1. Introduction
Horseshoe crabs (Chelicerata, Xiphosurida) are extant marine chelicerates that have a fossil record extending back to the Ordovician Period (Rudkin et al. Reference Rudkin, Young and Nowlan2008; Van Roy et al. Reference Van Roy, Orr, Botting, Muir, Vinther, Lefebvre, El Hariri and Briggs2010, Reference Van Roy, Briggs and Gaines2015). This fossil record, coupled with apparent evidence for evolutionary stasis and the co-called ‘living fossil’ condition, has resulted in examination of the group somewhat sporadically over the last two centuries (Bicknell & Pates, Reference Bicknell and Pates2020). However, over the past five years, a modern renaissance in xiphosurid research has occurred. This explosion of research is concurrent with the development of a new phylogenetic framework for Xiphosurida and the synthesis of taxonomy with geometric morphometric methods (Lamsdell & McKenzie, Reference Lamsdell and McKenzie2015; Lamsdell, Reference Lamsdell2016, Reference Lamsdell2020; Lerner et al. Reference Lerner, Lucas and Mansky2016, Reference Lerner, Lucas and Lockley2017; Błażejowski et al. Reference Błażejowski, Niedźwiedzki, Boukhalfa and Soussi2017; Naugolnykh, Reference Naugolnykh2017, Reference Naugolnykh2020; Zuber et al. Reference Zuber, Laaß, Hamann, Kretschmer, Hauschke, Van De Kamp, Baumbach and Koenig2017; Bicknell et al. Reference Bicknell, Klinkhamer, Flavel, Wroe and Paterson2018, Reference Bicknell, Amati and Ortega-Hernández2019 a, b, c, d, e, Reference Bicknell, Naugolnykh and Brougham2020, Reference Bicknell, Błażejowski, Wings, Hitij and Bottonin press ; Haug & Rötzer, Reference Haug and Rötzer2018; Shpinev, Reference Shpinev2018; Shpinev & Vasilenko, Reference Shpinev and Vasilenko2018; Bicknell, Reference Bicknell2019; Bicknell & Pates, Reference Bicknell and Pates2019, Reference Bicknell and Pates2020; Tashman et al. Reference Tashman, Feldmann and Schweitzer2019; Haug & Haug, Reference Haug and Haug2020; Lamsdell et al. Reference Lamsdell, Tashman, Pasini and Garassino2020 represent the majority of key publications). The taxonomic component of this renaissance has seen re-examination of both historically important and new specimens; a crucial direction for organizing oversplit groups (such as Euproops Meek, Reference Meek1867 and Paleolimulus Dunbar, Reference Dunbar1923) while simultaneously increasing the diversity of previously under-represented groups, such as Austrolimulidae. As a result, the austrolimulid diversity has increased from one genus to at least six genera (see Bicknell et al. Reference Bicknell, Naugolnykh and Brougham2020; Lamsdell, Reference Lamsdell2020). Continued examination of this family has demonstrated the origin of these extreme xiphosurid forms in the Permian Period (Bicknell, Reference Bicknell2019; Bicknell et al. Reference Bicknell, Naugolnykh and Brougham2020), followed by a Triassic diversification event and apparent extinction by the end of the Triassic Period (Lamsdell, Reference Lamsdell2020). New xiphosurid fossils need to be identified to thoroughly understand the timing of this extinction event. To align with this direction, here we assess a new horseshoe crab specimen from the lowermost Jurassic deposits of Upper Franconia, Bavaria, that represents the youngest austrolimulid, the third genus of the family known from German deposits, and increases the already diverse German xiphosurid fossil record (Table 1, Fig. 1).
