1. Introduction
The accurate identification of ancient orogenic/active zones within Neoarchaean–Palaeoproterozoic cratons and understanding their tectonic settings are matters of great scientific significance because they can provide insights into the formation and evolution of ancient cratons and the geodynamic processes of continental plate convergence and dispersal (Zhai & Santosh, Reference Zhai and Santosh2011; Zhao et al. Reference Zhao, Cawood, Li, Wilde, Sun, Zhang, He and Yin2012). The North China Craton (NCC) is the largest and oldest craton in China, containing ancient zircons and/or rocks as old as 3.8 Ga (Liu et al. Reference Liu, Nutman, Compston, Wu and Shen1992; Song et al. Reference Song, Nutman, Liu and Wu1996; Wan et al. Reference Wan, Song, Liu, Li, Yang, Zhang, Yang, Geng and Shen2001, Reference Wan, Liu, Song, Wu, Yang, Zhang and Geng2005; Liu, Wan & Wu, Reference Liu, Wan, Wu, Van Kranendonk, Smithies and Bennett2007), and it has been a hotspot for research into the early Precambrian period (Shen et al. Reference Shen, Geng, Song and Wan2005; Geng, Shen & Ren, Reference Geng, Shen and Ren2010; Nutman et al. Reference Nutman, Wan, Du, Friend, Dong, Xie, Wang, Sun and Liu2011; Sun et al. Reference Sun, Yang, Wu and Wilde2012; Zhang et al. Reference Zhang, Zhao, Shen, Li, Sun, Chan and Liu2012; Zhao & Zhai, Reference Zhao and Zhai2013; Zheng, Xiao & Zhao, Reference Zheng, Xiao and Zhao2013). In the past decade, a great deal of petrology, elemental and isotopic geochemistry, structural geology and, in particular, isotope chronology has been undertaken on the NCC, and there is increasing agreement that: (1) the lower Precambrian basement of the NCC consists of Eoarchaean to Palaeoarchaean (3.8–3.2 Ga), Mesoarchaean (3.2–2.8 Ga), Neoarchaean (2.8–2.5 Ga) and Palaeoproterozoic (2.5–1.8 Ga) metamorphic complexes that are covered by unmetamorphosed Mesoproterozoic caprocks (Bai, Reference Bai1993; Wu et al. Reference Wu, Geng, Shen, Wan, Liu and Song1998; Zhai, Bian & Zhao, Reference Zhai, Bian and Zhao2000; Zhai, Guo & Liu, Reference Zhai, Guo and Liu2005; Santosh, Sajeev & Li, Reference Santosh, Sajeev and Li2006; Santosh, Reference Santosh2010; Zhai et al. Reference Zhai, Li, Peng, Hu, Liu, Zhang, Guo, Kusky, Zhai and Xiao2010); and (2) the formation and evolution of this craton can be related to three major Palaeoproterozoic mobile belts, that is the Khondalite Belt, the Trans-North China Orogen and the Jiao–Liao–Ji Belt (Zhao et al. Reference Zhao, Wilde, Cawood and Sun2001, Reference Zhao, Sun, Wilde and Li2005), alternatively named the Liaoji, Jinyu and Fengzhen belts (Zhai & Peng, Reference Zhai and Peng2007; Zhai & Santosh, Reference Zhai and Santosh2011, Reference Zhai and Santosh2013), which tectonically divided the NCC further into four sub-blocks (the Yinshan, Ordos, Longgang and Langrim). Although many controversies remain, there is increasing agreement that the Khondalite Belt and Trans-North China Orogen represented two Himalaya-type continent–continent collisional belts, of which the formation of the Khondalite Belt between the Yinshan and Ordos blocks led to creation of the Western Block at 1.95 Ga (Zhao et al. Reference Zhao, Sun, Wilde and Li2003, Reference Zhao, Wilde, Guo, Cawood, Sun and Li2010; Xia et al. Reference Xia, Sun, Zhao and Luo2006a ,Reference Xia, Sun, Zhao, Wu, Xu, Zhang and Luo b , Reference Xia, Sun, Zhao, Wu, Xu and Zhang2008, Reference Xia, Sun, Zhao, Wu and Xie2009; Yin et al. Reference Yin, Zhao, Sun, Xia, Wei, Zhou and Leung2009, Reference Yin, Zhao, Guo, Sun, Xia, Zhou and Liu2011, Reference Yin, Zhao, Wei, Sun, Guo and Zhou2014; Zhao, Reference Zhao2009; Li et al. Reference Li, Zhao, Santosh, Liu and Dai2011; Wang et al. Reference Wang, Lu, Chu, Xiang, Zhang and Liu2011), the formation of the Jiao–Liao–Ji Belt between the Longgang and Langrim blocks gave rise to the Eastern Block, and the Western and Eastern blocks were then united along the Trans-North China Orogen to form the coherent NCC at c. 1.85 Ga (Wilde, Zhao & Sun, Reference Wilde, Zhao and Sun2002; Zhao et al. Reference Zhao, Cawood, Wilde and Sun2002, Reference Zhao, Wilde, Sun, Guo, Kroner, Li, Li and Wu2008a ,Reference Zhao, Wilde, Sun, Li, Li and Zhang b , Reference Zhao, Cawood, Li, Wilde, Sun, Zhang, He and Yin2012; Kröner et al. Reference Kröner, Wilde, Li and Wang2005; Wilde & Zhao, Reference Wilde and Zhao2005; Liu et al. Reference Liu, Zhao, Wilde, Shu, Sun, Li, Tian and Zhang2006; Zhang et al. Reference Zhang, Zhao, Sun, Wilde, Li and Liu2006, Reference Zhang, Zhao, Li, Sun, Liu, Wilde, Kroner and Yin2007, Reference Zhang, Zhao, Li, Sun, Wilde, Liu and Yin2009, Reference Zhang, Zhao, Shen, Li, Sun, Chan and Liu2012, Reference Zhang, Zhao, Shen, Li and Sun2015).
However, the formation and evolution of the Jiao–Liao–Ji Belt remains controversial, with respect to its material composition, tectonic attributes and geodynamics (Jiang, Reference Jiang1987; Zhang, Yang & Liu, Reference Zhang, Yang and Liu1988; Li et al. Reference Li, Yang, Liu and Liu1997, Reference Li, Hao, Han and Zhao2003, Reference Li, Zhao, Santosh, Liu and Dai2011, Reference Li, Zhao, Liu, Dai, Suo, Song and Wang2012; Zhao et al. Reference Zhao, Cawood, Wilde and Sun2002, Reference Zhao, Sun, Wilde and Li2003, Reference Zhao, Sun, Wilde and Li2005, Reference Zhao, Cawood, Li, Wilde, Sun, Zhang, He and Yin2012; Luo et al. Reference Luo, Sun, Zhao, Li, Xu, Ye and Xia2004, Reference Luo, Sun, Zhao, Li, Ayers, Xia and Zhang2008; Wan et al. Reference Wan, Song, Liu, Wilde, Wu, Shi, Yin and Zhou2006; Santosh, Sajeev & Li, Reference Santosh, Sajeev and Li2006; Santosh et al. Reference Santosh, Tsunogae, Li and Liu2007, Reference Santosh, Sajeev, Li, Liu and Itaya2009a ,Reference Santosh, Wan, Liu, Chunyan and Li b ; Santosh, Wilde & Li, Reference Santosh, Wilde and Li2007; Li & Zhao, Reference Li and Zhao2007; Wang et al. Reference Wang, Lu, Chu, Xiang, Zhang and Liu2011, Reference Wang, Ren, Lu, Kang, Chu, Yu and Zhang2015; Zhai & Santosh, Reference Zhai and Santosh2011; Meng et al. Reference Meng, Liu, Cai and Cui2013a , Reference Meng, Liu, Liu, Cui, Yang, Shi, Kong and Lian c , Reference Meng, Liu, Liu, Liu and Yang2014; Liao et al. Reference Liao, Zhang, Jin, Piao and Zheng2016).
Some researchers consider that the Jiao–Liao–Ji Belt represents a continent– or arc–continent collisional belt, along which the Archaean Liaobei–Jinan Complex (Longgang Block) in the north and the Liaonan–Nangrim Complex (Nangrim Block) in the south collided to form a coherent block in Palaeoproterozoic time (Hu, Reference Hu1992; Bai, Reference Bai1993; Bai & Dai, Reference Bai, Dai, Ma and Bai1998; Reference He and YeHe & Ye, 1998a ,b; Faure et al. Reference Faure, Lin, Moni and Bruguier2004; Lu et al. Reference Lu, Wu, Guo, Wilde, Yang, Liu and Zhang2006). In contrast, based on analyses of volcanic rocks, granitoids and metamorphic P–T paths, another school of thought interprets it as a Palaeoproterozoic intra-continental rift zone along the eastern continental margin of the NCC (Zhang & Yang, Reference Zhang and Yang1988; Peng & Xu, Reference Peng and Xu1994; Peng & Palmer, Reference Peng and Palmer1995; Yang et al. Reference Yang, Li, Liu and Liu1995; Liu et al. Reference Liu, Chen, Sha and Wang1997; Li et al. Reference Li, Han, Liu, Yang and Ma2001, Reference Li, Hao, Han and Zhao2003, Reference Li, Zhao, Sun, Han, Hao, Luo and Xia2005; Luo et al. Reference Luo, Sun, Zhao, Li, Xu, Ye and Xia2004, Reference Luo, Sun, Zhao, Li, Ayers, Xia and Zhang2008; Li & Zhao, Reference Li and Zhao2007). Such controversy has arisen because the Jiao–Liao–Ji Belt is made up of diverse materials and has a complex tectonic history. It contains not only thick Palaeoproterozoic volcano-sedimentary successions, but also a large number of Archaean–Palaeoproterozoic granitic rocks, gneisses and meta-mafic rocks of various types (e.g. Jiang, Reference Jiang1987; Zhang, Yang & Liu, Reference Zhang, Yang and Liu1988; LBGMR, 1989; JBGMR, 1991; Bai, Reference Bai1993), and it has undergone a complex series of metamorphism and deformation (Li et al. Reference Li, Hao, Han and Zhao2003, Reference Li, Zhao, Sun, Han, Hao, Luo and Xia2005). Moreover, the latest research shows that the middle segment of the Jiao–Liao–Ji Belt extends eastwards to the southeastern coastal area of Liaodong Peninsula (Meng et al. Reference Meng, Liu, Cai and Cui2013a , Reference Meng, Liu, Liu, Cui, Yang, Shi, Kong and Lian c ; Liu et al. Reference Liu, Liu, Wang, Liu and Cai2015), and the northern segment extends northeastwards to the Kwanmo and Nangrim blocks (previously considered Archaean) on either side of the Machollyong Group in Korea (Zhang et al. Reference Zhang, Wu, Wilde, Zhai, Lu and Sun2004, Reference Zhang, Wu, Yang, Kim and Han2016; Wu et al. Reference Wu, Li, Yang, Jin and Han2016). The rocks in the belt have all been transformed by Palaeoproterozoic thermal events, suggesting that the extent of the Palaeoproterozoic Jiao–Liao–Ji mobile Belt might have been wider than originally thought, and on this basis it can possibly be redefined as the Liao–Ji–Chao Belt (Wu et al. Reference Wu, Li, Yang, Jin and Han2016).
All this means that many more integrated studies are needed on the stratigraphy, petrogenesis, metamorphic evolution, geochronology and tectonic deformation of the Jiao–Liao–Ji Belt if we are to reach a comprehensive understanding of its formation and evolution in Palaeoproterozoic time. Moreover, a systematic comparative study of the lower Precambrian rocks within the Jiao–Liao–Ji Belt and adjacent regions is also necessary, particularly with regards to the nature of their protoliths and tectonic histories. In this context, we systematically studied the petrology, zircon U–Pb geochronology and Lu–Hf isotopes, and elemental geochemistry of the metasedimentary rocks in the upper part of the Ji'an Group in SE Jilin Province. Our new analytical results enable us to discuss protolith formation and metamorphic ages, source properties, petrogenesis and tectonic setting, and then constrain the tectonic history of the Palaeoproterozoic Jiao–Liao–Ji Belt, as well as the formation and evolution of the early crust in this region.
