1. Introduction
The late Ordovician and early Silurian graptolite faunas from the northern margin of Gondwana are crucial to determining the age and event stratigraphy of the region's petroleum rich rocks (P. Legrand, unpub. Ph.D. thesis, Univ. Michel de Montaigne-Bordeaux III, 1999; Legrand, Reference Legrand2003; Armstrong et al. Reference Armstrong, Turner, Makhlouf, Weedon, Williams, Al Smadi and Abu Salah2005; Loydell, Reference Loydell2007). This is particularly the case as limestone-hosted shelly faunas are generally absent (Walker, Wilkinson & Ivany, Reference Walker, Wilkinson and Ivany2002; cf. Fortey & Cocks, Reference Fortey and Cocks2005; Cherns & Wheeley, Reference Cherns and Wheeley2007), and most localities yield low-diversity graptoloid faunas comprising long-lived cosmopolitan forms and short-lived endemic taxa (Underwood, Deynoux & Ghienne, Reference Underwood, Deynoux and Ghienne1998; P. Legrand, unpub. Ph.D. thesis, Univ. Michel de Montaigne-Bordeaux III, 1999; Zalasiewicz, Reference Zalasiewicz2001; Legrand, Reference Legrand2003). However, recent detailed studies of more complete successions from the Hirnantian and Llandovery of northern Gondwana (e.g. Underwood, Deynoux & Ghienne, Reference Underwood, Deynoux and Ghienne1998; Štorch & Massa, Reference Štorch, Massa, Salem and Oun2003; Loydell, Reference Loydell2007, Reference Loydell2012 b; Zalasiewicz et al. Reference Zalasiewicz, Williams, Miller, Page and Blackett2007) have shown the total regional graptolite fauna to be comparable to those of peri-Gondwanan Europe, affording relatively detailed biostratigraphic correlation for the very latest Ordovician and early Silurian of northern Gondwana (Loydell, Reference Loydell2007, Reference Loydell2012 a).
Nonetheless, in the late Ordovician of North Africa the picture is more complicated: though the occurrence of an endemic graptoloid fauna has been documented and a regional stratigraphy erected (see P. Legrand, unpub. Ph.D. thesis, Univ. Michel de Montaigne-Bordeaux III, 1999; Legrand, Reference Legrand2003), the precise age of the local zonation remains uncertain. This may be in part owing to a glaciotectonic unconformity that persists through much of Africa and Arabia of Caradoc (viz. Sandbian – mid Katian) to Hirnantian age (Destombes, Holland & Willefert, Reference Destombes, Holland, Willefert and Holland1985; P. Legrand, unpub. Ph.D. thesis, Univ. Michel de Montaigne-Bordeaux III, 1999; Legrand, Reference Legrand2000, Reference Legrand2003; Sutcliffe et al. Reference Sutcliffe, Harper, Salem, Whittington and Craig2001; Ghienne et al. Reference Ghienne, Boumendjal, Paris, Videt, Racheboeuf and Ait Salem2007; A. A. Page, unpub. Ph.D. thesis, Univ. Leicester, 2007; Le Heron & Craig, Reference Le Heron and Craig2008). This unconformity has recently been recognized in the northern and eastern flanks of the Kufra Basin (Le Heron & Howard, Reference Le Heron and Howard2010; Le Heron et al. Reference Le Heron, Armstrong, Wilson, Howard and Gindre2010). Legrand (Reference Legrand2003, p. 19) noted that understanding the regional stratigraphy of the ‘Algerian Sahara and adjacent regions . . . [is hindered by lack of constraint concerning] (a) epeirogenic movements and erosion; (b) the nature of the glacial, periglacial, deltaic and fluvial sediments; (c) one glaciation, multiple glaciations or only a polyphase glaciation; (d) the varying importance of unconformities; (e) the age of the uppermost Ordovician formations, i.e. whether they are uppermost Ashgillian or upper Caradocian–upper Ashgillian and the precise age of the last Hirnantia fauna’. Nevertheless, if Ordovician or early Silurian graptolites can be precisely identified, regional if not global correlation is possible.
In this study, we describe Normalograptus kufraensis, a new species of normalograptid graptolite from a thin marker horizon in the Lower Palaeozoic Jabal Eghei succession of the western Kufra Basin in southern Libya. Though graptolites from the Murzuq Basin in NW Libya have been recently documented (Loydell, Reference Loydell2012 b), the graptolites of the Kufra Basin are poorly known. These specimens, uncovered in CASP fieldwork in November–December 2008, are the first graptolites from Kufra Basin outcrops, and comprise well-preserved specimens that may be of key importance for understanding regional stratigraphy, particularly the timings of glaciation and of the onset of anoxia.
