporites lundbladii Zone (Vigran et al. Reference Vigran, Mangerud, Mørk, Worsley and Hochuli2014). The Rhaetogonyaulax rhaetica Zone also likely correlates with the T3n palynocomplex of the Russian Barents Shelf (Fig. 3). The Rhaetogonyaulax rhaetica Zone is equivalent to the Heibergella spp. Composite Assemblage Zone defined by Rismyhr et al. (Reference Simms and Ruffell2018) on Spitsbergen. However, the latter seems to be more taxonomically diverse due to its occurrence in a more distal depositional setting.
Comments: The Rhaetogonyaulax rhaetica Dinoflagellate Cyst Zone is distinguished from the Rhaetogonyaulax spp. Zone of Vigran et al. (Reference Vigran, Mangerud, Mørk, Worsley and Hochuli2014), which was described from the uppermost De Geerdalen Formation (Isforden Member) at Festningen on Spitsbergen. The latter zone is likely an equivalent of our Rhaetogonyaulax arctica Zone described here, and is assigned a latest Carnian – early Norian age. Contrary to observations reported in Vigran et al. (Reference Vigran, Mangerud, Mørk, Worsley and Hochuli2014, p. 74), common to abundant specimens of R. rhaetica were not recorded in assemblages from the uppermost De Geerdalen Formation in our subsequent analysis elsewhere on Svalbard or the Barents Shelf. However, since Festningen occupied a more distal position in the basin, it is possible that R. rhaetica has a longer range at that locality, with a first occurrence during the late Carnian Age.
Age: The Rhaetogonyaulax rhaetica Dinoflagellate Cyst Zone is dated as early Norian (Lacian) by an ammonoid fauna from the Flatsalen Formation on Hopen, which includes Argosirenites nelgechensis, a form typical of the Mojsisovicsites kerri Zone of British Columbia (Korčinskaya, Reference Korčinskaya and Semevskij1980; Smith, Reference Smith1982).
3.d.3. Quadraeculina anellaeformis Zone
Definition: The Quadraeculina anellaeformis Zone was originally defined as the informal ‘Limbosporites lundbladii – Quadraeculina anellaeformis Assemblage’ by (Paterson & Mangerud, Reference Paterson and Mangerud2015). It is elevated to full zonal status here. The base of the zone is defined by the first consistent occurrence of L. lundbladiae and Q. anellaeformis (Fig. 4b) and the absence of dinoflagellate cysts and acritarchs, which characterize the preceding Rhaetogonyaulax rhaetica Dinoflagellate Cyst Zone. The latter feature is clearly facies controlled but seems to be related to a regionally significant regression, corresponding with the change from the marine shales of the Flatsalen Formation to the deltaic sandstones of the Svenskøya Formation (Paterson et al. Reference Paterson, Mangerud, Cetean, Mørk, Lord, Klausen and Mørkved2016, Reference Paterson, Morris and Mangerud2019a). The top of the zone is defined by the base of the overlying Ricciisporites spp. Zone.
Biozonal assemblages: Araucariacites australis, Chasmatosporites apertus, C. hians, Cycadopites spp., Deltoidospora spp., Kyrtomisporis gracilis, K. laevigatus, K. speciosus, Limbosporites lundbladiae and Quadraeculina anellaeformis. A representative selection of taxa from the Limbosporites lundbladii and Ricciisporites spp. zones are illustrated in Figure 10.
Occurrence: The Quadraeculina anellaeformis Zone has been recorded in preparations from the lower part of the Svenskøya Formation on Hopen (Paterson & Mangerud, Reference Paterson and Mangerud2015) and Kong Karls Land (Smelror et al. Reference Smelror, Larssen, Olaussen, Rømuld and Williams2019), and in stratigraphical cores from the Sentralbanken High (Paterson et al. Reference Paterson, Mangerud, Holen, Landa, Lundschien and Eide2019a). Assemblages corresponding to the zone have also been recorded in the middle part of the Fruholmen Formation in exploration wells in the Nordkapp Basin (Paterson & Mangerud Reference Paterson and Mangerud2017) (Fig. 3).
