1. Introduction
Permian–Triassic boundary (PTB) volcanic ash beds are widely distributed in South China (Fig. 1). Their nature and origin have been studied for decades due to a possible relationship with the PTB mass extinction event and the assemblage of Pangea (e.g. Yin et al. Reference Yin, Feng, Lai, Baud and Tong2007; Shen et al. Reference Shen, Crowley, Wang, Bowring, Erwin, Sadler, Cao, Rothman, Henderson, Ramezani, Zhang, Shen, Wang, Wang, Mu, Li, Tang, Liu, Liu, Zeng, Jiang and Jin2011a; Burgess et al. Reference Burgess, Bowring and Shen2014; Baresel et al. Reference Baresel, Bucher, Brosse, Cordey, Guodun and Schaltegger2017). Petrographic and mineralogical studies demonstrate a felsic nature for these volcanic ash beds (He et al. Reference He, Chai and Ma1989; Yin et al. Reference Yin, Huang, Zhang, Yang, Ding, Bi and Zhang1989, Reference Yin, Huang, Zhang, Hansen, Yang, Ding, Bie, Sweet, Yang, Dickins and Yin1992; Yang et al. Reference Yang, Wu, Yin, Xu, Zhang and Bi1991). The connection between the PTB mass extinction and the Siberian Large Igneous Province led to the conclusion that these ash beds may represent distal deposits from basaltic volcanisms of the Siberian Large Igneous Province (e.g. Shen et al. Reference Shen, Algeo, Hu, Zhang, Zhou, Xia, Xie and Feng2012). Studies on platinum-group elements in the PTB global stratotype section and point Meishan section also suggest a source from the Siberian Large Igneous Province and probably the Emeishan Large Igneous Province (Xu et al. Reference Xu, Lin, Shen, Qi, Xie and Ouyang2007). Coeval tuffs found on the uppermost of the Emeishan basalt succession have led to the conclusion that these tuffs were sourced from the Emeishan Large Igneous Province (Zhu et al. Reference Zhu, Zhang, Hou and Kang2011). However, recent studies (Yang et al. Reference Yang, Cawood, Du, Huang, Huang and Tao2012; Gao et al. Reference Gao, Zhang, Xia, Feng, Chen, Zheng, Griffin, O’Reilly, Pearson, Wang, Wu, Zhong and Sun2013, Reference Gao, Chen, Zhang, Griffin, Xia, Wang, Jiang, Xia and O’Reilly2015; He et al. Reference He, Zhong, Xu and Li2014) based on U–Pb ages, trace elements and Hf isotopes of zircons suggest that these volcanic ash beds may be sourced from convergent continental margins in the eastern Tethyan region, but precise locations are subject to debate. Existing studies argue for the convergent continental margin of the southwestern South China Craton (Yang et al. Reference Yang, Cawood, Du, Huang, Huang and Tao2012; Gao et al. Reference Gao, Zhang, Xia, Feng, Chen, Zheng, Griffin, O’Reilly, Pearson, Wang, Wu, Zhong and Sun2013, Reference Gao, Chen, Zhang, Griffin, Xia, Wang, Jiang, Xia and O’Reilly2015) or the convergent continental margin in the East Kunlun area (He et al. Reference He, Zhong, Xu and Li2014). In addition, these convergent events may have led to the amalgamation of East Asian blocks and Pangea (e.g. Metcalfe Reference Metcalfe, Hall and Blundell1996, Reference Metcalfe2013; Huang et al. Reference Huang, Yan, Piper, Zhang, Yi, Yu and Zhou2018; Zhao et al. Reference Zhao, Zhang, Wang, Huang, Dong, Li and Yu2018). The timing of the amalgamation is still under debate, however (e.g. Scotese & McKerrow, Reference Scotese, McKerrow, McKerrow and Scotese1990; Collins, Reference Collins2003; Scotese, Reference Scotese2004; Golonka et al. Reference Golonka, Krobicki, Pająk, Nguyen and Zuchiewicz2006; Golonka, Reference Golonka2007; Metcalfe, Reference Metcalfe, Buffetaut, Cuny, Le Loeuff and Suteethorn2009; van der Meer et al. Reference van der Meer, Spakman, van Hinsbergen, Amaru and Torsvik2010; Cocks & Torsvik, Reference Cocks and Torsvik2013; Stampfli et al. Reference Stampfli, Hochard, Vérard, Wilhem and von Raumer2013; Domeier & Torsvik, Reference Domeier and Torsvik2014; Huang et al. Reference Huang, Yan, Piper, Zhang, Yi, Yu and Zhou2018; Zhao et al. Reference Zhao, Zhang, Wang, Huang, Dong, Li and Yu2018).

Fig. 1. Distribution of PTB volcanic ash beds in South China (upper part) and correlation of representative PTB sections (lower part). The semitransparent areas in the upper map represent the distribution of volcanic ash beds, and n represents the number of sections. Volcanic ash bed localities and lithological columns are compiled from Yin et al. (Reference Yin, Huang, Zhang, Yang, Ding, Bi and Zhang1989), Yang et al. (Reference Yang, Wu, Yin, Xu, Zhang and Bi1991, Reference Yang, Cawood, Du, Huang, Huang and Tao2012), Shen et al. (Reference Shen, Crowley, Wang, Bowring, Erwin, Sadler, Cao, Rothman, Henderson, Ramezani, Zhang, Shen, Wang, Wang, Mu, Li, Tang, Liu, Liu, Zeng, Jiang and Jin2011a), Zhu et al. (Reference Zhu, Zhang, Hou and Kang2011), Gao et al. (Reference Gao, Zhang, Xia, Feng, Chen, Zheng, Griffin, O’Reilly, Pearson, Wang, Wu, Zhong and Sun2013, Reference Gao, Chen, Zhang, Griffin, Xia, Wang, Jiang, Xia and O’Reilly2015), Burgess et al. (Reference Burgess, Bowring and Shen2014), He et al. (Reference He, Zhong, Xu and Li2014), Liao et al. (Reference Liao, Hu, Cao, Wang, Yao and Wan2016a, b), Baresel et al. (Reference Baresel, Bucher, Brosse, Cordey, Guodun and Schaltegger2017) and the SHP section in this study. Tectonic boundaries are from Zhang et al. (Reference Zhang, Dong, Lai, Guo, Meng, Liu, Cheng, Yao, Zhang, Pei and Li2004).
