1. Introduction
The well-exposed Ordovician strata in the Nyalam region, southern Tibet (Fig. 1) are composed of shallow-marine carbonate, fossiliferous rocks and some siltstone and shale. The sediments accumulated on the north Indian continental margin of Gondwana; however, much of these were lost as a result of the subduction of Greater India beneath Asia and subsequent Cenozoic deformation (e.g. Yin & Harrison, Reference Yin and Harrison2000; Sengör & Natal’in, 2005; Yin, Reference Yin2006; Gehrels et al. Reference Gehrels, Kapp, Decelles, Pullen, Blakey, Weislogel, Ding, Guynn, Martin, Mcquarrie and Yin2011; Martin, Reference Martin2017). Today, these lower Palaeozoic sedimentary rocks are preserved in the Tethyan–Himalayan block of Himalaya and are part of the Tethyan Himalayan Sequence (THS; Palaeoproterozoic–Eocene; Yin & Harrison, Reference Yin and Harrison2000; Yin, Reference Yin2006; Cawood & Buchan, Reference Cawood and Buchan2007; Myrow et al. Reference Myrow, Hughes, Goodge, Fanning, Williams, Peng, Bhargava, Parcha and Pogue2010, Reference Myrow, Hughes, McKenzie, Treloar and Searle2018).
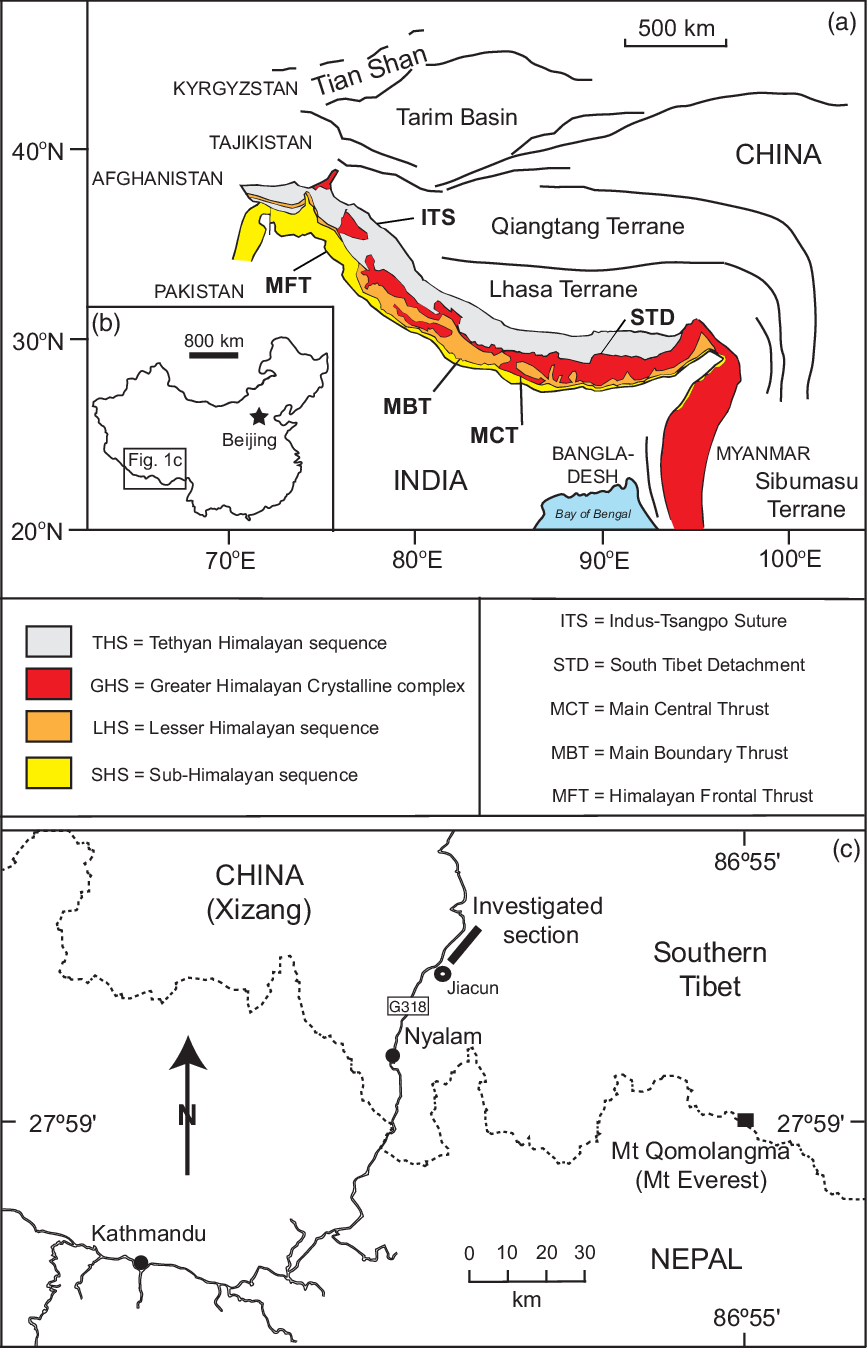
Fig. 1. (a) Himalayan tectonic significant structural elements and terranes. (b) Location of Tibet in China. (c) Location of the section at Jacun and to the north of Nyalam.
Harper et al. (Reference Harper, Zhan, Stemmerik, Liu, Donovan, Stouge, Gutiérrez-Marco, Rábano and García-Bellido2011), Donovan et al. (Reference Donovan, Harper, Zhan, Stemmerik, Liu and Stouge2012) and Zhan et al. (Reference Zhan, Harper, Jin, Liang, Liu, Stemmerik and Stouge2014) presented data based on fossils collected in 2009 from the Ordovician sedimentary rocks of the Chiatsun Group in the section north of the town of Nyalam, Everest area (Fig. 1c). These papers focused on the crinoid and brachiopod assemblages; this paper is a continuation of this series of papers. This study is mainly confined to the Lower and Upper formations and presents the results from newly recorded conodonts from the same Middle (Darriwilian) Ordovician strata of the Nyalam section. The aim of this contribution is to document the new recovered material, combine it with previous information of the Middle Ordovician conodont fauna of southern Tibet, and discuss the stratigraphial distribution of the species in order to accurately correlate to other Peri-Gondwana terranes in China, Thailand and Malaysia.