Biostratigraphic correlation: The Quadraeculina anellaeformis Zone correlates with the ‘Limbosporites lundbladii – Quadraeculina anellaeformis Assemblage’ of Paterson & Mangerud (Reference Paterson and Mangerud2015), which was described from the lower part of the Svenskøya Formation on Hopen. Equivalent assemblages have been documented in the Fruholmen Formation in exploration wells from the southern Barents Sea as ‘assemblage SBS-II’ (Paterson & Mangerud Reference Paterson and Mangerud2017), and from the Svenskøya Formation in stratigraphical cores from the Sentralbanken High (Paterson et al. Reference Mangerud, Paterson and Riding2018). The zone correlates with ‘Assemblage B1’ of Hochuli et al. (Reference Hochuli, Colin, Vigran and Collinson1989) and the upper part of the Limbosporites lundbladii Zone of Vigran et al. (Reference Vigran, Mangerud, Mørk, Worsley and Hochuli2014). On Hopen, the Quadraeculina anellaeformis Zone corresponds to an interval rich in lycopsid megaspores, recently defined as the Dijkstraisporites beutleri – Verrutriletes preutilis Zone (Paterson et al. Reference Paterson, Morris and Mangerud2019b).
Age: Regionally, outcrops of the Svenskøya Formation (and correlative interval of the Fruholmen Formation) are devoid of age-diagnostic macrofossil remains. The presence of an early Norian ammonoid fauna in the underlying Flatsalen Formation (Korčinskaya, Reference Korčinskaya and Semevskij1980; Smith, Reference Smith1982) constrains the age of the Svenskøya Formation as Norian or younger; however, since the two formations are separated by an erosional unconformity, it is uncertain how much of the Norian is missing. In the absence of direct independent faunal control, a Norian–(?) Rhaetian age has been proposed (Paterson & Mangerud, Reference Paterson and Mangerud2015; Paterson et al. Reference Paterson, Mangerud, Holen, Landa, Lundschien and Eide2019a).
3.e. Rhaetian
3.e.1. Ricciisporites spp. Zone
Definition: The Ricciisporites spp. Zone is defined based on the first common occurrence of Ricciisporites annulatus and R. tuberculatus, with consistent occurrences of Cerebropollenites spp., Rogalskaisporites ambientis, Polycingulatisporites crenulatus and P. mooniensis. The top of the zone is marked by the last occurrences of several taxa including Aratrisporites laevigatus, A. macrocavatus, Kyrtomisporis gracilis, K. laevigatus, K. speciosus, Limbosporites lundbladiae, Ovalipollis spp., Ricciisporites annulatus, R. tuberculatus and taeniate bisaccate pollen.
Biozonal assemblages: Aratrisporites spp., Baculatisporites spp., Cerebropollenites macroverrucosus, Chasmatosporites apertus, C. hians, Cingulizonates rhaeticus, Concavisporites crassexinus, Deltoidospora spp., Ischyosporites sp., Kyrtomisporis gracilis, K. laevigatus, K. speciosus, Limbosporites lundbladiae, Lunatisporites rhaeticus, Ovalipollis ovalis, Polycingulatisporites crenulatus, P. mooniensis, Quadraeculina anellaeformis, Ricciisporites annulatus, R. tuberculatus, Striatella parva, S. seebergensis, Trachysporites spp., Zebrasporites interscriptus and Z. laevigatus.
Occurrence: Assemblages corresponding to the Ricciisporites spp. Zone have been recorded from the uppermost Svenskøya Formation on Hopen and in preparations from the Fruholmen Formation in exploration wells from the Nordkapp Basin (Paterson & Mangerud, Reference Paterson and Mangerud2015, Reference Paterson and Mangerud2017).
Comments: The Ricciisporites spp. Zone was previously described as the ‘Rogalskaisporites ambientis Assemblage’ by Paterson & Mangerud (Reference Paterson and Mangerud2015). Despite the probable correlation with the Ricciisporites tuberculatus Zone of Vigran et al. (Reference Vigran, Mangerud, Mørk, Worsley and Hochuli2014), Paterson & Mangerud (Reference Paterson and Mangerud2015) proposed R. ambientis as an alternative to the nominate taxon, due to the absence of R. tuberculatus on Hopen. Instead, assemblages on Hopen contained laevigate specimens more consistent with the taxonomic circumscription of Ricciisporites annulatus. Subsequent investigation of the correlative Fruholmen Formation in the Nordkapp Basin has shown both R. annulatus and R. tuberculatus to be present in the same assemblages, while Rogalskaisporites ambientis is absent. It is therefore possible that the distribution of each of these taxa is facies dependent.