2. Regional geology
The Palaeoproterozoic Jiao–Liao–Ji Belt lies in the eastern part of the Eastern Block of the NCC, which is dominated by Neoarchaean rocks with minor Mesoarchaean and Palaeoarchaean components (Zhao et al. Reference Zhao, Wilde, Cawood and Lu1998, Reference Zhao, Sun, Wilde, Li and Zhang2006; Lu et al. Reference Lu, Zhao, Wang and Hao2008; Wu et al. Reference Wu, Zhao, Sun, Yin, Li and Tam2012). In general, this belt lies between the Liaobei–Jinan Complex (Longgang Block) and the Liaonan–Nangrim Complex (Nangrim Block), and trends NE–E in a belt-like distribution, extending from Gaixian in the southwest, through Dandong to Tonghua in the northeast (and further east into the northern Korean Peninsula), with its southern segment extending across the northern Bohai Sea into eastern Shandong (Jiao–Liao–Ji Belt; Fig. 1; Li & Yang, Reference Li and Yang1997; Li et al. Reference Li, Han, Liu, Yang and Ma2001, Reference Li, Zhao, Sun, Han, Hao, Luo and Xia2005, Reference Li, Zhao, Santosh, Liu and Dai2011; Zhao et al. Reference Zhao, Wilde, Cawood and Sun2001, Reference Zhao, Sun, Wilde and Li2005, Reference Zhao, Kröner, Wilde, Sun, Li, Li, Zhang, Xia and He2007, Reference Zhao, Sun, Wilde and Li2011; Li & Zhao, Reference Li and Zhao2007). Recently, some scholars have suggested that the Palaeoproterozoic Wuhe Group in the Bengbu and Huoqiu areas of Anhui Province is the southwestern extension of the Jiao–Liao–Ji Belt, based on newly obtained isotopic age data (Guo & Li, Reference Guo and Li2009; Liu et al. Reference Liu, Wang, Rolfo, Groppo, Gu and Song2009, Reference Liu, Liu, Wang, Liu and Cai2015; Wan et al. Reference Wan, Dong, Wang, Xie and Liu2010; Liu & Wang, Reference Liu and Wang2012; Wang et al. Reference Wang, Liu, Santosh and Gu2013).

Figure 1. (a) Tectonic location of the NCC. (b) Precambrian regional geological map of the Eastern Block in the NCC, modified after Zhao et al. (Reference Zhao, Sun, Wilde and Li2005). The location of Figure 2 is indicated by the rectangle. WB – Western Block; EB – Eastern Block; TNCO – Trans-North China Orogen; YB – Yinshan Block; OB – Ordos Block.
The Jiao–Liao–Ji Belt comprises mainly sedimentary and volcanic rock successions that were metamorphosed to greenschist to lower-amphibolite facies in association with the emplacement of granitic and mafic igneous intrusions (LBGMR, 1989; JBGMR, 1991; Lu, Reference Lu1996; Li et al. Reference Li, Yang, Liu and Liu1997, Reference Li, Han, Liu, Yang and Ma2001, Reference Li, Hao, Han and Zhao2003; Lu et al. Reference Lu, Wu, Lin, Sun, Zhang and Guo2004 a,b, Reference Lu, Wu, Guo and Yin2005, Reference Lu, Wu, Guo, Wilde, Yang, Liu and Zhang2006; Luo et al. Reference Luo, Sun, Zhao, Li, Xu, Ye and Xia2004, Reference Luo, Sun, Zhao, Li, Ayers, Xia and Zhang2008; Li & Zhao, Reference Li and Zhao2007; Tam et al. Reference Tam, Zhao, Liu, Zhou, Sun and Li2011, Reference Tam, Zhao, Sun, Li, Wu and Yin2012; Wang et al. Reference Wang, Lu, Chu, Xiang, Zhang and Liu2011; Dong et al. Reference Dong, Ma, Liu, Xie, Liu, Li and Wan2012). According to research on the rock assemblages, stratigraphy and tectonics, the belt can be divided along the line of Gaixian–Taziling–Meadowian–Aiyang into a northern zone, which comprises the Fenzishan, North Liaohe and Laoling groups (Luo et al. Reference Luo, Sun, Zhao, Li, Xu, Ye and Xia2004, Reference Luo, Sun, Zhao, Li, Ayers, Xia and Zhang2008; Li et al. Reference Li, Zhao, Sun, Han, Hao, Luo and Xia2005; Zhao et al. Reference Zhao, Sun, Wilde and Li2005; Lu et al. Reference Lu, Wu, Guo, Wilde, Yang, Liu and Zhang2006; Zhou et al. Reference Zhou, Wilde, Zhao, Zheng, Jin, Zhang and Cheng2008; Tam et al. Reference Tam, Zhao, Liu, Zhou, Sun and Li2011, Reference Tam, Zhao, Sun, Li, Wu and Yin2012), and a southern zone that consists of the Jingshan, South Liaohe and Ji'an groups (He & Ye, Reference He and Ye1998a ,Reference He and Ye b ; Li et al. Reference Li, Zhao, Sun, Han, Hao, Luo and Xia2005; Zhao et al. Reference Zhao, Sun, Wilde and Li2005). These two zones are possibly separated by ductile shear zones and faults (Li, Yang & Liu, Reference Li, Yang and Liu1996; Liu & Li, Reference Liu and Li1996; Liu et al. Reference Liu, Chen, Sha and Wang1997). Of these groups, the South and North groups were originally referred to as the Liaohe Group, generally showing a transition from a lower arkose and volcanic-rich sequence, through a carbonate-rich sequence, to an upper argillaceous-rich unit as shown in Figure 3a (LBGMR, 1989). However, further studies show that some spatial variations in different locations exist within the Liaohe Group, and thus suggest that the South and North groups are used, the former being characterized by large amounts of volcanic rocks, while the latter is characterized by more clastic and carbonate rocks (Zhang, Yang & Liu, Reference Zhang, Yang and Liu1988; LBGMR, 1989; JBGMR, 1991; Lu, Reference Lu1996). For the Ji'an and Laoling groups, they are generally considered to be the same as the South and North Liaohe groups according to the most recent geological studies (Lu et al. Reference Lu, Wu, Guo, Wilde, Yang, Liu and Zhang2006; Meng et al. Reference Meng, Wang, Yang, Cai, Ji and Li2017, in press), although the definition and composition of them has changed over the past three decades since their establishment (Zhang, Yang & Liu, Reference Zhang, Yang and Liu1988; LBGMR, 1989; JBGMR, 1991; Lu, Reference Lu1996). Unfortunately, owing to intense deformation (Li et al. Reference Li, Zhao, Sun, Han, Hao, Luo and Xia2005), the original contact relationship between these groups or the further formations within them is still obscured (Liu et al. Reference Liu, Liu, Wang, Liu and Cai2015) and their true stratigraphic nature is open to question (Lu et al. Reference Lu, Wu, Guo, Wilde, Yang, Liu and Zhang2006). Overall, the South and North comprised by these groups are currently considered to be separated by ductile shear zones and faults (Li, Yang & Liu, Reference Li, Yang and Liu1996; Liu & Li, Reference Liu and Li1996; Liu et al. Reference Liu, Chen, Sha and Wang1997, Reference Liu, Liu, Wang, Liu and Cai2015; Li et al. Reference Li, Zhao, Sun, Han, Hao, Luo and Xia2005). With regard to the metamorphism as shown in Figure 4, current studies show that the North Liaohe and Laoling groups followed a clockwise P–T trajectory, whereas the South Liaohe and Ji'an groups followed a counterclockwise P–T trajectory, and thus these two zones have been considered to have formed in different settings or in different parts of a common setting (Bai, Reference Bai1993; Lu, Reference Lu1996; Bai & Dai, Reference Bai, Dai, Ma and Bai1998; He & Ye, Reference He and Ye1998a ,Reference He and Ye b ; Li et al. Reference Li, Hao, Han and Zhao2003).
The subject of the present study, the Ji'an Group, is exposed mainly in the Tonghua area in the southeastern part of Jilin Province in the northern segment of the Jiao–Liao–Ji Belt, and exposures in the Qinghe, Caiyuan and Heping areas are particularly complete (Fig. 2). The Ji'an Group, from the base upwards, can be divided into the Mayihe, Huangchagou and Dadongcha formations (Fig. 3b). Of these, the Mayihe Formation is characteristically boron-bearing, and its main rock types include various altered felsitic and granitic rocks (traditionally named ‘variable granulite-palygorskite’), dolomitic marble, serpentinized olivine marble, boron- and iron-bearing serpentinite and amphibolite, among others. The Mayihe Formation is basically the same as the Lieryu and Gaoguyu formations of the South Liaohe Group in the southeastern part of Liaoning Province. The Huangchagou Formation is characteristically graphite-bearing, and almost all the rock types contain some graphite, including graphite-bearing biotite schists and gneisses, graphite- and diopside-bearing felsitic granitic rocks, graphite-bearing Ca–Mg-silicate rocks, and graphite-bearing marbles and amphibolites. These strata are basically the equivalent of those in the Dashiqiao Formation of the South Liaohe Group, though the lithologies are slightly different (Lu et al. Reference Lu, Wu, Guo, Wilde, Yang, Liu and Zhang2006). The Dadongcha Formation is characteristically Al-rich, and the main rock types are biotite–plagioclase gneisses, garnet–sillimanite–plagioclase gneisses, monzonitic gneisses, cordierite–sillimanite–plagioclase gneisses and biotite felsitic rocks, intercalated with thin-bedded quartzites and feldspathic quartzites. The strata in the Dadongcha Formation are basically similar to those of the Gaixian Formation as shown in Figure 3 (LBGMR, 1989; JBGMR, 1991).

Figure 2. Geological sketch map of southeastern Jilin Province in NE China (modified after JBGMR, 1991), indicating the locations of representative samples.

Figure 3. Lithostratigraphic units of the (a) Liaohe and (b) Ji'an groups (after JBGMR, 1991; Li et al. Reference Li, Zhao, Sun, Han, Hao, Luo and Xia2005)

Figure 4. Metamorphic P–T paths of Palaeoproterozoic volcano-sedimentary rocks (after He & Ye, Reference He and Ye1998b ; Li et al. Reference Li, Hao, Han and Zhao2003)
3. Samples and petrographic characteristics
Rock samples selected for this study were collected from the major rock types of the metasedimentary rocks in the upper part of the Ji'an Group, mainly biotite–plagioclase gneisses, amphibole-bearing biotite–plagioclase gneisses, garnet-bearing quartzites, and quartz-bearing mica or muscovite schists. Details of sampling locations, lithologies and mineral compositions are shown in Figures 2 and 3b, and the main characteristics of these rocks are described as follows.
Biotite–plagioclase gneiss. Fresh surfaces of these rocks are mainly grey or grey-black, and they have a medium-grained granoblastic texture with a variable grain size of 0.3–1.8 mm, displaying a gneissic structure (Fig. 5a). Minerals present are composed of plagioclase, biotite, quartz, some aluminium-rich minerals such as cordierite and garnet, and small amounts of opaque minerals (Fig. 5b). Of these, the biotite makes up 20–25% of the rock, is light green–brown in colour, subhedral, orientated and with grain sizes of 0.5–0.9 mm. The quartz makes up 25–30%, and forms granular mosaics with grain sizes of 0.3–1.2 mm. The plagioclase is subhedral–anhedral, granular and with grain sizes of 0.4–1.8 mm, and makes up 40–45% of the rock. The content of the subhedral cordierite and/or garnet is relatively small (5–10%), and they are 0.5–2.0 mm in grain size. In addition, the main accessory minerals are zircon and magnetite.

Figure 5. Representative field photographs and photomicrographs showing the petrographic features of metasedimentary rocks in the study area. (a, b) Biotite–plagioclase gneiss. (c) Amphibolite. (d) Garnet-bearing quartzite. (e, f) Quartz-bearing mica/muscovite schist. Amp – amphibole; Pl – plagioclase; Crd – cordierite; Q – quartz; Bt – biotite; Ms – muscovite; Grt – garnet.
Amphibole-bearing biotite–plagioclase gneiss. Fresh surfaces on this rock type are grey–black or greyish green, and the rock has a medium- to fine-grained (0.5–1.5 mm) granoblastic texture and gneissic structure (Fig. 5c). The minerals present include plagioclase, biotite, quartz, K-feldspar, amphibole and small amounts of opaque minerals (Fig. 5c). The biotite makes up 20–25% of the rock, and is light green–brown, subhedral–anhedral, oriented and with a grain size of 0.2–0.7 mm. The quartz makes up 15–20% of the rock, and is granular with a grain size of 0.3–1.2 mm. The plagioclase is subhedral–anhedral and granular, with a grain size of 0.3–1.0 mm, and it makes up c. 30–35% of the rock. The K-feldspar is subhedral–anhedral and granular, with a grain size of 0.4–0.9 mm, and it makes up c. 5% of the rock. The amphibole is light green in colour, has a grain size of 0.3–0.8 mm, and makes up less than 5% of the rock. The main accessory minerals are zircon and magnetite.