2. Geological setting and stratigraphy of the Chiatsun Group
2.a. Geology
The 2400-km-long Himalayan Belt is traditionally divided into four major parallel and lithotectonic belts or tectonic zones that have been recognized along-strike along the length of the mountain chain (e.g. Heim & Gansser, Reference Heim and Gansser1939; Gansser, Reference Gansser1964; Le Fort, Reference Le Fort1975; Fuchs, Reference Fuchs1981; DeCelles et al. Reference DeCelles, Gehrels, Quade, Kapp, Ojha and Upreti1998, Reference DeCelles, Robinson and Zandt2002; Hodges, Reference Hodges2000; Murphy & Yin, Reference Murphy and Yin2003; Chakrabarti, Reference Chakrabarti2016) (Fig. 1b). These structural belts are separated from one another by N-dipping faults and thrust zones that were formed as a result of shortening caused by the collision of the India Plate and the Euroasian (Tibetan) Plate during the Cenozoic Era (Hodges, Reference Hodges2000; Yin & Harrison, Reference Yin and Harrison2000; Gehrels et al. Reference Gehrels, Decelles, Martin, Ojha, Pinhassi and Upreti2003; Yin, Reference Yin2006; Kellett et al. Reference Kellett, Cottle, Larson, Treloar and Searle2018; Searle & Treloar, Reference Searle, Treloar, Treloar and Searle2019). The major structural boundaries of the India–Tibet collision system include the main frontal thrust (MFT), main boundary thrust (MBT), main central thrust (MCT), South Tibetan detachment (SDT normal fault) and the Indus (Yarlung)–Tsangpo suture zone (ITS) (e.g. Gansser, Reference Gansser1983; Burchfiel et al. Reference Burchfiel, Zhiliang, Hodges, Yuping, Royden, Changrong and Jiene1992; Hodges, Reference Hodges2000; Murphy & Yin, Reference Murphy and Yin2003; Yin, Reference Yin2006) (Fig. 1a). These traditional structural subdivisions are currently being revised based on new information indicating that the major structures cross major lithological units and metamorphic rocks and often intergrade laterally with their non-metamorphic equivalent strata (Yin, Reference Yin2006; Yin et al. Reference Yin, Dubey, Webb, Kelty, Grove, Gehrels and Burgess2010; McKenzie et al. Reference McKenzie, Hughes, Myrow, Choi and Park2011; Martin, Reference Martin2017; Myrow et al. Reference Myrow, Hughes, McKenzie, Treloar and Searle2018; Searle & Treloar, Reference Searle, Treloar, Treloar and Searle2019).
The Indus–Tsangpo suture zone (ITS, Fig. 1a), extending for c. 3000 km from Myanmar in the east to Afghanistan in the west, represents the northern limit of the Himalaya (Searle, Reference Searle1991; Garzanti, Reference Garzanti1999; Hodges, Reference Hodges2000; Yin & Harrison, Reference Yin and Harrison2000; Searle & Treloar, Reference Searle, Treloar, Treloar and Searle2019). It defines the areas of collision between the Indian and the Eurasian plates. The major stratigraphical divisions south of the ITS includes the Tethyan Himalaya Sequence (THS), Greater Himalaya Crystalline Complex (GHC), the Lesser Himalaya Sequence (LHS) and the Sub-Himalayan sequence (Fig. 1a). The Tethyan (Tibetan) Himalaya Sequence that lies immediately to the south of the ITS is composed of Precambrian (Archean and Proterozoic) crystalline basement rocks, and an overlying cover of Palaeozoic–Mesozoic marine sedimentary rocks and Palaeozoic–Cenozoic magmatic rocks (e.g. Garzanti et al. Reference Garzanti, Casnedi and Jadoul1986, Reference Garzanti, Baud and Mascle1987; Myrow et al. Reference Myrow, Thompson, Hughes, Paulsen, Sell and Parcha2006, Reference Myrow, Hughes, Searle, Fanning, Peng and Parcha2009; Yin, Reference Yin2006; Zou et al. Reference Zou, Zhu, Jia and Zhou2006). The GHC comprises high-grade metamorphic rocks. The Lesser Himalaya consists of clastic sediments and low-grade metamorphic sedimentary rocks (Brookfield, Reference Brookfield1993). The Sub-Himalayan sequence (Neogene Siwalik Group) is a molasse basin composed of continental sediments that eroded the rising Himalaya to the north.
Events around the Proterozoic–Palaeozoic boundary commenced with Neoproterozoic rifting, and the subsequent separation along the northern margin of Gondwanaland opened the Palaeo-Tethys Ocean. During this interval, a passive margin formed with the development of an extensive siliciclastic and carbonate platform (e.g. Yin & Kuo, Reference Yin and Kuo1978a, b; Garzanti et al. Reference Garzanti, Casnedi and Jadoul1986; Brookfield, Reference Brookfield1993; Steck, Reference Steck2003; Cawood et al. Reference Cawood, Johnson and Nemchin2007; Myrow et al. Reference Myrow, Hughes, Searle, Fanning, Peng and Parcha2009, Reference Myrow, Hughes, McKenzie, Treloar and Searle2018; Torsvik et al. Reference Torsvik, Paulson, Hughes, Myrow and Ganerød2009; Hughes, Reference Hughes2016). The margin convergence and subduction together with the assembly of Gondwana blocks was initiated at c. 510 Ma (late Cambrian). The Indian and Antarctic cratons collided during the late Cambrian Period, developing a prominent unconformity that separates the Cambrian marine strata from the Ordovician marine sedimentary rocks. The unconformity, which is indicated by a conspicuous and laterally extensive Ordovician conglomerate, was probably the response to the accretion of an outboard block or terrane during latest Cambrian – earliest Ordovician time (e.g. Garzanti et al. Reference Garzanti, Casnedi and Jadoul1986; Gehrels et al. Reference Gehrels, Decelles, Martin, Ojha, Pinhassi and Upreti2003, Reference Gehrels, Decelles, Ojha and Upreti2006; Zhou et al. Reference Zhou, Liu and Liang2004; Cawood et al. Reference Cawood, Johnson and Nemchin2007; Myrow et al. Reference Myrow, Hughes, McKenzie, Pelgay, Thomson, Haddad and Fanning2016, Reference Myrow, Hughes, McKenzie, Treloar and Searle2018). The early Palaeozoic tectonic phase with the penetrative deformation, metamorphism and igneous activity has been referred to the Kurgiakh (Srikantia et al. Reference Srikantia, Ganesan, Rao, Sinha and Turkey1980) and/or Bhimphedian Orogeny (Cawood et al. Reference Cawood, Johnson and Nemchin2007). The accretion of the terrane, followed by the development of a foreland basin and sedimentation, persisted in a passive margin setting until the beginning of the India–Euroasia collision during the Palaeogene Period (e.g. Hodges, Reference Hodges2000; Yin, Reference Yin2006).