The first consistent occurrence of Cerebropollenites macroverrucosus is also characteristic of this zone. The taxon has previously been considered diagnostic of the Jurassic (e.g. Vigran et al. Reference Vigran, Mangerud, Mørk, Worsley and Hochuli2014). However, co-occurrence of the taxon with Aratrisporites spp. and striate bisaccate pollen (e.g. Paterson & Mangerud, Reference Paterson and Mangerud2015) is suggestive of a Rhaetian age.
Biostratigraphic correlation: The Ricciisporites spp. Zone correlates with ‘Assemblage A’ of Hochuli et al. (Reference Hochuli, Colin, Vigran and Collinson1989) and the Rhaetian-aged Ricciisporites tuberculatus Zone of Vigran et al. (Reference Vigran, Mangerud, Mørk, Worsley and Hochuli2014). The zone likely corresponds to the ‘T3r palynocomplex’ of the Russian Barents Shelf (Fig. 3).
Age: Regionally, the Svenskøya Formation, and correlative interval of the Fruholmen Formation, lack age-diagnostic macrofossil remains. However, ammonoid data from the underlying Flatsalen Formation (Korčinskaya, Reference Korčinskaya and Semevskij1980; Smith, Reference Smith1982) constrains the age of the Svenskøya Formation as Norian or younger. Paterson & Mangerud (Reference Paterson and Mangerud2015) proposed a tentative Rhaetian age for the zone, in agreement with previous interpretations by Hochuli et al. (Reference Hochuli, Colin, Vigran and Collinson1989) and Vigran et al. (Reference Vigran, Mangerud, Mørk, Worsley and Hochuli2014).
4. Discussion
The palynozonation presented here provides an enhanced stratigraphical resolution for the Middle–Upper Triassic stratigraphy of the Norwegian Barents Sea, which builds upon the regional stratigraphic framework established by previous workers (e.g. Hochuli et al. Reference Hochuli, Colin, Vigran and Collinson1989; Vigran et al. Reference Vigran, Mangerud, Mørk, Worsley and Hochuli2014). The primary contribution of this present work is in the refinement of the Late Triassic zonal scheme, which in the previous scheme was divided into four zones (Fig. 3) corresponding to a timespan of 35.6 Ma (e.g. Ogg et al. Reference Ogg, Ogg and Gradstein2016). The recognition of seven spore-pollen zones thus improves the biozonal resolution for a key stratigraphic interval, which includes economically significant hydrocarbon source and reservoir units (Henricksen et al. Reference Henricksen, Ryseth, Larssen, Heide, Rønning and Stoupakova2011; Lundschien et al. Reference Lundschien, Høy and Mørk2014; Ryseth Reference Ryseth, Martinius, Ravnås, Howell, Steel and Wonham2014). The results of this study therefore make an important contribution to ongoing exploration in the region. This is particularly true for Barents Sea North, an area not yet opened for exploration. In a recent evaluation of the region (NPD Reference Paterson and Mangerud2017) the Norwegian Petroleum Directorate estimates that Triassic reservoirs make the largest contribution to resource potential with no less than 56% of the area’s recoverable resources. However, geological uncertainty is still high; the palynozonation presented here therefore provides much-needed age control in this relatively unexplored area.
The present work also contributes to a greater understanding of Triassic palynofloras on the northern Pangaean margin, which have been convincingly demonstrated to be taxonomically and quantitatively distinct from those of other areas such as the Germanic or Alpine regions. Several palynofloral trends have been noted, which likely reflect palaeoenvironment and, perhaps, palaeoclimatic control. These are briefly commented upon here; however, a more complete interpretation of their significance awaits further study, particularly in the Russian sector of the Barents Sea.