Garnet-bearing quartzite. The fresh surfaces of these rocks are grey–white or greyish, which display a medium- to fine-grained granoblastic texture and a massive structure. The dominant mineral is quartz (90–95%) with small amounts of garnet (< 5%), and both of them are rounded or irregularly shaped with a variable grain size of 0.3–1.8 mm (Fig. 5d). In addition, there also exist a few opaque minerals, such as zircons and magnetites.
Quartz-bearing mica/muscovite schist. Fresh surfaces of these rocks are mostly greyish-white or grey, and they are fine-grained, with a granular, lamellar crystalloblastic texture and a well-developed schistosity (Fig. 5e, f). The mica schists and muscovite schists have similar minerals, and both of them are composed of biotite, muscovite, plagioclase, quartz and small amounts of magnetite. Of these rocks, the content of muscovite is relatively high (25–30%) in the muscovite schists, but less than 10% in the mica schists. The biotites are subhedral with grain sizes of 0.2–1.0 mm, and they make up 5–10% of the rock. The quartz grains (50–55% of the rock) form granular mosaics, with grain sizes of 0.3–1.2 mm. The plagioclase is subhedral and evenly distributed, making up 5–10% of the rock and with grain sizes of 0.3–0.8 mm.
4. Analytical methods
4.a. Zircon U–Pb dating
The samples were crushed and zircons were separated using conventional heavy liquid and magnetic separation methods at the Langfang Regional Geological Survey Institute in Hebei Province. Zircon grains were picked out and pasted on the surface of an epoxy resin disc with the aid of a binocular microscope. After polishing the grains, they were examined under transmitted and reflected light. Cathodoluminescence (CL) images of the zircons were collected on a HTACHI S-3000N scanning electron microscope (SEM) with a GATAN Chroma CL probe at the Beijing SHRIMP Centre, Chinese Academy of Geological Sciences, Beijing, China. Zircon U–Pb dating and in situ analyses of rare earth elements (REEs) were carried out with an Agilent 7500a quadrupole inductively coupled plasma mass spectrometer (ICP-MS) and a Neptune multiple-collector ICP-MS coupled to a laser at the Laboratory Centre, Xi'an Centre of Geological Survey (Xi'an), and the State Key Laboratory of Geological Processes and Mineral Resources, China University of Geosciences (Beijing), China. A 193 nm excimer laser was focused on the surfaces of the zircon grains with an energy density of 10 J/cm2. The laser beam diameter was 30 μm and the repetition rate was 5 Hz. Helium was used as a carrier gas to transport the ablated aerosol to the laser ablation ICP-MS (LA-ICP-MS). Each set of eight sample analyses was bracketed with analyses of zircon standards 91500 and GJ-1, and the glass standard NIST612. Each spot analysis comprised the acquisition of c. 5 s of background data and 45 s of sample data. 207Pb/206Pb, 206Pb/238U, 207U/235U and 208Pb/232Th ratios were corrected for instrumental isotopic and elemental fractionation effects by using the analyses of zircon standard 91500. Trace element concentrations and U–Pb isotopic compositions were calculated using the program GLITTER 4.4.1 and corrected using 29Si as an internal standard and NIST612 as an external standard. Common Pb was corrected following Anderson (Reference Anderson2002). Weighted mean U–Pb ages and concordia plots were processed using ISOPLOT 3.0, with uncertainties quoted at the 2σ and 95% confidence levels (Ludwig, Reference Ludwig2003). Details of the analytical procedures and data processing methods can be found in Song et al. (Reference Song, Niu, Wei, Ji and Su2010) and Liu et al. (Reference Liu, Hu, Gao, Günther, Xu, Gao and Chen2008).
4.b. In situ Lu–Hf isotope analyses of zircon
In situ zircon Hf isotope analyses were performed using a New Wave UP213 laser coupled to a Neptune multiple-collector ICP-MS at the Laboratory Centre, Xi'an Centre of Geological Survey, Xi'an, China. Details of the instrumental conditions and data acquisition procedures are given by Wu, Yang & Xie (Reference Wu, Yang and Xie2006) and Hou et al. (Reference Hou, Li, Zou, Qu, Shi and Xie2007). A stationary laser ablation spot with a beam diameter of either 40 or 55 μm was used for the analyses. The ablated material was transported from the laser ablation cell using helium as a carrier gas, and then combined with argon in a mixing chamber before being introduced to the ICP-MS plasma. Corrections for 176Lu and 176Yb isobaric interferences on readings for 176Hf used the values of 176Lu/175Lu = 0.02658 and 176Yb/173Yb = 0.796218, respectively (Chu et al. Reference Chu, Taylor, Chavagnac, Nesbitt, Boella, Milton, German, Bayon and Burton2002). Instrumental mass bias was corrected by normalizing Yb isotope ratios to 172Yb/173Yb = 1.35274 (Chu et al. Reference Chu, Taylor, Chavagnac, Nesbitt, Boella, Milton, German, Bayon and Burton2002) and Hf isotope ratios to 179Hf/177Hf = 0.7325, using the exponential mass fractionation law. The mass bias behaviour of Lu was assumed to follow that of Yb. Details of the mass bias correction protocols are described by Iizuka & Hirata (Reference Iizuka and Hirata2005), Wu, Yang & Xie (Reference Wu, Yang and Xie2006) and Hou et al. (Reference Hou, Li, Zou, Qu, Shi and Xie2007). Zircon GJ1 was used as the reference standard and yielded a weighted mean 176Hf/177Hf ratio of 0.282008±27 (2σ) during this study. This ratio is indistinguishable from a weighted mean 176Hf/177Hf ratio of 0.282013±19 (2σ) obtained by Elhlou et al. (Reference Elhlou, Belousova, Griffin, Pearson and O'Reilly2006) for this standard. The individual Hf isotopic analyses were made directly on top of the U–Pb ablation pits. For this paper, all representative concordant and sub-concordant zircons with age discordances of < 5% (which we consider to be reliable and were used in binned frequency histograms) were selected for Hf isotope analyses. All Hf isotope data are reported with an error of 2σ of the mean, and the values of ε Hf were calculated using a 176Lu decay constant of 1.865×10–11 yr–1 (Scherer, Münker & Mezger, Reference Scherer, Münker and Mezger2001). Depleted mantle model ages (T DM) were calculated based on the measured 176Lu/177Hf and 176Hf/177Hf ratios with reference to depleted mantle with present-day values of 176Hf/177Hf = 0.28325 and 176Lu/177Hf = 0.0384 (Griffin et al. Reference Griffin, Pearson, Belousova, Jackson, van Achterbergh and O'Reilly2000). Average continent crustal (TC DM ) model ages were calculated for the magma source using the initial 176Hf/177Hf ratio of the zircon and assuming a mean crustal 176Lu/177Hf value of 0.015 (Griffin et al. Reference Griffin, Belousova, Shee, Pearson and O'Reilly2004).
4.c. Major and trace element analyses
To avoid the effects of weathering, the samples collected in the field were examined under the microscope so that relatively fresh samples could be selected for geochemical analysis. All whole-rock samples were crushed in an agate mill to ~ 200 mesh, after the removal of altered surfaces. Major and trace elements were determined using X-ray fluorescence spectrometry (XRF; Rigaku RIX 2100 spectrometer) and fused glass discs, and by using ICP-MS after acid digestion of samples in Teflon bombs. All the analyses were done at the National Research Centre for Geoanalysis, Chinese Academy of Geological Sciences, Beijing, China. Major elements and the trace elements Zr, Sr, Ba, Zn, Rb and Nb were determined using X-ray fluorescence spectrometry (XRF), and the instrument used was a Japan Science-3080 with errors of < 0.5%, and a Rigaku-2100 spectrometer with an error of Ba = 5% and other elements < 3%. The other elements, such as V, Co, Cu, Pb, U, Th, Ta and Hf, were determined using ICP-MS. The REEs were analysed (with errors of < 5%) using ICP-MS after REEs were separated using cation-exchange techniques. Moreover, the trace elements in zircon were analysed using LA-ICP-MS. Analytical uncertainties for LA-ICP-MS trace element analyses were ± 10% for elements with abundances lower than 10 ppm and c. ± 5% for elements with abundances higher than 10 ppm (Meng et al. Reference Meng, Liu, Cai and Cui2013a ; Zeng et al. Reference Zeng, Gao, Xie and Zeng2011).
5. Analytical results
5.a. Whole-rock major and trace element geochemistry
Previous studies have shown that the rock assemblage of the Ji'an Group is basically the same as that of the South Liaohe Group in the Jiao–Liao–Ji Belt, and the lower part of the Mayihe Formation contains a large amount of metavolcanic rock (JBGMR, 1991). The upper Huangchagou and Dadongcha formations are characterized by thick successions of metasedimentary rocks with minor metavolcanic rocks, which is also consistent with our field investigations and the fact that our samples fall mainly within the sedimentary area on the ((al+fm) – (c+alk)) versus Si discrimination diagram for protolith types of metamorphic rocks (Fig. 6a; Simonen, Reference Simonen1953). Moreover, these samples have generally undergone a low degree of hydrothermal alteration and metamorphism (JBGMR, 1991), which would have affected mobile elements such as K, Rb, Th and U (Taylor & McLennan, Reference Taylor and McLennan1985; Bhatia & Crook, Reference Bhatia and Crook1986; McLennan, Taylor & McCulloch, Reference McLennan, Taylor and McCulloch1990), and this is supported by the large variations in their contents, as shown in the primitive-mantle-normalized spider diagrams (Fig. 7b; online Supplementary Material Table S1 available at http://journals.cambridge.org/geo). Nevertheless, with respect to the indicators normally used for assessing the alteration-independent index of geochemical variations (Pearce, Ernewein & Bloomer, Reference Pearce, Ernewein, Bloomer and Smellie1995), the low values of LOI (loss of ignition < 3%) in our samples, the absence of Ce anomalies, carbonization or silicification, the collinear trends of MgO and TiO2, the analytical data for the high field strength elements (HFSEs; e.g. Nb, Ta, Y, Zr and Hf) (Meng et al. Reference Meng, Wang, Yang, Cai, Ji and Li2017) and the REE versus Zr plots (online Supplementary Material Table S1 available at http://journals.cambridge.org/geo), we infer that these elements, as well as the transition metals (Cr, Co and Ni), were immobile in our samples. In other words, much of the geochemistry of our metasedimentary samples was unaffected by weathering, transport, diagenesis, alteration or greenschist- to lower-amphibolite-facies metamorphism, and therefore the geochemical data can be used robustly to constrain the source characteristics, petrogenesis and tectonic setting (Taylor & McLennan, Reference Taylor and McLennan1985; Bhatia & Crook, Reference Bhatia and Crook1986; McLennan, Taylor & McCulloch, Reference McLennan, Taylor and McCulloch1990).

Figure 6. Classification diagrams for the metasedimentary samples, modified after Simonen (Reference Simonen1953), Herron (Reference Herron1988), Gromet, Dymek & Haskin (Reference Gromet, Dymek and Haskin1984) and McLennan et al. (Reference McLennan, Taylor and McCulloch1990), respectively. (a) (al+fm) – (c+alk) versus Si diagram. (b) Log(Fe2O3/K2O) versus log(SiO2/Al2O3) diagram. (c) La/Yb versus ΣREEs diagram. (d) La versus Th diagram.

Figure 7. (a) Chondrite-normalized REE and (b) primitive-mantle-normalized trace element patterns for samples of the metasedimentary rocks. Normalizing values are after Boynton (Reference Boynton and Henderson1984) and Sun & McDonough (Reference Sun, McDonough, Saunders and Norry1989), respectively.
The chemical compositions of the 39 samples of metasedimentary rock from the upper Ji'an Group are listed in online Supplementary Material Table S1 (available at http://journals.cambridge.org/geo). Although the rocks of different type have variable major element compositions, they all have similar geochemical characteristics (Fig. 6). The contents of SiO2 range from 51.28 to 74.53 wt% (average 62.43 wt%), similar to the shale average of 62.00 wt% (Condie, Reference Condie1993). Their TiO2, Al2O3, CaO, Na2O, K2O, FeO, Fe2O3 and MgO contents (in wt%) are 0.34–1.05, 11.45–25.81, 0.17–3.53, 0.39–3.84, 0.97–7.00, 1.20–4.53, 0.18–2.52 and 0.98–5.07, respectively (online Supplementary Table S1 available at http://journals.cambridge.org/geo). Their log(SiO2/Al2O3) values vary between 0.31 and 0.79, and log(FeOT/K2O) values between –0.85 and 0.21, so these samples fall mainly within the arkose and wacke fields on the diagram of Herron (Reference Herron1988), with just a few falling in the shale field (Fig. 6b).