2.b. The Nyalam–Jiacun region, southern Tibet
The upper Precambrian (Neoproterozoic) rocks in southern Tibet, which rest on a Precambrian crystalline basement (Zou et al. Reference Zou, Zhu, Jia and Zhou2006), are metamorphosed, folded and faulted and collectively referred to the Jolmo Lungma Group/Beiao Formation and Nyalam Group (Liu & Einsele, Reference Liu and Einsele1994). The overlying Cambrian rocks at the Nyalam site are structurally separated from the Precambrian deposits by the Lhotse detachment zone. The Cambrian meta-sedimentary rocks consist of sheared quartzite and psammitic schist with abundant granitic veins in the lower part, overlain by an upper unit composed of ribbon to parted dolostone. In the Nyalam region these sedimentary siliciclastic and carbonate rocks are referred to the Rouqiecun Group (Lombardo et al. Reference Lombardo, Pertusati, Borgi, Treloar and Searle1993). The Rouqiecun Group is mainly non-fossiliferous, but considered to be of early and middle Cambrian in age based on the biostratigraphical information recorded from the lateral equivalent strata in western Himalaya (Myrow et al. Reference Myrow, Hughes, Searle, Fanning, Peng and Parcha2009; Peng et al. Reference Peng, Hughes, Heim, Sell, Zhu, Myrow and Parcha2009; Hughes et al. Reference Hughes, Myrow, McKenzie, Harper, Bhargava, Tangri, Ghalley and Fanning2011; Hughes, Reference Hughes2016). Absolute dating based on zircons from the upper Rouqiecun Group indicates an age of 526 ± 7 Ma (Myrow et al. Reference Myrow, Hughes, Searle, Fanning, Peng and Parcha2009), confirming the middle Cambrian age of the upper Rouqiecun Group. The Rouqiecun laminated siliciclastic and crystalline carbonate rocks are overlain by the famous 109-m-thick Yellow Band, which is composed of foliated, yellow- to orange-weathering, bedded dolostone (Sakai et al. Reference Sakai, Sawada, Takigami, Orihashi, Danhara, Iwano, Kuwahara, Dong, Cai and Li2005; Myrow et al. Reference Myrow, Hughes, Searle, Fanning, Peng and Parcha2009).
The unconformable overlying Ordovician marine sedimentary carbonate rocks, exposed to the north of Nyalam, southern Tibet, are non-metamorphosed, but deformed (Wang, Reference Wang1974; Myrow et al. Reference Myrow, Hughes, Searle, Fanning, Peng and Parcha2009; Zhan et al. Reference Zhan, Harper, Jin, Liang, Liu, Stemmerik and Stouge2014). In the Nyalam region the non-metamorphosed Ordovician strata are structurally separated from the Cambrian Yellow Band by composite low angle faults named Qomolangma-Lhotse detachments (= South Tibetan detachment of Burchfiel et al. Reference Burchfiel, Zhiliang, Hodges, Yuping, Royden, Changrong and Jiene1992; Sakai et al. Reference Sakai, Sawada, Takigami, Orihashi, Danhara, Iwano, Kuwahara, Dong, Cai and Li2005; Myrow et al. Reference Myrow, Hughes, Searle, Fanning, Peng and Parcha2009).
2.c. Previous research on Ordovician rocks of southern Tibet
The carbonate rocks at Nyalam (Tethyan Himalayan) and Mount Qomolangma (Mount Everest) were initially considered to belong to the Carboniferous–Permian systems (Odell, Reference Odell1924, Reference Odell1925; Wager, Reference Wager and Ruttledge1934; Gansser, Reference Gansser1964). The Tibet Scientific Expedition in the Jolmo Lungma Region (1966–1968), arranged by the Chinese Academy of Sciences, generated much new information on the stratigraphy and geology of the Nyalam succession and the rocks on the northern slope of Mount Qomolangma (e.g. Mu et al. Reference Mu, Wen, Wang, Zhang and Yin1973; Wang, Reference Wang1974; Mu & Wu, Reference Mu and Wu1975; Yin & Kuo, Reference Yin and Kuo1978a, b, Reference Yin and Kuo1979; Yin & Kuo, Reference Yin and Kuo1979; Wen et al. Reference Wen, Zhang, Wang, Sun, Dong, Yin, Wu, Chen, Wang, He, Mu, Geng, Liao, Chen and Guo1984; Yin, Reference Yin1987; Burchfiel et al. Reference Burchfiel, Zhiliang, Hodges, Yuping, Royden, Changrong and Jiene1992; Zhan et al. Reference Zhan, Harper, Jin, Liang, Liu, Stemmerik and Stouge2014). The limestone on the top of Mount Qomolangma was assigned to the Lower and Middle Ordovician in the Chinese chronostratigraphic scheme (Liu et al. Reference Liu, Ma, Jin and He1962; Liu, Reference Liu1976, Reference Liu1979; Qian, Reference Qian1976; Zhan et al. Reference Zhan, Harper, Jin, Liang, Liu, Stemmerik and Stouge2014).
Harris et al. (Reference Harris, Repetski, Kauffman and Sando1987) reported and illustrated Middle Ordovician conodonts from a section exposed along the east side of the road between Yagru Xongla Pass and Nyalam, c. 24 km NNE of Nyalam (Fig. 1c). Harris et al. (Reference Harris, Repetski, Kauffman and Sando1987) also recorded a fauna from a red limestone horizon of the Upper Formation and referred this conodont fauna to the Pygodus serra conodont Zone, but the sample position within the succession of the Chiatsun Group is unknown. Qiu (Reference Qiu1988) presented the first Ordovician conodont zonation for southern, northern and eastern Tibet (Fig. 2) based on collections from strata to the north of Nyalam (southern Tibet). However, the precise stratigraphic position of the conodont samples and zones within the succession was not provided. Recently, Harper et al. (Reference Harper, Zhan, Stemmerik, Liu, Donovan, Stouge, Gutiérrez-Marco, Rábano and García-Bellido2011), Donovan et al. (Reference Donovan, Harper, Zhan, Stemmerik, Liu and Stouge2012) and Zhan et al. (Reference Zhan, Harper, Jin, Liang, Liu, Stemmerik and Stouge2014) described brachiopods and updated information on the crinoid fauna, and Yu et al. (Reference Yu, Fang, Munnecke, Li, Li, Wang and Zhang2019) have investigated the sedimentary features and illustrated conodonts extracted from within the Lower Formation (= Alai Formation of those authors). Zhang et al. (Reference Zhang, Zhan, Zhen, Wang, Yuan, Fang, Ma and Zhang2019, fig. 5) presented a correlation scheme for all of China with information from southern Tibet, and provided moderate modifications to the original conodont zonal scheme of Qiu (Reference Qiu1988).

Fig. 2. Previous biozonation and stratigraphical nomenclature from southern Tibet compared with the nomenclature used in this study (conodont zones are from Qiu (Reference Qiu1988) and Yu et al. (Reference Yu, Fang, Munnecke, Li, Li, Wang and Zhang2019)). The biozones of Qiu (Reference Qiu1988) are slightly revised; the biozones labelled in bold are those established in this paper. Brachiopod associations are from Harper et al. (Reference Harper, Zhan, Stemmerik, Liu, Donovan, Stouge, Gutiérrez-Marco, Rábano and García-Bellido2011) and Zhan et al. (Reference Zhan, Harper, Jin, Liang, Liu, Stemmerik and Stouge2014); cephalopod assemblages are from Wang (Reference Wang1974).