2. Geological setting
Marine and continental Palaeozoic and Mesozoic sandstones and shales of up to 2600 m in thickness fill the Kufra Basin in SE Libya (e.g. Bellini et al. Reference Bellini, Giori, Ashuri, Benelli, Salem, Sbeta and Bakbak1991). This paper describes material recovered from Jabal Eghei, an outcrop belt located at the western margin of the Kufra Basin (Fig. 1). Sections were studied about 100 km north of the Libya–Chad border. The graptolite-bearing bed belongs to the Tanezzuft Formation, which is described below as well as in Le Heron et al. (in press).
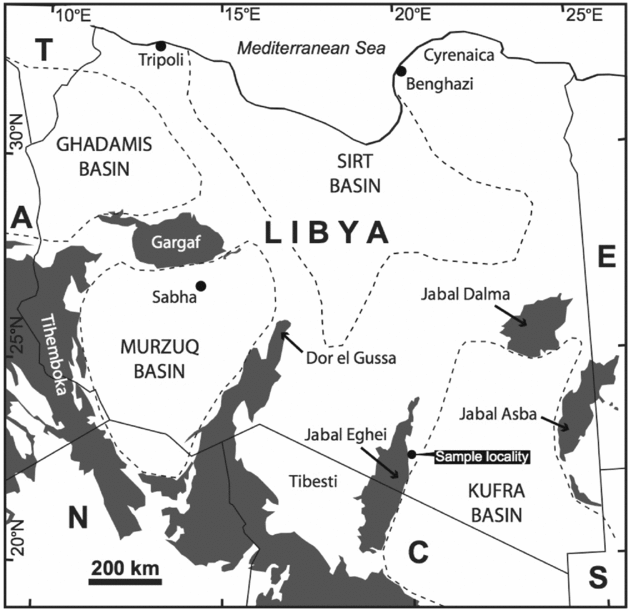
Figure 1. Map of Libya showing surface outcrops with Palaeozoic rocks (dark grey colour). The sampling locality of graptolite-bearing specimens is indicated.
In Jabal Eghei, the Tanezzuft Formation displays distinct sedimentological characteristics not encountered elsewhere in the Kufra Basin (Fig. 2). These include, from the base up, (1) a calcareous, bryozoa-bearing sandstone immediately at the basal contact of the formation to the underlying Mamuniyat Formation, (2) Planolites-bearing sandstones, (3) a soft-sediment striated surface of putative glacial origin (Le Heron et al. Reference Le Heron, Meinhold, Page and Whitham2005), (4) an interval with calcareous, pebble-sized clasts (?dropstones) below and above this surface, (5) a second bioturbated horizon, and further up a shale succession with (6) an impressive (20 cm thick) marker bed containing well-preserved graptolites. The Tanezzuft Formation has a maximum thickness of about 50 m owing to post-depositional erosion.

Figure 2. Schematic stratigraphic column (left) and field photograph (right) to illustrate the location of the graptolite-bearing bed within the Lower Palaeozoic succession of the western margin of the Kufra Basin.
Note that macrofossils are generally rare in the Lower Palaeozoic succession of southern Libya, and palynomorphs have mostly been destroyed at outcrop by intensive surface weathering. This hampers precise dating and correlation with successions elsewhere in Libya and North Africa. More common are trace fossils (e.g. Lüning et al. Reference Lüning, Craig, Fitches, Mayouf, Busrewil, El Dieb, Gammudi, Loydell and McIlroy1999; Seilacher et al. Reference Seilacher, Lüning, Martin, Klitzsch, Khoja and Craig2002), but their use for stratigraphy is limited and controversial. Though drilling boreholes may recover material for palynological analysis from below the weathering zone (e.g. Grignani, Lanzoni & Elatrash, Reference Grignani, Lanzoni, Elatrash, Salem, Hammuda and Eliagoubi1991), it is expensive and time consuming. Where drilling is not possible, the intensive search for macrofossils is the only alternative. In the Kufra Basin, graptolites have only previously been found in boreholes through the Tanezzuft Formation in Jabal Dalma (Grignani, Lanzoni & Elatrash, Reference Grignani, Lanzoni, Elatrash, Salem, Hammuda and Eliagoubi1991). The uppermost shales of the formation have a latest Rhuddanian or earliest Aeronian age (cyphus or triangulatus biozones) based on microfossil data (Lüning et al. Reference Lüning, Craig, Fitches, Mayouf, Busrewil, El Dieb, Gammudi, Loydell and McIlroy1999). Exposures of the basal part of the Tanezzuft Formation were hitherto unknown in the Kufra Basin. Hence, the presence of normalograptids below the microfossil-bearing shales provides new age constraints for the basal part of the Tanezzuft Formation. As the graptolite-bearing marker bed overlies putative glacially-influenced deposits, this delimits both the temporal extent of ice cover and the onset of anoxic shale deposition in the Kufra Basin.