Chondrite-normalized REE patterns for these metasedimentary rocks are characterized by relative enrichments in REEs (ΣREEs = 83.64–511.62 ppm, average = 233.44 ppm), distinct fractionation of the light and heavy REEs ((La/Yb)N = 5.54–79.61 with an average value of 16.51), enrichments in LREEs (79.38–485.83 ppm, average = 214.59 ppm), flat HREEs (4.26–32.50, average = 18.85 ppm) and obvious negative Eu anomalies (δEu = 0.26–0.89, except for two unusual analytical spots with values of 1.79 and 2.73) (Fig. 7a; online Supplementary Material Table S1 available at http://journals.cambridge.org/geo).
Primitive-mantle-normalized trace element diagrams show that these rocks are relatively enriched in large-ion lithophile elements (LILEs; e.g. Rb, Ba, K and Pb) and strongly depleted in HFSEs (e.g. Nb, Ta, P and Ti) (Fig. 7b). Moreover, all the samples exhibit relatively strong linear correlations between SiO2 and each of Th, Y and Zr, suggesting that HFSE concentrations were related to and controlled by felsic source components, and were relatively immobile during weathering, diagenesis and low-grade metamorphism (Lahtinen et al. Reference Lahtinen, Huhma, Kontinen, Kohonen and Sorjonen-Ward2010). On a diagram used to distinguish the origins of sediments (La/Yb versus ΣREEs; Fig. 6c), our metasedimentary samples appear mainly to have been sandstones, greywackes, claystones and shales. This is generally consistent with the protolith identified from Figure 6d, which shows a La versus Th diagram, used principally for distinguishing Archaean from post-Archaean sediments, indicating that the protolith was mainly sediment of post-Archaean age, with minor amounts of Archaean material. These features are also generally consistent with the geochemical properties of metamorphosed sedimentary rocks in the upper part of the South Liaohe Group in the southwest of the study area (our unpub. data), suggesting they could have similar sources of sediment.
5.b. Zircon U–Pb dating
U–Pb ages for zircons from specimens of garnet-bearing biotite felsic gneiss, amphibole-bearing biotite–plagioclase gneiss, biotite–plagioclase gneiss and quartz-bearing mica schist that were collected from the metamorphosed supracrustal rocks in the upper part of the Ji'an Group in southeastern Jilin Province are presented in online Supplementary Material Table S2 (available at http://journals.cambridge.org/geo). Hf isotopic data for the concordant zircons are listed in online Supplementary Material Table S3 (available at http://journals.cambridge.org/geo) and Figure 8 shows CL images of representative zircons.
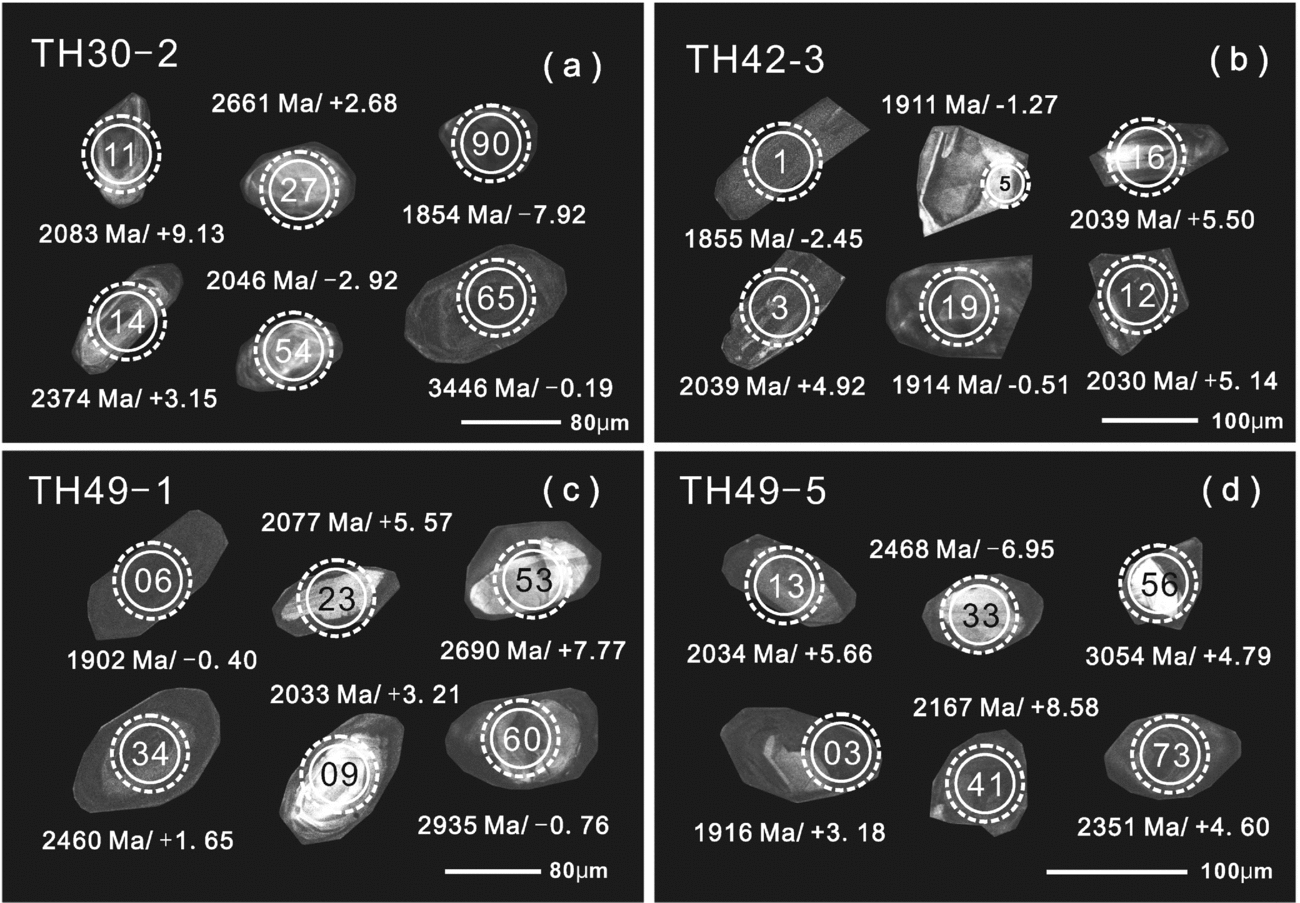
Figure 8. Representative CL images of selected zircons from the metasedimentary rocks in the study area. The dashed circles delimit the areas of LA-(MC)-ICP-MS analytical sites; the corresponding 207Pb–206Pb ages and their εHf values are shown.
Sample TH30-2 is a medium-grained garnet-bearing biotite felsic gneiss sampled from a site located 1.3 km to the southwest of Toudao County (i.e. west of Qinghe Town in southern Tonghua City; 125°12′33.38″E, 41°30′25.45″N; Fig. 2). Zircons from this sample are mainly euhedral–subhedral, columnar or rounded, and 60–120 μm in size. The CL images show that almost all the grains are dark grey or bright, and display oscillatory growth-zoning (Fig. 8a). Their high Th/U ratios (0.29–1.43) indicate formation by crystallization from a magma (Hoskin & Ireland, Reference Hoskin and Ireland2000; Belousova et al. Reference Belousova, Griffin, O'Reilly and Fisher2002). One exceptional grain had an unclear internal structure and a low Th/U ratio (0.02), indicating a metamorphic origin (Tomaschek et al. Reference Tomaschek, Kennedy, Villa, Lagos and Ballhaus2003; Dubińska, Bylina & Kozłowski, Reference Dubińska, Bylina and Kozłowski2004). The dating results show that 71 of the 90 analytical results are located on or near the concordant line (Fig. 9a), with the concordant 207Pb–206Pb ages varying from 1854 to 3446 Ma. Of these, the date of 1854±21 Ma represents the metamorphic age of a zircon, while all the others are the ages of magmatic zircons. Among the analyses, three of the zircons with the youngest magmatic ages of 2044–2053 Ma yielded a 207Pb–206Pb weighted mean age of 2048±23 Ma (MSWD = 0.05), and this represents the maximum possible depositional age. The other magmatic zircons had 207Pb–206Pb concordant ages of 2077–3446 Ma, with peak ages at c. 2079, 2148, 2273, 2368–2421, 2451–2545 and 2670 Ma (Fig. 9a). There were also two even more ancient zircons with ages of 2923 and 3446 Ma. In summary, we consider that the maximum possible depositional age of the protoliths of these garnet-bearing biotite felsic gneisses is 2048 Ma, and the rocks were metamorphosed at 1854 Ma. The 2077–3446 Ma ages represent magmatic zircons with ages that are clearly older than the depositional age of the original sediment.

Figure 9. U–Pb isotopic compositions of zircons from metasedimentary rocks in the study area.
Sample TH42-3 is a medium- to fine-grained amphibole-bearing biotite–plagioclase gneiss sampled from a site 0.4 km to the north of Changchungou County (i.e. west of Huadian Town in southern Tonghua City; 125°34′18.89″E, 41°19′09.99″N; Fig. 2). Zircons from this sample are mainly subhedral and short or long columnar, plate-like or irregular in shape. The grain sizes are 70–120 μm. The CL images and trace element analyses show that the zircons have complex internal structures, indicating a range of genetic types (Fig. 8b). Of these, the columnar or plate-like grains with growth zones or stripe-like absorption patterns have high Th/U ratios (0.64–1.31), indicating a magmatic origin (Hoskin & Ireland, Reference Hoskin and Ireland2000; Belousova et al. Reference Belousova, Griffin, O'Reilly and Fisher2002), while the grains that are grey, homogeneous, columnar, cloudy or dappled, with bright edge structures, have low Th/U ratios (0.01–0.13), indicating a metamorphic origin (Tomaschek et al. Reference Tomaschek, Kennedy, Villa, Lagos and Ballhaus2003; Dubińska, Bylina & Kozłowski, Reference Dubińska, Bylina and Kozłowski2004). The dating results were obtained from 20 analyses located on or near the concordant line, with 207Pb–206Pb ages varying between 1846 and 2053 Ma (Fig. 9b). Of these, the metamorphic zircons have the lowest two 207Pb–206Pb weighted mean ages of 1854 and 1906 Ma, while all the other magmatic ones have 207Pb–206Pb ages between 2021 and 2053 Ma, with a weighted mean age of 2038±10 Ma (n = 13, MSWD = 0.31) (Fig. 9b). We consider, therefore, that the maximum depositional age of the protolith of this sample is c. 2038 Ma, and that the sediments were metamorphosed at 1854 and 1906 Ma.
Sample TH49-1 is a medium-grained biotite–plagioclase gneiss sampled from a site located 0.5 km south of Xiaodongcha County (i.e. northwest of Liuye Town in northern Ji'an City; 125°59′57.17″E, 41°18′41.11″N; Fig. 2). Zircons from this sample are mainly subhedral, spindle-shaped, short, columnar or granular, and the grains range in size from 40 to 120 μm. The CL images show that the grains can be divided into two groups: one with grey homogeneous features, and the other with dark-grey homogeneous rims and irregular cores with stripe-like absorption or growth zones (Fig. 8c). The first group, and the rims of the second group, have low Th/U ratios (0.03–0.14), suggesting a metamorphic origin (Tomaschek et al. Reference Tomaschek, Kennedy, Villa, Lagos and Ballhaus2003; Dubińska, Bylina & Kozłowski, Reference Dubińska, Bylina and Kozłowski2004), while the cores of the second group have relatively high Th/U ratios (0.26–1.43), suggesting magmatic crystallization (Hoskin & Ireland, Reference Hoskin and Ireland2000; Belousova et al. Reference Belousova, Griffin, O'Reilly and Fisher2002). The dating results show that 74 of the 90 analyses are located on or near the concordant line (Fig. 9c), with 207Pb–206Pb ages varying from 1885 to 2935 Ma. Of these, the 35 analytical spots on metamorphic zircons gave the lowest ages in the age spectrum, with 207Pb–206Pb concordant ages of 1885–1915 Ma and a weighted mean age of 1901±6 Ma (MSWD = 0.44 (Fig. 9c), which suggests the metamorphism of the sedimentary protolith of the biotite–plagioclase gneiss took place at c. 1901 Ma. The youngest magmatic zircons have 207Pb–206Pb ages varying from 2033 to 2039 Ma, yielding a weighted mean age of 2037±21 Ma (MSWD = 0.04; Fig. 9c), and this represents the maximum possible depositional age of the sedimentary protolith of the biotite–plagioclase gneiss. All the other magmatic zircons have concordant ages of 2070–2460 Ma, with peaks at 2080, 2153, 2198, 2272, 2353 and 2460 Ma as shown in Figure 9c, and there are also three even more ancient zircons with ages of 2690, 2790 and 2935 Ma. The 2070–2935 Ma ages represent magmatic zircons with ages that are clearly older than the depositional age of the original sediment.