Firstly, the high abundance of Aratrisporites spp. in Anisian deposits of the Greater Barents Sea area is a feature which is recognized in coeval deposits of both the western (Hochuli & Vigran Reference Hochuli and Vigran2010; Vigran et al. Reference Vigran, Mangerud, Mørk, Worsley and Hochuli2014; Paterson & Mangerud Reference Paterson and Mangerud2017) and eastern regions (Pavlov et al. Reference Pavlov, Fefilova and Lodkina1985; Mørk et al. Reference Mørk, Vigran, Korchinskaya, Pchelina, Fefilova, Vavilov and Weitschat1993; Fefilova, Reference Fefilova and Nekhorosheva2013). This particular genus has been recovered in situ in sporangial remains of various heterosporous lycopsids of the families Isoetaceae and Pleuromeiaceae (Balme, Reference Balme1995; Taylor et al. Reference Taylor, Taylor and Krings2009), which likely grew in coastal lagoons or mangrove-type habitats (Retallack, Reference Retallack1975, Reference Retallack1997; Kürschner & Herngreen, Reference Kürschner and Herngreen2010). As noted by Hochuli & Vigran (Reference Hochuli and Vigran2010), in lower palaeolatitudes this taxon is particularly common in assemblages with other evidence for high humidity (c.f. Hochuli & Frank, Reference Hochuli and Frank2000). The apparent uniformity in Anisian palynofloras across the Greater Barents Sea suggests that the area comprised a single flora province during that time. These Anisian assemblages contrast with those described from central and southern European localities, where assemblages are composed almost exclusively of xerophytic taxa such as Triadispora, considered indicative of arid palaeoclimatic conditions (e.g. Kürschner & Herngreen, Reference Kürschner and Herngreen2010). Nonetheless, some differences are apparent between Anisian palynofloras from the western and eastern Barents Sea. For instance, there is a marked increase in bisaccate pollen and acritarchs in deposits of Svalbard and the Norwegian Barents Sea that is not recognized in the Russian sector (Mørk et al. Reference Mørk, Vigran, Korchinskaya, Pchelina, Fefilova, Vavilov and Weitschat1993). This likely reflects the fact that much of western Barents Sea was situated in a more distal basinal setting during this time, while the eastern sector was dominated by an extensive coastal plain (Lundschien et al. Reference Lundschien, Høy and Mørk2014; Klausen et al. Reference Klausen, Ryseth, Helland-Hansen, Gawthorpe and Laursen2015). The influx in bisaccate pollen in the Norwegian Barents Sea during the Anisian may therefore be due to the ‘Neves effect’ of Chaloner & Muir (Reference Chaloner, Muir, Murchison and Westoll1968).
The almost total dominance of Leschikisporis aduncus is a common feature in upper Carnian De Geerdalen on Svalbard and in the correlative upper Snadd Formation in many areas of the Barents Sea (Hochuli & Vigran, Reference Hochuli and Vigran2010; Vigran et al. Reference Vigran, Mangerud, Mørk, Worsley and Hochuli2014; Paterson & Mangerud, Reference Paterson and Mangerud2015, Reference Paterson and Mangerud2017; Paterson et al. Reference Paterson, Mangerud and Mørk2017, Reference Paterson, Mangerud, Holen, Landa, Lundschien and Eide2019a). This spore has been isolated from fertile fronds of the fern Asterotheca merianii (Bhardwaj & Singh, Reference Bhardwaj and Singh1957; Vasilevskaya, Reference Vasilevskaya, Sokolova and Vasilevskaya1972; Kustatscher & Van Konijnenburg-van Cittert, Reference Kustatscher and Van Konijnenburg-Van Cittert2011), which is equally prevalent among late Carnian macrofloras from Svalbard (Pott, Reference Pott2014). Based on the size of several A. merianii fossils, Pott (Reference Pott2014) argued that these plants may have been quite tall ‘tree-like’ ferns, similar to modern Dicksonia species. The plant is interpreted to have grown in humid swamp habitats (Roghi et al. Reference Roghi, Gianolla, Minarelli, Pilati and Preto2010), which is consistent with the dominance of L. aduncus in coal layers on Svalbard (Bjærke & Manum, Reference Bjærke and Manum1977; Vigran et al. Reference Vigran, Mangerud, Mørk, Worsley and Hochuli2014; Paterson & Mangerud, Reference Paterson and Mangerud2015). The wide distribution of assemblages belonging to the L. aduncus Zone in the western Barents Sea region suggests the establishment of a vast coastal plain swamp. Intriguingly, this coincides with the maximum regressive phase of the De Geerdalen deltaic system (Klausen et al. Reference Klausen, Ryseth, Helland-Hansen, Gawthorpe and Laursen2015). Paterson et al. (Reference Paterson and Mangerud2015) interpreted the dominance of L. aduncus to reflect the basinwards expansion of humid coastal plain habitats accompanying this marine regression. The co-occurrence of certain pollen taxa typically considered indicative of drier conditions, such as Ovalipollis, in assemblages of the Leschikisporis aduncus Zone probably reflects an allochthonous palynoflora transported by wind and rivers from the hinterland.