3. Material and method
This paper presents the study of over 30 graptolites preserved on 15 weathered slabs collected from exposures. Specimens CAM SM X.50191.1 to X.50191.15 were recovered from a ~ 20 cm thick marker bed exposed in Jabal Eghei (N 22.662307, E 19.962156) at the western margin of the Kufra Basin, southern Libya (Figs 1, 2). This bed is characterized by its more brownish colour compared to the surrounding greyish–reddish shales. In addition to the graptolite fauna, the same horizon or a horizon a few centimetres above yielded specimens X.50191.16 (N 22.71759, E 19.970541), a brachiopod similar to ?Eocoelia (D. A. T. Harper, Copenhagen, pers. comm. to G. Meinhold), and X.50191.18 (N 22.662307, E 19.962156), an orthoconic nautiloid cephalopod (Fig. 3). The latter preserves no morphological features, precluding identification at a higher taxonomic level. Though the brachiopod cannot be confidently ascribed to Eocoelia, this genus is well known from the early Silurian (e.g. Jin, Reference Jin2003). There may be other Eocoelia-like brachiopods in older strata, however.

Figure 3. Shelly fossils from the Tanezzuft Formation in Jabal Eghei, western Kufra Basin. (a) Brachiopod similar to ?Eocoelia X.50191.16. (b) Orthocone, X.50191.17.
The graptolites were studied using reflected light microscopy, with selected specimens measured, drawn and/or photographed. Measurements and characters recorded in these graptolites (Table 1) follow those described by Loydell (Reference Loydell2007) as useful for identifying biserial graptolites with particular attention paid to dorso-ventral widths (DVWs) and two theca repeat distances (2TRDs) sensu Howe (Reference Howe1983). These measurements are critical in speciating normalograptids, which tend otherwise to be very morphologically similar (cf. Melchin, Reference Melchin2008; Blackett et al. Reference Blackett, Page, Zalasiewicz, Williams, Rickards and Davies2009). One major problem in the identification of biserial graptolites in high latitude sequences is that they may show considerable intraspecific variation, especially in the genus Normalograptus (e.g. Legrand, Reference Legrand1977, Reference Legrand2000, Reference Legrand2009; P. Legrand, unpub. Ph.D. thesis, Univ. Michel de Montaigne-Bordeaux III, 1999; Loydell, Reference Loydell2007) with identification requiring measurements taken at several different levels along the rhabdosome in tens of specimens.
Table 1. Mean thecal measurements and standard deviations (σ) in millimetres for the population of Normalograptus kufraensis from the graptolite-bearing bed of the Tanezzuft Formation in Jabal Eghei, Kufra Basin, Libya

As well as comparing the measurements of N. kufraensis with the type material and recent descriptions of N. ajjeri, N. angustus and N. arrikini, species which bear a close resemblance to it (Table 2), Table 3 compares it with width and thecal packing measurements in 44 other graptolites assigned to Normalograptus. Measurements have been extracted from descriptions in recent publications, giving as representative a view of current species concepts as possible. References to type material are also included. The list of taxa is far from exhaustive. For example, Legrand (unpub. Ph.D. thesis, Univ. Michel de Montaigne-Bordeaux III, 1999) included many taxa that have never been formally described, and the fusiform Mauritanian specimens of Underwood, Deynoux & Ghienne (Reference Underwood, Deynoux and Ghienne1998) have been omitted as no measurements were given in that work. Taxa such as N. bicaudatus (Chen & Lin, Reference Chen and Lin1978), N. bifurcatus Loydell (Reference Loydell2007), N. lubricus (Chen & Lin, Reference Chen and Lin1978), N. minor (Huang, Reference Huang1982), N. rhizinus (Li & Yang, Reference Li and Yang1983) and N. trifilis (Manck, Reference Manck1923) have been excluded as they are readily identified by their proximal ornamentation. Taxa with elaborate nemata are included in the list, however, as this feature may not be so readily preserved. Taxa only known from pre-Hirnantian strata have been omitted as many problems have arisen from Katian or older climacograptids being misidentified as Normalograptus (Štorch et al. Reference Štorch, Mitchell, Finney and Melchin2011) and recent monographic studies of these strata are comparatively rare. This low-diversity older fauna is listed in Goldman et al. (Reference Goldman, Mitchell, Melchin, Fan and Wu2011), which shows that N. normalis and N. angustus were the only species to survive the mid Katian extirpation and range into the Hirnantian when Normalograptus diversified markedly. Though the measurements given in Table 3 are the basis of much of the classification of Normalograptus, further information such as the nature of the supragenicular wall and median septum or degree of geniculation should be assessed before a confident diagnosis can be made. In addition to giving minimum and maximum values for thecal measurements (e.g. Tables 2, 3), future authors may wish to quote average measurements of mean plus or minus standard deviation (e.g. Table 1) and illustrate graphs of measurements in similar taxa (e.g. Loydell, Reference Loydell2007, text-fig. 9; Blackett et al. Reference Blackett, Page, Zalasiewicz, Williams, Rickards and Davies2009, fig. 6). These techniques allow comparison and distinction more readily. Nonetheless, the data in Table 3 may be of some use for distinguishing taxa within what is often a morphologically anonymous group.