Table 1. Records of named Palaeozoic and Mesozoic xiphosurids from German deposits. Order by Family, then time periods
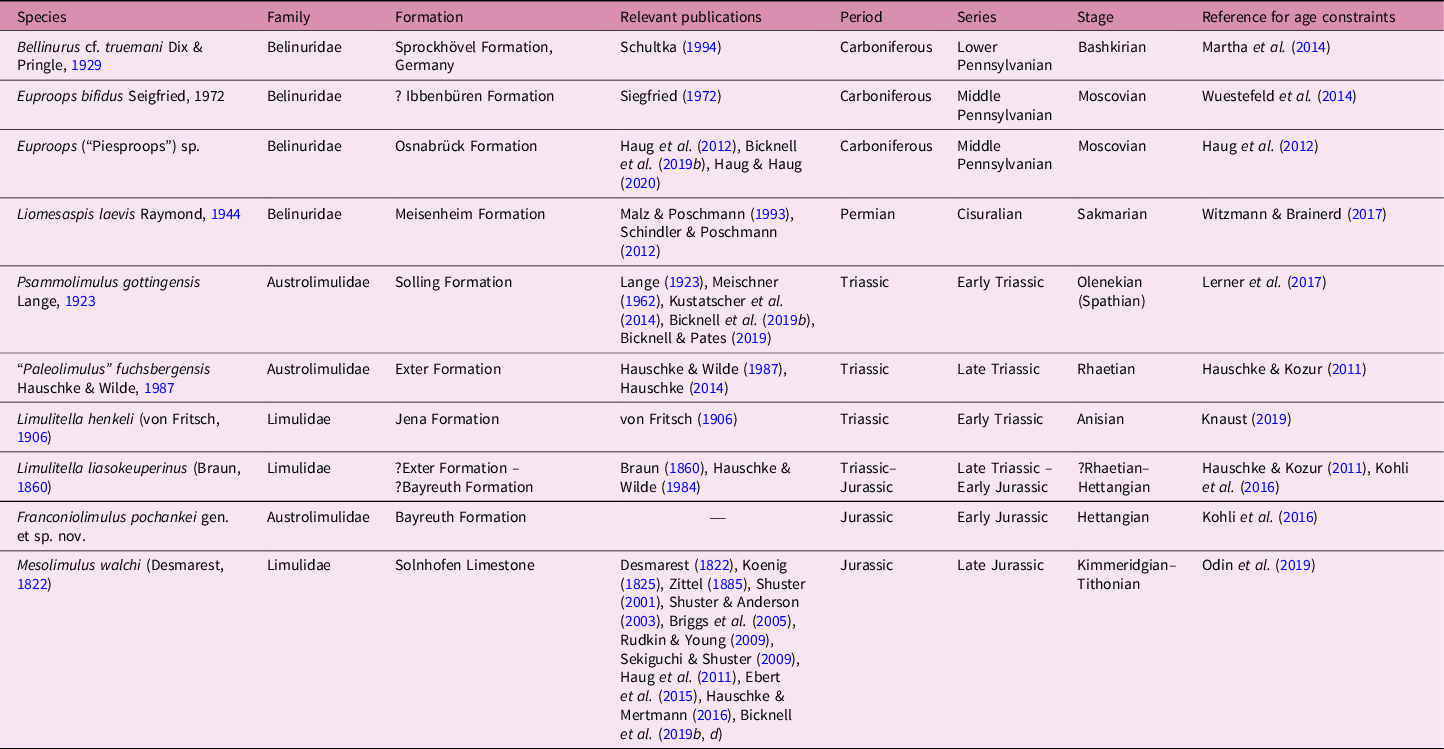

Fig. 1. Temporal ranges of Palaeozoic and Mesozoic xiphosurids from German deposits. See Table 1 for further information.
2. Institutional acronyms
AM F: Australian Museum, Sydney, New South Wales, Australia; GZG INV: Geowissenschaftliches Zentrum der Georg-August-Universität Geowissenschaftliches Museum, Göttingen, Germany; MMF: Geological Survey of New South Wales, Londonderry, New South Wales, Australia; SSN: Paläontologisches Museum Nierstein, Nierstein, Germany; UCM: University of Colorado Museum of Natural History, Boulder, Colorado, USA; USNM: United States National Museum, Washington, DC, USA; UTGD: Geology Department, University of Tasmania, Tasmania, Australia.
3. Geological context
The specimen considered here was collected in 1999 by Harald Stapf from a clay lens at the Pechgraben locality; a sand pit of the Bocksrück Sandgrube GmbH & Co. KG, situated near Neudrossenfeld, Franconia, Bavaria, Southern Germany (TK 1:25 000, No. 5935 Marktschorgast, 50° 00' 08.1” N, 11° 32' 26.2” E; Fig. 2). Following Kohli et al. (Reference Kohli, Ware and Bechly2016), the Pechgraben material likely represents an outcrop of the Bayreuth Formation. The Bayreuth Formation is considered temporally contiguous with the Psilonotenton Formation (lower Hettangian) and the overlying Angulatensandstein Formation (upper Hettangian; Fig. 3); however, these formations cannot be confidently discerned at Pechgraben (Bloos et al. Reference Bloos, Dietl and Schweigert2006). The Bayreuth Formation is largely composed of sandstones with intercalated clay lenses, the latter locally rich in exceptionally preserved terrestrial plant fossils indicative of a fluviatile-limnic to brackish environment with limited coastal influence (Bloos et al. Reference Bloos, Dietl and Schweigert2006).

Fig. 2. Map of Germany showing close-up of specimen locality at Pechgraben (red star).