Although the conodont fauna presented by Harris et al. (Reference Harris, Repetski, Kauffman and Sando1987) and Qiu (Reference Qiu1988) are in need of taxonomic revision, these authors were the first to provide basic information on conodonts from the Middle and Upper Ordovician succession in southern Tibet.
3. Study area
3.a. Location
The Ordovician carbonate platform in the Himalayas is accessible on both sides of the road between the Yagru Xongla Pass and Nyalam, where a succession composed of marine carbonates, fossiliferous sedimentary rocks and minor shale is well exposed (Fig. 1c). These sections represent the Tibetan lower Palaeozoic strata and the whole of the Tethys (Tibetan) Himalayas. The conodont specimens documented here were recovered from the outcrops in the area along the Friendship Highway extending from Nyalam to Jiacun, and the studied section is located c. 50 km north of the town of Nyalam (Figs 1c, 3). The Nyalam district lies c. 75 km to the west of Mount Qomolangma (Mount Everest) (Fig. 1c), and the two areas are geological similar and laterally comparable (Wang, Reference Wang1974; Yin, Reference Yin1987; Qiu, Reference Qiu1988; Sakai et al. Reference Sakai, Sawada, Takigami, Orihashi, Danhara, Iwano, Kuwahara, Dong, Cai and Li2005; Myrow et al. Reference Myrow, Hughes, Searle, Fanning, Peng and Parcha2009; Harper et al. Reference Harper, Zhan, Stemmerik, Liu, Donovan, Stouge, Gutiérrez-Marco, Rábano and García-Bellido2011; Zhan et al. Reference Zhan, Harper, Jin, Liang, Liu, Stemmerik and Stouge2014; Corthouts et al. Reference Corthouts, Lageson and Shaw2015).
3.b. Stratigraphy
Several named lithological units (and subunits) have been introduced for the Middle Ordovician platformal deposits and Upper Ordovician deeper-water succession in Nyalam (e.g. Wang, Reference Wang1974; Lin & Qiu, Reference Lin and Qiu1982; Wei & Tan, Reference Wei and Tan1983; Xia, Reference Xia1983, Reference Xia1997; Chen, Reference Chen1984; Qiu, Reference Qiu1988; Zhang, Reference Zhang1993; Shi & Wang, Reference Shi and Wang1999; Zhao et al. Reference Zhao, Huang, Du, Zhang, Xiao and Tan2005). Here the stratigraphic nomenclature of Wang (Reference Wang1974) is used, that is, following Zhan et al. (Reference Zhan, Harper, Jin, Liang, Liu, Stemmerik and Stouge2014), and the Nyalam Middle Ordovician and Upper Ordovician deposits are referred to the Chiatsun Group and the overlying Hungshantou Formation (Mu et al. Reference Mu, Wen, Wang, Zhang and Yin1973; Wang, Reference Wang1974; Fig. 2). The depositional environment of the Middle–Upper Ordovician deposits generally reflects a deepening succession, that is, from platformal warm-water proximal deposits (Harper et al. Reference Harper, Zhan, Stemmerik, Liu, Donovan, Stouge, Gutiérrez-Marco, Rábano and García-Bellido2011; Yu et al. Reference Yu, Fang, Munnecke, Li, Li, Wang and Zhang2019) to the middle part of a carbonate shelf in the middle Darriwilian deposits, changing to deeper-water deposition in the upper Chiatsun Group to deep-water deposition of the Hungshantou Formation during late Middle – Late Ordovician time.
The contact between the Chiatsun Group and the underlying Yellow Band is tectonic (Burchfiel et al. Reference Burchfiel, Zhiliang, Hodges, Yuping, Royden, Changrong and Jiene1992; Sakai et al. Reference Sakai, Sawada, Takigami, Orihashi, Danhara, Iwano, Kuwahara, Dong, Cai and Li2005; Myrow et al. Reference Myrow, Hughes, Searle, Fanning, Peng and Parcha2009). This lower contact in southern Tibet also serves as the boundary between metamorphosed strata below and non-metamorphosed sedimentary rocks above the boundary. The Chiatsun Group is composed of the Lower and Upper formations (Figs 2, 4).
The Lower Formation, 726 m thick, consists of carbonates comprising rhythmic peritidal dolostone, bioclastic shelf limestone and sandy limestone (Yu et al. Reference Yu, Fang, Munnecke, Li, Li, Wang and Zhang2019). Myrow et al. (Reference Myrow, Hughes, Searle, Fanning, Peng and Parcha2009) observed a prominent thrombolite marker bed, 65 m thick, from c. 105 m above the base of the Lower Formation that caps the lowermost limestone beds. Above the thrombolite marker the beds are composed of rhythmic bedded, peritidal carbonates intercalated with dolomitic limestone and subtidal limestone (Figs 3, 4). The lower part accumulated in a warm, shallow-water depositional environment (Harper et al. Reference Harper, Zhan, Stemmerik, Liu, Donovan, Stouge, Gutiérrez-Marco, Rábano and García-Bellido2011; Yu et al. Reference Yu, Fang, Munnecke, Li, Li, Wang and Zhang2019), whereas the higher strata represent increasingly deeper and cooler subtidal deposits with silty intercalations (Harper et al. Reference Harper, Zhan, Stemmerik, Liu, Donovan, Stouge, Gutiérrez-Marco, Rábano and García-Bellido2011; Zhan et al. Reference Zhan, Harper, Jin, Liang, Liu, Stemmerik and Stouge2014).