Table 2. Comparative thecal measurements in millimetres for graptolites similar to Normalograptus kufraensis sp. nov including populations from type localities as highlighted

* – th 12; † – th 11; ‡ – distal max.
All measurements are as quoted in cited texts, except for 2TRDs from Legrand (Reference Legrand1977, Reference Legrand2009, unpub. Ph.D. thesis, Univ. Michel de Montaigne-Bordeaux III, 1999) and Štorch & Feist (Reference Štorch and Feist2008) and DVWs – excluding distal maxima recorded in Legrand (Reference Legrand1977, Reference Legrand2009, unpub. Ph.D. thesis, Univ. Michel de Montaigne-Bordeaux III, 1999) and Štorch & Feist (Reference Štorch and Feist2008) – which were taken from figured specimens in those works.
Table 3. Comparative thecal measurements in millimetres for normalograptids

* – proximal; † – th4; ‡ – th6; § – th10; ∥ – th 11; ¶ – throughout; ** – fusiform
[1] – Hirnantian–Rhuddanian of central Wales, UK (Blackett et al. Reference Blackett, Page, Zalasiewicz, Williams, Rickards and Davies2009); [2] – localities listed in Loydell (Reference Loydell2007, text-fig. 9) as given in Blackett et al. (Reference Blackett, Page, Zalasiewicz, Williams, Rickards and Davies2009); [3] – Hirnantian of the Yangtze Region, China (Chen et al. Reference Chen, Fan, Melchin and Mitchell2005); [4] – Rhuddanian of the Kurama Range, Uzbekistan (Koren’ & Melchin, Reference Koren’ and Rickards2004); [5] – Llandovery of the southern Urals, Russia (Koren’ & Rickards, Reference Koren’ and Rickards2004); [6] – Hirnantian of Algeria (Legrand, Reference Legrand2009); [7] – Hirnantian–Rhuddanian of Jordan (Loydell, Reference Loydell2007); [8] – Hirnantian or Llandovery of the Southern Uplands, Scotland (Loydell, Reference Loydell2007); [9] – Rhuddanian of the Murzuq Basin, Libya (Loydell, Reference Loydell2012 b); [10] – Hirnantian of Nevada, USA (Štorch et al. Reference Štorch, Mitchell, Finney and Melchin2011); [11] – Table 2 (this study); [12] – Rhuddanian of the Wye Valley, Wales, UK (Zalasiewicz & Tunnicliff, Reference Zalasiewicz and Tunnicliff1994). All measurements are as quoted in descriptions or tables. An Excel spreadsheet version of these data is available from the authors on request.
4. Preservation and palaeoecology
The graptolites are preserved in heavily weathered shales. They are generally cast as bas-relief impressions, though some specimens preserve periderm or the residue thereof (e.g. Fig. 4a–c). A few specimens are preserved in part-relief as steinkerns of iron oxides pseudomorphing pyrite (e.g. Fig. 4a). Regardless of the degree of weathering or flattening, the morphological fidelity displayed by these fossils can be very high with fusellae apparent in both 2D and 3D specimens. However, fossils occurring in coarser-grained rocks within the collection are more poorly preserved. Most graptolite assemblages display current alignment and many are in scalariform view (see Fig. 5). There is no evidence of tectonic deformation in either the rocks or fossils and the black colour of the periderm suggests little thermal alteration (cf. Goodarzi & Norford, 1989).

Figure 4. Photomicrographs of Normalograptus kufraensis sp. nov. from the graptolite-bearing bed of the Tanezzuft Formation in Jabal Eghei, western Kufra Basin. Specimen numbers: (a) X.50191.10, (b) X.50191.6b, (c) X.50191.6a, (d) X.50191.13. Scale bar = 1 mm.

Figure 5. Camera lucida drawings of Normalograptus kufraensis sp. nov. from the graptolite-bearing bed of the Tanezzuft Formation in Jabal Eghei, western Kufra Basin. Specimen numbers: (a) X.50191.6a, (b) X.50191.3c, holotype, (c) X.50191.1, (d) X.50191.13, (e) X.50191.3e, (f) X.50191.3d, (g) X.50191.14b, (h) X.50191.3a. Scale bars = 2 mm (a–d, f–h); 1 mm (e).