Sample TH49-5 is a fine-grained quartz-bearing mica schist, also sampled from a site located 0.5 km south of Xiaodongcha County (i.e. northwest of Liuye Town in northern Ji'an City; 125°59′57.17″E, 41°18′41.11″N; Fig. 2). Zircons from this sample are mainly subhedral, short or long, columnar, rounded or irregular, and with a size range of 40–120 μm. The CL images show that most of the zircons fall into two groups: the first has core–rim structures where the irregular cores are grey or light-grey and display weak stripe-like absorption, and where the rims are grey and homogeneous; the second group is made up of single grains that show weak growth-zoning (Fig. 8d). The trace element analyses show that the grey homogeneous rims have low Th/U ratios (0.02–0.09), suggesting a metamorphic origin (Tomaschek et al. Reference Tomaschek, Kennedy, Villa, Lagos and Ballhaus2003; Dubińska, Bylina & Kozłowski, Reference Dubińska, Bylina and Kozłowski2004), while all the other zircon types have high Th/U ratios (0.22–2.55), suggesting a magmatic origin (Hoskin & Ireland, Reference Hoskin and Ireland2000; Belousova et al. Reference Belousova, Griffin, O'Reilly and Fisher2002). The dating results show that 71 of the 79 analyses are located on or near the concordant line, with concordant or sub-concordant 207Pb–206Pb ages between 1859 and 3234 Ma (Fig. 9d). Of these, the metamorphic zircons display two distinct ages: a 207Pb–206Pb weighted mean age of 1919±9 Ma (n = 17, MSWD = 1.60), and a single age of 1859±23 Ma. All the other ages are for magmatic zircons, and among these, 12 analyses with the youngest ages of 2020–2048 Ma yielded a 207Pb–206Pb weighted mean age of 2037±11 Ma (MSWD = 0.38), which we take to represent the maximum possible depositional age of the sedimentary protolith. All the other magmatic grains show concordant ages of 2069–2493 Ma, with ages at c. 2088, 2123–2242, 2288 and 2351–2493 Ma (Fig. 9d). Moreover, there were four even more ancient zircons with ages of 2666, 2814, 3054 and 3234 Ma. Thus, we consider that the maximum possible depositional age for the protoliths of these quartz-bearing mica schists is 2037 Ga, that the sediments recorded two-stage metamorphic events at c. 1859 and 1919 Ma, and that the 2070–3446 Ma ages represent magmatic zircons that are clearly older than the depositional age of the sediment.
In summary, the petrology, zircon U–Pb geochronology, microstructures and trace element analyses of the metasedimentary rocks of the upper Ji'an Group show that the zircons from different samples are very similar in terms of their age spectrum characteristics, internal structures and compositions, which means they are all comparable in terms of the timing of protolith formation, metamorphism and the compositional characteristics of the source rocks, which in turn means they can all be discussed together, as a whole. The analytical results show that all the concordant ages in these samples gave the following mean or statistical peak ages: 1855, 1912, 2035, 2082, 2178, 2343–2421, 2451–2545, 2643–2814 and 2923–3446 Ma (Fig. 10a).

Figure 10. (a) Zircon U–Pb age spectra t(Ma), and (b) εHf(t) versus T(Ma) diagram for analysed zircons from the metasedimentary rocks in the study area.
5.c. Zircon Lu–Hf isotope data
Garnet-bearing biotite-felsic gneiss (TH30-2). In this specimen, the metamorphic zircons with ages of c. 1854 Ma have an εHf(t) value of –7.92 and a corresponding average continent crustal (TC DM ) model age of 3.03 Ga. The magmatic zircons with peak ages of 2048 Ma have εHf(t) values and (TC DM ) model ages of –2.92 to –0.93 and 2.74–2.86 Ga, respectively. The other magmatic zircons with ages of c. 2079, 2133–2168, 2367 and 2450–2545 Ma have εHf(t) values of +6.52 to +9.20, +5.76 to +7.27, –5.18 to +3.54 and –5.63 to +8.05 (further divided into –5.63 to –0.35 and +1.19 to +8.05), respectively, and corresponding (TC DM ) model ages of 2.11–2.28, 2.30–2.37, 2.73–3.29 and 2.52–3.36 Ga (3.05–3.36 and 2.52–2.94 Ga). The zircons with ages of 2643–2698 Ma have εHf(t) values of –1.07 to +2.68 and +6.53 to +7.43, and (TC DM ) model ages of 2.98–3.20 and 2.70–2.74 Ga, respectively. The two oldest zircons with ages of 2923 and 3446 Ma have εHf(t) values of –0.55 and –0.19, and (TC DM ) model ages of 3.39 and 3.76 Ga, respectively (online Supplementary Material Table S3 available at http://journals.cambridge.org/geo).
Amphibole-bearing biotite–plagioclase gneiss (TH42-3). In this specimen, the metamorphic zircons with ages of c. 1854 and 1906 Ma have εHf(t) values of –0.41 to +2.45 and –3.15 to +0.53, respectively, and corresponding average continent crustal (TC DM ) model ages of 2.56–2.68 and 2.52–2.77 Ga. The magmatic zircons with a peak age of 2038 Ma have εHf(t) values that fall into two groups of +3.16 to +6.94 and –3.69 to –0.89, with corresponding (TC DM ) model ages of 2.24–2.46 and 2.72–2.90 Ga (online Supplementary Material Table S3 available at http://journals.cambridge.org/geo).
Biotite–plagioclase gneiss (TH49-1). In this specimen, the metamorphic zircons with ages of c. 1901 Ma have εHf(t) values of –3.22 to +3.13 and (TC DM ) model ages of 2.36–2.77 Ga. The youngest magmatic zircons with a peak age of c. 2037 Ma have εHf(t) values of +3.21 to +8.78 and (TC DM ) model ages of 2.11–2.46 Ga. The other magmatic zircons with ages of c. 2080, 2121–2277, 2353 and 2460 Ma have εHf(t) values of +5.40 to +8.59, +1.79 to +9.60, –0.17 to +4.82 and +1.65, respectively, and corresponding (TC DM ) model ages of 2.15–2.37, 2.15–2.48, 2.61–2.93 and 2.89 Ga. The three oldest zircons with ages of 2690, 2790 and 2935 Ma have εHf(t) values of +7.77, +7.79 and –0.76, respectively, and corresponding (TC DM ) model ages of 2.67, 2.75 and 3.41 Ga (online Supplementary Material Table S3 available at http://journals.cambridge.org/geo).
Quartz-bearing mica schist (TH49-5). The metamorphic zircons with ages of c. 1.91 Ga have εHf(t) values of –2.71 to +3.29 and (TC DM ) model ages of 2.37–2.76 Ga. The youngest magmatic zircons with peak ages of c. 2037 Ma have εHf(t) values of –3.06 to +7.38 and (TC DM ) model ages of 2.18–2.87 Ga. The other magmatic zircons with ages of c. 2088, 2123–2288, 2351 and 2468–2493 Ma have εHf(t) values of +5.81 to +9.76, +3.11 to +9.36, +4.60 and –6.95 to +0.98, respectively, and corresponding (TC DM ) model ages of 2.08–2.35, 2.17–2.59, 2.62 and 2.96–3.44 Ga. The oldest zircons with ages of 2666, 2814 and 3054–3234 Ma have εHf(t) values of +1.68, +6.81 and +4.79 to +5.59, respectively, and (TC DM ) model ages of 3.05, 2.83 and 3.15–3.23 Ga (online Supplementary Material Table S3 available at http://journals.cambridge.org/geo).
To summarize, the zircon geochronology results for different samples have a high degree of similarity, as mentioned above, and likewise the Lu–Hf isotope data for coeval zircons with different origins, and in different samples, have similar Hf isotopic features. We conclude, therefore, that the Hf isotopic characteristics of these zircons can be discussed together, as a whole. Zircons with mean or statistical peak ages of 1855, 1912, 2035, 2082, 2178, 2343–2421, 2451–2545 and 2643–2814 (Fig. 10a) have Lu–Hf isotopic characteristics that give corresponding εHf(t) values of –7.92 to –0.41, –3.22 to +3.29, –3.69 to +8.78 (further divided into –3.69 to –0.89 and +1.97 to +8.78), +5.40 to +9.76, +3.11 to +9.60, –5.18 to +4.82, –6.95 to +8.05 (further divided into –6.95 to –0.35 and +1.19 to +8.05) and +6.53 to +7.79 (except some analyses with –1.07 to +2.68), respectively. The corresponding average continent crustal (TC DM ) model ages are 2.56 to 3.03, 2.37 to 2.77, 2.11–2.90 (2.72–2.90 and 2.11–2.53 Ga), 2.08 to 2.37, 2.15 to 2.57, 2.61 to 3.29, 2.50–3.44 (3.05–3.44 and 2.52–2.94 Ga) and 2.67–2.83 (except some analyses with 2.98–3.20 Ga), respectively (Fig. 10b; online Supplementary Material Table S3 available at http://journals.cambridge.org/geo). The ages of the oldest zircon grains (2923–3446 Ma) can be subdivided into groups of 2923–2935, 3054–3234 and 3446 Ma, which have corresponding εHf(t) values of –0.76 to –0.55, +4.79 to +5.59 and –0.19, and (TC DM ) model ages of 3.39–3.41, 3.15–3.23 and 3.76 Ga, respectively (Fig. 10b; online Supplementary Material Table S3 available at http://journals.cambridge.org/geo).
6. Discussion
6.a. Nature of the protolith of metasedimentary rocks in the Ji'an Group
Previous studies have shown that the upper Ji'an Group in SE Jilin Province, in the northern segment of the Jiao–Liao–Ji Belt, is characterized by thick successions of metasedimentary rocks, which now consist of several types of schist, gneiss and marble, with some metasandstones and metasiltstones (JBGMR, 1991). The analyses of 39 samples of these metasedimentary rocks show that they are generally enriched in Al2O3, depleted in CaO and FeOT, have K2O/Na2O values of > 1, and contain garnet and cordierite, all of which are consistent with a metasedimentary origin (Fig. 6a). Together with the fields in which these rocks plot on various discrimination diagrams (Fig. 6b, c), we consider that the protoliths of the metasedimentary rocks in the upper Ji'an Group were mainly medium- to fine-grained clastic sediments dominated by sandy, wacke and claystone material, together with some shale.
6.b. Provenance of metasedimentary rocks in the Ji'an Group
Although the chemical compositions of clastic sedimentary rocks depend on the compositions of the source rocks, and may be affected by processes such as sorting during transport, diagenesis, chemical weathering and regional metamorphism (Nesbitt & Young, Reference Nesbitt and Young1982; Bhatia & Crook, Reference Bhatia and Crook1986; Roser & Korsch, Reference Roser and Korsch1986; McLennan et al. Reference McLennan, Hemming, McDaniel, Hannson, Johnsson and Basu1993; Cullers, Reference Cullers1994a ,b; Fedo, Nesbitt & Young, Reference Fedo, Nesbitt and Young1995; Fedo, Eriksson & Krogstad, Reference Fedo, Eriksson and Krogstad1996), the fact that some chemical substances are relatively immobile (e.g. Al2O3, TiO2, REEs, Y, Th, Nb, Zr, Hf, Ta and some LILEs such as Rb, Ga, Cs), but may by enriched during weathering (Nesbitt & Young, Reference Nesbitt and Young1982, Reference Nesbitt and Young1984; Harnois, Reference Harnois1988; McLennan et al. Reference McLennan, Hemming, McDaniel, Hannson, Johnsson and Basu1993), allows those chemicals to be used as a basis for discussing protolith compositions, provenance and tectonic setting.