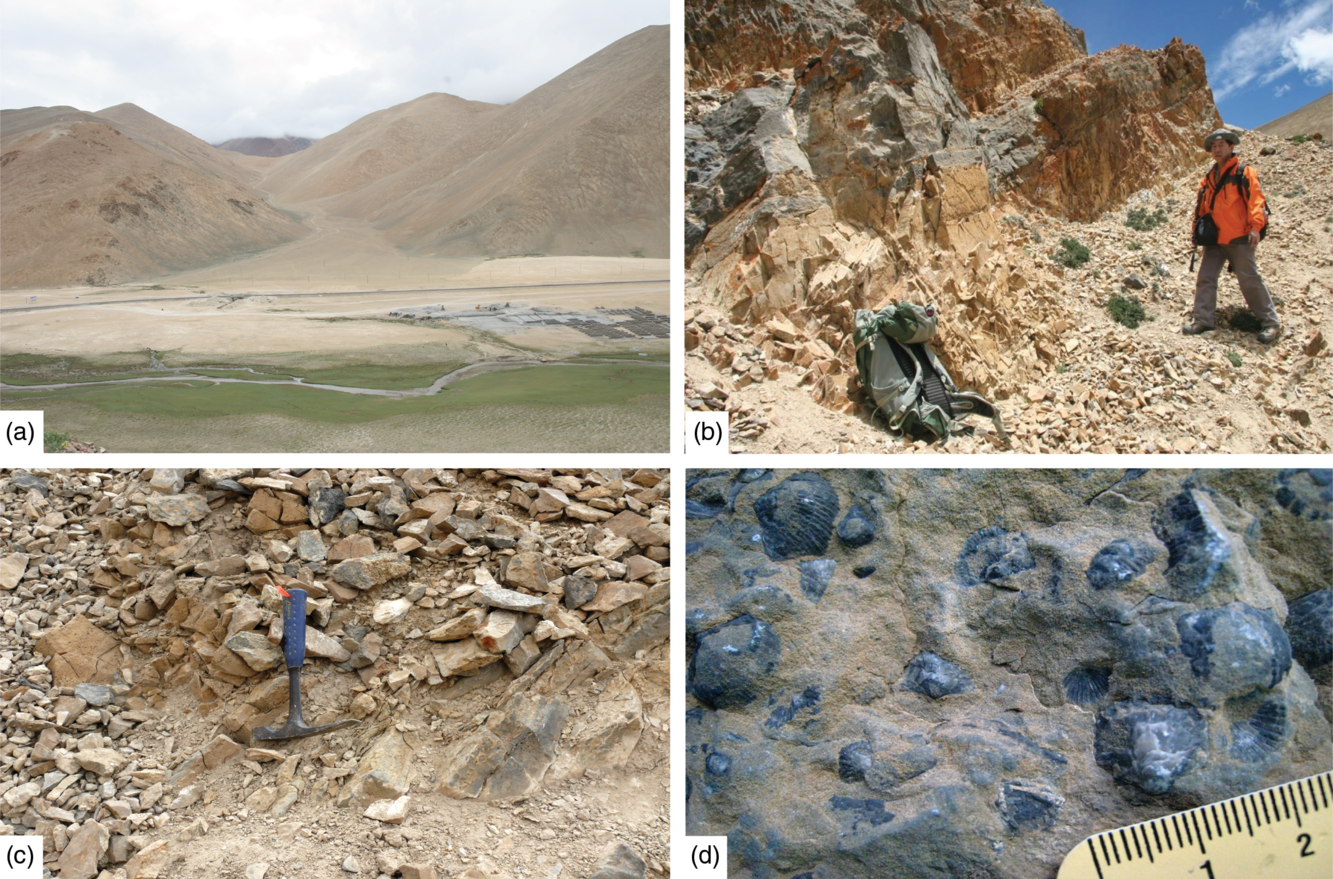
Fig. 3. Photographs of (a) the lower–middle part of the Lower Formation of the Chiatsun Group with brachiopods in the hill to the right of the photo; the Upper Formation of the Chiatsun Group with crinoids is seen in the hill to the left of the valley, on the far side of the G318, Friendship Highway, adjacent to the Village of Jiacun, northern Nyalam, Tibet (see also Donovan et al. Reference Donovan, Harper, Zhan, Stemmerik, Liu and Stouge2012). (b) Dolomite and limestone cycles within the Lower Formation, on the G318, Friendship Highway, adjacent to the village of Jiacun, northern Nyalam, Tibet. (c) Sporadic shelly lenses, dominated by brachiopods in the Lower Formation on the Friendship Highway, adjacent to village of Jiacun, Tibet (see also Zhan et al. Reference Zhan, Harper, Jin, Liang, Liu, Stemmerik and Stouge2014). (d) Field photograph of shell concentrations dominated by orthidine brachiopods (locality as for (c)). Scale graduated in millimetres.

Fig. 4. Vertical column of the Chiatsun Group with position of the collected samples and ranges of selected conodont species recorded from the studied interval in the Nyalam–Jiacun section. sh – shale; si – siltstone; s – sandstone; d – dolostone; l – limestone; p – packstone; t – thrombolite.
The sedimentary rocks of the Lower Formation are assigned to the Middle Ordovician based on its brachiopods, cephalopods, trilobites and conodonts (Wang, Reference Wang1974; Chen, Reference Chen1975, Reference Chen1984; Liu, Reference Liu1976, Reference Liu1979; Yin & Kuo, Reference Yin and Kuo1978a, b; Harris et al. Reference Harris, Repetski, Kauffman and Sando1987; Yin, Reference Yin1987; Qiu, Reference Qiu1988; Burchfiel et al. Reference Burchfiel, Zhiliang, Hodges, Yuping, Royden, Changrong and Jiene1992; Zhan et al. Reference Zhan, Harper, Jin, Liang, Liu, Stemmerik and Stouge2014). Biostratigraphically, the Lower Formation is subdivided into the lower Ordosoceras–Manchuroceras Biozone and the upper Dideroceras–Paradnatoceras Biozone. Zhan et al. (Reference Zhan, Harper, Jin, Liang, Liu, Stemmerik and Stouge2014) referred the brachiopod fauna from the Lower Formation to the Aporthophyla–Paralenorthis Association and assigned the Lower Formation – except for the lowermost non-fossiliferous part – to the Darriwilian (Middle Ordovician) strata (Fig. 2). Harris et al. (Reference Harris, Repetski, Kauffman and Sando1987), Qiu (Reference Qiu1988) and Yu et al. (Reference Yu, Fang, Munnecke, Li, Li, Wang and Zhang2019) illustrated early middle Darriwilian (Middle Ordovician) conodonts from the Lower Formation.
The conformably overlying Upper Formation (pars = Jiaqu Formation of Lin & Qiu, Reference Lin and Qiu1982; Qiu, Reference Qiu1988; Fig. 2) is 97 m thick. The base of the Upper Formation is placed at the base of the reddish to grey crinoid packstone. The Upper Formation consists of marine, red, crinoid-rich limestone followed by an upper purplish-red, argillaceous limestone (Wang, Reference Wang1974) (Figs 3, 4). The uppermost Upper Formation yields nautiloids in abundance, crinoids and brachiopods; it is referred to the widespread Asian Sinoceras chinese Zone (Sandbian Stage; Upper Ordovician; Wang, Reference Wang1974; Chen, Reference Chen1975, Reference Chen1984) (Fig. 2). Qiu (Reference Qiu1988) reported uppermost Middle – lower Upper Ordovician conodonts from the Upper Limestone.
The c. 70-m-thick Hungshantou Formation overlies conformably the Chiatsun Group. The Hungshantou Formation is composed mainly of brown-grey shale and calcareous siltstone (Mu et al. Reference Mu, Wen, Wang, Zhang and Yin1973; Wang, Reference Wang1974). It is overlain by an extensive succession of Silurian sedimentary deposits. The Hungshantou Formation is referred to the Katian Amorphognathus superbus conodont Zone (Upper Ordovician; Qiu, Reference Qiu1988) and represents the highest Ordovician conodont yielding unit in the region.