The graptoloid fauna were probably cratonic invaders (sensu Finney & Berry, Reference Finney and Berry1997) that lived above the shelf rather than in the open ocean. This interpretation is consistent with the geological setting and seems sensible given the similarity in preservation and faunal composition with on-shelf assemblages from the Silurian of Saudi Arabia reported by Zalasiewicz et al. (Reference Zalasiewicz, Williams, Miller, Page and Blackett2007). Eocoelia occurs in shallow waters (Ziegler, Cocks & Bambach, Reference Ziegler, Cocks and Bambach1968), and other eocoeliomorphs such as the one in our section could plausibly share this habitat. Pyritization or partial-pyritization of graptolites most likely reflects deposition under dysoxic conditions in which the graptolites became a locally reducing micro-environment, whilst the abundant occurrence of flattened graptolites probably represents preservation in a fully anoxic environment (Underwood, Reference Underwood1992; Underwood & Bottrell, Reference Underwood and Bottrell1994; A. A. Page, unpub. Ph.D. thesis, Univ. Leicester, 2007; Zalasiewicz et al. Reference Zalasiewicz, Williams, Miller, Page and Blackett2007; Loydell et al. Reference Loydell, Butcher, Frýda, Lüning and Fowler2009). Though causes of anoxia are various, transgressive black shales are common in shelf successions of this age, arguably deposited in response to deglaciation (Armstrong et al. Reference Armstrong, Turner, Makhlouf, Weedon, Williams, Al Smadi and Abu Salah2005; Page et al. Reference Page, Zalasiewicz, Williams, Popov, Williams, Haywood, Gregory and Schmidt2007).
5. Systematic palaeontology
Family normalograptidae Štorch & Serpagli, Reference Štorch and Serpagli1993 emend. Melchin et al. (Reference Melchin, Mitchell, Naczk-Cameron, Fan and Loxton2011).
Genus Normalograptus Legrand (Reference Legrand1987) emend. Melchin & Mitchell (Reference Melchin, Mitchell, Barnes and Williams1991) emend. Melchin et al. (Reference Melchin, Mitchell, Naczk-Cameron, Fan and Loxton2011).
Type species. [OD] Climacograptus scalaris var. normalis Lapworth (Reference Lapworth1877).
Diagnosis. Biserial graptolites of astogenic Pattern H with unornamented climacograptid thecae having straight or concave interthecal septa throughout the rhabdosome and a full median septum, which may be delayed.
Normalograptus kufraensis sp. nov.
Holotype. Specimen CAM SM X.50191.3c (Fig. 5b) from the Tanezzuft Formation, Jabal Eghei, Libya (N 22.662307, E 19.962156).
Material. 30+ graptolites on samples CAM SM X.50191.1 to X.50191.15, of which 23 were sufficiently well-preserved for detailed morphological study, being CAM SM X.50191.1a–c, X.50191.3a–f; X.50191.4a, X.50191.6a–b, X.50191.7a–b, X.50191.8a, X.50191.9a; X.50191.10a, X.50191.12a, X.50191.13a, X.50191.14a–b and X.50191.15a–b. These specimens are stored in the Sedgwick Museum, University of Cambridge, UK.
Locality and horizons. All specimens come from a single marker bed at the western margin of the Kufra Basin, southern Libya (Figs 1, 2).
Preservation. As above.
Etymology. After the Kufra Basin where the graptolites were found.
Diagnosis. Normalograptid with a long virgella, long, straight nema, climacograptid thecae with long, parallel supragenicular walls and a full, straight median septum; 2TRDs and DVWs range, respectively, between 1.55–1.90 mm and 0.70–1.20 mm at theca 21, between 1.60–2.10 mm and 0.85–1.45 mm at theca 51, and 1.90–2.35 mm and 1.05–1.45 mm distally. Maximum width achieved at or around the sixth thecal pair.
Description. Mature rhabdosomes are up to 24 mm long consisting of 25 thecal pairs. The rhabdosome widens rapidly achieving constant maximum DVWs and 2TRDs by thecae 4–6. The nema and virgella protrude up to at least 5 and 7 mm from either end of the rhabdosome, respectively. The undivided virgella runs straight down following the line of the median septum in all but one example. The sicula ranges between 1.60 and 2.40 mm in length, and 0.30 and 0.45 mm in width; its apex typically lies between thecae 21–22, though rarely it may reach slightly more distally than the level of theca 22. Theca 11 grows down 0.20–0.30 mm below the sicula aperture and is 0.90–1.20 mm long, whilst the length of the exposed margin of the sicula below theca 12 is 0.25–0.45 mm. Thecae are of typical climacograptid form with sharp genicula and long, straight supragenicular walls that run parallel to the median septum. The thecal apertures are horizontal and a complete median septum is apparent in most specimens. A summary of 2TRDs and DVWs for these specimens is given in Table 1 and Figure 6.