CIA values (Chemical Index of Alteration, CIA = mol (Al2O3/(Al2O3+CaO*+Na2O+K2O)*100, where CaO* refers to the CaO present in silicate minerals; Nesbitt & Young, Reference Nesbitt and Young1982) have been widely applied to quantifying the degree of weathering (Nesbitt & Young, Reference Nesbitt and Young1982; Long et al. Reference Long, Sun, Yuan, Xiao and Cai2008). If there is pronounced chemical weathering in the source area, feldspars would have been preferentially decomposed, leading to enrichments in Al2O3 and depletions in K2O and Na2O. In general, fresh igneous rocks have CIA values lower than 50, while residual clays have values close to 100 (McLennan et al. Reference McLennan, Hemming, McDaniel, Hannson, Johnsson and Basu1993). The metasedimentary rocks from the upper Ji'an Group have CIA values of 54.86 to 72.87 (average = 65.41; online Supplementary Material Table S1 available at http://journals.cambridge.org/geo; Fig. 11a), which are higher than the value for fresh upper continental crust (UCC = 48; Rudnick & Gao, Reference Rudnick and Gao2003) but lower than the values for Post Archaean Australian Shale (PAAS = 70–75; McLennan et al. Reference McLennan, Hemming, McDaniel, Hannson, Johnsson and Basu1993), implying a relatively low compositional maturity and relatively weak weathering of the protolith (Nesbitt & Young, Reference Nesbitt and Young1984; Fedo, Nesbitt & Young, Reference Fedo, Nesbitt and Young1995;). The Index of Compositional Variability (ICV, mol (Fe2O3+K2O+Na2O+CaO+MgO+TiO2)/Al2O3; Cox, Lowe & Cullers, Reference Cox, Lowe and Cullers1995) is an important parameter for quantifying the maturity of sediments and revealing the tectonic setting (Cox, Lowe & Cullers, Reference Cox, Lowe and Cullers1995; Roser et al. Reference Roser, Cooper, Nathan and Tulloch1996). In general, sediments with low ICV values were probably derived from a mature sedimentary source with large amounts of clay minerals, indicating a recycling of sediment in a passive tectonic setting. On the other hand, clastic sediments with high ICV values indicate the circulation of immature sediments in an active tectonic setting (Cox, Lowe & Cullers, Reference Cox, Lowe and Cullers1995; Roser et al. Reference Roser, Cooper, Nathan and Tulloch1996). Our samples have relatively high values of ICV (mainly > 1; online Supplementary Material Table S1 available at http://journals.cambridge.org/geo; Fig. 11a), and, together with their relatively low CIA values (lower than PAAS), this indicates that the protoliths were derived from a weakly weathered sedimentary source in an active tectonic setting. The geochemical features of these metasediments are also consistent with a mainly acidic arc source, but there was some input from ancient crustal material, as indicated by Figure 11b (a diagram based on Floyd & Leveridge, Reference Floyd and Leveridge1987), and a minor contribution from Archaean sediments is indicated by trace element compositions (Fig. 6d) and the existence of a few ancient zircons (Fig. 9).

Figure 11. (a) ICV versus CIA diagram (after Nesbitt & Young, Reference Nesbitt and Young1984 and Cox, Lowe & Cullers, Reference Cox, Lowe and Cullers1995) and (b) La/Th versus Hf diagram (after Floyd & Leveridge, Reference Floyd and Leveridge1987) for metasedimentary rocks in the study area.
The transition group elements V, Cr, Co and Ni are compatible elements that tend to be concentrated in mafic–ultramafic rocks during magmatic differentiation, and HFSEs (e.g. Nb, Ta, Zr and Hf) are relatively stable (Hofmann, Reference Hofmann1988; McLennan, Taylor & McCulloch, Reference McLennan, Taylor and McCulloch1990). In general, mafic rocks have values of Ti/Zr >> 50 and Cr/Zr > 1, and Th/Cr, Th/Sc, La/Sc and La/Co ratios of 0.018–0.046, 0.05–0.22, 0.43–0.86 and 0.14–0.38, respectively. In contrast, the values of these same ratios in felsic rocks are < 20, < 0.5, 0.13–2.7, 0.84–20.5, 2.5–16.3 and 1.8–13.8, respectively (Cullers, Reference Cullers2000). Our analytical results for the metasedimentary rocks in the upper Ji'an Group show that these same ratios have values of 3.01–36.67, 0.02–0.93, 0.10–4.21, 0.25–6.13, 0.96–18.81 and 0.56–19.27, respectively, which are different from those of mafic rocks, but similar to those of felsic rocks. This geochemical evidence is a further indication that the upper Ji'an Group metasedimentary rocks were derived mainly from an acidic magmatic rock source, and is consistent with the previous observation that almost all samples fall within the acidic arc source on Figure 11b (Floyd & Leveridge, Reference Floyd and Leveridge1987).
The zircon dating results for our metasedimentary samples show the existence of numerous magmatic zircons with ages of 2020–2545 Ma (peaks at 2035, 2082, 2178, 2343–2421 and 2451–2545 Ma), along with a few grains with even older ages of 2643–3446 Ma (online Supplementary Material Table S2 available at http://journals.cambridge.org/geo; Figs 9, 10a). The relatively young set of ages is consistent with the ages of K-rich granites in southeastern Jilin Province (c. 2084 Ma), the Liao'Ji granites (2176–2143 Ma; Lu et al. Reference Lu, Wu, Lin, Sun, Zhang and Guo2004 a,b, Reference Lu, Wu, Guo, Wilde, Yang, Liu and Zhang2006; Li et al. Reference Li, Zhao, Sun, Han, Hao, Luo and Xia2005; Wan et al. Reference Wan, Song, Liu, Wilde, Wu, Shi, Yin and Zhou2006; Li & Zhao, Reference Li and Zhao2007), meta-mafic rocks in central Liaodong Peninsula (2.17–2.15 Ga; Hao et al. Reference Hao, Li, Zhao, Sun, Han and Zhao2004; Li & Chen, Reference Li and Chen2014; Meng et al. Reference Meng, Liu, Liu, Liu and Yang2014; Li et al. Reference Li, Chen, Liu, Zhang and Yang2015) and metavolcanic rocks within the Jiao–Liao–Ji Belt (2.19–2.16 Ga; Li & Chen, Reference Li and Chen2014; Yang, Chen & Yan, Reference Yang, Chen and Yan2015; Chen et al. Reference Chen, Li, Wang, Zhang and Yan2016; Meng et al. in press). The older sets of zircon ages (from relatively few grains) are similar to the ages of inherited or captured zircons within the rocks mentioned above, the ages of rocks in the Liaobei–Jinan Complex to the north and the Liaonan–Nangrim Complex to the south (3.85–2.50 Ga; Liu et al. Reference Liu, Nutman, Compston, Wu and Shen1992; Song et al. Reference Song, Nutman, Liu and Wu1996; Wu et al. Reference Wu, Zhao, Wilde and Sun2005, Reference Wu, Zhang, Yang, Xie and Yang2008; Liu, Wan & Wu, Reference Liu, Wan, Wu, Van Kranendonk, Smithies and Bennett2007; Zhang et al. Reference Zhang, Ying, Tang, Li, Feng and Santosh2011; Meng et al. Reference Meng, Liu, Liu, Cui, Yang, Shi, Kong and Lian2013c ), and the ages of magmatic detrital zircons in the metamorphosed supracrustal rocks of the Jiao–Liao–Ji Belt (Luo et al. Reference Luo, Sun, Zhao, Li, Xu, Ye and Xia2004, Reference Luo, Sun, Zhao, Li, Ayers, Xia and Zhang2008; Lu et al. Reference Lu, Wu, Guo, Wilde, Yang, Liu and Zhang2006; Zhang et al. Reference Zhang, Ying, Tang, Li, Feng and Santosh2011; Meng et al. Reference Meng, Liu, Cai and Cui2013a ,Reference Meng, Liu, Cui, Liu, Liu and Shi b ; Li et al. Reference Li, Chen, Liu, Zhang and Yang2015; Liu et al. Reference Liu, Liu, Wang, Liu and Cai2015; Wang et al. Reference Wang, Ren, Lu, Kang, Chu, Yu and Zhang2015). Taking into account all the geochemical evidence discussed above, we consider that the original sediments of the metasedimentary rocks in the upper Ji'an Group were derived mainly from Palaeoproterozoic granites and coeval acidic metavolcanic rocks, with minor contributions from granitic rocks in the Archaean basement.
6.c. Protolith formation and metamorphic ages of metasedimentary rocks in the Ji'an Group
Many studies have reported the depositional ages of metamorphosed Palaeoproterozoic crustal rocks in the Jiao–Liao–Ji Belt, but this research was concentrated mainly on the South and North Liaohe groups of Liaoning Province. For example, Luo et al. (Reference Luo, Sun, Zhao, Li, Xu, Ye and Xia2004, Reference Luo, Sun, Zhao, Li, Ayers, Xia and Zhang2008) considered the maximum depositional ages of the protoliths of the South and North Liaohe groups to be 2.03 Ga, and that both groups of rocks were metamorphosed at c. 1.93–1.90 Ga, as indicated by U–Pb dating of zircons in biotite schists, mica schists and biotite gneisses. Li et al. (Reference Li, Chen, Liu, Zhang and Yang2015) considered the depositional age of the protolith of the North Liaohe Group to be 1.98–1.90 Ga, based on a geochronological study of three metasedimentary rocks. Meng et al. Reference Meng, Liu, Cui, Liu, Liu and Shi(2013b) determined a depositional age of 2.03–1.89 Ga for the South Liaohe Group, based on a geochronological study of the upper sedimentary rocks. Lu et al. (Reference Lu, Wu, Guo, Wilde, Yang, Liu and Zhang2006) proposed a maximum depositional age of c. 2.0 Ga for the protoliths of the South and North Liaohe groups, and proposed that the rocks in both areas were metamorphosed at 1.85 Ga. Although there are some differences in the proposed timing of events, it seems to be generally agreed that the deposition of the protoliths in the North and South Liaohe groups occurred between 2.03 and 1.90 Ga. There has been less research on the depositional age of the Ji'an Group, although Lu et al. (Reference Lu, Wu, Guo, Wilde, Yang, Liu and Zhang2006) suggested that the depositional ages of the protoliths of the gneisses and amphibolites fell between 2.13 and 1.98 Ga; however, these dating results are flawed because of marked Pb loss and the fact that the amphibolites only contained metamorphic zircons. An amphibolite from the Ji'an Group yielded a metamorphic age of 1864±8 Ma (Wang et al. Reference Wang, Ren, Lu, Kang, Chu, Yu and Zhang2015). Liu et al. (Reference Liu, Liu, Wang, Liu and Cai2015) suggested a depositional age of 2.10–1.95 Ga for the Ji'an Group metasediments, based on a geochronological study. However, in all these studies the nature of the protolith of the metamorphic rocks was not fully investigated, and the attributions of ages are therefore open to question. In addition, the variations in the determined depositional ages are also relatively large.
Our study of the metasedimentary rocks from the upper part of the Ji'an Group has allowed us to establish the characteristics of the sedimentary protolith (Fig. 6a). Zircon CL images, trace element analyses and U–Pb data show that all zircons with ages of 1861–1846 Ma (peak at 1855 Ma) and 1926–1889 Ma (peak at 1912 Ma) are grey, homogeneous or cloud-like in appearance under CL, and they have low Th/U ratios (Figs 8, 9), indicating a metamorphic origin (Tomaschek et al. Reference Tomaschek, Kennedy, Villa, Lagos and Ballhaus2003; Dubińska, Bylina & Kozłowski, Reference Dubińska, Bylina and Kozłowski2004). These two peak ages of metamorphism are also consistent with the ages of metamorphism of the metavolcanic rocks in the lower Ji'an Group (Meng et al. in press), the above-mentioned South and North Liaohe groups (Luo et al. Reference Luo, Sun, Zhao, Li, Xu, Ye and Xia2004, Reference Luo, Sun, Zhao, Li, Ayers, Xia and Zhang2008; Lu et al. Reference Lu, Wu, Guo, Wilde, Yang, Liu and Zhang2006; Meng et al. Reference Meng, Liu, Cui, Liu, Liu and Shi2013b ), the meta-mafic rocks in the mid-eastern part of Liaodong Peninsula (1.91 Ga; Li & Chen, Reference Li and Chen2014; Meng et al. Reference Meng, Liu, Liu, Liu and Yang2014) and the two ages of metamorphism (1.9 and 1.87–1.85 Ga) for the Palaeoproterozoic Nanpu Group in the Nangrim Block of the Korean Peninsula (Zhao et al. Reference Zhao, Zhang, Wu, Li, Yang, Jin and Cui2016), all of which indicate the wider region underwent two metamorphic events at 1.91 and 1.85 Ga.