4. Materials, methods and repository
Nine limestone and dolostone samples were collected from the Chiatsun Group in the Nyalam succession. Eight samples from 400 g to 1 kg in weight from the upper Lower Formation and one sample from the Upper Formation (Table 1) were processed for conodonts using the buffered standard techniques (Jeppsson & Anehus, Reference Jeppsson and Anehus1995). The residues were washed through sieves and split at 500–1000 µm, 250–500 µm and 63–250 µm followed by heavy liquid separation with a density of 2.84 g cm–3 (i.e. Jeppsson & Anehus, Reference Jeppsson and Anehus1999).
Table 1. Number of specimens in each sample from the Chiatsun Group, Nyalam

Different types of lithology were examined (i.e. dolostone, dolomitic limestone and limestone). Three out of the 12 processed samples collected from the succession yielded conodonts, and all with low to moderate yield (Table 1). The peritidal dolostone and dolomitic limestone of the Lower Formation of the Chiatsun Group were barren, whereas the bioturbated mudstone-wackestone yielded a low number of specimens. The red crinoid packstone sample of the lower Upper Formation of the Chiatsun Group produced a relatively abundant, characteristic and diverse conodont fauna.
The specimens were handpicked under the stereomicroscope. The preservation of the conodonts is poor to moderate and many specimens are fragile and unfortunately fragmented; some specimens also exhibit surficial alteration, which is indicated by recrystallized minerals in the scanning electron microscope (SEM). Such specimens appeared better preserved and were easier to identify under the light microscope. Despite this, and in general, almost all the conodont specimens have retained their original morphology and could be identified to genus and species level. All recovered conodont specimens are black to light grey with a colour alteration index (CAI) of 5 to 5.5, demonstrating that they and the host rocks have been heated (after deposition and lithification) to temperatures in excess of 360°C (Epstein et al. Reference Epstein, Epstein and Harris1977; Rejebian et al. Reference Rejebian, Harris and Huebner1987).
A total of 268 identifiable conodont elements, referable to 14 genera and 16 species, were recovered from the section. An additional three non-identified taxa are present. The conodonts and their sample-by-sample occurrence are listed in Table 1. The illustrated specimens were photographed using a SEM (FEI Quanta).
The illustrated specimens are housed at the collection of the State Key Laboratory of Palaeobiology and Stratigraphy, Nanjing Institute of Geology and Palaeontology, Chinese Academy of Sciences, Nanjing, China (NIGP) with the catalogue numbers NIGP 172256 – NIGP 172280. The remaining collection is housed in the micropalaeontological collection of the Natural History Museum of Denmark, University of Copenhagen, Copenhagen, Denmark.
5. Results
5.a. Conodonts: taxonomical comments
The taxa presented by Harris et al. (Reference Harris, Repetski, Kauffman and Sando1987) and Qiu (Reference Qiu1988) were described under older terminology, and re-identification of the illustrated Darriwilian specimens is briefly discussed here in terms of current multielement taxonomy. The emended identifications form the basis for the revised biozones at Nyalam (Section 5.b below; Fig. 2).
Amorphognathus variabilis Sergeeva, Reference Sergeeva1963
Qiu (Reference Qiu1988, pl. 6, fig. 15)
Here = Lenodus variabilis (Sergeeva, Reference Sergeeva1963).
Amorphognathus variabilis is referred to the multielement genus Lenodus Sergeeva (e.g. Stouge & Bagnoli, Reference Stouge and Bagnoli1990, Reference Stouge and Bagnoli1999; Bagnoli & Stouge, Reference Bagnoli and Stouge1997; Zhang, Reference Zhang1998a; Löfgren & Zhang, Reference Löfgren and Zhang2003; Stouge et al. Reference Stouge, Bagnoli and Rasmussen2020). The specimen figured by Qiu (Reference Qiu1988) and referred to Amorphognathus variabilis is not considered to represent this taxon. However, the illustrated specimen does not allow for any more precise identification and the taxon is not considered further.
Cordylodus horris (sic) Barnes & Poplawski, Reference Barnes and Poplawski1973
Qiu (Reference Qiu1988, pl. 4, fig. 19)
The correct species name was probably intended to be ‘horridus’ based on the original authors’ concept for the taxon. Nevertheless, the illustrated specimen more likely represents the M element of the multielement genus Erraticodon Dzik. Genus et species indet. A of this paper resembles that specimen.
Eoplacognathus jianyeensis An & Ding, Reference An and Ding1982
Qiu (Reference Qiu1988, pl. 4, figs 7–9)
Here = Yangtzeplacognathus jianyeensis (An & Ding, Reference An and Ding1982).
Qiu (Reference Qiu1988, fig. 7) is Yangtzeplacognathus jianyeensis, whereas Qiu (Reference Qiu1988, figs 8, 9) more likely represent Y. protoramosus (Chen et al. Reference Chen, Chen and Zhang1983) (but with a query).
Eoplacognathus foliaceus Fahræus, 1966 (sic)
Qiu (Reference Qiu1988, pl. 4, figs 10, 11)
Here = Yangtzeplacognathus foliaceus (Fåhræus, Reference Fåhræus1966), following Zhang (Reference Zhang1998a).
Eoplacognathus reclinatus (Fahræus, 1966) (sic)
Qiu (Reference Qiu1988, pl. 4, figs 13, 14)
Here = Yangtzeplacognathus spp.
Qiu (Reference Qiu1988) recorded several platform elements and assigned these to Eoplacognathus reclinatus. However, the illustrated platformal specimens represent Pa and Pb elements of the multielement genus Yangtzeplacognathus, and some of them are here referred to Y. protoramosus (Chen et al. Reference Chen, Chen and Zhang1983). The multielement genus Yangtezeplacognathus was not introduced until later (Zhang, Reference Zhang1998b).
Eoplacognathus robustus Bergström, Reference Bergström, Sweet and Bergström1971
Qiu (Reference Qiu1988, pl. 4, figs 4–6)
Here = Yangtzeplacognathus spp.
The illustrated specimens (Qiu, Reference Qiu1988, pl. 4, figs 4–6) are here referred to the genus Yangtzeplacognathus Zhang, Reference Zhang1998b. However, the species assignment is less certain. The specimen of Qiu (Reference Qiu1988, fig. 4) represents either Y. jianyeensis or Y. protoramosus. However, the specimens on Qiu (Reference Qiu1988, figs 5, 6) are here referred to Y. protoramosus.
Eoplacognathus suecicus Bergström, Reference Bergström, Sweet and Bergström1971
Qiu (Reference Qiu1988, pl. 3, figs 19, 20)
Here = Eoplacognathus suecicus Bergström, Reference Bergström, Sweet and Bergström1971.