The PTB mass extinction was proposed to be triggered by Siberian Large Igneous Province flood basalt volcanism, which induced a world-wide lethally hot climate (e.g. Svensen et al. Reference Svensen, Planke, Polozov, Schmidbauer, Corfu, Podladchikov and Jamtveit2009; Joachimski et al. Reference Joachimski, Lai, Shen, Jiang, Luo, Chen, Chen and Sun2012; Sun et al. Reference Sun, Joachimski, Wignall, Yan, Chen, Jiang, Wang and Lai2012; Retallack, Reference Retallack2013; Cui & Kump, Reference Cui and Kump2015; Shen et al. Reference Shen, Feng, Algeo, Li, Planavsky, Zhou and Zhang2016). Additionally, the timing of the PTB biocrisis and late Permian – Early Triassic convergent margin volcanism in the Palaeotethyan region has suggested that the Palaeotethys ignimbrite flare-up may also have contributed to the PTB mass extinction (e.g. Isozaki, Reference Isozaki2009; Gao et al. Reference Gao, Zhang, Xia, Feng, Chen, Zheng, Griffin, O’Reilly, Pearson, Wang, Wu, Zhong and Sun2013; He et al. Reference He, Zhong, Xu and Li2014), but details of their relationship are still unclear.
In this study, we present data from three newly found PTB volcanic ash beds in the western Hubei area, South China, including zircon U–Pb ages, trace elements and Hf isotopes, and compare them with other PTB volcanic ash beds in South China. The source and tectonic affinity of these ashes are discussed. In addition, we also explore their possible relationship with the PTB mass extinction and the assemblage of Pangea.
2. Geological background
In South China, PTB volcanic ash beds, most of which have been diagenetically altered to clay beds, are widely distributed; up to 35 beds with a total thickness of up to 20 cm may be found in a single section (Fig. 1) (Yin et al. Reference Yin, Huang, Zhang, Yang, Ding, Bi and Zhang1989; Yang et al. Reference Yang, Wu, Yin, Xu, Zhang and Bi1991; He et al. Reference He, Zhong, Xu and Li2014). Their distinct color (e.g. white, yellow, light grey or greyish-green) and high content of clay minerals make them distinguishable from their surrounding rocks in the outcrop (Yang et al. Reference Yang, Wu, Yin, Xu, Zhang and Bi1991; Yin et al. Reference Yin, Huang, Zhang, Hansen, Yang, Ding, Bie, Sweet, Yang, Dickins and Yin1992; Hong et al. Reference Hong, Zhang, Li, Xue, Xia and Yu2008). Weighted mean ages of zircons from these ash beds range from 254.31 ± 0.07 Ma to 246.8 ± 1.3 Ma (Shen et al. Reference Shen, Crowley, Wang, Bowring, Erwin, Sadler, Cao, Rothman, Henderson, Ramezani, Zhang, Shen, Wang, Wang, Mu, Li, Tang, Liu, Liu, Zeng, Jiang and Jin2011a; Zhu et al. Reference Zhu, Zhang, Hou and Kang2011; Yang et al. Reference Yang, Cawood, Du, Huang, Huang and Tao2012; Gao et al. Reference Gao, Zhang, Xia, Feng, Chen, Zheng, Griffin, O’Reilly, Pearson, Wang, Wu, Zhong and Sun2013, Reference Gao, Chen, Zhang, Griffin, Xia, Wang, Jiang, Xia and O’Reilly2015; Burgess et al. Reference Burgess, Bowring and Shen2014; He et al. Reference He, Zhong, Xu and Li2014; Liao et al. Reference Liao, Hu, Cao, Wang, Yao and Wan2016a; Baresel et al. Reference Baresel, Bucher, Brosse, Cordey, Guodun and Schaltegger2017).
The Shuanghe Poumian (SHP) section is located at the village of Shuanghe village, western Hubei area in the northern margin of South China Craton (30° 9′ 49.6″ N, 109° 48′ 6.4″ E). A continuous and well-exposed upper Permian – Lower Triassic deepwater succession has recently been identified (Figs 1, 2). The lower part of the SHP section is composed of the upper Permian Dalong Formation and the upper part is the Lower Triassic Daye Formation (Fig. 3). The Dalong Formation is c. 60 m thick and mainly composed of carbonaceous mudstone and chert with mudstone occasionally interbedded (Fig. 3). The lower part of the Daye Formation is approximately 25 m thick and mainly composed of interbedded limestone and mudstone (Fig. 3). The rest part of the Daye Formation is composed of limestone of approximately 800 m thickness. Biostratigraphy indicates a time range of late Wuchiapingian (late Permian) to Induan (Early Triassic), that is, c. 255–250 Ma (Gradstein et al. Reference Gradstein, Ogg, Schmitz and Ogg2012), for the deposition of the Dalong Formation and the lower part of the Daye Formation (Fig. 4) (Zhang et al. Reference Zhang, He, Zhang, Yang and Wu2009; Feng & Algeo, Reference Feng and Algeo2014; Zheng et al. Reference Zheng, Mou, Wang, Xiao and Chen2019). Three PTB volcanic ash beds (beds 1 to 3) were studied (Figs 1, 3).