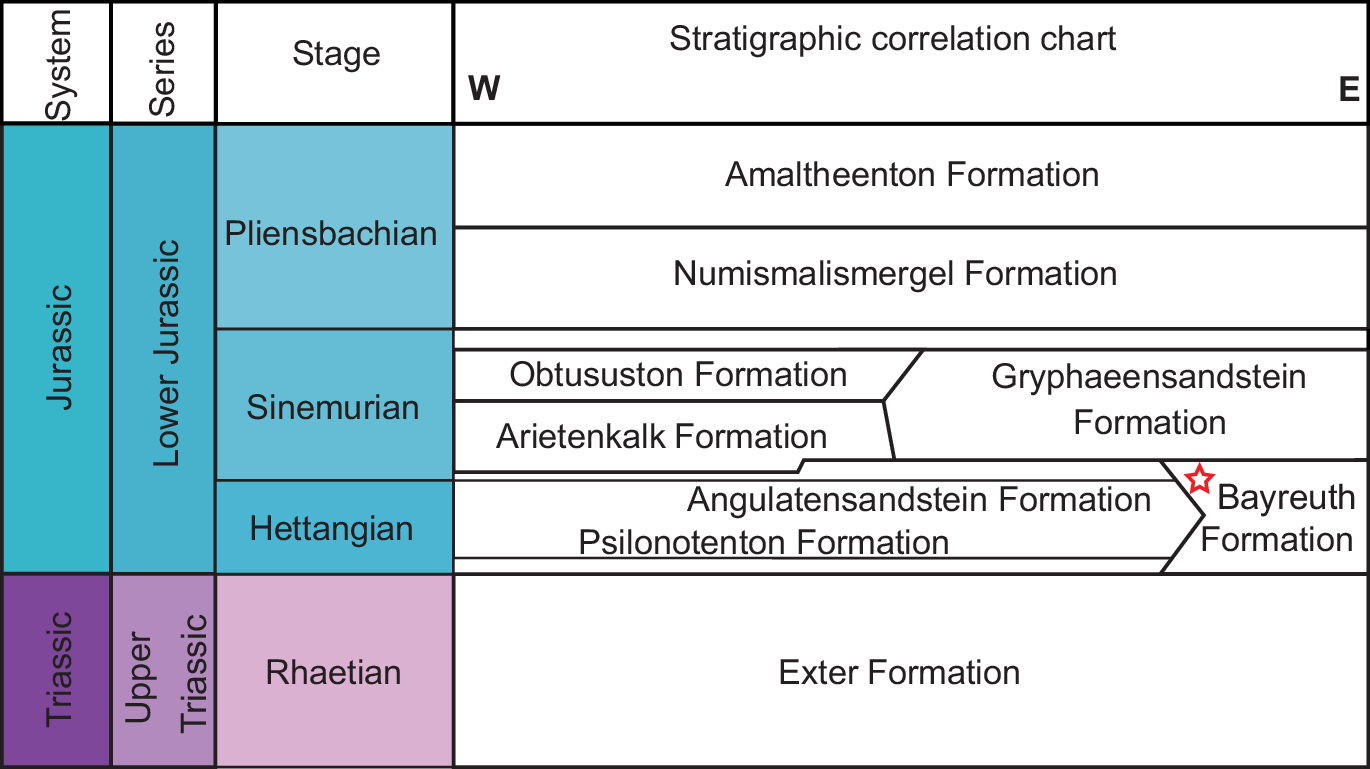
Fig. 3. Simplified correlation of Triassic–Jurassic sequences in the Bayreuth region. Stratigraphic position of units containing Franconiolimulus pochankei gen. et. sp. nov. highlighted (red star).
The Bayreuth Formation at Pechgraben shows an extraordinary level of preservation (suggesting it may represent a Konservat Lagerstätte) and, as such, has been examined variably over the last 160 years (Braun, Reference Braun1860; Weber, Reference Weber1968; van Konijnenburg-van Cittert & Schmeißner, Reference van Konijnenburg-van Cittert and Schmeißner1999; Bauer et al. Reference Bauer, Kustatscher, Dütsch, Schmeißner, Krings and van Konijnenburg-van Cittert2015). In particular, a variety of distinct plant groups have been described from the locality (van Konijnenburg-van Cittert et al. Reference van Konijnenburg-van Cittert, Schmeißner and Dütsch2001; van Konijnenburg-van Cittert, Reference van Konijnenburg-van Cittert2010; Bauer et al. Reference Bauer, Kustatscher, Dütsch, Schmeißner, Krings and van Konijnenburg-van Cittert2015; Kustatscher et al. Reference Kustatscher, van Konijnenburg-van Cittert, Bauer and Krings2016). Curiously, animal remains are rarely identified at Pechgraben (Braun, Reference Braun1860; Weber, Reference Weber1968; Emmert, Reference Emmert1977; Bloos et al. Reference Bloos, Dietl and Schweigert2006; Kohli et al. Reference Kohli, Ware and Bechly2016). Aside from horseshoe crabs, animal fossils include eggs from freshwater hybodont sharks Palaeoxyris alterna Fischer et al. Reference Fischer, Voigt, Schneider, Buchwitz and Voigt2011 and Palaeoxyris muensteri (Presl, Reference Presl and Strenberg1838) (Fischer et al. Reference Fischer, Voigt, Schneider, Buchwitz and Voigt2011), a freshwater mussel – Anoplophora (Syn. Anodonta) liasokeuperina (Braun, Reference Braun1860) – and rare dragonfly fossils (Bechly, Reference Bechly2015; Kohli et al. Reference Kohli, Ware and Bechly2016). Taken together, the Pechgraben site likely preserves a low-energy freshwater environment (possibly an oxbow lake) that was surrounded by dense vegetation (Weber, Reference Weber1968; Schmeissner & Hauptmann, Reference Schmeissner and Hauptmann1998; van Konijnenburg-van Cittert et al. Reference van Konijnenburg-van Cittert, Schmeißner and Dütsch2001).