The abrupt transition to the Protodiploxypinus Zone in uppermost Carnian strata marks the disappearance of L. aduncus in the western Barents Sea, and the replacement of fern-spore-dominated palynofloras by assemblages rich in Protodiploxypinus and the alga Plaesiodictyon mosellanum. Protodiploxypinus was produced by a xerophytic coastal pioneer plant (Brugman et al. Reference Brugman, Van Bergen, Kerp and Traverse1994), while P. mosellanum is thought to reflect fresh- to brackish-water habits (Brenner, Reference Brenner1992; Brenner & Foster, Reference Brenner and Foster1994; Wood & Miller, Reference Wood and Miller1998; Wood & Benson, Reference Wood and Benson2000). On Hopen, the palynofloral turnover coincides with a marine transgression (Lord et al. Reference Lord, Solvi, Ask, Mørk, Hounslow and Paterson2014), leading Paterson et al. (Reference Paterson and Mangerud2015) to propose that it was driven by an inundation of coastal plain habitats by brackish water, resulting in the destruction of the habitats in which Asterotheca meriani was growing. This interpretation is consistent with the continued presence of L. aduncus in the Rhaetian in the more proximal eastern region of the Barents Sea (e.g. Mørk et al. Reference Mørk, Vigran, Korchinskaya, Pchelina, Fefilova, Vavilov and Weitschat1993; Fefilova, Reference Fefilova and Nekhorosheva2013); its disappearance in the Norwegian Barents is therefore apparently local, and driven by palaeoenvironmental rather than climatic change.
The uppermost Carnian Protodiploxypinus spp. Zone is also notable for the first occurrence of the fern spores Kyrtomisporis gracilis, K. laevigatus and K. speciosus, the lycopsid spore Retitriletes austroclavatidites and the pollen taxon Quadraeculina anellaeformis. In central and southern European localities, these taxa do not typically appear until the Rhaetian Age (e.g. Cirilli, Reference Cirilli2010; Kürschner & Herngreen, Reference Kürschner and Herngreen2010); their first occurrence in the Barents Sea therefore apparently occurs c. 20 Ma earlier than elsewhere. We postulate that these taxa first evolved on the northern coastline of Pangaea during the late Carnian Age, perhaps in response to the palaeoenvironmental and palaeoecological change triggered by rising sea levels. The taxa then spread to most of central and southern Europe by the Rhaetian Age during a humid phase.
The influx of Classopollis pollen during early Norian time in both the western (Vigran et al. Reference Vigran, Mangerud, Mørk, Worsley and Hochuli2014; Paterson & Mangerud Reference Paterson and Mangerud2015; Paterson et al. Reference Paterson, Mangerud, Cetean, Mørk, Lord, Klausen and Mørkved2016) and eastern sectors of the Barents Sea (Pavlov et al. Reference Pavlov, Fefilova and Lodkina1985; Fefilova, Reference Fefilova and Gramberg1988; Mørk et al. Reference Mørk, Vigran, Korchinskaya, Pchelina, Fefilova, Vavilov and Weitschat1993) corresponds to a regionally marine transgression (i.e. the S6/N1 sequence boundary of Klausen et al. Reference Klausen, Ryseth, Helland-Hansen, Gawthorpe and Laursen2015). The increase in Classopollis may therefore reflect the spread of halophytic conifers (see Alvin, Reference Alvin1982; Abbink et al. Reference Abbink, Van Konijnenburg-Van Cittert and Visscher2004), which colonized tidal flat environments created by rising relative sea levels. However, since an increase in Circumpolles including Classopollis is also a common feature of lower Norian assemblages from lower palaeolatitudes (Lund Reference Lund1977; Schuurman Reference Schuurman1977, Reference Schuurman1979; Orłowska-Zwolińska Reference Orłowska-Zwolińska1985; Kürschner & Herngreen, Reference Kürschner and Herngreen2010; Fijałkowska-Mader et al. Reference Fijałkowska-Mader, Heunisch and Szulc2015), their proliferation may be of wider palaeoclimatic significance.