Fig. 2. (a) Global palaeogeography at PTB (modified from Spörli et al. Reference Spörli, Aita, Hori, Takemura, Spörli, Takemura and Hori2007). (b) Changhsingian palaeogeography of South China (modified from Feng et al. Reference Feng, Yang, Jin, He, Wu, Xin, Bao and Tan1996b; Feng & Algeo, Reference Feng and Algeo2014). 1 – land; 2 – continental facies; 3 – littoral detrital facies; 4 – shallow-water carbonate; 5 –deep-water chert-mudstone; NCC – North China Craton; SCC – South China Craton; SHP – Shuanghe Poumian.

Fig. 3. Lithologic column of the SHP section and sample locations.

Fig. 4. The late Permian – Early Triassic succession of the study area (after Zhang et al. Reference Zhang, He, Zhang, Yang and Wu2009; Zheng et al. Reference Zheng, Mou, Wang, Xiao and Chen2019). The ages of the succession are from Gradstein et al. (Reference Gradstein, Ogg, Schmitz and Ogg2012).
3. Samples and analytical methods
3.a. Sample description
Volcanic ash beds 1 to 3 were sampled and labelled as ZC, ZE and ZG, respectively (Fig. 3). These volcanic ash beds are 4–8 cm thick. They are distinct from their surrounding rocks in the outcrop due to their unique colour and high content of clay minerals similar to other sections in the adjacent area (Hong et al. Reference Hong, Zhang, Li, Xue, Xia and Yu2008; Gao et al. Reference Gao, Zhang, Xia, Feng, Chen, Zheng, Griffin, O’Reilly, Pearson, Wang, Wu, Zhong and Sun2013). Bed 1 is yellow to grey in colour and interbedded with carbonaceous mudstone at the top of the Dalong Formation. It contains millimetre-scale volcanic debris, indicating a proximal volcanic eruption (Fig. 5a, b). Beds 2 and 3 are grey-green in colour and interbedded with mudstone or limestone at the lower part of the Daye Formation (Fig. 5c, d).

Fig. 5. Outcrop photographs of the studied volcanic ash beds in the SHP section: (a, b) bed 1 (sample ZC) and (c, d) bed 3 (sample ZG).
3.b. Analytical methods
Zircon grains were separated using a combination of heavy liquid and magnetic separation techniques. Zircon grains were handpicked and mounted in epoxy and polished. Zircon grains were imaged by a Gatan mini-cathodoluminescence (CL) spectroscope attached to a JSM6510 scanning electron microscope at Langfang Yuheng Mineral and Rock Service Ltd., Hebei, China, in order to reveal their internal structures.
U–Pb dating and trace-element analysis of zircons were conducted using laser ablation inductively coupled plasma mass spectrometry (LA-ICP-MS) at the Key Laboratory for the study of focused Magmatism and Giant Ore Deposits, Ministry of Natural Resources, in Xi’an Center of Geological Survey, China Geological Survey. Reference materials, 91500 and Plesovice zircons, and NIST610 glass were used. Laser ablation was performed using a GeoLas Pro laser-ablation system coupled to an Agilent 7700 × ICP-MS and the beam diameter was 24 μm. Each analysis incorporated a background acquisition of approximately 10 s (gas blank) followed by 40 s data acquisition. The Agilent Chemstation was utilized for the acquisition of each individual analysis. Off-line selection and integration of background and analytical signals, and time-drift correction and quantitative calibration for U–Pb dating, were performed using UranOS 2.06a (http://www.sediment.uni-goettingen.de/staff/dunkl/software) and trace-element analyses using Iolite 3.0 (http://iolite-software.com/). Weighted mean U–Pb ages (with 95% confidence) and concordia plots were generated by IsoPlot 3.75 (Ludwig, Reference Ludwig2012). Grain ages use 207Pb/206Pb ages for grains older than 1.0 Ga and 206Pb/238U ages for younger grains.
In situ zircon Hf isotope analysis was performed using a GeoLas Pro laser-ablation system coupled to a Neptune multiple-collector ICP-MS at Xi’an Center of Geological Survey, China Geological Survey. Details of the instrumental conditions and data acquisition procedures are described by Meng et al. (Reference Meng, Liu, Liu, Liu, Yang, Wang, Shi and Cai2014). A beam diameter of 32 μm was used on top of the existing laser pit for U–Pb for those zircons with large grain sizes. Correction factors for 176Lu and 176Yb isobaric interferences on 176Hf are 176Lu/175Lu = 0.02658 and 176Yb/173Yb = 0.796218 (Chu et al. Reference Chu, Taylor, Chavagnac, Nesbitt, Boella, Milton, German, Bayon and Burton2002). Instrumental mass bias was corrected by normalizing Yb isotope ratios to 172Yb/173Yb = 1.35274 (Chu et al. Reference Chu, Taylor, Chavagnac, Nesbitt, Boella, Milton, German, Bayon and Burton2002) and Hf isotopic ratios to 179Hf/177Hf = 0.7325 using an exponential mass fractionation law. The mass bias behaviour of Lu was assumed to follow that of Yb. Details of the mass bias correction protocols are described in Iizuka & Hirata (Reference Iizuka and Hirata2005), Wu et al. (Reference Wu, Yang and Xie2006) and Hou et al. (Reference Hou, Li, Zou, Qu, Shi and Xie2007). Zircon GJ-1 was used as the reference standard and yielded a weighted mean 176Hf/177Hf ratio of 0.282030 ± 40 (2σ) for this study. This ratio overlaps with a weighted mean 176Hf/177Hf ratio of 0.282013 ± 19 (2σ) obtained by Elhlou et al. (Reference Elhlou, Belousova, Griffin, Pearson and O’Reilly2006). T Hf and T Hf2 are single- and two-stage Hf model ages, respectively. The ε Hf, T Hf and f Lu/Hf in this study are calculated following Meng et al. (Reference Meng, Liu, Liu, Liu, Yang, Wang, Shi and Cai2014), where: the decay constant for 176Lu is 1.867 × 10−11 a−1 (Amelin, Reference Amelin2005); 176Lu/177 Hf and 176Hf/177 Hf ratios for the chondritic reservoir are 0.0332 and 0.282772, respectively (Blichert-Toft & Albarede, Reference Blichert-Toft and Albarede1997); 176Lu/177Hf and present-day 176Hf/177Hf ratios for the depleted mantle are 0.0384 and 0.28325, respectively (Griffin et al. Reference Griffin, Pearson, Belousova, Jackson, van Achterbergh, O’Reilly and Shee2000); and the 176Lu/177 Hf ratio for average continental crust is 0.015 (Griffin et al. Reference Griffin, Wang, Jackson, Pearson and O’Reilly2002).