4. Methods
The studied specimen is housed in the Paläontologisches Museum Nierstein, Nierstein, Rhineland-Palatinate, Germany and was assigned specimen number SSN 8PG35. The specimen was photographed using a Canon EOS 800D with a Canon EFS 35 mm macro lens under normal and low-angle halogen light to highlight all features. The camera was controlled by Capture One Pro 12.1. Measurements were digitally obtained from photographs using ImageJ. For comparison, photographs of other austrolimulids were requested from collections or gathered by the authors. We follow the systematic taxonomy of Bicknell & Pates (Reference Bicknell and Pates2020) and the anatomical terms presented in Bicknell (Reference Bicknell2019) and Bicknell et al. (Reference Bicknell, Lustri and Brougham2019 c, Reference Bicknell, Naugolnykh and Brougham2020).
5. Systematic palaeontology
Subphylum Chelicerata Heymons, Reference Heymons1901
Class Xiphosura Latreille, Reference Latreille1802
Order Xiphosurida Latreille, Reference Latreille1802
Suborder Limulina Richter & Richter, Reference Richter and Richter1929
Superfamily Limuloidea Zittel, Reference Zittel1885
Family Austrolimulidae Riek, Reference Riek1955
Genus Franconiolimulus gen. nov.
Etymology. Generic name combines the Upper Franconian origin of the specimen with the generic name of the iconic American horseshoe crab; Limulus.
Type and only species. Franconiolimulus pochankei gen. et sp. nov. type and only species.
Diagnosis. An austrolimulid with kinked genal spines that terminate over half way along the thoracetron, an anteriorly effaced, keeled cardiac lobe, and a medial thoracetronic lobe without pronounced keeling.
Franconiolimulus pochankei sp. nov.
Figures 4–6
Diagnosis. Same as for genus.
Etymology. The species name pochankei honours Hartmut Pochanke, whose knowledge of the Pechgraben geology and aid in specimen collection resulted in the identification of the studied specimen.
Holotype specimen. SSN 8PG35
Location. 95521 Neudrossenfeld, district Pechgraben, owner Bocksrück Sandgrube GmbH & Co. KG (50° 00' 08.1” N, 11° 32' 26.2” E)
Formation, type locality, age. Bayreuth Formation, Pechgraben, Early Jurassic, Hettangian.
Distribution. Limited to the Pechgraben fossil-bearing clay lenses.
Preservation. SSN 8PG35 is preserved as a slightly vaulted, cream- to orange-coloured prosoma and thoracetron on a slab of light-brown-coloured clay.
Description. SSN 8PG35 is a slightly deformed articulated prosoma and thoracetron (Fig. 4). The specimen is 48.8 mm long. Prosoma is completely preserved, has a parabolic outline, is 30.1 mm long at midline and is 47.0 mm wide at the widest section. A thin prosomal rim is preserved, with a maximum width of 1.3 mm. Slight deformation of the exoskeleton is noted about the anterior right margin of the prosoma (Fig. 5). Both ophthalmic ridges are preserved. Left ophthalmic ridge is 19.4 mm long and lacks concavity. Right ophthalmic ridge is 21.2 mm long and is slightly concave. Ophthalmic ridges do not converge anteriorly. Lateral compound eyes are preserved on the ophthalmic ridges, c. 7.5 mm anteriorly from prosoma–thoracetron border (Fig. 4d). A cardiac lobe is present. The cardiac lobe is 8.0 mm wide posteriorly, tapering anteriorly into an apparently triangular shape. A pronounced cardiac ridge is noted, 20.1 mm long (Fig. 5). The ridge becomes less pronounced anteriorly. Ocelli are not observed. Both genal spines are preserved and splay laterally from the thoracetron. Genal spines extend posteriorly to terminate over half way along thoracetron. Kinks are observed approximately half way along the genal spine length. Left genal tip is 24.2 mm from the organismal midline. Angle between the left genal spine and left side of the thoracetron is 65.2°. Right genal spine is 19.0 mm from the organismal midline. Angle between the right genal spine and right side of the thoracetron is 66.9°. An occipital band is preserved along the left genal spine. Band starts at the lateral-most section of prosoma–thoracetron joint and extends along the genal spine, becoming effaced towards spine terminus (Fig. 4c). Prosomal–thoracetronic hinge is pronounced, 21.3 mm wide and 1.8 mm long. No prosomal appendages are preserved.