The specimens are correctly identified by Qiu (Reference Qiu1988). The two illustrated elements of Eoplacognathus suecicus are right and left pastiniplanate elements sensu Zhang (Reference Zhang1998b) of that species. The stage of development points towards the Pygodus anitae Subzone of the Eoplacognathus suecicus Zone (Darriwilian, Middle Ordovician).
Eoplacognathus zgierzensis Dzik, Reference Dzik1976
Qiu (Reference Qiu1988, pl. 4, figs 15, 16)
Here = Yangtzeplacognathus sp.
Eoplacognathus zgierensis Dzik has a Lenodus type of apparatus, that is, a septimembrate apparatus (Stouge & Bagnoli Reference Stouge and Bagnoli1990, Reference Stouge and Bagnoli1999; Stouge et al. Reference Stouge, Bagnoli and Rasmussen2020). Löfgren & Zhang (Reference Löfgren and Zhang2003) placed E. zgierzensis in synonymy with Lenodus pseudoplanus. However, the illustrated specimens from Tibet (Qiu Reference Qiu1988, pl. 4, figs 15, 16) and referred to E. zgierzensis Dzik by Qiu (Reference Qiu1988), should probably be assigned to the genus Yangtzeplacognathus.
Histiodella serrata Harris, Reference Harris1962
Qiu (Reference Qiu1988; pl. 4, fig. 18)
Here = Histiodella sp. A Stouge et al. Reference Stouge, Du, Zhao, Gutierrez-Marco, Rábano and García-Bellido2011
The illustrated specimen (Qiu, Reference Qiu1988, pl. 4, fig. 18) is incomplete, missing the upper anterior part. The cusp, a significant character for species identification, is however preserved. The cusp is wide in lateral view (= H. holodentata character) and the anterior denticles are apparently relatively high (= Histiodella sp. A character), and the specimen is assigned to Histiodella sp. A.
The pectiniform P element of Histiodella sp. A is fully denticulated with fused, apically free and compressed denticles. The cusp is situated about one-third from the posterior margin of the element. The cusp is wide in lateral view; the apex of the cusp is almost equal in height to the tallest anterior denticles. Histiodella sp. A differs from H. holodentata by its higher anterior denticles. In H. holodentata the anterior denticles are lower than the apex of the cusp. H. sp. A differs from H. kristinae by its wider cusp, the anterior denticles are not taller than the apex of the cusp and the cusp is situated closer to the midlength in H. kristinae.
Histiodella sp. A represents the intermediate evolutionary stage between H. holodentata Ethington & Clark, Reference Ethington and Clark1981 and the advanced Histiodella kristinae Stouge, Reference Stouge1984 (see Stouge et al. Reference Stouge, Du, Zhao, Gutierrez-Marco, Rábano and García-Bellido2011; Stouge, Reference Stouge2012). Stouge (Reference Stouge1984, pl. 18, figs 4, 5, 7, 9–11) illustrated the complete apparatus of Histiodella sp. A.
Histiodella sp. A was first assigned to Histiodella tableheadensis (Stouge, Reference Stouge1984, pl. 18, fig. 7 only). Histiodella sp. A has been assigned to Histiodella holodentata (e.g. Du et al. Reference Du, Zhao, Huang, Tan, Wang, Yang, Zhang and Xiao2005, pl. 1, fig. 23; Chen et al. Reference Chen, Zhang, Bergström and Xu2006; Wang et al. 2013c, fig. 7I, J; Zhen et al. Reference Zhen, Wang, Zhang, Bergström, Percival and Cheng2011, fig. 14A, B) and more recently to Histiodella cf. H. holodentata (e.g. Feltes et al. Reference Feltes, Albanesi and Bergström2016, fig. 3C; Jing et al. Reference Jing, Zhou and Wang2016a; Serra et al. Reference Serra, Ortega, Feltes and Albanesi2017b, fig. 7B; Wu et al. Reference Wu, Stouge, Zhan, Liu and Liang2016, Reference Wu, Stouge, Zhang, Wang and Song2019) or Histiodella kristinae (e.g. Du et al. Reference Du, Zhao, Huang, Tan, Wang, Yang, Zhang and Xiao2005, pl. 1, figs 17, 18; Zhang, Reference Zhang1998a, pl. 9, fig. 17; Wang et al. 2013c, fig. 7F; Mestre & Heredia, 2016).
Species of Histiodella are widely distributed in Asia, including China (e.g. Zhang Reference Zhang1998a; Du et al. Reference Du, Zhao, Huang, Tan, Wang, Yang, Zhang and Xiao2005; Stouge et al. Reference Stouge, Du, Zhao, Gutierrez-Marco, Rábano and García-Bellido2011; Wang et al. 2013c; Jing et al. Reference Jing, Zhou and Wang2016a; Wu et al. Reference Wu, Stouge, Zhan, Liu and Liang2016, Reference Wu, Stouge, Zhang, Wang and Song2019). The species dealt with here and used for correlation comprise Histiodella holodentata Ethington & Clark, Histiodella sp. A Stouge et al., Histiodella kristinae Stouge and Histiodella bellburnensis Stouge, Reference Stouge1984. Except for Histiodella sp. A each of these Histiodella species is the zonal nominate species at zonal or subzonal levels in China, elsewhere in Asia, Argentina and Laurentia (e.g., Stouge Reference Stouge1984, Reference Stouge2012; Feltes et al. Reference Feltes, Albanesi, Bergström, Albanesi and Ortega2013, Reference Feltes, Albanesi and Bergström2016; Serra et al. Reference Serra, Albanesi, Ortega and Bergström2015, Reference Serra, Feltes, Henderson and Albanesi2017a, b, Reference Serra, Feltes, Albanesi and Goldman2019; Jing et al. Reference Jing, Zhou and Wang2016a; Wu et al. Reference Wu, Stouge, Zhan, Liu and Liang2016, Reference Wu, Stouge, Zhang, Wang and Song2019).
Wang et al. (2013c) formally introduced Histiodella wuhaiensis recorded from Inner Mongolia (margin of the North China Plate); however, this species is not well known. Jing et al. (Reference Jing, Zhou and Wang2016a) interpreted the species as the intermediate link between H. kristinae and H. bellburnensis as a result of its stratigraphical range in North China; however, it is equally possible that H. wuhaiensis is a member of a different lineage of Histiodella, which derived from H. sinuosa Graves & Ellison and includes, or is con-specific with, Histiodella labiosa Bauer, Reference Bauer2010 based on morphological similarities (= Histiodella sp. A Sweet et al. Reference Sweet, Ethington and Barnes1971; = Histiodella sp. 2 Harris et al. Reference Harris, Bergström, Ethington and Ross1979; cf. Bauer, Reference Bauer2010).
