1. Introduction
Present-day convergent margins are often characterized by the occurrence of ophiolites along the upper plate(s) in a large variety of settings related to terrane accretion processes (see Dilek & Furnes, Reference Dilek and Furnes2014, and references therein). This study focuses on the island of Karpathos (Dodecanese, Greece), which belongs to the Aegean fore-arc (Fig. 1) and is located at the junction between two important ophiolite domains of the Mediterranean region: the western region (Dinarides, Hellenides) and the eastern region (Taurides, Cyprus, Syria) (Robertson, Reference Robertson2002). Although the Neogene history of the Eastern Mediterranean region is fairly well understood following a very large number of studies and the application of powerful tools such as high-resolution seismic tomography (Royden & Faccenna, Reference Royden and Faccenna2018, and references therein), our knowledge of older regional palaeogeographies is less accurate. In the Aegean Sea, the origin of scattered blocks and nappes constituting the Cyclades, Crete and the Dodecanese is not well understood. In particular, there are some difficulties in establishing precise geological correlations between these islands and the surrounding continental margins (Roche et al. Reference Roche, Conand, Jolivet and Augier2018).

Fig. 1. Structural map of the southern Aegean region with location of the island of Karpathos (black frame) and the distribution of ophiolites in southern Greece and western Turkey. Compiled from Bornovas & Rontogianni-Tsiabaou (Reference Bornovas and Rontogianni-Tsiabaou1983), Jolivet et al. (Reference Jolivet, Rimmelé, Oberhänsli, Goffé and Candan2004, Reference Jolivet, Faccenna, Huet, Labrousse, Le Pourhiet, Lacombe, Lecomte, Burov, Denèle, Brun, Philippon, Paul, Salaün, Karabulut, Piromallo, Monié, Gueydan, Okay, Oberhänsli, Pourteau, Augier, Gadenne and Driussi2013), van Hinsbergen et al. (Reference Van Hinsbergen, Zachariasse, Wortel and Meulenkamp2005, Reference Van Hinsbergen, Kaymakçi, Spakman and Torsvik2010), Ersoy et al. (Reference Ersoy, Cemen, Helvaci and Billor2014) and Gürer et al. (Reference Gürer, Plunder, Kirst, Corfu, Schmid and van Hinsbergen2018). The ‘eastern’ and ‘western’ ophiolite regions refer to the classification by Robertson (Reference Robertson2002). IAE: Izmir–Ankara–Erzincan Suture Zone.
Due to its ‘pivotal’ position between the ophiolite regions of Greece and Turkey, Karpathos has attracted significant geological attention for more than a century (see Appendix ‘Pioneering studies on Karpathos’, online Supplementary Material at https://doi.org/10.1017/S0016756819000657), but diverging interpretations about the age of the ophiolite have led to conflicting views: while K–Ar geochronology documented Late Cretaceous isotopic ages (Koepke et al. Reference Koepke, Kreuzer and Seidel1985; Hatzipanagiotou, Reference Hatzipanagiotou1991; Koepke et al. Reference Koepke, Seidel and Kreuzer2002), the sedimentary rocks overlying the ophiolite were considered to be early Early Cretaceous based on calpionellids, suggesting that the ophiolite could be as old as Late Jurassic (Davidson-Monett, Reference Davidson-Monett1974; Aubouin et al. Reference Aubouin, Bonneau and Davidson1976). These two distinct ages led to very different palaeogeographic scenarios: the Karpathos ophiolite has been interpreted as a remnant of the Pindos Ocean (Aubouin et al. Reference Aubouin, Bonneau and Davidson1976), the Northern Neotethys (Robertson, Reference Robertson2002) or the Southern Neotethys due to age and petrological affinities with Late Cretaceous ophiolites of Turkey, Syria and Oman (Koepke et al. Reference Koepke, Seidel and Kreuzer2002).
Since no modern radiolarian study has ever been undertaken on the island, we have revisited Karpathos and reassessed the potential of its supra-ophiolitic sedimentary strata, with three major objectives: (1) to solve this chronological conundrum by focusing on radiolarian cherts, (2) to improve the correlations between Karpathos and the surrounding Mediterranean ophiolites (Greece, Crete, Rhodes, Turkey) and (3) to develop a better understanding of Neotethys palaeogeography and tectonics in the Aegean region.
2. Geological setting
2.a. The Xindothio Unit
The Karpathos ophiolite forms relatively small exposures (Fig. 2a−c) of mainly serpentinized peridotite with minor occurrences of gabbro and dolerite dykes (‘dismembered’ type after Coleman, Reference Coleman1977). Early geological studies provided some preliminary descriptions of the magmatic rocks and associated sedimentary strata (de Stefani et al. Reference de Stefani, Forsyth Major, Barbey, de Stefani, Forsyth Major and Barbey1895; Martelli, Reference Martelli1916; Christodoulou Reference Christodoulou1960; Aubouin & Dercourt, Reference Aubouin and Dercourt1970; online Supplementary Material at https://doi.org/10.1017/S0016756819000657). Later, geological mapping demonstrated that these rocks lie within tectonic slices at the top of the nappe pile exposed in the central part of the island and belong to a tectono-stratigraphic succession described as the ‘Xindothio Unit’ (Davidson-Monett, Reference Davidson-Monett1974; Aubouin et al. Reference Aubouin, Bonneau and Davidson1976; Hatzipanagiotou, Reference Hatzipanagiotou1987). The Xindothio Unit overlies the Kalilimni Unit, a Jurassic to Eocene parautochthonous carbonate platform (Fig. 2a), and comprises eight subunits from base to top (Fig. 3a): ammonite-bearing marl, belemnites-bearing sandstone, platy limestone with filaments (Halobia), grey platy limestone, mafic and ultramafic rocks (serpentinite, gabbro, dolerite), red radiolarite and pink microcrystalline limestone, platy green limestone, fine-grained limestone and siliceous beds. The base of the Xindothio Unit has been dated as early Late Triassic (Carnian) with ammonites (Tropites subbullatus; Aubouin et al. Reference Aubouin, Bonneau and Davidson1976), while the top is Campanian–Maastrichtian based on planktonic foraminifera (Globotruncana stuarti, G. stuartiformis and G. contusa; Aubouin et al. Reference Aubouin, Bonneau and Davidson1976).