Fig. 4. Franconiolimulus pochankei gen. et. sp. nov., holotype (SSN 8PG35). (a) Complete specimen. Boxes indicate close-up of the specimen in (b–d). (b) Close-up of cardiac lobe and associated ridge. (c) Close-up of left side of posterior prosoma and thoracetron. Occipital band (white arrow) is effaced distally. Thoracetronic border shows a small free lobe (black arrow) and notable tapering of the thoracetron. (d) Close-up of right genal spine. Ophthalmic ridge with lateral compound eye is prominent (white arrow). Images are converted to greyscale. Image credit: Andreas Hecker.

Fig. 5. Interpretative drawing of Franconiolimulus pochankei gen. et. sp. nov., holotype (SSN 8PG35) showing key morphological features. Abbreviations: cl – cardiac lobe; cr – cardiac ridge; eye – lateral compound eye; fl – free lobe; gsk – genal spine kink; ob – occipital band; oph – ophthalmic ridge; rim – prosomal rim; tml – thoracetronic medial lobe. Dotted lines indicate deformation of the exoskeleton.
The thoracetron is partly preserved: the left side is completely preserved and the proximal section of the right side is preserved (Figs 4, 5). As such, width measurements will not represent life-size. Shape of the left side suggests the thoracetron would be strongly trapezoidal (see Fig. 6). It is 17.8 mm long, 23.2 mm wide anteriorly, increasing to a width of 25.6 mm at 5.8 mm posteriorly from the prosoma–thoracetron joint, tapering to 10.7 mm wide posteriorly. At the maximum width, the thoracetron is c. 50% narrower than the prosoma. A medial lobe is visible, slightly domed, but poorly defined. The lobe is slightly triangular, tapering from 8.3 mm to becoming effaced posteriorly. No segmentary furrows are present. The left pleural lobe is not segmented, 17.8 mm long, 12.4 mm wide, tapering to the posterior specimen edge (Fig. 4c). No marginal rim is preserved on the left side. A reduced free lobe is present on anterior section of pleural lobe (Fig. 4c). Left pleural lobe truncates markedly towards the posterior-most region. The right pleural lobe is poorly preserved, and appears to have been injured as the edge seems slightly cicatrized. The thoracetron–telson articulation and telson are not preserved.
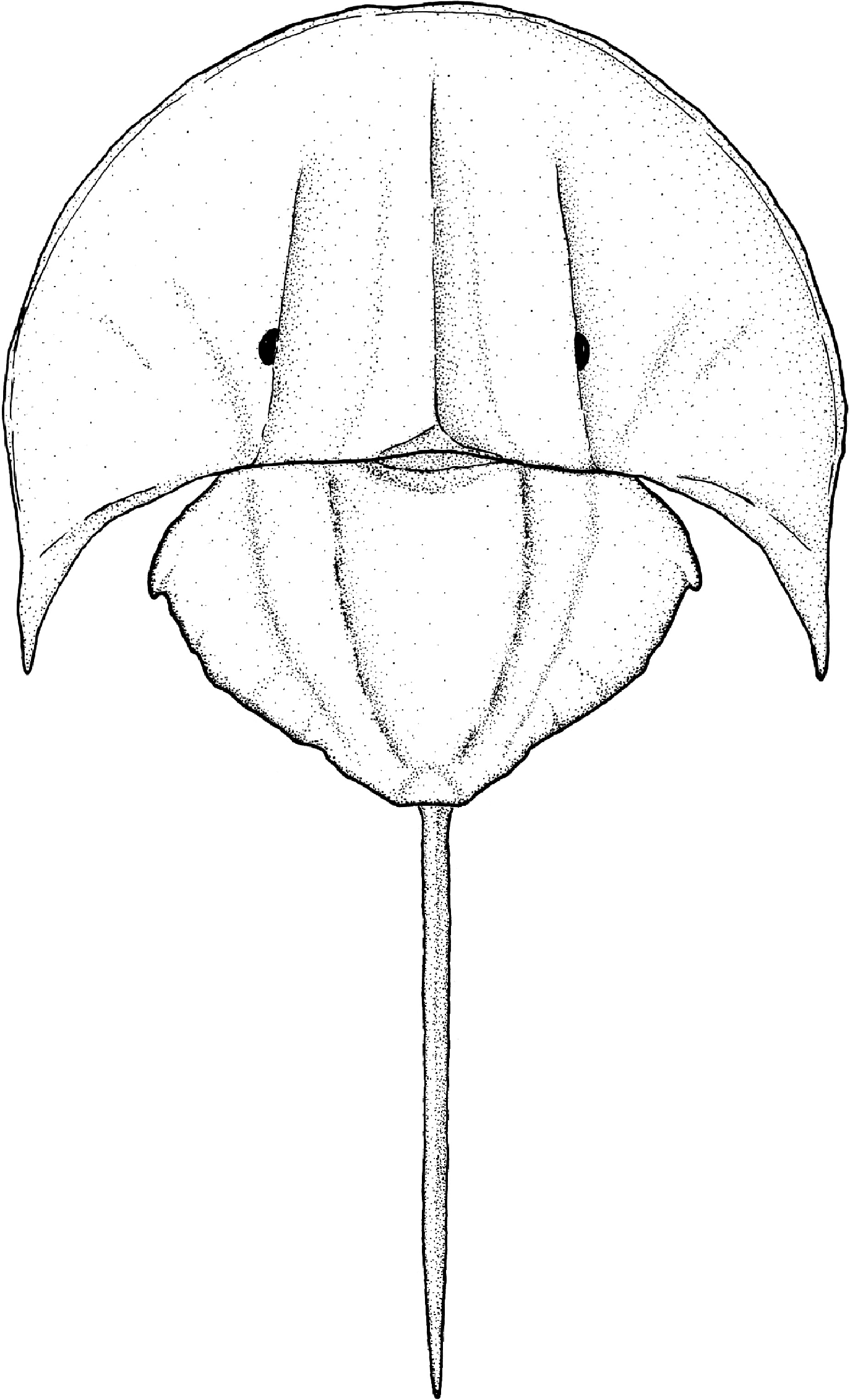
Fig. 6. Idealized reconstruction of Franconiolimulus pochankei gen. et. sp. nov. Reconstruction credit: Andreas Hecker.