4. Results
4.a. Zircon U–Pb ages
A total of 43 zircons from sample ZC (bed 1), 40 from sample ZE (bed 2) and 57 from sample ZG (bed 3) were dated (online Supplementary Table S1, available at http://journals.cambridge.org/geo). Analysed zircons are 60–200 µm prismatic euhedral crystals (Fig. 6), suggesting little transportation. Most of them have distinct oscillatory zoning, implying a magmatic origin (Fig. 6).

Fig. 6. Representative zircon CL images of our samples and their U–Pb ages and ε Hf(t) values. Circles represent laser pits.
Among all zircons, 27 from ZC, 29 from ZE and 42 from ZG yield concordant U–Pb ages, and abundant ages fall near the PTB ranging from 237.2 ± 14.8 Ma to 271 ± 14.3 Ma (n = 90), which we used to calculate ages for the studied ash beds and obtained 252.2 ± 3.6 Ma (MSWD = 1.6) for Bed 1 (ZC), 251.6 ± 4.9 Ma (MSWD = 0.12) for Bed 2 (ZE) and 250.4 ± 2.4 Ma (MSWD = 0.46) for Bed 3 (ZG) (Supplementary Table S1; Fig. 7). Inherited zircon ages range from 444.8 ± 42.7 Ma to 2305.8 ± 60.4 Ma (n = 8) (Supplementary Table S1; Fig. 7).

Fig. 7. Zircon U–Pb concordia plots of samples (data-point error ellipses are 2σ).
4.b. Zircon trace elements
All zircons were analysed for their trace elements (online Supplementary Table S2, available at http://journals.cambridge.org/geo) and those with concordant U–Pb ages are summarized as follows. For all the samples, yttrium (Y) content ranges from 196 to 7340 ppm, hafnium (Hf) content ranges from 11090 to 18600 ppm and lead (Pb) content ranges from 11 to 735 ppm. The Th/U ratio ranges from 0.308 to 0.88 for sample ZC, 0.205–1.429 for sample ZE and 0.235–1.064 for sample ZG (Supplementary Table S1), indicating a magmatic origin (Rubatto, Reference Rubatto2002). The chondrite-normalized rare Earth element (REE) pattern shows enrichment of heavy rare Earth elements (HREE), positive Ce anomaly and negative Eu anomaly, similar to other PTB volcanic ash beds in South China (Fig. 8).
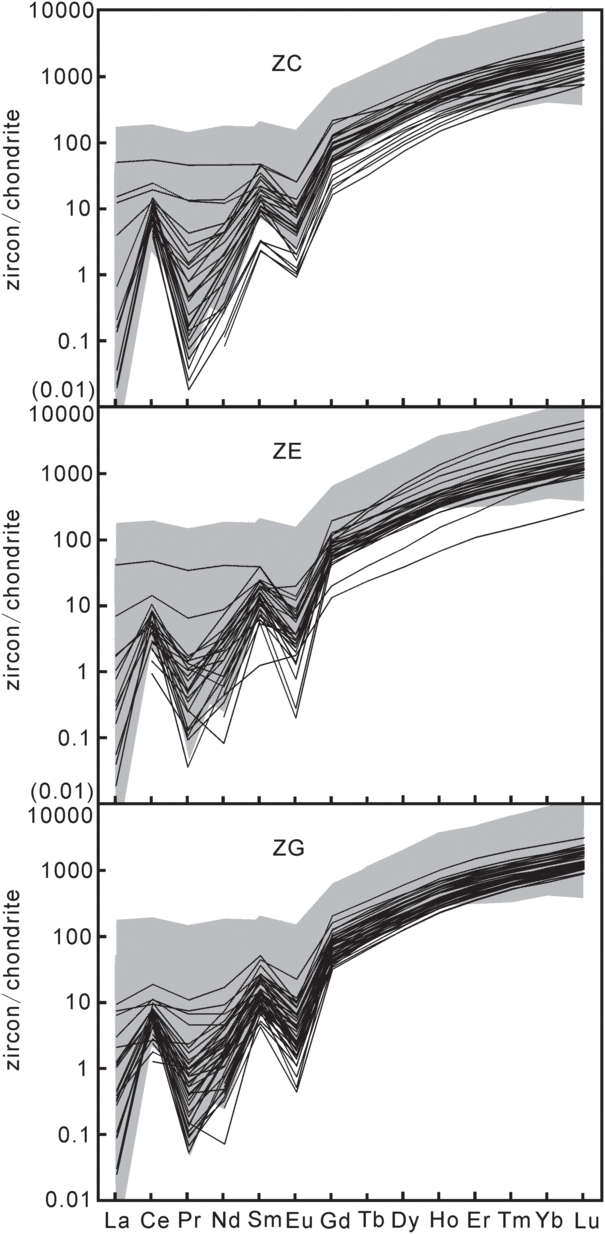
Fig. 8. Chondrite-normalized REE patterns for zircons. Chondritic values used for normalization are from Taylor & McLennan (Reference Taylor and McLennan1981). Curves represent zircons from the SHP section. Shaded areas are compiled from other PTB volcanic ash beds in South China (Yang et al. Reference Yang, Cawood, Du, Huang, Huang and Tao2012; Gao et al. Reference Gao, Zhang, Xia, Feng, Chen, Zheng, Griffin, O’Reilly, Pearson, Wang, Wu, Zhong and Sun2013, Reference Gao, Chen, Zhang, Griffin, Xia, Wang, Jiang, Xia and O’Reilly2015).