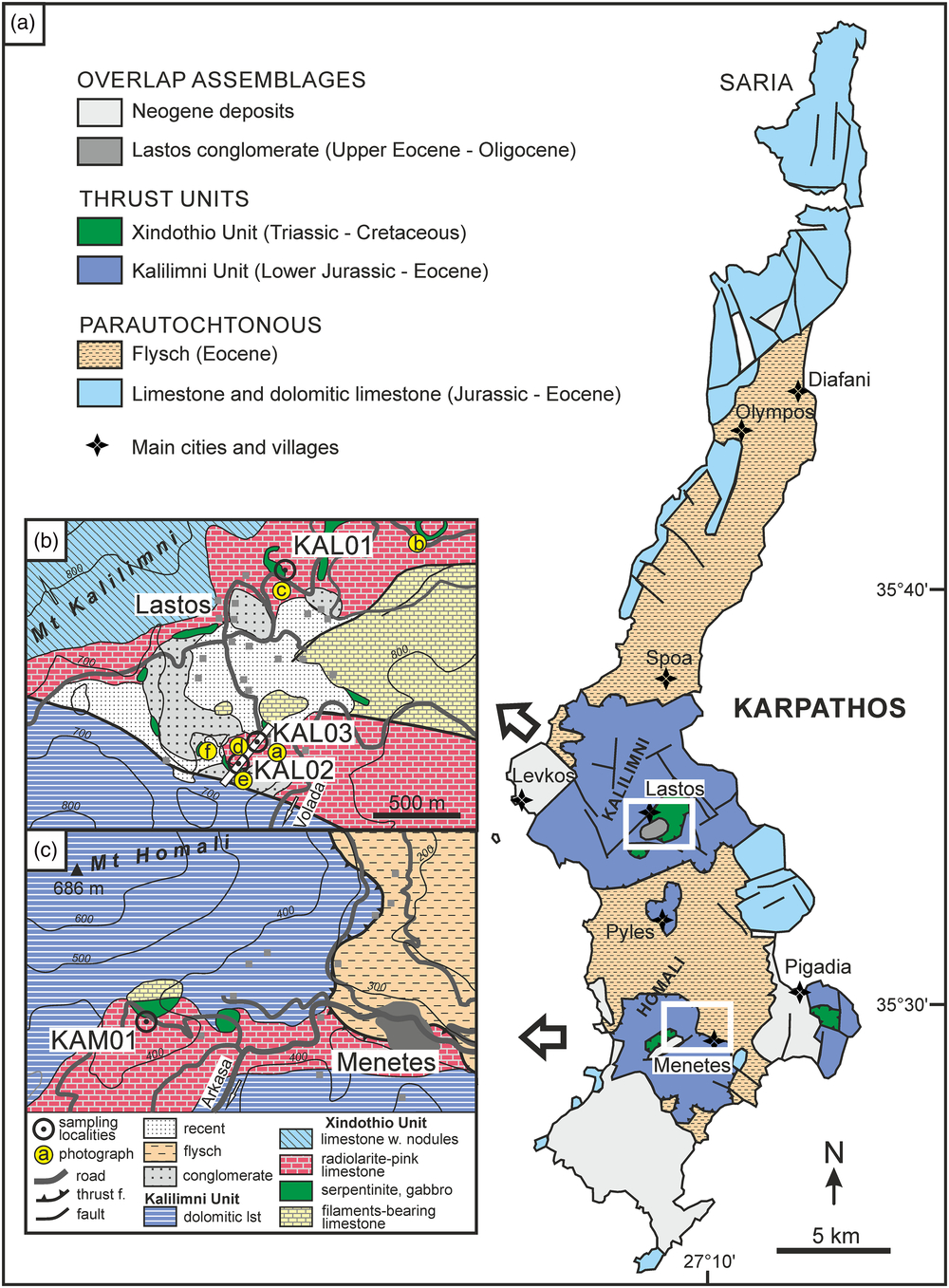
Fig. 2. (a) Geological and structural map of Karpathos (modified after Aubouin et al. Reference Aubouin, Bonneau and Davidson1976). (b) Geological map of the Lastos area and location of the radiolarian localities KAL01 (section ‘North Lastos road’), KAL02 and KAL03 (section ‘Hill 730 m’ outlined by a white band). Topography from the Karpathos–Kasos map sheet 1:30 000 (Psimenos et al. Reference Psimenos, Reppas, Mouzaki, Tassoula and Kodoni2017). Geology based on Christodoulou (Reference Christodoulou1968), Davidson-Monett (Reference Davidson-Monett1974), Aubouin et al. (Reference Aubouin, Bonneau and Davidson1976) and personal field observations. (c) Geological map of the Menetes area and location of the radiolarian locality KAM01. Topography from the Karpathos–Kasos map sheet 1:30 000 (Psimenos et al. Reference Psimenos, Reppas, Mouzaki, Tassoula and Kodoni2017). Geology from the Karpathos map sheet (Christodoulou, Reference Christodoulou1968).
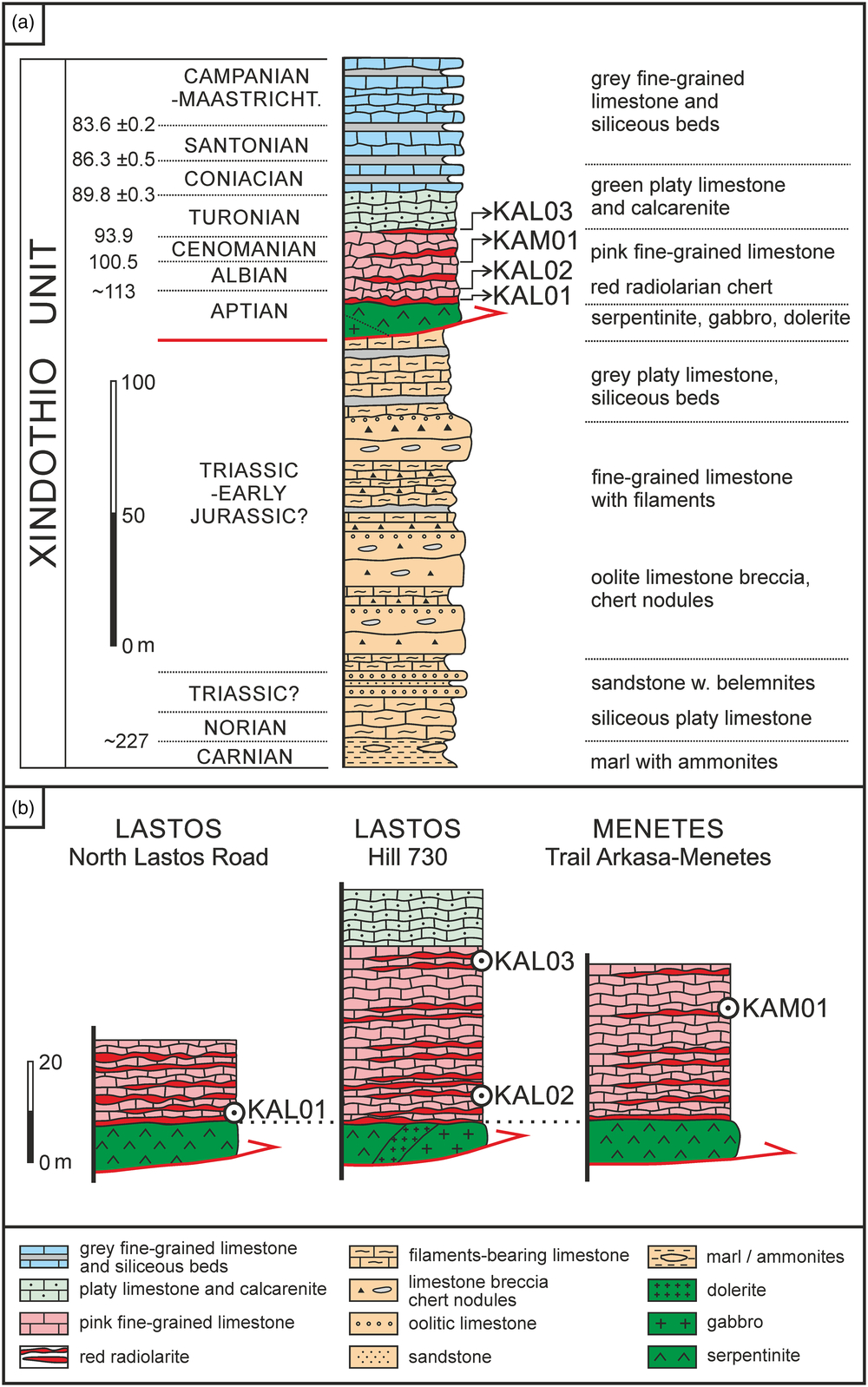
Fig. 3. (a) Synthetic lithostratigraphic column of the Xindothio Unit succession (modified after Aubouin et al. Reference Aubouin, Bonneau and Davidson1976). Basal radiolarites overlying mafic/ultramafic rocks are presently dated as Aptian (this study) instead of Berriasian (Aubouin et al. Reference Aubouin, Bonneau and Davidson1976). Isotopic ages are from Cohen et al. (Reference Cohen, Finney, Gibbard and Fan2013, updated 2018). Dotted lines (left): probable positions of stage boundaries based on biochronological data; red line: thrust fault at the base of mafic/ultramafic rocks; dotted lines (right): boundaries between subunits. (b) Sections in the Lastos and Menetes areas and stratigraphic positions of the radiolarian samples.