Remarks. Austrolimulids represent the extreme morphological limitations of the xiphosurid Bauplan (Fig. 7). This is manifested in hypertrophied genal spines (hypertrophy here refers to overdeveloped spines, often with a marked splay when compared with limulid genal spines; see Austrolimulus fletcheri Riek, Reference Riek1955, Psammolimulus gottingensis Lange, Reference Lange1923, Tasmaniolimulus patersoni Bicknell, Reference Bicknell2019 and Vaderlimulus tricki Lerner et al. Reference Lerner, Lucas and Lockley2017) and decreased size of major exoskeletal sections (Dubbolimulus peetae Pickett, Reference Pickett1984). The hypertrophied genal spines of the Pechgraben horseshoe crab indicates an austrolimulid alignment (Fig. 6). The lack of an extreme lateral genal spine splay excludes SSN 8PG35 from A. fletcheri and V. tricki (Fig. 7a, h) and the lack of a inflated free lobe excludes SSN 8PG35 from Ps. gottingensis (Fig. 7c, d). The kinks along the genal spines are comparable to T. patersoni and Panduralimulus babcocki Allen & Feldmann, Reference Allen and Feldmann2005 (Fig. 7b, e, f). However, SSN 8PG35 lacks the M-shaped ophthalmic ridge joint known from T. patersoni and the strongly keeled medial thoracetronic lobe of Pa. babcocki. Finally, as SSN 8PG35 lacks a highly reduced thoracetron, the material is excluded from D. peetae (Fig. 7g). As such, we assert that the placement of the Pechgraben horseshoe crab into a new genus and species is a valid taxonomic conclusion. Franconiolimulus pochankei gen. et sp. nov. therefore represents the youngest austrolimulid and illustrates that the family survived into the Jurassic Period.
Consideration must be given to Limulitella liasokeuperinus (Braun, Reference Braun1860) as this taxon is known from Upper Triassic (?Rhaetian) through to the lowermost Jurassic (?Hettangian) deposits of Franconia. There is poor stratigraphic control on the taxon; however, it seems that the material is from either the Exter Formation or Bayreuth Formation (Hauschke & Wilde, Reference Hauschke and Wilde1984). Assuming that L. liasokeuperinus is from the Bayreuth Formation, it is imperative that we demonstrate how SSN 8PG35 is distinct from L. liasokeuperinus. Considering the original work in Braun (Reference Braun1860, fig. 1; note that, according to Hauschke & Wilde, Reference Hauschke and Wilde1984, the holotype has since been lost), the line drawing shows the ophthalmic ridges forming an M-shaped joint anterior to a well-defined cardiac lobe. Both of these features are unknown to SSN 8PG35. The only other comparative material from Franconia ascribed to L. cf. liasokeuperinus was presented in Hauschke & Wilde (Reference Hauschke and Wilde1984, exemplar 1). This partial prosoma shows pronounced cardiac lobe furrows, ophthalmic ridges joining anteriorly and an elongate diamond-shaped feature along the cardiac lobe ridge. These features are not observed in SSN 8PG35. To this end, we assert that SSN 8PG35 is distinct from L. liasokeuperinus. Possible evidence for two xiphosurids in the Bayreuth Formation suggests that the depositional environment of the formation may have represented an ideal habitat for freshwater horseshoe crabs that also allowed for preservation of cuticular exoskeletons. Such taxonomic abundance is known to the Bear Gulch Limestone (Mississippian, Serpukhovian), Montana, USA; the Mazon Creek Lagerstätte within the Francis Creek Shale Member of the Carbondale Formation (Pennsylvanian, Moscovian), Illinois, USA; the Wellington Formation (Cisuralian, Wolfcampian), Oklahoma, USA; and the Alcover Limestone Formation (Middle Triassic, Ladinian), Spain (Bicknell & Pates, Reference Bicknell and Pates2020). Further examination of the Exter and Bayreuth formations is needed to determine the true xiphosurid diversity of these interesting deposits.