5. Systematic palynology
Genus Kyrtomisporis (Mädler) Van der Eem, Reference Van der Eem1983
Type species Kyrtomisporis laevigatus Mädler, Reference Mädler1964
Kyrtomisporis moerki sp. nov. Figure 6b.
2017 Kyrtomisporis sp. A; Paterson, Mangerud & Mørk, pl. 2, 12–15.
2017 Kyrtomisporis sp. A; Paterson & Mangerud, fig. 3.
2019a Kyrtomisporis sp. A; Paterson, Mangerud, Holen, Landa, Lundschien & Eide, pl. 2, M.
Derivation of name. Named after the Norwegian geologist Dr Atle Mørk in recognition of his contribution to Triassic stratigraphy and sedimentology in the Norwegian Arctic.
Holotype. PMO 234.376 B, core 7830/5-U-1, 68.82 m depth (slide A), England Finder slide coordinates M44-1, Figure 6b.
Paratypes. Core 7830/5-U-1: PMO 234.385 A, 68.82 m depth (slide B), England Finder slide coordinates V53-1, Figure 11k; PMO 234.376 C, 68.82m depth (slide A), England Finder slide coordinates Q40-1, Figure 11l; PMO 234.376 A, 68.82m depth (slide A), England Finder slide coordinates K40-4, Figure 11m.
Diagnosis. Trilete spores, kyrtomate and cingulate. Amb roundly triangular with convex interradials. Laesurae simple, slightly sinuous and bordered by narrow labra extending to inner margins of cingulum. Proximal face with kyrtome, fusing with cingulum at apices; kyrtome 8–(13)–20 µm at the narrowest point and 12–(19)–25 μm wide at the widest point. Proximal and distal surface densely ornamented with an apiculate sculpture consisting of verrucae, pila and spinules. Sculptural elements 0.5–(1.2)–1.8 µm wide, 0.6–(1.4)–6 µm tall, projecting along the margins of the amb and kyrtome. Cingulum 3–(7)–12 µm wide, faint, often obscured by the dense ornament.
Description. A species of Kyrtomisporis that is characterized by a scabrate–apiculate ornament on both proximal and distal faces. Proximal surface with arcuate thickenings in the interradial areas, bordering the laesurae. In some specimens the thickenings form simple tori; however, in most specimens the thickenings comprise a true kyrtome which extends to the cingulum at the apices, where they occasional form circular, button-like protrusions, as present in other members of the genus. Spores also observed as rare tetrads.
Dimensions. 54–(64)–80 µm (based on 25 measured specimens).
Type locality. Stratigraphic core 7830/5-U-1, offshore Kong Karls Land, Barents Sea.
Type stratum. Snadd Formation (lower De Geerdalen Formation equivalent).
Age. Late Triassic (early Carnian).
Comparison. Kyrtomisporis moerki sp. nov. is distinguished from all other members of the genus by its scabrate to apiculate ornament on both proximal and distal surfaces. Kyrtomisporis ervii Van der Eem, Reference Van der Eem1983 and K. pseudoreticulatus Sivak & Shang, Reference Sivak and Shang1989 are characterized by a distal ornament of irregular rugulae, often perpendicular to the interradials. Kyrtomisporis coronarius (Chang) Li & Shang, Reference Li and Shang1980 and K. gracilis Bjærke and Manum, Reference Bjærke and Manum1977, have a radially rugulate ornament, with large bulbous projections along the amb in equatorial view. Kyrtomisporis corrugatus Cameron, Reference Cameron1974 is distinguished by its strongly crenulated cingulum. Kyrtomisporis laevigatus Mädler, Reference Mädler1964 is distinguished by its sparse distal ornament of broad-based, low verrucae. Kyrtomisporis speciosus Mädler, Reference Mädler1964 has a dense verrucate, papillate to coalescent rugulate ornament. Kyrtomisporis niger Bjærke & Manum, Reference Bjærke and Manum1977 is characterized by a chagrenate to laevigate surface, and a distal face with five to seven low, faint transverse ridges, 3–5 µm wide. Concavisporites scabratus Bjærke & Manum, Reference Bjærke and Manum1977 is superficially similar to Kyrtomisporis moerki sp. nov. based on its tori and scabrate ornament, but is acingulate and has a smooth outer surface in optical section. Habrozonosporites Lu & Wang, Reference Lu and Wang1980 possesses a kyrtome and has a similar ornament, but is camerate and acingulate.