Mafic and ultramafic rocks are commonly found in tectonic contact with the underlying units, whereas the radiolarites depositionally overlie the ophiolite at many locations in the Lastos and Menetes areas (Davidson-Monett, Reference Davidson-Monett1974; Aubouin et al. Reference Aubouin, Bonneau and Davidson1976; Hatzipanagiotou, Reference Hatzipanagiotou1987, Reference Hatzipanagiotou1988) (Figs 2a–c, 3a). The depositional nature of this contact is supported by good lateral continuity of the radiolarites, which show no significant deformation. The rarity of basalts and the direct contact between the magmatic rocks and the radiolarites suggest that the Karpathos ophiolite formed by relatively slow spreading. Near the contact, red chert beds are 5–15 cm thick, whereas these beds are less abundant up-section and crop out as thin continuous layers or chert nodules. Overall, the radiolarite – pink-limestone subunit is c. 40 m thick (Figs 3a, 4).
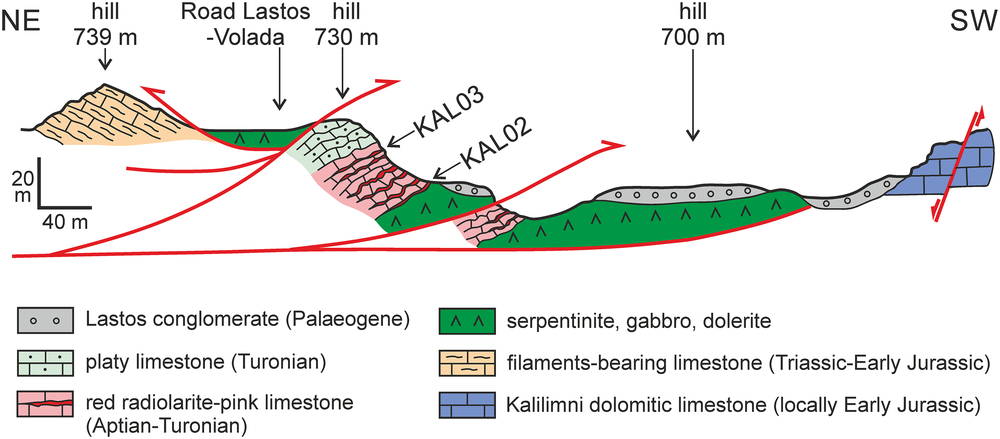
Fig. 4. Schematic cross-section of the south Lastos area including section ‘Hill 730 m’ and position of samples KAL02 and KAL03 close to the contact with mafic/ultramafic rocks (modified after Aubouin et al. Reference Aubouin, Bonneau and Davidson1976). Note that the Xindothio Unit is structurally situated above the dolomitic limestones of the Kalilimni Unit but underwent Cenozoic extension and normal faulting visible in the SW part of the section.
2.b. Previous ages of the ophiolite and overlying strata
Vinassa de Regny (Reference Vinassa de Regny1901) provided the first study of Karpathos radiolarians, which he observed within ‘phtanites’ (black cherts) and ‘argillaceous jaspers’ (red-maroon cherts) collected in the Lastos area (Fig. 2a, b). This pioneering work led to the description of 58 radiolarian morphotypes (online Supplementary Material at https://doi.org/10.1017/S0016756819000657), although most of the identified genera are now classified as nomen dubium (O’Dogherty et al. Reference O’Dogherty, Carter, Dumitrica, Goričan, De Wever, Bandini, Baumgartner and Matsuoka2009). In the light of current radiolarian taxonomy, some of the forms illustrated by Vinassa de Regny (Reference Vinassa de Regny1901) resemble the modern genera Archaeospongoprunum, Hiscocapsa, Paronaella, Pseudoeucyrtis, Pseudodictyomitra and Triactoma, but their occurrence is difficult to confirm given the discrepancies between 1900s drawings and present-day taxonomy based on three-dimensional scanning electron microscope (SEM) observations. Vinassa de Regny (Reference Vinassa de Regny1901) suggested that the faunal assemblage was possibly Late Cretaceous, although this age determination was tentative due to the absence of radiolarian biozonation at the time. Later, an age determination was obtained on the radiolarite – pink-limestone subunit with one calpionellid assemblage (Davidson-Monett, Reference Davidson-Monett1974; Aubouin et al. Reference Aubouin, Bonneau and Davidson1976) and was assigned to the middle–late Berriasian (145−139.8 Ma; Cohen et al. Reference Cohen, Finney, Gibbard and Fan2013, updated 2018). Consequently, these authors suggested that the ophiolite could be as old as Late Jurassic.
The K–Ar isotopic ages obtained later on the magmatic rocks (hornblende grains extracted from intrusive dolerites) are much younger than the overlying sedimentary strata (Table 1; Koepke et al. Reference Koepke, Kreuzer and Seidel1985, Reference Koepke, Seidel and Kreuzer2002; Hatzipanagiotou, Reference Hatzipanagiotou1991). In Lastos, two ages range between 84.7 and 79.6 Ma, encompassing the middle Santonian − middle Campanian interval (Cohen et al. Reference Cohen, Finney, Gibbard and Fan2013, updated 2018). In Menetes, the isotopic ages range between 99.5 and 88.4 Ma, corresponding to the middle Cenomanian − middle Coniacian interval.