4.c. Zircon Hf isotopes
Sixty zircon grains were analysed by in situ zircon Hf isotope analysis (online Supplementary Table S3, available at http://journals.cambridge.org/geo; Fig. 9a). ε Hf(t) values of the zircons aged c. 240–270 Ma range from –18.17 to –3.91, which is generally more negative than zircons from other volcanic ash beds in South China (Fig. 9b). Their T Hf ages range from 0.84 to 1.46 Ga and T Hf2 ages range from 1.33 to 2.23 Ga. For inherited zircons, the ε Hf(t) values range from –50.98 to –9.59 with T Hf ages of 1.05–2.73 Ga and T Hf2 ages of 1.69–4.23 Ga.

Fig. 9. ε Hf(t) plots for zircons: (a) from the SHP section; and (b) of age c. 240–270 Ma different PTB sections in South China. Daxiakou section is from Gao et al. (Reference Gao, Zhang, Xia, Feng, Chen, Zheng, Griffin, O’Reilly, Pearson, Wang, Wu, Zhong and Sun2013), Zunyi section is from Gao et al. (Reference Gao, Chen, Zhang, Griffin, Xia, Wang, Jiang, Xia and O’Reilly2015), Meishan section, Chaotian section, Shangsi section and Dongpan section are from He et al. (Reference He, Zhong, Xu and Li2014).
5. Discussion
5.a. Source and tectonic affinity of the volcanic ash beds
Trace elements may be diagnostic for source rocks and related tectonic backgrounds for magmatic zircons (Shnukov et al. Reference Shnukov, Andreev and Savenok1997; Belousova et al. Reference Belousova, Griffin, O’Reilly and Fisher2002; Grimes et al. Reference Grimes, John, Kelermen, Mazdab, Wooden, Cheadle, Hanghoj and Schwartz2007; Gao et al. Reference Gao, Zhang, Xia, Feng, Chen, Zheng, Griffin, O’Reilly, Pearson, Wang, Wu, Zhong and Sun2013). Y versus U and Y versus (Yb/Sm)N plots show that zircons from PTB volcanic ash beds in the SHP section, as well as in other PTB sections in South China, were mainly distributed in the areas of felsic rocks, such as granitoids, larvikites and syenite pegmatites, and the area of mafic rocks that overlaps with felsic rocks (Fig. 10a, b). The Y versus Hf plot points to an intermediate to felsic source (Fig. 10c). The Y versus (U/Yb) diagram shows that these zircons were most likely sourced from felsic volcanic sources at convergent continental margins before post-collisional extension (Fig. 10d).

Fig. 10. Trace-element diagrams for source rock and tectonic setting discrimination of the zircons aged c. 240–270 Ma. (a–c) Y versus U, Y versus (Yb/Sm)N and Hf versus Y diagrams for source-rock discrimination (Shnukov et al. Reference Shnukov, Andreev and Savenok1997; Belousova et al. Reference Belousova, Griffin, O’Reilly and Fisher2002), where subscript N represents chondrite-normalized value; I – kimberlites; II – ultramafic, mafic and intermediate rocks; III – quartz-bearing intermediate and felsic rocks; IV – felsic rocks with high SiO2 content; V – greisens; VI – alkaline rocks and alkaline metasomatites of alkaline complexes; VII – carbonatites. (d) U/Yb versus Y diagram for tectonic setting discrimination (Grimes et al. Reference Grimes, John, Kelermen, Mazdab, Wooden, Cheadle, Hanghoj and Schwartz2007; Gao et al. Reference Gao, Zhang, Xia, Feng, Chen, Zheng, Griffin, O’Reilly, Pearson, Wang, Wu, Zhong and Sun2013). The red circle represents zircons from felsic rocks at convergent continental margins before post-collisional extension. The shaded area represents data compiled from other PTB volcanic ash beds in South China (after Yang et al. Reference Yang, Cawood, Du, Huang, Huang and Tao2012; Gao et al. Reference Gao, Zhang, Xia, Feng, Chen, Zheng, Griffin, O’Reilly, Pearson, Wang, Wu, Zhong and Sun2013, Reference Gao, Chen, Zhang, Griffin, Xia, Wang, Jiang, Xia and O’Reilly2015).
The values of ε Hf(t) and T Hf2 ages of the zircons aged c. 240–270 Ma in the SHP section as well as in other PTB sections in South China indicate that the parent magma might be mainly sourced from Palaeoproterozoic–Mesoproterozoic crustal materials (online Supplementary Table S3, available at http://journals.cambridge.org/geo; Figs 9, 11). However, pre-Palaeoproterozoic T Hf2 ages from ancient zircon cores (Figs 9, 11) provide a possibility that the source of magma may require some contribution from younger (post-Mesoproterozoic) juvenile materials. Slightly positive to slightly negative ε Hf(t) values in some sections also imply a contribution from juvenile mantle-derived mafic materials to the source magma (Fig. 9b) (e.g. Gao et al. Reference Gao, Zhang, Xia, Feng, Chen, Zheng, Griffin, O’Reilly, Pearson, Wang, Wu, Zhong and Sun2013).

Fig. 11. T Hf2 ages of zircons from different PTB volcanic ash beds in South China. Daxiakou section is from Gao et al. (Reference Gao, Zhang, Xia, Feng, Chen, Zheng, Griffin, O’Reilly, Pearson, Wang, Wu, Zhong and Sun2013), Zunyi section is from Gao et al. (Reference Gao, Chen, Zhang, Griffin, Xia, Wang, Jiang, Xia and O’Reilly2015), and Meishan, Chaotian, Shangsi and Dongpan sections are from He et al. (Reference He, Zhong, Xu and Li2014).