All the other zircons with concordant ages ranging from 2020 to 3446 Ma display growth zones or stripe-like absorption patterns under CL, which, together with their high Th/U ratios, indicate a magmatic origin (Hoskin & Ireland, Reference Hoskin and Ireland2000; Belousova et al. Reference Belousova, Griffin, O'Reilly and Fisher2002). Online Supplementary Material Table S1 (available at http://journals.cambridge.org/geo) shows that the youngest peak ages for these magmatic zircons vary from 2020 to 2053 Ma (peak at 2035 Ma, Fig. 10a), which is also consistent with our unpublished data for metasedimentary rocks of the Laoling and Guanghua groups, and of the North and South Liaohe groups (2.03 Ga; Luo et al. Reference Luo, Sun, Zhao, Li, Xu, Ye and Xia2004, Reference Luo, Sun, Zhao, Li, Ayers, Xia and Zhang2008; Meng et al. Reference Meng, Liu, Cui, Liu, Liu and Shi2013b ; Li et al. Reference Li, Chen, Liu, Zhang and Yang2015). Moreover, this peak age is also consistent with the geochronological results for metasedimentary rocks in the Jiande area of Korea (peak at 2.02 Ga; Liao et al. Reference Liao, Zhang, Jin, Piao and Zheng2016). All these pieces of evidence point to a maximum depositional age of 2.03 Ga for the protoliths of the metasedimentary rocks in the upper Ji'an Group, and two metamorphic events at 1.91 and 1.85 Ga. In addition, older magmatic zircons with ages of 3446–2069 Ma have been recorded (Figs 9, 10a), and these ages are also clearly consistent with those of the metasedimentary rocks in the Machollyong Group of Korea (Liao et al. Reference Liao, Zhang, Jin, Piao and Zheng2016), indicating that they all may have been derived from Neoarchaean–Palaeoproterozoic granitic rocks, as well as the metamorphosed crustal rocks mentioned above (Luo et al. Reference Luo, Sun, Zhao, Li, Xu, Ye and Xia2004, Reference Luo, Sun, Zhao, Li, Ayers, Xia and Zhang2008; Zhao et al. Reference Zhao, Sun, Wilde and Li2005; Lu et al. Reference Lu, Wu, Guo, Wilde, Yang, Liu and Zhang2006; Li & Zhao, Reference Li and Zhao2007; Yang et al. Reference Yang, Wu, Xie and Liu2007; Wang et al. Reference Wang, Lu, Chu, Xiang, Zhang and Liu2011, Reference Wang, Ren, Lu, Kang, Chu, Yu and Zhang2015; Zhang et al. Reference Zhang, Ying, Tang, Li, Feng and Santosh2011; Meng et al. Reference Meng, Liu, Cai and Cui2013a ,Reference Meng, Liu, Cui, Liu, Liu and Shi b ,Reference Meng, Liu, Liu, Cui, Yang, Shi, Kong and Lian c , Reference Meng, Liu, Liu, Liu and Yang2014; Li & Chen, Reference Li and Chen2014; Liu et al. Reference Liu, Liu, Wang, Liu and Cai2015).
6.d. Tectonic setting of Palaeoproterozoic metasedimentary rocks of the Ji'an Group
Two main models have been proposed to explain the tectonic history of the Jiao–Liao–Ji Belt. The first is that formation of the belt involved the opening and closing of an intra-continental rift along the eastern continental margin of the NCC (Zhang & Yang, Reference Zhang and Yang1988; Peng & Palmer, Reference Peng and Palmer1995), and this model has received support from previous studies (Chen, Li & Zhang, Reference Chen, Li and Zhang2003; Li et al. Reference Li, Hao, Han and Zhao2003, Reference Li, Zhao, Sun, Liu, Hao, Han, Luo and Yang2004, Reference Li, Zhao, Sun, Han, Hao, Luo and Xia2005, Reference Li, Zhao, Sun, Han, Zhao and Hao2006; Luo et al. Reference Luo, Sun, Zhao, Li, Xu, Ye and Xia2004, Reference Luo, Sun, Zhao, Li, Ayers, Xia and Zhang2008; Zhao et al. Reference Zhao, Sun, Wilde and Li2005; Li & Zhao, Reference Li and Zhao2007). The second model is that the belt represents a continent– or arc–continent collisional belt (Bai, Reference Bai1993; Reference He and YeHe & Ye, 1998a ,b; Faure et al. Reference Faure, Lin, Moni and Bruguier2004; Lu et al. Reference Lu, Wu, Guo, Wilde, Yang, Liu and Zhang2006; Wang et al. Reference Wang, Lu, Chu, Xiang, Zhang and Liu2011, Reference Wang, Ren, Lu, Kang, Chu, Yu and Zhang2015; Li & Chen, Reference Li and Chen2014; Meng et al. Reference Meng, Liu, Liu, Liu and Yang2014; Li et al. Reference Li, Chen, Liu, Zhang and Yang2015; Yang, Chen & Yan, Reference Yang, Chen and Yan2015; Chen et al. Reference Chen, Li, Wang, Zhang and Yan2016).
Rift opening and closure model. This model was first proposed by Zhang, Yang & Liu (Reference Zhang, Yang and Liu1988) and has been repeatedly modified by other workers (Li et al. Reference Li, Hao, Han and Zhao2003, Reference Li, Zhao, Sun, Liu, Hao, Han, Luo and Yang2004, Reference Li, Zhao, Sun, Han, Hao, Luo and Xia2005, Reference Li, Zhao, Sun, Han, Zhao and Hao2006, Reference Li, Zhao, Santosh, Liu and Dai2011, Reference Li, Zhao, Liu, Dai, Suo, Song and Wang2012; Hao et al. Reference Hao, Li, Zhao, Sun, Han and Zhao2004; Luo et al. Reference Luo, Sun, Zhao, Li, Xu, Ye and Xia2004, Reference Luo, Sun, Zhao, Li, Ayers, Xia and Zhang2008; Li & Zhao, Reference Li and Zhao2007). The general view is that the Palaeoproterozoic metamorphosed crustal rocks in the Liao–Ji area represent the volcanic and sedimentary deposits within a continental rift system, that the Longgang and Nangrim blocks were originally part of a single continental block, and that the formation of the Jiao–Liao–Ji Belt was related to the early Palaeoproterozoic opening of this intracontinent rift system and its closure in late Palaeoproterozoic time. The main evidence for this model includes: (1) the Liao–Ji granites being A-type granites that formed during the early stage of rifting (Zhang, Yang & Liu, Reference Zhang, Yang and Liu1988; Sun et al. Reference Sun, Armstrong, Lambert, Jiang and Wu1993; Peng & Palmer, Reference Peng and Palmer1995; Li et al. Reference Li, Hao, Han and Zhao2003; Li & Zhao, Reference Li and Zhao2007); (2) the felsic rocks (traditionally called leptynites and leptites) and amphibolites within the Liaohe Group being representatives of a bimodal volcanic rock assemblage (Sun et al. Reference Sun, Armstrong, Lambert, Jiang and Wu1993; Peng & Palmer, Reference Peng and Palmer1995; Li et al. Reference Li, Han, Liu, Yang and Ma2001, Reference Li, Hao, Han and Zhao2003); (3) the South and North Liaohe groups having developed structures related to early extension and late compression (Li et al. Reference Li, Zhao, Sun, Han, Hao, Luo and Xia2005); and (4) the South Liaohe and Ji'an groups having followed a low-pressure counterclockwise P–T path (Lu, Reference Lu1996; He & Ye, Reference He and Ye1998a ,Reference He and Ye b ).
Continental-arc–continent collision model. This model, first proposed by Bai (Reference Bai1993), involves the South and North Liaohe groups belonging in common to either the south margin of the Longgang Block (Bai, Reference Bai1993; Wang et al. Reference Wang, Lu, Chu, Xiang, Zhang and Liu2011, Reference Wang, Ren, Lu, Kang, Chu, Yu and Zhang2015) or the north margin of the Nangrim Block (Faure et al. Reference Faure, Lin, Moni and Bruguier2004; Lu et al. Reference Lu, Wu, Lin, Sun, Zhang and Guo2004 a,b; Lu et al. Reference Lu, Wu, Guo, Wilde, Yang, Liu and Zhang2006). The main evidence for this model includes: (1) considering the Archaean Longgang and Nangrim blocks to be significantly different in their rock assemblages, ages and metamorphism, so that the Longgang Block has ages of 3.8–2.5 Ga with granitic rocks dominant, as well as a granulite facies metamorphism (Wu et al. Reference Wu, Geng, Shen, Wan, Liu and Song1998), while the Nangrim Block has ages of 2.50–2.44 Ga, with quartz diorites and tonalites dominant (Sun, Lin & Ge, Reference Sun, Lin and Ge1992), as well as an amphibolite-facies metamorphism; (2) considering the metavolcanic rocks and mafic intrusions within the Jiao–Liao–Ji Belt to be the products of arc magmatism (Bai & Dai, Reference Bai, Dai, Ma and Bai1998; Faure et al. Reference Faure, Lin, Moni and Bruguier2004; Lu et al. Reference Lu, Wu, Guo, Wilde, Yang, Liu and Zhang2006; Wang et al. Reference Wang, Lu, Chu, Xiang, Zhang and Liu2011, Reference Wang, Ren, Lu, Kang, Chu, Yu and Zhang2015; Li & Chen, Reference Li and Chen2014; Meng et al. Reference Meng, Liu, Liu, Liu and Yang2014; Li et al. Reference Li, Chen, Liu, Zhang and Yang2015; Chen et al. Reference Chen, Li, Wang, Zhang and Yan2016); (3) considering the North Liaohe and Laoling groups to have followed a medium-pressure clockwise P–T path (He & Ye, Reference He and Ye1998a ,Reference He and Ye b ).
Continent–continent (terranes) collision model. This model was proposed by Reference He and YeHe & Ye (1998a ,b), and they point to obvious differences in the rock assemblages and metamorphism of the South and North Liaohe groups, which should therefore belong to different continental margins. In other words, the Longgang and Nangrim blocks were two independent blocks before Palaeoproterozoic time, and the formation of the Jiao–Liao–Ji Belt was related to the 1.9–1.8 Ga collision between these two blocks.
These various views give rise to the following question: In what tectonic setting did the Jiao–Liao–Ji Belt form? Although our whole-rock geochemical and zircon U–Pb and Hf isotopic data are not able to unequivocally resolve this controversy, the data do provide constraints on the above models. As mentioned above, our metasedimentary samples are enriched in LREEs, depleted in HREEs, have negative Eu anomalies, relatively high ratios of La/Sc, La/Y and Ti/Zr, and low ratios of Sc/Cr, all of which suggests sedimentary rocks that were deposited in an active continental margin setting (Bhatia & Crook, Reference Bhatia and Crook1986; Long et al. Reference Long, Sun, Yuan, Xiao and Cai2008). Moreover, the values of δCe in these samples vary between 0.84 and 1.28, which is significantly higher than at mid-ocean ridges (0.29) or in basaltic basins (0.55), but similar to continental margin basalts (0.90–1.30; Bhatia & Crook, Reference Bhatia and Crook1986; Murray et al. Reference Murray, Buchholtz ten Brink, Jones, Gerlach and Russ1990). These observations are also consistent with the high ICV and low CIA values of our samples, which indicate an active tectonic setting (Fig. 11a; Floyd & Leveridge, Reference Floyd and Leveridge1987) and an acidic arc source (Fig. 11b; Floyd & Leveridge, Reference Floyd and Leveridge1987). In tectonic discrimination diagrams such as La/Sc–Ti/Zr, Th–Co–Zr/10 and Th–Sc–Zr/10 (Bhatia & Crook, Reference Bhatia and Crook1986), nearly all our samples plot in or around the field of an active continental margin (Bhatia & Crook, Reference Bhatia and Crook1986; Long et al. Reference Long, Sun, Yuan, Xiao and Cai2008) (Fig. 12). In addition, lots of latest studies on the continuous calc-alkaline metavolcanic rocks within the Ji'an (Meng et al. Reference Meng, Wang, Yang, Cai, Ji and Li2017) and South Liaohe groups (our unpub. data), a clockwise metamorphic evolutionary P–T trajectory on Al-rich gneisses of the Ji'an, South Liaohe and Jingshan groups (Liu et al. Reference Liu, Liu, Wang, Liu and Cai2015), middle Palaeoproterozoic meta-mafic rocks in the centre of Liaodong Peninsula (Li et al. Reference Li, Chen, Liu, Zhang and Yang2015; Li & Chen, Reference Li and Chen2014; Meng et al. Reference Meng, Liu, Liu, Liu and Yang2014; Yang, Chen & Yan, Reference Yang, Chen and Yan2015; Chen et al. Reference Chen, Li, Wang, Zhang and Yan2016) and adakitic granites with ages of c. 1.90 Ga in the central–eastern Liaodong Peninsula (Wang et al. Reference Wang, Ren, Lu, Kang, Chu, Yu and Zhang2015), indicated the existence of the Palaeoproterozoic subduction within the northern segment of the Jiao–Liao–Ji Belt. Taking all the above into account, we consider that the protoliths of the metasedimentary rocks in the Ji'an Group have arc-related geochemical features, and the formation of them could be also related to subduction.