Table 1. K–Ar isotopic ages in the Lastos and Menetes areas (Koepke et al. Reference Koepke, Seidel and Kreuzer2002) and correlation with geological stages (Cohen et al. Reference Cohen, Finney, Gibbard and Fan2013, updated 2018)

3. Studied sections and methods
Our study focused on identifying the contact between mafic/ultramafic rocks and the overlying radiolarian cherts in order to select a few localities for sampling. This contact is exposed in the high valley of Lastos on the eastern flank of Mount Kalilimni (Figs 2b, 5) and near the village of Menetes on the southern flank of Mount Homali (Fig. 2c). To maximize the quality of the faunas, we selected a variety of radiolarian samples in accordance with specific field techniques previously applied to suture zones and fore-arc belts (Cordey & Krauss, Reference Cordey and Krauss1990; Cordey & Cornée, Reference Cordey and Cornée2009). Radiolarians were then extracted by repetitive leaching of samples with low-concentration hydrofluoric acid (HF) and then hand-picked and mounted on aluminium stubs for SEM observation and taxonomical identifications (Tabletop SEM Phenom ProX, University of Lyon).

Fig. 5. Field views of the studied Karpathos units and localities (see Fig. 2b for locations of photographs). (a) Overview of section ‘Hill 730 m’ in Lastos (Figs 2b, 3b, 4) and subunits within the Xindothio Unit (Fig. 3); in the distance towards the west: parautochthonous limestone exposures of Kalilimni Unit; Mount Kalilimni (1215 m) is the highest point on the island. (b) Exposure of serpentinite (white arrows) overlain by the red radiolarite – pink-limestone subunit along the north Lastos road (Fig. 2b). White dashed line: contact between ultramafic and sedimentary rocks. White dotted lines: bedding of radiolarite and pink limestone, parallel to the contact between ultramafic and sedimentary rocks. (c) Close-up of the contact between serpentinite and radiolarite (dashed line) along the north Lastos road near locality KAL01 (Fig. 2b). (d) Pink limestone and chert nodules (white arrows) exposed in the middle part of section ‘Hill 730 m’ (total thickness 1 m). (e) Close-up of thick radiolarite beds at the base of section ‘Hill 730 m’ (Lastos, Figs 2b, 3, 4). (f) Exposure of the Lastos conglomerate (Eocene) displaying a variety of pebbles of radiolarite (white arrows), serpentinite (yellow arrows) and limestones from the underlying Kalilimni and Xindothio units.
In Lastos, our samples were collected from two sections:
1/ section ‘North Lastos road’ (Fig. 2b; Table 2). This section is composed of an outcrop of serpentinites depositionally overlain by radiolarian cherts and pink limestones. KAL01 was collected from a red radiolarite bed situated 30 cm above the serpentinite body (Fig. 3b). Similar stratigraphic contacts between serpentinites and radiolarites are visible at several locations along the North Lastos road (Figs 2b, 5b, c).
2/ section ‘Hill 730 m’ (Fig. 2b; Table 2). This section was previously described by Davidson-Monett (Reference Davidson-Monett1974) and Aubouin et al. (Reference Aubouin, Bonneau and Davidson1976) under the name ‘section of hill 738 m’ (Figs 3b, 4) and renamed to follow the revised topography available on the Karpathos–Kasos map sheet (Psimenos et al. Reference Psimenos, Reppas, Mouzaki, Tassoula and Kodoni2017). Sample KAL02 is from a bed of red radiolarian chert at the base of the radiolarite – pink-limestone subunit located just above the contact with gabbros and serpentinites (Figs 3b, 5a, e). Sample KAL03 is from a red nodular radiolarian chert bed and was collected at the top of the radiolarite – pink-limestone subunit (Figs 3b, 5d).
Table 2. Geographic coordinates of radiolarian samples from Karpathos

Near Menetes, our sample locality KAM01 (Fig. 2c; Table 2) is from a bed of radiolarite interbedded with pink limestones, 150 m to the south of a well-exposed serpentinite outcrop at the foot of Mount Homali (Fig. 3b).
4. Results
The preservation of radiolarians extracted from our chert samples varies from moderate to good at both the Lastos and Menetes locations. All of the radiolarian taxa and assemblages identified in the samples are listed in Table 3. Morphotypes with biochronological significance are illustrated in Figure 6.
Table 3. Radiolarian taxa and assemblages from Karpathos


Fig. 6. Scanning electron microphotographs (SEM) of radiolarian taxa from Lastos (KAL) and Menetes (KAM). For each sample: age and radiolarian assemblage. For each picture: radiolarian taxon, scale length.
- Sample KAL03 (Turonian); 1. Pseudodictyomitra pseudomacrocephala (SQUINABOL) (210 μm); 2. Dictyomitra napaensis PESSAGNO (200 μm); 3. Dictyomitra montisserei (SQUINABOL) (220 μm); 4. Stichomitra communis (SQUINABOL) (190 μm); 5. Afens sp. (200 μm); 6. Crucella cachensis PESSAGNO (180 μm); 7. Patellula verteroensis (PESSAGNO) (160 μm).
- Sample KAL01 (Aptian); 8. Cenodiscaella sphaeroconus (RÜST) (200 μm); 9. Homoeoparonaella sp. cf. speciosa (PARONA) (320 μm); 10. Crucella messinae PESSAGNO (240 μm); 11. Crucella euganea SQUINABOL (240 μm); 12. Ultranapora sp. cf. durhami PESSAGNO (140 μm); 13. Pseudodictyomitra lodogaensis PESSAGNO (180 μm); 14. Dictyomitra montisserei (SQUINABOL) (180 μm); 15. Xitus sp. aff. spicularius (ALIEV) (210 μm); 16. Archaeodictyomitra gracilis (SQUINABOL) (200 μm); 17. Archaeodictyomitra gracilis (SQUINABOL) (180 μm); 18. Hiscocapsa asseni (TAN) (120 μm); 19. Hiscocapsa grutterinki (TAN) (150 μm).
- Sample KAL02 (early−middle Albian); 20. Obeliscoites sp. cf. perspicuus (SQUINABOL) (180 μm); 21. Xitus spicularius (ALIEV) (190 μm); 22. Dactyliosphaera lepta FOREMAN (200 μm); 23. Dactyliosphaera sp. cf. lepta FOREMAN (200 μm); 24. Mesosaturnalis sp. (190 μm); 25. Hexapyramis sp. cf. precedis JUD (190 μm); 26. Cyclastrum satoi (TUMANDA) (180 μm).
- Sample KAM01 (middle Albian); 27. Cenodiscaella tuberculatum (DUMITRICA) (160 μm); 28. Staurosphaeretta sp. (130 μm); 29. Thanarla brouweri (TAN) (120 μm); 30. Archaeospondoprumum renaensis PESSAGNO (180 μm); 31. Distylocapsa micropora (SQUINABOL) (180 μm).