In summary, PTB volcanic ash beds in South China may be sourced from intermediate to felsic volcanisms at convergent continental margins before post-collisional extension. Their source magma may have been mainly derived from Palaeoproterozoic–Mesoproterozoic crustal materials with juvenile mantle-derived mafic materials contributing to varying degrees.
5.b. Location of the volcanic source
Two theories exist for the source of PTB volcanic ash beds in South China. A first view suggests that these volcanic ash beds may be sourced from the Siberian Large Igneous Province and probably the Emeishan Large Igneous Province (e.g. Xu et al. Reference Xu, Lin, Shen, Qi, Xie and Ouyang2007; Zhu et al. Reference Zhu, Zhang, Hou and Kang2011; Shen et al. Reference Shen, Algeo, Hu, Zhang, Zhou, Xia, Xie and Feng2012). For the Siberian Large Igneous Province, the maximum age for pyroclastic magmatism was dated at 255.58 ± 0.38 Ma and the youngest felsic tuff bed was dated at 251.403 ± 0.048 Ma (Burgess & Bowring, Reference Burgess and Bowring2015), indicating an overlap in timing with those coeval volcanic ash beds in South China except for those later during Early Triassic time. For the Emeishan Large Igneous Province in southwestern South China, the major basaltic eruption is thought to have occurred at c. 260 Ma (He et al. Reference He, Xu, Chung, Xiao and Wang2003) and the associated intermediate to felsic plutons were dated at c. 260–251 Ma (Xu et al. Reference Xu, Luo, Huang, He, Xiao, Xie and Shi2008; Shellnutt et al. Reference Shellnutt, Wang, Zhou and Yang2009; Zhong et al. Reference Zhong, Zhu, Hu, Xie, He, Liu and Chu2009, Reference Zhong, Campbell, Zhu, Allen, Hu, Xie and He2011; He, Reference He2016), regardless of the compositional discrepancy, also suggesting a timing overlap with those coeval volcanic ash beds in South China except for those later during Early Triassic time. A second view argues that they may have been sourced from intermediate to felsic volcanism that is related to the closure of the Palaeotethys Ocean or the formation of the Pangea supercontinent (Clark et al. Reference Clark, Wang, Orth and Gilmore1986; He et al. Reference He, Chai and Ma1989, Reference He, Zhong, Xu and Li2014; Yin et al. Reference Yin, Huang, Zhang, Yang, Ding, Bi and Zhang1989, Reference Yin, Huang, Zhang, Hansen, Yang, Ding, Bie, Sweet, Yang, Dickins and Yin1992, Reference Yin, Feng, Lai, Baud and Tong2007; Yang et al. Reference Yang, Wu, Yin, Xu, Zhang and Bi1991, Reference Yang, Cawood, Du, Huang, Huang and Tao2012; Isozaki et al. Reference Isozaki, Shimizu, Yao, Ji and Matsuda2007; Gao et al. Reference Gao, Zhang, Xia, Feng, Chen, Zheng, Griffin, O’Reilly, Pearson, Wang, Wu, Zhong and Sun2013, Reference Gao, Chen, Zhang, Griffin, Xia, Wang, Jiang, Xia and O’Reilly2015).
Our data argue for a convergent continental margin source for the PTB ash beds in South China; however, the precise location of the source volcanism is subject to debate. Existing studies argue for the convergent continental margin of the southwestern South China Craton (Yang et al. Reference Yang, Cawood, Du, Huang, Huang and Tao2012; Gao et al. Reference Gao, Zhang, Xia, Feng, Chen, Zheng, Griffin, O’Reilly, Pearson, Wang, Wu, Zhong and Sun2013, Reference Gao, Chen, Zhang, Griffin, Xia, Wang, Jiang, Xia and O’Reilly2015) or the convergent continental margin in the East Kunlun area (He et al. Reference He, Zhong, Xu and Li2014). However, except for the intermediate to felsic nature and their tectonic affinity to magmatic arc, the link between these possible parent volcanisms and PTB volcanic ash beds in South China was mainly developed based on obtained ages and the spatial variation in the thickness and numbers of the PTB ash beds.