Genus Semiretisporis Reinhardt, Reference Reinhardt1962
Type species: Semiretisporis gothae Reinhardt, Reference Reinhardt1962
Semiretisporis hochulii sp. nov. Figures 5d, 10a–f.
1985 ‘Semiretisporis barentszi’ van Veen, p. 98–99, pl. 9, 7; pl. 10, 7, 8, 10, pl. 11, 1.
1989 Semiretisporis sp. 1 Hochuli, Colin & Vigran, pl. 4, 1,2, p. 142.
1998 Semiretisporis sp. A Vigran, Mangerud, Mørk, Bugge & Weitschat, pl. 9, 1.
2008 Semiretisporis sp. 1 ‘barentzii’ Hounslow, Yu, Mørk, Weitschat, Vigran, Karloukovski & Orchard, fig. 7.
2014 ‘Semiretisporis barentzii’ Vigran, Mangerud, Mørk, Worsley & Hochuli, p. 82, 150, 154, 155, 173, 175, 263.
2014 ‘Semiretisporis sp. A (barentzii)’ Vigran, Mangerud, Mørk, Worsley & Hochuli, p. 90, 97, 98, 143, 149, 263; pl. 18, I.
Holotype. PMO 234.369 A, core 7534/4-U-1, 189.66 m depth, England Finder slide coordinates K54, Figure 5d.
Paratypes. Core 7534/4-U-1: PMO 234.367 A, 171.83 m depth, 5B, England Finder slide coordinates C45-4, Figure 11a; PMO 234.367 B, 171.83 m depth, 5B, England Finder slide coordinates K61, Figure 11b; PMO 234.372 E, 194.53 m depth, 7B, England Finder slide coordinates U41-4, Figure 11c; PMO 234.368 B, 175.10 m depth, 6B, England Finder slide coordinates G45, Figure 11d; PMO 234.368 A, 175.10 m depth, 6B, England Finder slide coordinates F46-4, Figure 11e; PMO 234.367 C, 171.83 m depth, 5B, England Finder slide coordinates O49, Figure 11f.
Derivation of name: The species is named in honour of the late Swiss palynologist Peter A. Hochuli in recognition of his contribution to Triassic palynology in the Barents Sea region.
Diagnosis: Trilete, cingulizonate, semireticulate spores. Amb triangular to subtriangular, with an irregular, often serrated outline. Trilete mark indistinct. Proximal surface essentially smooth. Distal surface sparsely ornamented with baculae, verrucae and echinae, which on some specimens coalesce into a loosely defined reticulum. Ornament more densely concentrated on the equator, fusing to form a cingulum (4–16 μm wide), bordered by a zona (7–18 μm wide). Narrow, digitate rugulae, 2–7 μm wide, radiate from the cingular thickening, and fuse with the outer margin of the zona, delineating lumina 3–8 μm wide.
Description: A species of Semiretisporis that is characterized by a combination of verrucate, echinate and baculate sculptural elements, which fuse to form a cingulum, from which narrow rugulae radiate, merging with the outer margins of a zona. The ornament defines a loose and inconsistently developed reticulate pattern. The density, size and distribution of the sculptural elements and degree of reticulum connectivity varies considerable between specimens, as does the overall equatorial spore diameter, and zona and cingulum width.
Dimensions. Total diameter 58–(69)–86 µm; inner body 26–(33)–45 µm (based on 22 measured specimens).
Type locality: Stratigraphical core 7534/4-U-1, Sentralbanken High, Barents Sea.
Type horizon: lower Snadd Formation.
Stratigraphic range: Ladinian – lower Carnian (Echinitosporites iliacoides–Podosporites vigraniae zones).