KAL01 comprises 16 identified taxa (Table 3). Among them, an assemblage composed of Archaeodictyomitra gracilis, Crucella euganea, Crucella messinae, Hiscocapsa asseni, Hiscocapsa grutterinki and Pseudodictyomitra lodogaensis corresponds to the unitary associations zones (UAZ) 4−9 of O’Dogherty (Reference O’Dogherty1994) of early–late Aptian age. Other Early Cretaceous species include Cenodiscaella sphaeroconus, Homoeoparonaella sp. cf. speciosa, Mesosaturnalis sp., Paronaella sp., Pseudocrucella sp. aff. kubischa, Pseudoeucyrtis(?) fusus, Stichomitra communis, Ultranapora sp. cf. durhami and Xitus sp. aff. spicularius. Among them, Stichomitra communis is known from the Aptian−Turonian interval (UAZ 42−46; Goričan, Reference Goričan1994). We interpret the morphotype illustrated in Figure 6 (no. 14) as Dictyomitra montisserei, which we consider to have a slightly longer age range than previously proposed (UAZ 10−20 of Albian−Turonian age; O’Dogherty, Reference O’Dogherty1994).
KAL02 is composed of eight radiolarian taxa (Table 3). The co-occurrence of Crucella messinae, Cyclastrum satoi, Dactyliosphaera lepta, Hexapyramis sp. cf. precedis, Mesosaturnalis levis and Xitus spicularius points to UAZ 11−12 of early–middle Albian age (O’Dogherty, Reference O’Dogherty1994). Some other taxa have longer age ranges, such as Alievium sp. (Bajocian–Maastrichtian, O’Dogherty et al. Reference O’Dogherty, Carter, Dumitrica, Goričan, De Wever, Bandini, Baumgartner and Matsuoka2009) and Obeliscoites sp. cf. perspicuus (UAZ 1−19, Barremian–Cenomanian; O’Dogherty, Reference O’Dogherty1994).
KAL03 comprises ten radiolarian taxa (Table 3). Alievum sp. cf. superbum, Crucella cachensis and Dictyomitra napaensis are known as Turonian (Pessagno, Reference Pessagno1976). Other taxa have been found to co-occur in Turonian biozones, such as Stichomitra communis (UAZ 42−48 of late Aptian–Turonian; Goričan, Reference Goričan1994), Dictyomitra montisserei (UAZ 10−20, Albian–Turonian; O’Dogherty, Reference O’Dogherty1994), Pseudodictyomitra pseudomacrocephala (UAZ 10−21, Albian–Turonian; O’Dogherty, Reference O’Dogherty1994) and Afens sp. (Turonian–Campanian; O’Dogherty et al. Reference O’Dogherty, Carter, Dumitrica, Goričan, De Wever, Bandini, Baumgartner and Matsuoka2009).
KAM01 contains seven radiolarian taxa, including species reported from the biozonation of O’Dogherty (Reference O’Dogherty1994), such as Distylocapsa micropora (UAZ 10−18) and Thanarla brouweri (UAZ 1−12), corresponding to a middle Albian age. Other taxa are Archaeospongoprunum renaensis (Albian; Pessagno, Reference Pessagno1977), Cenodiscaella tuberculatum (early Cenomanian − ?early Turonian; Pessagno, Reference Pessagno1977), Staurosphaeretta sp. (late Aptian − early Turonian; O’Dogherty et al. Reference O’Dogherty, Carter, Dumitrica, Goričan, De Wever, Bandini, Baumgartner and Matsuoka2009), Pseudodictyomitra lodogaensis (UAZ 42−46, late Aptian − late Albian; Goričan, Reference Goričan1994) and Triactoma sp. (early Pliensbachian − late Turonian; O’Dogherty et al. Reference O’Dogherty, Carter, Dumitrica, Goričan, De Wever, Bandini, Baumgartner and Matsuoka2009).
In summary, the radiolarian ages established for the radiolarite – pink-limestone subunit of the Xindothio Unit are, from base to top: early–late Aptian (KAL01; ∼125−113 Ma; Cohen et al. Reference Cohen, Finney, Gibbard and Fan2013, updated 2018), early–middle Albian (KAL02; ∼113−105 Ma), middle Albian (KAM01; ∼110−105 Ma) and Turonian (KAL03; ∼93.9−89.8 Ma) (Fig. 3a, b).
5. Discussion
5.a. Comparison with previous microfossil ages
The three Aptian and Albian radiolarian ages (∼125−105 Ma) obtained at the base of the radiolarite – pink-limestone subunit at Lastos (KAL01, KAL02) and Menetes (KAM01) are significantly younger than the Berriasian age (145−139.8 Ma) previously reported from the same sequence (Davidson-Monett, Reference Davidson-Monett1974; Aubouin et al. Reference Aubouin, Bonneau and Davidson1976). A similar issue applied on Rhodes, where Danelian et al. (Reference Danelian, Bonneau, Cadet, Poisson and Vrielynck2001) established that Berriasian calpionellids from the Profitis Ilias succession had been misidentified. This situation could also be the case in Karpathos. Another hypothesis is that these calpionellids have been reworked. In any case, based on results obtained in three sections in two separate areas (Lastos and Menetes; Fig. 3a, b), our data show that the radiolarites of the Xindothio Unit are not Berriasian but rather Aptian–Albian.
Our younger radiolarian assemblage is Turonian (∼93.9−89.8 Ma) and was obtained at the top of the radiolarite – pink-limestone subunit (KAL03). Foraminifera of similar age were reported from the base of the platy limestone subunit (Davidson-Monett, Reference Davidson-Monett1974; Aubouin et al. Reference Aubouin, Bonneau and Davidson1976).
5.b. Comparison with K–Ar isotopic ages
K–Ar isotopic ages of Koepke et al. (Reference Koepke, Seidel and Kreuzer2002) are shown in Table 1. At Menetes, the age difference with the radiolarian data is minor, as the oldest isotopic age of 95.3 ± 4.2 Ma fits into the early Cenomanian (Cohen et al. Reference Cohen, Finney, Gibbard and Fan2013, updated 2018). At Lastos, the age difference is greater: while radiolarians near the contact are Aptian, the oldest isotopic age is middle Santonian (84.7 Ma, Table 1). To explain such a discrepancy, four hypotheses are considered below:
1/ Aptian–Albian radiolarians were reworked and redeposited in younger Late Cretaceous sediments. This process seems unlikely since the radiolarian assemblages do not show any unusual mixing of incompatible taxa (Table 3). These assemblages also document a coherent succession of upward-younging ages (Aptian → early–middle Albian → middle Albian → Turonian; Fig. 3a). In addition, the Turonian ages obtained independently with planktonic foraminifera (Aubouin et al. Reference Aubouin, Bonneau and Davidson1976) and radiolarians (this study) near the contact between radiolarite – pink-limestone and platy limestone subunits are both older than the K–Ar isotopic ages of Koepke et al. (Reference Koepke, Seidel and Kreuzer2002).