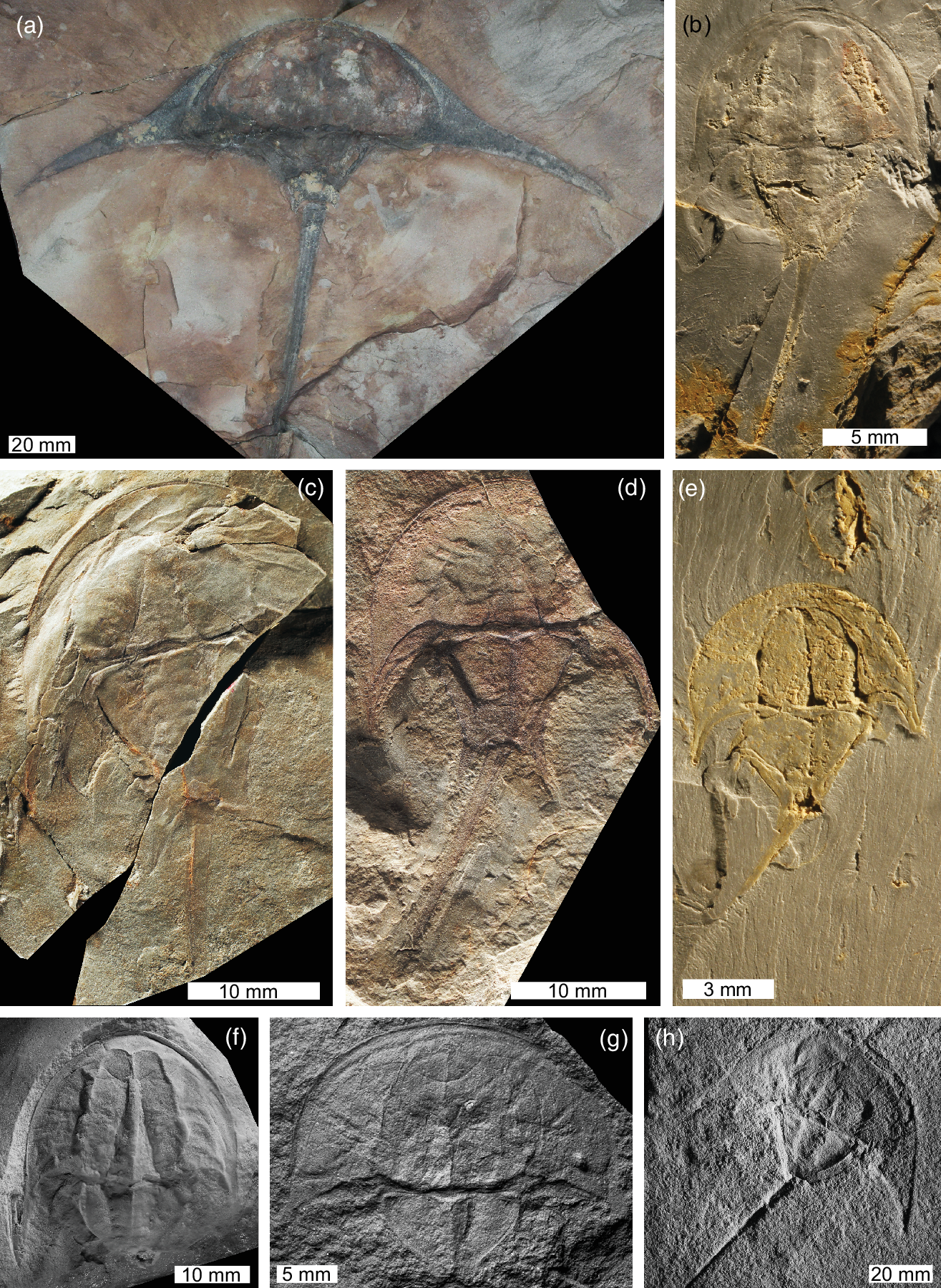
Fig. 7. Other representatives of Austrolimulidae. (a) Austrolimulus fletcheri from the Beacon Hill Formation (Middle Triassic, Ladinian), New South Wales, Australia. AM F38274, holotype. (b, e) Panduralimulus babcocki from the Maybelle Limestone, Lueders Formation (Permian, Cisuralian, Kungurian), Texas, USA: (b) USNM 520723, holotype and (e) USNM 520724, paratype. (c, d) Psammolimulus gottingensis from the Solling Formation (Lower Triassic, Olenekian, Spathian), Lower Saxony, Germany: (c) GZG INV 15376a and (d) GZG INV 45730a. (f) Tasmaniolimulus patersoni from the Jackey Shale (Permian, Lopingian), Tasmania, Australia. UTGD 123979, holotype. (g) Dubbolimulus peetae from the Ballimore Formation (Middle Triassic, Ladinian), New South Wales, Australia. MMF 27693, holotype. (h) Vaderlimulus tricki from the lower shale unit, Thaynes Group (Lower Triassic, Olenekian, Spathian), Idaho, USA. UCM 140.25, holotype. Image credit: (a) Josh White; (b, e, f) Russell Bicknell; (c, d) Gerhart Hundertmark; (g) David Barnes; and (h) Allan Lerner. (f) Coated in ammonium chloride sublimate.