Comparison: Semiretisporis hochulii sp. nov. is distinguished from S. gothae Reinhardt Reference Reinhardt1962, and S. maljavkinae Schulz Reference Schulz1967, due to the more irregular nature of its reticulum and zona margin.
Comment: This species was informally described as Semiretisporis ‘barentszi’ by van Veen (1985). However, the name was not effectively published based on ICBN Article 30.9 and Note 3, Recommendation 30A.4 (Turland et al. 2018). Furthermore, no holotype was designated in van Veen’s description.
Genus: Podosporites
Type species: Podosporites tripakshi Rao, Reference Rao1943
Podosporites vigraniae sp. nov. Figures 5k, 6n, 11g–j.
Holotype. PMO 234.374 B, core 7534/4-U-1, 216.09 m depth, England Finder slide coordinates S42-2, Figure 5k.
Paratypes. PMO 234.379 A, core 7830/5-U-1, 91.57 m depth (slide B), England Finder slide coordinates G47-1, Figure 6n; PMO 234.394 C, core 7533/2-U-1, 164.75 m depth, England Finder slide coordinates D49-2, Figure 11g; PMO 234.383 E, core 7830/5-U-1, 126.94 m depth (slide A), England Finder slide coordinates F44-1, Figure 11h; PMO 234.372 B, core 7534/4-U-1, 194.53 m depth, 7B, England Finder slide coordinates E37-4, Figure 11i; PMO 234.392 E, core 7533/2-U-1, 157.98 m depth, England Finder slide coordinates L61-4, Figure 11j.
Derivation of name: The species is named after the Norwegian palynologist Jorunn Os Vigran, in recognition of her contributions to Triassic palynostratigraphy in the Barents Sea region.
Diagnosis: Bisaccate or trisaccate, monosulcate pollen. Central body circular or oval in shape. Sacci rudimentary, situated on distal surface, forming low, sinuous or vermiculate ridges bordering a broad sulcal opening. Sulcus circular to oval in shape. Exine thick (3–6 μm) with a granulate to verrucate texture.
Description: A large species of Podosporites characterized by a thick wall, and poorly developed sacci. The sacci are displaced to the distal surface, where they form sinuous to vermiculate (occasionally straight) ridges 3–(4)–8 µm in width and height, which border the sulcus. Sulcal opening commonly gaping, 7–(17)–26 µm in width at the widest point. The exine is coarsely ornamented by granulae or low verrucae. Specimens are rarely folded due to the robust wall.
Dimensions. 50–(57)–77 µm width; 42–(53)–74 µm height (based on 15 measured specimens).
Type locality: Stratigraphical core 7534/4-U-1, Sentralbanken High, Barents Sea.
Type horizon: Snadd Formation (lower De Geerdalen Formation equivalent).
Stratigraphic range: Upper Anisian – upper Carnian (Protodiploxypinus decus – Leschikisporis aduncus zones).
Comparison: Podosporites vigraniae sp. nov. is closely comparable to P. amicus Scheuring, Reference Scheuring1970, which was described from the Carnian Gipskeuper of Switzerland. However, according to Scheuring (Reference Scheuring1970) P. amicus is smaller (38–43 µm width; 30–38 µm height) with a thinner wall (2–3 µm). Podosporites vigraniae sp. nov. is therefore distinguished by its consistently larger size, thicker wall and coarser ornamentation.
Acknowledgements
This research was funded by a Forum for Reservoir Characterization, Reservoir Engineering and Exploration Technology Cooperation (FORCE) industry consortium, consisting of AkerBP (formerly Det Norske), Centrica, Chevron, ConocoPhillips, Dong, Eni, Lundin, Shell and Statoil. Niall W. Paterson received additional funding from the Norwegian Research Council through the ‘Internal and external forcing factors on the source-to-sink infill dynamics of the Lower Mesozoic Greater Barents Sea Basin’ (ISBAR) project (grant no. 267689). Both authors thank Malcolm Jones (Palynological Laboratory Services Limited) for preparation of palynological slides. The Norwegian Petroleum Directorate (NPD) and Applied Petroleum Technology are thanked for providing access to palynological preparations, exploration well samples and stratigraphical cores used in this study.
Declaration of Interest
The authors report no potential conflicts of interest.