2/ The contact between mafic/ultramafic rocks and radiolarites is tectonic. However, this hypothesis is not consistent with our field observations or those of Davidson-Monett (Reference Davidson-Monett1974), Aubouin et al. (Reference Aubouin, Bonneau and Davidson1976) and Hatzipanagiotou (Reference Hatzipanagiotou1988). The Xindothio Unit is not an accretionary-type ‘broken formation’ where subunits are chaotically juxtaposed. Instead, it shows good reproducibility of the stratigraphic contact between the magmatic rocks and the overlying radiolarian cherts, not only at Lastos but also at Menetes (Fig. 2b, c). In addition, there is good lateral continuity of the contact as shown by the parallel bedding of the radiolarite – pink-limestone subunit along strike (Fig. 5b, c). One could argue that the Karpathos ophiolite is dismembered and that mafic and ultramafic rocks may not be of the same age as the overlying strata, but in a dismembered or mélange-type configuration the radiolarian-bearing sedimentary matrix should be closely synchronous with or younger than any such olistoliths, not older (Cordey, Reference Cordey1998).
3/ The Karpathos ophiolite has a longer age range than previously envisaged, i.e. spanning the Early–Late Cretaceous, more precisely the Aptian–Santonian (from ∼125−113 Ma to 84.7 Ma), representing the interval between the radiolarites overlying the ophiolite and the oldest isotopic age in Lastos. In this case, the Xindothio radiolarites should be diachronous. Considering the consistent Early Cretaceous ages from Lastos and Menetes areas (this study), as well as the relatively small volumes of mafic and ultramafic rocks exposed on the island (Fig. 2a–c), we do not favour this hypothesis.
4/ Isotopic ages from Lastos have undergone some metamorphic overprint or are affected by argon loss. This is, to us, the most likely cause of the chronological discrepancy, as both phenomena can cause calculated K–Ar ages to be younger than the true ages of the dated material (McDougall & Harrison, Reference McDougall and Harrison1999). Similarly, it has been established that some of the youngest Cretan ophiolites underwent such overprinting during the Late Cretaceous (95 Ma; Koepke et al. Reference Koepke, Seidel and Kreuzer2002). Other authors have also shown that some Cretan isotopic ages record the age of obduction rather than oceanic crust formation (Liati et al. Reference Liati, Gebauer and Fanning2004; G. Stampfli, E. Champod, A. Vandelli, unpub. field guide, University of Lausanne, 2010). In Turkey, Ar–Ar isotopic ages of the metamorphic soles of the Lycian peridotites are mainly 92−90 Ma (Dilek et al. Reference Dilek, Thy, Hacker and Grundvig1999), an age range that is similar to the K–Ar isotopic ages on Karpathos. More recently, U–Pb ages on zircons from the metamorphic sole of the Marmaris ophiolite (Lycian Nappes) have shown that their tectonic emplacement occurred between 100.4 Ma and 93.5 Ma (Cenomanian), suggesting an Early Cretaceous age for the ophiolite and the associated sedimentary rocks (Güngör et al. Reference Güngör, Akal, Özer, Hasözbek, Sarı and Mertz-Kraus2018). Unfortunately, previous geochronological studies carried out on Karpathos (Koepke et al. Reference Koepke, Kreuzer and Seidel1985; Hatzipanagiotou, Reference Hatzipanagiotou1991; Koepke et al. Reference Koepke, Seidel and Kreuzer2002) did not document the precise locations for the K–Ar samples or indicate whether the magmatic rocks were overlain by sedimentary strata, preventing comparative sampling of sections.
We do not know to what extent the discrepancy between biochronological and geochronological data observed on Karpathos is a localized problem or a larger issue concerning Mesozoic ophiolites. Some isotopic ages established on these ophiolites using K–Ar methods in the 1980s and 1990s probably need to be tested with more up-to-date methods such as Ar–Ar and/or U–Pb, as carried out by Liati et al. (Reference Liati, Gebauer and Fanning2004) and Smith (Reference Smith2006), who obtained zircon ages on hornblendites and gabbros from Cretan ophiolites, and Parlak et al. (Reference Parlak, Karaoglan, Rizaoglu, Klötzli, Koller and Billor2013) who used hornblendes and zircons from ophiolites of the Tauride belt.
6. Eastern Mediterranean correlations and implications for Neotethys evolution
Tectonic reconstructions of the Eastern Mediterranean region imply the southward migration of the Aegean fore-arc relative to Eurasia since Eocene times, a result of the rollback of subducting African lithosphere (Zachariasse et al. Reference Zachariasse, Van Hinsbergen and Fortuin2008; Jolivet et al. Reference Jolivet, Faccenna, Huet, Labrousse, Le Pourhiet, Lacombe, Lecomte, Burov, Denèle, Brun, Philippon, Paul, Salaün, Karabulut, Piromallo, Monié, Gueydan, Okay, Oberhänsli, Pourteau, Augier, Gadenne and Driussi2013). Prior to the Palaeogene, the palaeogeographic evolution of the region has been described by many authors at the scale of the Tethyan Realm (Robertson, Reference Robertson2002; Barrier & Vrielynck, Reference Barrier and Vrielynck2008; Menant et al. Reference Menant, Jolivet and Vrielynck2016; Barrier et al. Reference Barrier, Vrielynck, Brouillet and Brunet2018), but these descriptions lack precision for the islands of the Aegean Sea. Several hypotheses have been proposed for the Karpathos ophiolite: initially, it was correlated with the ophiolites of Crete, both of which were considered the easternmost remnants of the Pindos Nappes of continental Greece and the Peloponnese (Figs 1, 7; Aubouin et al. Reference Aubouin, Bonneau and Davidson1976; van Hinsbergen et al. Reference Van Hinsbergen, Zachariasse, Wortel and Meulenkamp2005; Papanikolaou, Reference Papanikolaou2013; Ersoy et al. Reference Ersoy, Cemen, Helvaci and Billor2014; Pantopoulos & Zelilidis, Reference Pantopoulos and Zelilidis2014). Other authors have interpreted the Karpathos and Rhodes ophiolites as southwestward extensions of the Lycian Nappes exposed in SW Turkey (Fig. 1; Koepke et al. Reference Koepke, Seidel and Kreuzer2002; Robertson, Reference Robertson2002; van Hinsbergen et al. Reference Van Hinsbergen, Kaymakçi, Spakman and Torsvik2010). The ophiolites of Karpathos and Rhodes have also been associated with the Late Cretaceous ophiolites of Cyprus, Syria and Oman (Koepke et al. Reference Koepke, Seidel and Kreuzer2002), hence as potential remnants of a basin located to the south of Turkey, in a precursor position of the present-day Eastern Mediterranean Basin. In summary, these conflicting interpretations consider the Karpathos ophiolite as an element of the western Northern Neotethys (Pindos Zone), the central Northern Neotethys (Izmir–Ankara–Erzincan suture and Lycian Nappes), or the Southern Neotethys (Cyprus–Syria) (Fig. 7).