Figure 6. Scatter plot of dorso-ventral widths (DVW) and two theca repeat distances (2TRD) measured on Normalograptus kufraensis from the Lower Palaeozoic succession of Jabal Eghei, western Kufra Basin.
Discussion. With no bimodality observed in its populations and little variation between slabs, N. kufraensis clearly represents a single taxon, dissimilar in measurements to comparable graptolites (Tables 2, 3). Though N. kufraensis exhibits a considerable range of DVW and 2TRD variation, this may in part reflect the style of preservation, with some specimens flattened and others in relief. But such a range of variation is typical in normalograptid specimens from high palaeolatitudes (Loydell, Reference Loydell2007) and comparable to the range of variation of three-dimensionally preserved material from lower palaeolatitudes (e.g. Blackett et al. Reference Blackett, Page, Zalasiewicz, Williams, Rickards and Davies2009). The smaller range of variation seen in other taxa included in Table 2 may reflect the fact that many of these data are taken from measurements of figured specimens rather than large populations. Likewise, the range in virgella length apparent in N. kufraensis is typical of that in other normalograptids (see Chen et al. Reference Chen, Fan, Melchin and Mitchell2005; Loydell, Reference Loydell2007). This may reflect an ecophenotypic response to the local hydrodynamic regime as Rickards et al. (Reference Rickards, Rigby, Rickards and Swales1998) showed that the virgella served to align the graptolite to the ambient current. Though some specimens appear slightly fusiform (e.g. Fig. 5a, h), this is not apparent in most, especially not in any in biprofile preservation, suggesting it may be an artefact of flattening. The preponderance of subscalariform preservation may indicate that N. kufraensis had similar dorsoventral and lateral widths.
N. kufraensis belongs to a small distinctive clade of slender, elongate normalograptids with climacograptid thecae and long nemata and virgellae that superficially resemble the unadorned climacograptid Styracograptus Štorch et al. (Reference Štorch, Mitchell, Finney and Melchin2011). N. kufraensis may be confidently assigned to Normalograptus based on its narrow triangulate proximal end, however. Other more subtle distinctions between it and Styracograptus include simple distal thecal ontogeny from theca 21, a typical unflexed graptoloid sicula and the absence of cross bars on Normalograptus nemata (Goldman et al. Reference Goldman, Mitchell, Melchin, Fan and Wu2011). The distinction between the genera has simplified the synonymy of the Styracograptus-like normalograptids, with many Katian forms reassigned to Styracograptus. The specimens from Jabal Eghei cannot be accommodated in any established species: Table 2 compares its width and thecal packing with the Styracograptus-like normalograptids N. ajjeri, N. angustus and N. arrikini, and Table 3 compares it with other normalograptids. The elongate graptolite N. rectangularis is larger in all dimensions, and Normalograptus normalis like many other normalograptids is significantly more robust. Others differ markedly.
Though there is some overlap between the measurements of N. kufraensis and specimens of N. ajjeri, N. angustus and N. arrikini, the range of variation in N. kufraensis is centred almost exactly on the ‘gap’ in morphospace between the other taxa. N. angustus (Perner, Reference Perner1895) is too gracile, whilst the sister species N. ajjeri (Legrand, Reference Legrand1977) and N. arrikini Legrand (Reference Legrand2009) have their thecae too closely spaced (Table 2), as is true of many other slender normalograptids (cf. Loydell, Reference Loydell2007; Blackett et al. Reference Blackett, Page, Zalasiewicz, Williams, Rickards and Davies2009). Working on material from the late Ordovician of Nevada, Štorch et al. (Reference Štorch, Mitchell, Finney and Melchin2011) observed that N. angustus is more strongly geniculate than N. ajjeri, and that it may have an undulating median septum. N. kufraensis is intermediate in geniculation and has a fully straight median septum. Though there is some overlap between their the 2TRDs of specimens of N. ajjeri from Nevada and N. kufraensis, the Libyan graptolites have their thecae significantly more widely spaced on average (Tables 1, 2). By having climacograptid thecae, N. kufraensis appears more similar to N. ajjeri than N. arrikini. But as distortion in flattening can result in climacograptid thecae taking on a glyptograptid appearance (Briggs & Williams, Reference Briggs and Williams1981; Loydell, Reference Loydell2007), this distinction could be artefactual: the Algerian taxa are very similar indeed. Whilst Legrand (unpub. Ph.D. thesis, Univ. Michel de Montaigne-Bordeaux III, 1999) reported many ‘aff.’ forms of either taxon (Table 2), none of these agree with the dimensions of N. kufraensis. Although the Libyan graptolites appear to conform to N. sp. 1 of Štorch & Feist (Reference Štorch and Feist2008) in terms of their measurements (Table 2), the latter, which is only known from a single specimen, has a highly asymmetrical proximal end precluding synonymy. Thus N. kufraensis clearly represents a new species.