6. Discussion
The identification of a Jurassic austrolimulid genus demonstrates that the family had survived the supposed end-Triassic austrolimulid extinction and are now known from the time period when horseshoe crabs are considered representative of evolutionary stasis (Kin & Błażejowski, Reference Kin and Błażejowski2014). Comparing Franconiolimulus pochankei gen. et sp. nov. with the Triassic austrolimulids, an extreme genal spine splay and a reduction of exoskeletal sections are not observed in F. pochankei. This observation prompts two questions: (1) Why were austrolimulid morphologies so diverse during the Triassic? (2) Why are austrolimulids unknown after F. pochankei? The extreme morphological diversity of Triassic austrolimulids is often ascribed to habitation of freshwater conditions (Lerner et al. Reference Lerner, Lucas and Lockley2017; Bicknell, Reference Bicknell2019; Bicknell & Pates, Reference Bicknell and Pates2019, Reference Bicknell and Pates2020; Bicknell et al. Reference Bicknell, Naugolnykh and Brougham2020). The prevalence of overdeveloped genal spines may have decreased the impact of uni-directional freshwater currents (Bicknell, Reference Bicknell2019), potentially allowing them to be anchored and avoid being transported downstream. This evolutionary transition therefore resulted in notable innovations and extreme morphological variation within the group (Bicknell, Reference Bicknell2019; Bicknell & Pates, Reference Bicknell and Pates2019; Bicknell et al. Reference Bicknell, Naugolnykh and Brougham2020). Interestingly, Permian and Jurassic forms lack the notable genal splay common to Triassic austrolimulids (Compare Tasmaniolimulus patersoni and F. pochankei to Dubbolimulus peetae and Austrolimulus fletcheri). This may reflect habitation of more brackish conditions, or the lack of morphological stock that would have permitted and maintained such forms. An explanation for why freshwater conditions were explored by non-belinurid xiphosurids remains somewhat nebulous. A likely explanation is associated with freshwater niches that were left vacant after the end-Permian extinction (Hu et al. Reference Hu, Zhang, Chen, Zhou, Lü, Xie, Wen, Huang and Benton2011; Chen & Benton, Reference Chen and Benton2012). Permian-aged, freshwater xiphosurids with hypertrophied genal spines may have diversified during the Triassic Period to fill the vacant niches, capitalizing on freshwater conditions. This does not address why these forms are unknown after Early Jurassic time. Lamsdell (Reference Lamsdell2020) recently tackled xiphosurid evolutionary dynamics and considered how primarily marine Limulidae was descendant from non-marine ancestors (Błażejowski et al. Reference Błażejowski, Niedźwiedzki, Boukhalfa and Soussi2017; Lamsdell, Reference Lamsdell2020). This may be possible. However, this hypothesis is contradicted by the record of Triassic-aged marine limulids, such as Sloveniolimulus rudkini Bicknell et al. Reference Bicknell, Žalohar, Miklavc, Celarc, Križnar and Hitij2019 e from the Strelovec Formation (Middle Triassic, Anisian), Slovenia and Heterolimulus gadeai Vía & De Villalta, Reference Vía and De Villalta1966 from the Alcover Limestone Formation. It is therefore more likely that the divergence of austrolimulids from the more standard limulid morphology resulted in forms that were not optimized for palaeoenvironmental and palaeoecological changes. As such, austrolimulids were likely outcompeted by other freshwater organisms leading into the rest of the Jurassic Period.
Acknowledgements
This research was supported by funding from an Australian Postgraduate Award (to RDCB), a University of New England Postdoctoral Research Fellowship (to RDCB), a Charles Schuchert and Carl O Dunbar Grants-in-Aid award (to RDCB), and a James R Welch Scholarship (to RDCB). We thank Allan Lerner, David Barnes, Gerhart Hundertmark, and Josh White for photographs of specimens. We thank Harald Stapf, Isabella von Lichtan, Mark Florence, Matthew McCurry, and Yong-Yi Zhen for help with collections. We thank Jan Fischer, Joachim M Rabold, and Ulrike Albert for discussions regarding the Pechgraben ecology. Finally, we thank the two anonymous reviewers for their constructive comments that improved the direction and use of the text.
Conflict of interest
None.