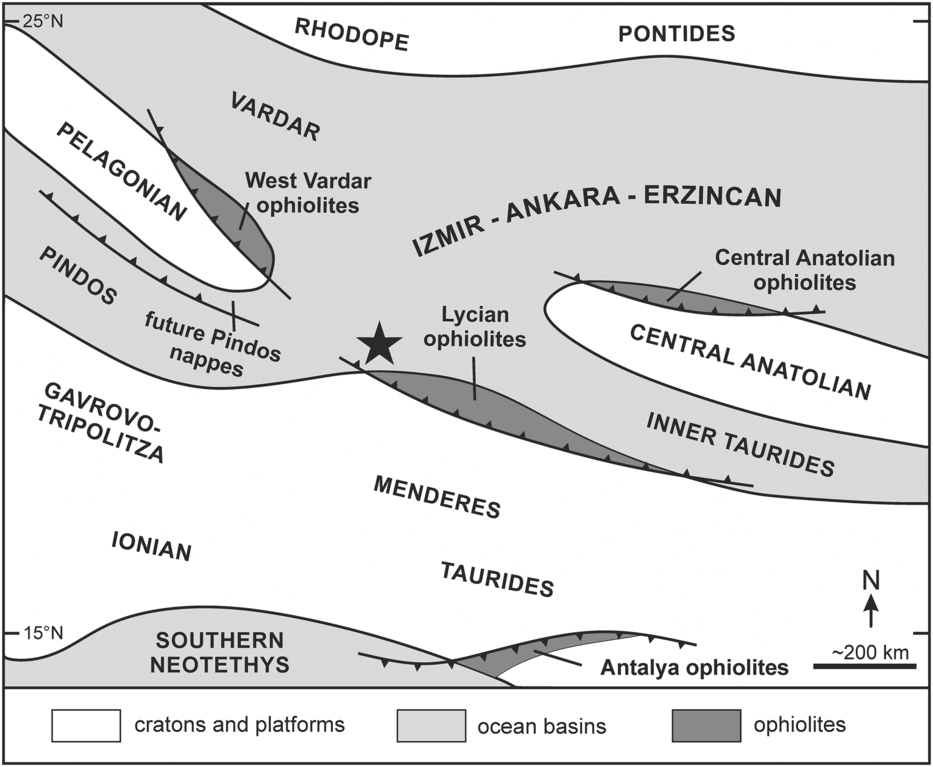
Fig. 7. Schematic palaeogeographic map of western Neotethyan basins in the Cretaceous. Compiled from Barrier & Vrielynck (Reference Barrier and Vrielynck2008), Robertson et al. (Reference Robertson, Parlak and Ustaömer2012), Menant et al. (Reference Menant, Jolivet and Vrielynck2016), Barrier et al. (Reference Barrier, Vrielynck, Brouillet and Brunet2018), Maffione & van Hinsbergen (Reference Maffione and van Hinsbergen2018). Star: hypothetical position of Karpathos ophiolite prior to obduction. Note that the ophiolites and the tectonic nappes depicted in the diagram have been emplaced diachronously: they just indicate the initial locations and relationships to the surrounding oceanic basins.
A geological review of ophiolites exposed in the Aegean fore-arc (Fig. 8a) shows that the ophiolite of Karpathos is younger than those of Crete, where K–Ar isotopic ages range from 160 to 140 Ma (Middle–Late Jurassic) (Koepke et al. Reference Koepke, Seidel and Kreuzer2002; Liati et al. Reference Liati, Gebauer and Fanning2004). Notably, the youngest Cretan radiolarites are Late Jurassic in age (G. Stampfli, E. Champod, A. Vandelli, unpub. field guide, University of Lausanne, 2010), similar to the Pindos radiolarites of continental Greece (De Wever & Cordey, Reference De Wever and Cordey1986). Another significant difference is that, on Karpathos, a shallow marine Upper Eocene conglomerate overlies the dismembered ophiolite together with the Mesozoic limestones (Kalilimni Unit) (Figs 2b, 5f), showing that these units were already tectonically assembled while flysch deposition was still continuing in the Pindos Basin farther west.

Fig. 8. (a) Map of the Aegean region prior to the complete southward migration of the Aegean fore-arc during the Neogene (modified after Garfunkel, Reference Garfunkel2004). The map shows the position of the ophiolites and the extensions of the Pindos and Lycian nappes, which have been proposed as potential sources for the ophiolite-bearing units of Crete, Karpathos and Rhodes. A–B: cross-section shown in (b). Isotopic ages, Crete: Koepke et al. (Reference Koepke, Seidel and Kreuzer2002); Liati et al. (Reference Liati, Gebauer and Fanning2004); Karpathos and Rhodes: Koepke, Seidel & Kreuzer (Reference Koepke, Seidel and Kreuzer2002). SW Turkey: Dilek et al. (Reference Dilek, Thy, Hacker and Grundvig1999); Güngör et al. (Reference Güngör, Akal, Özer, Hasözbek, Sarı and Mertz-Kraus2018) (see references for age ranges, error margins and local distribution). Age of radiolarites, Turkey: Danelian et al. (Reference Danelian, Robertson, Collins and Poisson2006); Karpathos: this study. IAE: Izmir–Ankara–Erzincan Suture Zone. (b) Cross-section A–B (Fig. 8a) from the Eastern Mediterranean Basin to the Izmir–Ankara–Erzincan Suture Zone (modified after van Hinsbergen et al. Reference Van Hinsbergen, Kaymakçi, Spakman and Torsvik2010), illustrating the southward thrusting of the Lycian Nappes and related ophiolites over Rhodes and Karpathos.
Our results indicate that the closest chronological and petrological similarities with the ophiolite of Karpathos are found in SW Turkey, more specifically within the Lycian Nappes (de Graciansky, Reference de Graciansky1967; Collins & Robertson, Reference Collins and Robertson1997, Reference Collins and Robertson2003; Dilek et al. Reference Dilek, Thy, Hacker and Grundvig1999; Robertson, Reference Robertson2002). In the Lycian Mélange, Danelian et al. (Reference Danelian, Robertson, Collins and Poisson2006) documented Jurassic and Early Cretaceous radiolarian cherts. In the Marmaris ophiolite, the youngest sediments associated with the ophiolite are pre-Cenomanian (Güngör et al. Reference Güngör, Akal, Özer, Hasözbek, Sarı and Mertz-Kraus2018).