N. kufraensis may have plausibly evolved from N. angustus in the latest Ordovician. The N. angustus lineage undergoes a considerable reduction in rhabdosome width in the Silurian (cf. Rickards, Reference Rickards1970; Hutt, Reference Hutt1974; Štorch, Reference Štorch1989; Štorch & Speragli, Reference Štorch and Serpagli1993; Chen et al. Reference Chen, Fan, Melchin and Mitchell2005; Loydell, Reference Loydell2007; Table 2), with the dimensions of some broader Ordovician forms of N. angustus overlapping with N. kufraensis a little (Table 2). Until this lineage has been explored in more detail, it is impossible to conclude as to whether certain specimens assigned to N. angustus may be better ascribed to N. kufraensis. N. angustus is both temporally and geographically wide ranging. N. ajjeri is also relatively long-lived, with Loydell (Reference Loydell2007) sensibly synonymizing almost all previous reports of N. normalis with it. He did not, however, discuss Katian reports of N. normalis (e.g. Williams, Reference Williams1982 a; Chen et al. Reference Chen, Rong, Mitchell, Harper, Fan, Zhan, Zhang, Li and Wang2000; Rickards, Reference Rickards2002), which might push its first appearance somewhat earlier. It seems at present, however, that N. kufraensis is best viewed as an intermediate between the broader Ordovician specimens of the N. angustus lineage and the sister species N. ajjeri and N. arrikini, which have a Hirnantian or younger age. This is certainly consistent with the pattern of Normalograptus biogeography and evolution: aside from N. angustus, normalograptids with climacograptid thecae are unknown between the mid Katian and Hirnantian, when they diversified considerably (Goldman et al. Reference Goldman, Mitchell, Melchin, Fan and Wu2011).
6. Biostratigraphic age constraints and deglacial anoxia
Graptolites from Jabal Eghei can be confidently assigned to the genus Normalograptus, which provides lower and upper bounds for the age of the graptolite-bearing marker bed and hence for the Tanezzuft Formation in Jabal Eghei. In the UK, Normalograptus is known from the murchisoni Zone of the Darriwilian (late Middle Ordovician) through to at least the magnus Zone of the Aeronian (mid Llandovery, Silurian) (Zalasiewicz et al. Reference Zalasiewicz, Taylor, Rushton, Loydell, Rickards and Williams2009). However, graptolites referred to the genus Glyptograptus extend to the griestoniensis Zone of the Telychian (late Llandovery, Silurian) (Zalasiewicz et al. Reference Zalasiewicz, Taylor, Rushton, Loydell, Rickards and Williams2009) and Melchin (Reference Melchin1998) showed that Llandovery ‘glyptograptids’ with a full distal median septum should be assigned to Normalograptus. Some of the earlier occurrences of Normalograptus may in fact be Styracograptus (Goldman et al. Reference Goldman, Mitchell, Melchin, Fan and Wu2011). At first sight though, N. kufraensis may potentially be of anything from Darriwilian to Llandovery in age.
The oldest age for N. kufraensis may be in principle inferred from stratophenetic considerations. As noted above, N. kufraensis is best viewed as an intermediate between the Ordovician branch of the N. angustus lineage and Legrand's sister species N. ajjeri and N. arrikini. Both N. angustus and N. ajjeri are long-ranging taxa, whereas N. arrikini is restricted to the latest Ordovician (Fig. 7). N. angustus is known from the earlier part of the linearis Zone (Katian, late Ordovician) to the cyphus Zone (Rhuddanian, early Silurian) (see Rickards, Reference Rickards1970; Hutt, Reference Hutt1974; Zalasiewicz et al. Reference Zalasiewicz, Taylor, Rushton, Loydell, Rickards and Williams2009; Goldman et al. Reference Goldman, Mitchell, Melchin, Fan and Wu2011, who reassigned reports of clingani Zone occurrences such as in Williams, Reference Williams1982 b to Styracograptus miserabilis). Loydell (Reference Loydell2007) states that N. ajjeri ranges from the Hirnantian to early Aeronian. If N. kufraensis was an evolutionary intermediate between broader Ordovician forms of N. angustus and N. ajjeri itself, then N. kufraensis must have originated in the Hirnantian suggesting the outcrop in Jabal Eghei could be of Hirnantian (persculptus Zone) or younger age. However, further data either on the stratigraphy of the Tanezzuft Formation or on the evolution of the N. angustus and N. ajjeri–N. arrikini lineages are needed for this to be confirmed.