During late Permian – Early Triassic time, the subduction of the Palaeotethys Ocean resulted in the formation of several convergent continental margins in the eastern Palaeotethys region (Huang et al. Reference Huang, Yan, Piper, Zhang, Yi, Yu and Zhou2018), including the Kunlun (e.g. Xiao et al. Reference Xiao, Windley, Hao and Li2002; Yan et al. Reference Yan, Bian, Korchagin, Pospelov, Li and Wang2008; Dai et al. Reference Dai, Wang, Hourigan and Santosh2013), Qinling (e.g. Guo et al. Reference Guo, Yan, Wang, Wang, Hou, Fu and Li2012; Yan et al. Reference Yan, Wang, Li, Xu and Deng2012, Reference Yan, Guo, Fu, Aitchison, Wang and Li2014; Dong & Santosh, Reference Dong and Santosh2016) and Simao–Indochina convergent continental margins (e.g. Cai & Zhang, Reference Cai and Zhang2009; Faure et al. Reference Faure, Lepvrier, Nguyen, Vu, Lin and Chen2014; Liu et al. Reference Liu, Wang, Li, Zi and Huangfu2018) (Fig. 12). These coeval convergent continental margins are the most likely sources for PTB ash beds in South China. Zircon Hf isotopes and model ages are useful indicators of tectonic setting and the nature of the associated magma, providing a possibility to precisely locate the parent volcanism of PTB ash beds in South China. Available data allow us to directly compare these Hf-related parameters of magmatic zircons in PTB ash beds in South China with those in coeval intermediate to felsic magmatic rocks (c. 255–246 Ma) in Simao–Indochina and East Kunlun convergent continental margins. For the coeval magmatic zircons in the Simao–Indochina convergent continental margin, both ε Hf(t) values and T Hf2 ages overlap those in PTB ash beds in South China, and their distribution patterns also show similarities with ε Hf(t) values of c. −12 to −4 and T Hf2 ages of c. 1.5–2.1 Ga (Fig. 13), indicating that the Simao–Indochina convergent continental margin may be the major source of PTB ash beds in South China. The coeval magmatic zircons in the East Kunlun convergent continental margin generally show higher ε Hf(t) values and younger T Hf2 ages compared with those in PTB ash beds in South China (Fig. 13), indicating the East Kunlun convergent continental margin is unlikely to be the major source. For the Qinling convergent continental margin, metamorphic ages of the subducted oceanic crust (Sun et al. Reference Sun, Williams and Li2002; Cheng et al. Reference Cheng, King, Nakamura, Vervoort, Zheng, Ota, Wu, Kobayashi and Zhou2009; Wu et al. Reference Wu, Hanchar, Gao, Sylvester, Tubrett, Qiu, Wijbrans, Brouwer, Yang, Yang, Liu and Yuan2009; Liu et al. Reference Liu, Wu, Gao, Wang, Peng, Gong, Liu and Yuan2011) and ages of arc-related volcanic rocks (Feng et al. Reference Feng, Du, Yin, Sheng and Xu1996a; Li et al. Reference Li, Hou, Yang, Sun, Zhang and Li2004) indicate that the subduction of the oceanic crust under the Qinling – North China composite continent occurred during Carboniferous time. Palaeomagnetic data (Zhao & Coe, Reference Zhao and Coe1987; Enkin et al. Reference Enkin, Yang, Chen and Courtillot1992; Yang et al. Reference Yang, Ma, Huang, Sun and Zhou1998; Zhu et al. Reference Zhu, Yang, Wu, Ma, Huang, Meng and Fang1998; Huang et al. Reference Huang, Yan, Piper, Zhang, Yi, Yu and Zhou2018) and high and ultrahigh-pressure eclogite facies metamorphic events in the Dabie mountains (Liu et al. Reference Liu, Jahn, Dong, Lou and Cui2008; Cheng et al. Reference Cheng, Zhang, Vervoort, Wu, Zheng, Zheng and Zhou2011; Gao et al. Reference Gao, Zheng and Chen2011; Liu & Liou, Reference Liu and Liou2011) support a scissors-like closure of the ocean between South and North China cratons that was initiated during Late Permian time, with its eastern part closed earlier. The final collision in the western orogen occurred during late Triassic time as evidenced by a large volume of collision-related granitoids in the western Qinling area (Wang et al. Reference Wang, Wang and Zhang2015). However, the suture zone has been intensely reworked by the Mesozoic and Cenozoic intracontinental orogeny and mostly covered by the Bashan arcuate thrust system (Zhang et al. Reference Zhang, Dong, Lai, Guo, Meng, Liu, Cheng, Yao, Zhang, Pei and Li2004), and the late Permian – Early Triassic intermediate to felsic magmatic rocks have not yet been found. However, as an adjacent coeval active continental margin (Fig. 12), it cannot be excluded as a possible source for the PTB ash beds in South China. Considering the large number of PTB ash beds and their wide distribution in South China (Fig. 1), they may not be derived from a single source. For example, magmatic zircons in the SHP section, adjacent to the Qinling convergent continental margin (Fig. 12), generally show lower ε Hf(t) values and older T Hf2 ages compared with those in other PTB ash beds in South China (Fig. 13), providing the possibility that they may be sourced from the Qinling convergent continental margin.

Fig. 12. Late Permian palaeogeography map of the Palaeotethys region showing the convergent continental margins around South China (modified from Huang et al. Reference Huang, Yan, Piper, Zhang, Yi, Yu and Zhou2018). Detailed palaeogeography of South China Craton is shown in Figure 2b.

Fig. 13. Zircon ε Hf(t) value and T Hf2 age histograms of PTB ash beds in South China and late Permian – Early Triassic intermediate to felsic magmatic rocks in Simao–Indochina and East Kunlun convergent continental margins. Data for South China PTB ash beds are compiled from Gao et al. (Reference Gao, Zhang, Xia, Feng, Chen, Zheng, Griffin, O’Reilly, Pearson, Wang, Wu, Zhong and Sun2013, Reference Gao, Chen, Zhang, Griffin, Xia, Wang, Jiang, Xia and O’Reilly2015) and He et al. (Reference He, Zhong, Xu and Li2014). Data for Simao–Indochina convergent continental margin are compiled from Zi et al. (Reference Zi, Cawood, Fan, Tohver, Wang and McCuaig2012), Li et al. (Reference Li, Wang, Yu, Hu, Ma and Huang2013) and Liu et al. (Reference Liu, Wang, Cawood, Fan, Cai and Xing2015). Data for East Kunlun convergent continental margin are compiled from Huang et al. (Reference Huang, Niu, Nowell, Zhao, Yu, Zhu, Mo and Ding2014), Ding et al. (Reference Ding, Liu and Yan2015) and Ren et al. (Reference Ren, Wang, Zhang, Wang, Huang, Feng, Teschner and Song2016).