Many authors have established a link between the Lycian Nappes and the Izmir–Ankara–Erzincan Suture Zone situated to the north of the Menderes metamorphic complex (Fig. 8a) (Robertson & Pickett, Reference Robertson, Pickett, Bozkurt, Winchester and Piper2000; Okay et al. Reference Okay, Tansel and Tüysüz2001; Collins & Robertson, Reference Collins and Robertson2003; Robertson et al. Reference Robertson, Ustaömer, Pickett, Collins, Andrew and Dixon2004). The Izmir–Ankara–Erzincan Suture Zone is itself considered a remnant of the Northern Neotethyan Ocean (Danelian et al. Reference Danelian, Robertson, Collins and Poisson2006) and records a long history of radiolarite accumulation. In the Central Sakarya Zone, the Dağküplü Mélange includes several slices of mid-ocean ridge metabasalts (MORB-type) that alternate with radiolarites dated to the Middle–Late Jurassic (Bathonian–Tithonian), Early Cretaceous (Hauterivian–Aptian) and early Late Cretaceous (Cenomanian) (Göncüoğlu et al. Reference Göncüoğlu, Turhan, Sentürk, Özcan, Uysal, Yaliniz, Bozkurt, Winchester and Piper2000, Reference Göncüoğlu, Yaliniz, Tekin and Turhan2001). Farther east in the Ankara Mélange, blocks of radiolarite associated with gabbro and pink micritic limestone are dated as Late Triassic, Early Jurassic, Late Jurassic and Early Cretaceous, including Aptian and Albian strata such as on Karpathos (Bragin & Tekin, Reference Bragin and Tekin1996). As in the Dağküplü Mélange, the youngest radiolarites of the Ankara Mélange are Cenomanian (Bragin & Tekin, Reference Bragin and Tekin1996). Previous authors noted great similarities in both petrography and trace elements between Karpathos and Rhodes mafic and ultramafic rocks and those of western Turkey, emphasizing the depleted nature of the peridotites and the geochemistry of dykes that are typical of supra-subduction zone ophiolites (Koepke et al. Reference Koepke, Seidel and Kreuzer2002).
Farther south in the Antalya Complex, MOR-type (mid-ocean ridge) volcanic rocks are overlain by Late Jurassic − Early Cretaceous radiolarian cherts (Robertson & Woodcock, Reference Robertson and Woodcock1982; Yilmaz, Reference Yılmaz, Dixon and Robertson1984; Robertson, Reference Robertson2002), partly coeval with Karpathos radiolarites. However, the Antalya Complex records a distinct geological history and tectonic setting of an oceanic domain located to the south of the Tauride carbonate platform (Fig. 7; Barrier & Vrielynck, Reference Barrier and Vrielynck2008; Robertson et al. Reference Robertson, Parlak and Ustaömer2012; Barrier et al. Reference Barrier, Vrielynck, Brouillet and Brunet2018).
The ophiolites of Karpathos and Rhodes have also been correlated with the Late Cretaceous ophiolites of Cyprus, Syria and Oman (Koepke et al. Reference Koepke, Seidel and Kreuzer2002) and hence interpreted as potential remnants of the Southern Neotethys (Fig. 7). However, this hypothesis is not supported by the tectonic interpretations that imply that the emplacement of the Karpathos and Rhodes ophiolites proceeded southward during the closure of a basin located to the north of Turkey (van Hinsbergen et al. Reference Van Hinsbergen, Kaymakçi, Spakman and Torsvik2010; Schettino & Turco, Reference Schettino and Turco2011).
In summary, the comparison of these various interpretations suggests that the Karpathos ophiolite originated in the central Northern Neotethys (Fig. 7; Dilek et al. Reference Dilek, Thy, Hacker and Grundvig1999; Robertson, Reference Robertson2002; Barrier & Vrielynck, Reference Barrier and Vrielynck2008; Menant et al. Reference Menant, Jolivet and Vrielynck2016; Barrier et al. Reference Barrier, Vrielynck, Brouillet and Brunet2018) and is linked to the Lycian Nappes and their root, the Izmir–Ankara–Erzincan Suture Zone. A slight difference lies in the age of the youngest radiolarites between these units: while Karpathos radiolarites are Aptian–Albian (125−100.5 Ma; Cohen et al. Reference Cohen, Finney, Gibbard and Fan2013, updated 2018), the youngest radiolarites of the Lycian Nappes and the Izmir–Ankara–Erzincan Suture Zone are consistently Cenomanian (100.5−93.9 Ma). In addition, the age range represented by the ophiolite is much shorter on Karpathos (late Early Cretaceous). This suggests that the Karpathos ophiolite may be a remnant of a short-lived segment of the Northern Neotethys that was originally located in the westernmost part of the Izmir–Ankara–Erzincan Suture Zone (Figs 7, 8). The convergence and closure of the Northern Neotethys during the Palaeogene then led to the thrusting of ophiolite-bearing tectonic slices over the Karpathos parautochthonous carbonate platform, similar to the Lycian Nappes over Rhodes (Fig. 8b). Eventually, the southward movement of the Aegean arc during the Neogene distorted the original Palaeogene configuration, separating Karpathos farther from the ancient Izmir–Ankara–Erzincan root zone.
7. Conclusions
Our study documents the occurrence of Early Cretaceous radiolarians in sedimentary rocks overlying mafic and ultramafic rocks on the island of Karpathos, with the following implications:
The older radiolarian assemblage provides a minimum Aptian age (∼125−113 Ma) for the ophiolite, while the younger assemblage indicates that the top of the radiolarite – pink-limestone subunit is Turonian (∼93.9−89.8 Ma). The Aptian and Albian radiolarian ages raise questions regarding the accuracy of a Berriasian age obtained previously from a single calpionellid assemblage in the same subunit.
The oldest radiolarian ages (late Early Cretaceous) are significantly older than those proposed for the ophiolite using K–Ar geochronology (Late Cretaceous, from 95.3 ± 4.2 Ma to 81.2 ± 1.6 Ma), suggesting that these isotopic ages may have been reset by Late Cretaceous metamorphism, or were affected by argon loss.
The Karpathos ophiolite should not be associated with the Pindos Nappes or the ophiolites of Cyprus or Syria but rather with the Lycian Nappes and their root located in the Izmir–Ankara–Erzincan Suture Zone, possibly representing the westernmost part of this structure. Consequently, the ophiolite of Karpathos is likely to be a remnant of the Northern Neotethys, not of the Pindos Ocean or the proto-Eastern Mediterranean Basin.
From an analytical viewpoint, our study suggests that previous K–Ar isotopic ages obtained from the ophiolites of the Mediterranean region should be revised with more recent and accurate methods (Ar–Ar, U–Pb), particularly for geological units that have been affected by syn- or post-emplacement metamorphism and deformation.
Author ORCIDs
Fabrice Cordey 0000-0002-3800-4037
Acknowledgements
This study was funded by the Laboratory of Geology of Lyon (LGLTPE, UMR5276) and by the National Research programme Tellus-INTERRVIE of the French CNRS-INSU (Centre National de la Recherche Scientifique – Institut National des Sciences de l’Univers). The authors are grateful to the editor Peter D Clift as well as Alastair HF Robertson and an anonymous reviewer for their very constructive comments concerning previous versions of the manuscript. Geological mapping by John Davidson in the 1970s was a valuable source of information. The authors also wish to thank Jean-Jacques Cornée, Sébastien Joannin (University of Montpellier) and Pierre Moissette (University of Athens) for our discussions on the geology of Karpathos and the Aegean fore-arc.
Declaration of Interest
None
Supplementary Material
To view supplementary material for this article, please visit https://doi.org/10.1017/S0016756819000657.