Figure 12. Tectonic discrimination diagrams (Bhatia & Crook, Reference Bhatia and Crook1986) for the analysed metasedimentary rocks in the study area. (a) Co–Th–Zr/10 diagram. (b) Sc–Th–Zr/10 diagram. (c) Ti/Zr versus La/Sc diagram. OIA – oceanic island arc; CIA – continental island arc; ACM – active continental margin; PM – passive continental margin.
However, although the Palaeoproterozoic rocks mentioned above show the geochemical features of arc-related magmatism (Li et al. Reference Li, Chen, Liu, Zhang and Yang2015; Chen et al. Reference Chen, Li, Wang, Zhang and Yan2016; Liao et al. Reference Liao, Zhang, Jin, Piao and Zheng2016), such studies were unable to account completely for the regional tectono-metamorphic differences (Li et al. Reference Li, Zhao, Sun, Han, Hao, Luo and Xia2005) and obscured contact relationships (Liu et al. Reference Liu, Liu, Wang, Liu and Cai2015) within the North and South zones of the Jiao–Liao–Ji Belt. All these studies, taking into account the occurrence of middle Palaeoproterozoic A-type granites and coeval metamorphosed mafic rocks in the northeast of the study area, which probably formed in an extensional tectonic setting (Li et al. Reference Li, Hao, Han and Zhao2003, Reference Li, Zhao, Sun, Liu, Hao, Han, Luo and Yang2004, Reference Li, Zhao, Sun, Han, Hao, Luo and Xia2005; Hao et al. Reference Hao, Li, Zhao, Sun, Han and Zhao2004; Li & Zhao, Reference Li and Zhao2007; Li et al. Reference Li, Chen, Liu, Zhang and Yang2015; Meng et al. Reference Meng, Liu, Liu, Liu and Yang2014; Chen et al. Reference Chen, Li, Wang, Zhang and Yan2016), and also provided an important source for the sediments of the Ji'an Group, lead us to the following inferences: (1) the regional Palaeoproterozoic and late Archaean granitoids older than 2.17 Ga formed the basement to the younger metamorphosed sedimentary rocks (Li et al. Reference Li, Hao, Han and Zhao2003, Reference Li, Zhao, Sun, Han, Zhao and Hao2006, Reference Li, Zhao, Santosh, Liu and Dai2011, Reference Li, Zhao, Liu, Dai, Suo, Song and Wang2012; Luo et al. Reference Luo, Sun, Zhao, Li, Xu, Ye and Xia2004, Reference Luo, Sun, Zhao, Li, Ayers, Xia and Zhang2008; Li & Zhao, Reference Li and Zhao2007), and protoliths of the latter formed in a complex tectonic setting; (2) subduction existed within the northern segment of the Jiao–Liao–Ji Belt in middle Palaeoproterozoic time (Li & Chen, Reference Li and Chen2014; Meng et al. Reference Meng, Liu, Liu, Liu and Yang2014, Reference Meng, Wang, Yang, Cai, Ji and Li2017; Li et al. Reference Li, Chen, Liu, Zhang and Yang2015; Yang, Chen & Yan, Reference Yang, Chen and Yan2015; Chen et al. Reference Chen, Li, Wang, Zhang and Yan2016), and eventually led to the collision and amalgamation of the Longgang and Nangrim blocks to form a coherent and uniform block; (3) such an amalgamation in the southeastern part of Jilin Province could not be finished before 2.03 Ga, i.e. the protolith formation age (i.e. 2.03 Ga) of the metasedimentary rocks in the Ji'an Group, and these rocks in the studied area formed in an active continental margin tectonic setting.
6.e. Constraints on crustal growth and the evolution of the northeastern margin of the Eastern Block
The closure temperature for Hf in zircon is so high that it is unaffected even by later magmatic processes or high-grade metamorphism, except when rim overgrowths form (Kinny, Compston & Williams, Reference Kinny, Compston and Williams1991; Kinny & Maas, Reference Kinny, Maas, Hanchar and Hoskin2003). Consequently, zircon Lu–Hf isotope studies are an excellent way of tracing and deciphering magmatic sources and petrogenetic processes, and hence tracing the history of crustal growth (Wu et al. Reference Wu, Li, Zheng and Gao2007). This is similar to the use of whole-rock Nd isotope model ages, although the model ages can be disturbed under certain conditions (Gruau et al. Reference Gruau, Rosing, Bridgwater and Gill1996; Moorbath & Kamber, Reference Moorbath and Kamber1998).
The Lu–Hf isotopic results suggest that these zircons have a variety of magmatic precursors, which is consistent with their complex 207Pb–206Pb and (TC DM ) model ages (Figs 9, 10). Zircons with ancient ages of c. 3446 and 2935–2923 Ma have negative εHf values (–0.19 and –0.76 to –0.55; Fig. 10b) as well as older (TC DM ) model ages (3.76 and 3.41–3.39 Ga; online Supplementary Material Table S3 available at http://journals.cambridge.org/geo), indicating two crustal recycling events and suggesting that their main sources were Neoarchaean to Palaeoarchaean crustal rocks, in spite of the lack of exposures of any such rocks with these ages in the immediate area. Zircons with ages of c. 3234–3054 Ma have positive εHf values (+4.79 to +5.59) and most plot close to the depleted mantle evolution line (Fig. 10b; online Supplementary Material Table S3 available at http://journals.cambridge.org/geo). These features and their (TC DM ) model ages of 3.23–3.15 Ga (close to their U–Pb ages; Fig. 10) indicate that these zircons crystallized in magmas produced during the partial melting of a coeval juvenile crust. Most of the εHf values for zircons with ages of c. 2814–2643 Ma are +6.53 to +7.79, but some values are in the range –1.07 to +2.68. These two sets of values plot close to the depleted mantle and chondrite evolution lines, respectively (Fig. 10b; online Supplementary Material Table S3 available at http://journals.cambridge.org/geo). These features, combined with their (TC DM ) model ages of 2.83–2.67 Ga (consistent with their U–Pb ages) and 3.20–2.98 Ga (Fig. 10), indicate that the rocks were derived mostly from the partial melting of coeval juvenile crustal material (of Neoarchaean age), with limited inputs of older Mesoarchaean crust, as indicated by the (TC DM ) model ages of zircons with ages of 3234–3054 Ma (Fig. 10). The εHf values of zircons with ages of c. 2545–2451 Ma are mainly in the range of +1.19 to +8.05, but a few are in the range –6.95 to –0.35. The corresponding (TC DM ) model ages are 2.90–2.54 and 3.44–3.05 Ga (Fig. 10b; online Supplementary Material Table S3 available at http://journals.cambridge.org/geo), respectively. These features suggest that the zircons crystallized from magmas derived from the partial melting of Mesoarchaean to Neoarchaean juvenile crustal material, with small contributions from older crustal rocks, which is consistent with studies of mafic volcanism in the Lushan region (Liu et al. Reference Liu, Wang, Rolfo, Groppo, Gu and Song2009) and tonalite–trondhjemite–granodiorite (TTG) gneisses in the Luxi (Du et al. Reference Du, Zhuang, Yang, Wan, Wang, Wang and Zhang2003, Reference Du, Yang, Zhuang, Wei, Wan, Ren and Hou2010; Wang, Reference Wang2009), Jiaodong (Jahn et al. Reference Jahn, Liu, Wan, Song and Wu2008) and Fuping and Hengshan (Guan et al. Reference Guan, Sun, Wilde, Zhou and Zhai2002; Kröner et al. Reference Kröner, Wilde, Li and Wang2005) regions. All these data indicate a significant event that involved coeval juvenile crustal growth accompanied by limited recycling of older crustal rocks. The zircon population of 2431–2343 Ma displays εHf values from –5.18 to +4.82 and (TC DM ) model ages of 3.29–2.61 Ga (clearly older than their U–Pb ages), which suggests an input from both reworked and juvenile crustal material (Fig. 10b; online Supplementary Material Table S3 available at http://journals.cambridge.org/geo).
The main zircon population with ages of 2288–2069 Ma have positive εHf values (+1.79 to +9.76) with (TC DM ) model ages of 2.74–2.08 Ga, and plot close to the depleted mantle evolution line (Fig. 10b; online Supplementary Material Table S3 available at http://journals.cambridge.org/geo), which indicates that these zircons crystallized from magmas that were derived directly from the partial melting of coeval juvenile crust, indicating a significant and continuous Palaeoproterozoic crustal growth event. The zircon population with ages of 2053–2021 Ma have εHf values and (TC DM ) model ages of –3.69 to +8.78 and 2.90–2.11 Ga, and the wide range of εHf values and the relatively old (TC DM ) model ages indicate both the recycling of an older crust and the addition of juvenile crustal material (Fig. 10b; online Supplementary Material Table S3 available at http://journals.cambridge.org/geo). The metamorphic zircons with a peak age of 1855 Ma have εHf values that vary from –7.92 to –0.41 and (TC DM ) model ages of 3.03 to 2.56 Ga, which are much older than their U–Pb ages. Likewise, the metamorphic zircons with a peak age of 1912 Ma have εHf values that vary from –3.22 to +3.29, and (TC DM ) model ages of 2.77 to 2.37 Ga, which are much older than the U–Pb ages. Therefore, these data for the metamorphic zircons indicate two-stage metamorphic events at c. 1.85 and 1.91 Ga (Fig. 10b; online Supplementary Material Table S3 available at http://journals.cambridge.org/geo). Based on the above observations, and taking into account the regional geological data for the Jiao–Liao–Ji Belt and neighbouring regions (i.e. the northeastern margin of the Eastern Block in the NCC), we consider: (1) that ancient crustal material as old as 3.76 Ga existed in southeastern Jilin Province or adjacent areas, comparable to the ancient zircons and/or rocks in Anshan, northern Liaoning Province (3.8 Ga; Liu et al. Reference Liu, Nutman, Compston, Wu and Shen1992; Song et al. Reference Song, Nutman, Liu and Wu1996; Wan et al. Reference Wan, Song, Liu, Li, Yang, Zhang, Yang, Geng and Shen2001, Reference Wan, Liu, Song, Wu, Yang, Zhang and Geng2005; Liu, Wan & Wu, Reference Liu, Wan, Wu, Van Kranendonk, Smithies and Bennett2007); and (2) there were multiple events involving crustal growth, with the main events taking place at 3.23–3.05, 2.80–2.65, 2.54–2.45 and 2.28–2.08 Ga, and with two-stage metamorphic events taking place at c. 1.91 and 1.85 Ga (Fig. 10b; online Supplementary Material Table S3 available at http://journals.cambridge.org/geo).
7. Conclusions
Our geochemical, isotopic and geochronological study of the metasedimentary rocks of the upper Ji'an Group, SE Jilin, NE China, has led us to the following conclusions.
(1) The protoliths of the metasedimentary rocks in the lower part of the Ji'an Group within the northern segment of the Jiao–Liao–Ji Belt were mainly sandstones, greywackes and claystones, together with some shales. New zircon U–Pb data indicate the maximum depositional age of the protoliths to be 2.03 Ga, and the sediments recorded two-stage metamorphic events at 1.91 and 1.85 Ga.
(2) The source of the original upper Ji'an Group sediments was mainly Palaeoproterozoic granites and coeval acid volcanic rocks, along with minor contributions from granitic rocks in the Archaean basement.
(3) The protoliths of the metasedimentary rocks in the upper part of the Ji'an Group were formed in an active continental margin setting.
(4) These rocks provide evidence of the existence of ancient crustal materials as old as 3.76 Ga, multiple crustal growth events that took place along the northeastern margin of the Eastern Block of the NCC at 3.23–3.05, 2.80–2.65, 2.54–2.45 and 2.28–2.08 Ga.
Acknowledgements
This research was supported financially by the National Natural Science Foundation of China (Grant Nos. 41572169, 40725007, and 41202136), the China Geological Survey Programme (Grant No. 12120114021601, 12120114021401, 12120114061901), the Basic Scientific Research Foundation of the Institute of Geology, CAGS (J1507), and the State Key Laboratory of Geological Processes and Mineral Resources, China University of Geosciences (Beijing).
Supplementary material
To view supplementary material for this article, please visit https://doi.org/10.1017/S0016756817000632.