Figure 7. Stratigraphic range of the graptolites Normalograptus angustus, N. ajerri and N. arrikini with proposed relationship to N. kufraensis illustrated. The biozonation and correlation between regions follows Loydell (Reference Loydell2012a ). See text for other references.
The uppermost shales of the Tanezzuft Formation have a latest Rhuddanian or earliest Aeronian age (cyphus or triangulatus biozones) (Lüning et al. Reference Lüning, Craig, Fitches, Mayouf, Busrewil, El Dieb, Gammudi, Loydell and McIlroy1999). Grignani, Lanzoni & Elatrash (Reference Grignani, Lanzoni, Elatrash, Salem, Hammuda and Eliagoubi1991) mentioned Climacograptus medius Törnquist from core samples of well KW-2, drilled by AGIP in Jabal Asba in 1975. The occurrence of this graptolite suggests a late Ordovician (Hirnantian) or early Llandovery age (Rhuddanian to early Aeronian) for the studied core interval (Lüning et al. Reference Lüning, Craig, Fitches, Mayouf, Busrewil, El Dieb, Gammudi, Loydell and McIlroy1999). These data indicate that the graptolite marker bed must have been deposited before the early Aeronian. The graptolite-bearing bed occurs above a glaciogenic unconformity thought to be of Hirnantian age (Le Heron & Howard, Reference Le Heron and Howard2010). Given the stratophenetic argument that N. kufraensis emerged from the N. angustus lineage in the latest Ordovician, the lowermost shales of the Tanezzuft Formation were deposited at sometime in or after the Hirnantian and before the earliest Aeronian.
The sedimentary succession at Jabal Eghei records deglaciation and a short-lived episode of transgressive anoxia (Le Heron et al. In press). This is seen elsewhere in northern Gondwana and at lower latitudes in which the onset of anoxia occurs in the persculptus Biozone (Armstrong et al. Reference Armstrong, Turner, Makhlouf, Weedon, Williams, Al Smadi and Abu Salah2005; Page et al. Reference Page, Zalasiewicz, Williams, Popov, Williams, Haywood, Gregory and Schmidt2007). However, the temporal extent of the unconformity in this section has yet to be determined and the Rhuddanian also contains notable intervals of anoxia. Though the biostratigraphic resolution is currently too coarse to definitively place these shales in the Hirnantian, and the conventional view is that marine flooding of the Kufra Basin occurred in the early Silurian (Lüning et al. Reference Lüning, Craig, Fitches, Mayouf, Busrewil, El Dieb, Gammudi, Loydell and McIlroy1999), there is increasing evidence pointing to a prolonged deglacial oceanic anoxic episode originating in the latest Ordovician (Page et al. Reference Page, Zalasiewicz, Williams, Popov, Williams, Haywood, Gregory and Schmidt2007). In Jabal Eghei, however, the anoxia was short-lived.
7. Conclusions
An assemblage of Normalograptus kufraensis nov. sp. – an intermediate between N. angustus (Perner) and N. ajjeri (Legrand) – has been recovered from exposures in the western Kufra Basin of southern Libya. Although it is morphologically similar to the long-ranging graptolites N. angustus and N. ajjeri, stratophenetic considerations suggest N. kufraensis from Jabal Eghei may have been of Hirnantian age or younger. If so, this would show that the basal part of the Tanezzuft Formation is exposed at the western margin of the Kufra Basin. Thus, following late Ordovician glaciation, major marine flooding of the Kufra Basin may have begun in the Hirnantian or earliest Rhuddanian (see also Grignani, Lanzoni & Elatrash, Reference Grignani, Lanzoni, Elatrash, Salem, Hammuda and Eliagoubi1991; CASP, unpub. data), plausibly earlier than thought. Establishing the relationship between anoxia, deglaciation and organic carbon burial at this time may further constrain our understanding of the Early Palaeozoic Icehouse climate (Page et al. Reference Page, Zalasiewicz, Williams, Popov, Williams, Haywood, Gregory and Schmidt2007).
Acknowledgements
We gratefully thank Yousef Abutarruma, Ramadan Aburawi, Ahmed I. Asbali, Ibrahim Baggar, Faraj Said and Bindra Thusu for their scientific support and guidance for the project work in Libya. We also would like to thank the logistics team, provided by Bashir Grenat, for assistance in the field. The administrative and logistical support of staff at LPI is gratefully acknowledged. David Loydell, Barrie Rickards and Petr Štorch are thanked for their comments and provision of literature. Mike Melchin and Jan Zalasiewicz's advice and constructive reviews further improved the manuscript. The consortium of subscribing oil and gas companies is thanked for its financial support to the CASP Southern Basins of Libya Project. This is Cambridge Earth Sciences contribution number ES.2572.