5.c. Geological implications
The c. 252 Ma PTB mass extinction caused a reduction of c. 70% of terrestrial and c. 90% of marine species and the ecosystem took 5 Ma or longer to recover from the extinction (Chen et al. Reference Chen, Algeo and Bottjer2014; Algeo et al. Reference Algeo, Chen and Bottjer2015). The Siberian Large Igneous Province flood basalt volcanism was proposed to trigger the PTB mass extinction by inducing the lethally hot climate (Wignall, Reference Wignall2001; Retallack & Jahren, Reference Retallack and Jahren2008; Svensen et al. Reference Svensen, Planke, Polozov, Schmidbauer, Corfu, Podladchikov and Jamtveit2009; Joachimski et al. Reference Joachimski, Lai, Shen, Jiang, Luo, Chen, Chen and Sun2012; Shen et al. Reference Shen, Algeo, Hu, Zhang, Zhou, Xia, Xie and Feng2012, Reference Shen, Algeo, Feng, Zhou, Feng, Zhang and Huang2013, Reference Shen, Feng, Algeo, Li, Planavsky, Zhou and Zhang2016; Sun et al. Reference Sun, Joachimski, Wignall, Yan, Chen, Jiang, Wang and Lai2012; Retallack, Reference Retallack2013; Cui & Kump, Reference Cui and Kump2015). In addition, Isozaki (Reference Isozaki2009) and He et al. (Reference He, Zhong, Xu and Li2014) proposed that possible Palaeotethys ignimbrite flare-up may have injected a large volume of sulphur aerosols and ash particles into the late Permian – Early Triassic stratosphere, which may have caused severe environmental changes in the biosphere in a ‘volcanic ash winter’ scenario. Shen et al. (Reference Shen, Sun, Lin, Liu and Chai2011b) and Gao et al. (Reference Gao, Zhang, Xia, Feng, Chen, Zheng, Griffin, O’Reilly, Pearson, Wang, Wu, Zhong and Sun2013) proposed that, as a plausible heat source, silicic volcanism at a convergent continental margin related to Palaeotethys subduction may also have triggered the widespread wildfires on the continent, evidenced by combustion-derived black carbon and polynuclear aromatic hydrocarbons found in sediments in the PTB global stratotype section and point Meishan section. Our data reveal that the long-term intermediate to felsic volcanism at convergent continental margins around South China overlap temporally with the PTB biocrisis triggered by the Siberian Large Igneous Province flood basalt volcanism.
Pangea formed at c. 320–250 Ma with Laurasia in the north and Gondwana in the south (Fig. 12) (Smith & Livermore, Reference Smith and Livermore1991; Murphy & Nance, Reference Murphy and Nance2008; Stampfli et al. Reference Stampfli, Hochard, Vérard, Wilhem and von Raumer2013). However, it is controversial whether East Asian blocks have ever been a part of Pangea before it broke up during Early Jurassic time (e.g. Scotese & McKerrow, Reference Scotese, McKerrow, McKerrow and Scotese1990; Collins, Reference Collins2003; Scotese, Reference Scotese2004; Golonka et al. Reference Golonka, Krobicki, Pająk, Nguyen and Zuchiewicz2006; Golonka, Reference Golonka2007; Metcalfe, Reference Metcalfe, Buffetaut, Cuny, Le Loeuff and Suteethorn2009; van der Meer et al. Reference van der Meer, Spakman, van Hinsbergen, Amaru and Torsvik2010; Cocks & Torsvik, Reference Cocks and Torsvik2013; Stampfli et al. Reference Stampfli, Hochard, Vérard, Wilhem and von Raumer2013; Domeier & Torsvik, Reference Domeier and Torsvik2014; Huang et al. Reference Huang, Yan, Piper, Zhang, Yi, Yu and Zhou2018; Zhao et al. Reference Zhao, Zhang, Wang, Huang, Dong, Li and Yu2018). Regarding the South China Craton and the Simao–Indochina block, most reconstructions propose that they may have been separated from Pangea by the Palaeotethys Ocean (e.g. Collins, Reference Collins2003; Scotese, Reference Scotese2004; Golonka, Reference Golonka2007; Metcalfe, Reference Metcalfe, Buffetaut, Cuny, Le Loeuff and Suteethorn2009; Cocks & Torsvik, Reference Cocks and Torsvik2013; Domeier & Torsvik, Reference Domeier and Torsvik2014). Recent studies on palaeomagnetism and orogenic belts of East Asian blocks argue that these two blocks may have been connected to Pangea by c. 220 Ma (Huang et al. Reference Huang, Yan, Piper, Zhang, Yi, Yu and Zhou2018; Zhao et al. Reference Zhao, Zhang, Wang, Huang, Dong, Li and Yu2018). Our data support the theory that strong magmatic activity may have taken place in the Simao–Indochina active continental margin and possibly the Qinling active continental margin of North China Craton during late Permian – Early Triassic time, indicating that eastern branches of the Palaeotethys Ocean, which separated the South China Craton and Simao–Indochina block from Pangea, had not yet been completely consumed during this time (Fig. 12).
6. Conclusions
Three volcanic ash beds (beds 1 to 3) were reported in the late Permian – Early Triassic SHP section in the western Hubei area, South China. LA–ICP–MS U–Pb dating of zircons yields ages of 252.2 ± 3.6 Ma for bed 1, 251.6 ± 4.9 Ma for bed 2 and 250.4 ± 2.4 Ma for bed 3. Zircon trace-element signatures and Hf isotopes indicate that the PTB volcanic ash beds in South China were likely sourced from coeval intermediate to felsic volcanic sources at the Simao–Indochina convergent continental margin. The Qinling active continental margin may be another source, but this possibility requires further investigation before being considered seriously.
Our new data indicate that convergent continental margin magmatism took place around South China during late Permian – Early Triassic time, especially in the Simao–Indochina active continental margin and possibly the Qinling active continental margin. These volcanisms took place around the same time as the PTB biocrisis triggered by the Siberian Large Igneous Province. In addition, our data indicate that the South China Craton and Simao–Indochina block had not been amalgamated with Pangea by late Permian – Early Triassic time.
Acknowledgments
This work was supported by the Shale Gas Development Co. Ltd, Hubei Province, China (contract no. HBYYQ-GC1075) and the National Natural Science Foundation of China (project no. 41772113). Binsong Zheng was supported by the Joint Training study program for excellent doctoral candidates at the School of Earth Sciences, China University of Geosciences (Wuhan). We would also like to thank the editor and the reviewers for their constructive comments and suggestions.
Supplementary material
To view supplementary material for this article, please visit https://doi.org/10.1017/S001675681900133X.