Naimanodus ani (Zhao et al. Reference Zhao, Huang, Du, Zhang, Xiao and Tan2005)
Cornuodus ani Zhao et al. Reference Zhao, Huang, Du, Zhang, Xiao and Tan2005
Namianodus degtyarevi Tolmacheva, Reference Tolmacheva2013
Here = Naimanodus ani (Zhao et al. Reference Zhao, Huang, Du, Zhang, Xiao and Tan2005)
The genus Naimanodus Tolmacheva, Reference Tolmacheva2013 is composed of elongated, striated coniform elements and recorded from Kazakhstan, Kyrgystan and Altai (Russia). Naimanodus degtyarevi Tolmacheva, Reference Tolmacheva2013 is the type species. However, Zhao et al. (Reference Zhao, Huang, Du, Zhang, Xiao and Tan2005) described a new species named Cornuodus ani from the Tarim Basin, which is here considered to be identical to N. degtyarevi Tolmacheva. The Nyalam specimens are therefore referred to Naimanodus ani (Zhao et al. Reference Zhao, Huang, Du, Zhang, Xiao and Tan2005).
Most specimens of the species recorded here are of the type described as ‘thin elongated with variably twisted base’ (Tolmacheva, Reference Tolmacheva2013, p. 186). One of the Tibet specimens referred to Panderodus cf. P. sulcatus (Fåhræus) by Harris et al. (Reference Harris, Repetski, Kauffman and Sando1987, pl. 2, fig. 2B) considered to be an elongate element of N. ani. Scolopodus cf. S. cornuformis Sergeeva of Harris et al. (Reference Harris, Repetski, Kauffman and Sando1987, pl. 2, fig. 2C) is broken, but based on the general outline of the illustrated specimen it probably represents an elongate coniform element of this species.
Pygodus serra (Hadding, Reference Hadding1913)
Here = Pygodus serra (Hadding, Reference Hadding1913).
The phylogeny of the genus is unresolved. Bergström (Reference Bergström, Sweet and Bergström1971, Reference Bergström1983) considered Pygodus serra the ancestor of P. anserinus. Zhang (Reference Zhang1998b) and Heredia et al. (Reference Heredia, Mestre, Soria and Kaufmann2017) proposed that there are two lineages of Pygodus, where P. serra Hadding evolved independently from P. anserinus and P. anserinus evolved from P. protoanserinus Zhang. Bergström (Reference Bergström2007a) instead argued that P. serra and P. protoanserinus are identical species. Wang & Qi (Reference Wang and Qi2001) described the new species Pygodus xinjiangensis from the Tarim basin. Wang et al. (Reference Wang, Zhen, Zhang and Wu2016) considered P. xinjiangensis the ancestor of P. serra and, at the same time, placed P. protoanserinus in (junior) synonymy with P. serra following Bergström (Reference Bergström, Sweet and Bergström1971, Reference Bergström1983, Reference Bergström2007a).
The Nyalam specimens at hand are morphologically identical to Pygodus protoanserinus (pars) sensu Zhang (Reference Zhang1998a, b; see also Pygodus serrus ambonis Xiong et al. Reference Xiong, Wu and Wang2015, pl. 2, figs 13, 14) rather than Pygodus serra (Hadding) sensu stricto, that is, the type specimen of the genus and species. Despite this, the traditional identification of P. serra is used here: the Pa element of Pygodus serra is characterized by having three rows of nodes on the platform, awaiting clarification of the phylogeny of the genus and a better understanding of the species.
Pygodus anserinus Lamont & Lindström, Reference Lamont and Lindström1957
pars = Pygodus protoanserinus Zhang (Reference Zhang1998b)
Here = The early type of Pygodus anserinus.
The Tibet specimens, here referred to P. anserinus, have three rows of nodes and an incipient to an incomplete fourth row of nodes on the platform. The incipient fourth row of node is developed distally on the traverse ridges on the platform connecting the sub-median ridge with the lateral margin.
The Pa elements of the later form of Pygodus anserinus are characterized by having a prominent fourth ridge with nodes crossing the platform, connecting to or almost reaching the cusp.
Qiu (Reference Qiu1988) referred one specimen to Pygodus anserinus. However, the illustrated specimen (Qiu, Reference Qiu1988, pl. 6, fig. 14) does not belong to the multielement genus Pygodus, but is better assigned to the multielement genus Baltoniodus Lindström, Reference Lindström, Sweet and Bergström1971; perhaps it should be referred to B. variabilis (Bergström, Reference Bergström1962), but the illustrated specimen is broken, prohibiting a secure identification.
Stolodus stola Lindström, Reference Lindström1955
Qiu (Reference Qiu1988, pl. 4, fig. 17)
Qiu (Reference Qiu1988, pl. 4, fig. 17) referred one specimen to Stolodus stola Lindström. Stolodus (Lindström) is a very characteristic Lower Ordovician monospecific genus that is restricted to the Oepikodus evae Zone (Floian Stage, Lower Ordovician). However, the illustrated specimen of Qiu cannot be positively identified from the photo.
5.b. Conodont zonation and correlation
The positions and ranges of the most significant taxa recorded in this study are shown in Figure 4, and most of the species mentioned in the text are illustrated in Figures 5 and 6.

Fig. 5. Conodonts recovered from the Lower Formation. Chiatsun Group, Nyalam County, southern Tibet. (a, b) Histiodella sp. A. (a) Fragment of the anterior part of P element; lateral view showing the height of the anterior denticles, sample T-4, NIGP 172256. (b) Lateral view of broken P element; identification is based on the width of the cusp and the height of the anterior denticles, sample T-4, NIGP 172257. (c, d, h) Ansella jemlandica (Löfgren, Reference Löfgren1978). (c) Planoconvex (S) element, lateral view, sample T-4, NIGP 172258. (d) Biconvex (P) element, lateral view, sample T-4, NIGP 172259. (h) Biconvex (P) element, lateral view, sample T-4, NIGP 172263. (e) Genus et species indet. A, ?M element, lateral view, sample T-11, NIGP 172260. (f, g, k) Pygodus serra (Hadding, Reference Hadding1913). (f) Pygodontiform (P) element, upper view, sample T-11, NIGP 172261. (g) Pygodontiform (P) element, upper view, sample T-11, NIGP 172262. (k) Haddingdontiform element, lateral view, sample T-11, NIGP 172266. (i, j, m) Drepanoistodus sp. (i, j) Drepanodontiform elements, lateral views, sample T-11, NIGP 172264, 172265. (m) Oistodontiform element, lateral view, sample T-11, NIGP 172268. (l) Cornuodus longibasis (Lindström, Reference Lindström1955), cornuodontiform element, lateral view, Sample T-11, NIGP 172267. (n) Genus et species indet. B, ?P element, lateral view, sample T-11, NIGP 172269. (o) Naimanodus ani (Zhao et al. Reference Zhao, Huang, Du, Zhang, Xiao and Tan2005). Symmetrical slender element, lateral view, sample T-11, NIGP 172270. Scale bars: 200 μm.
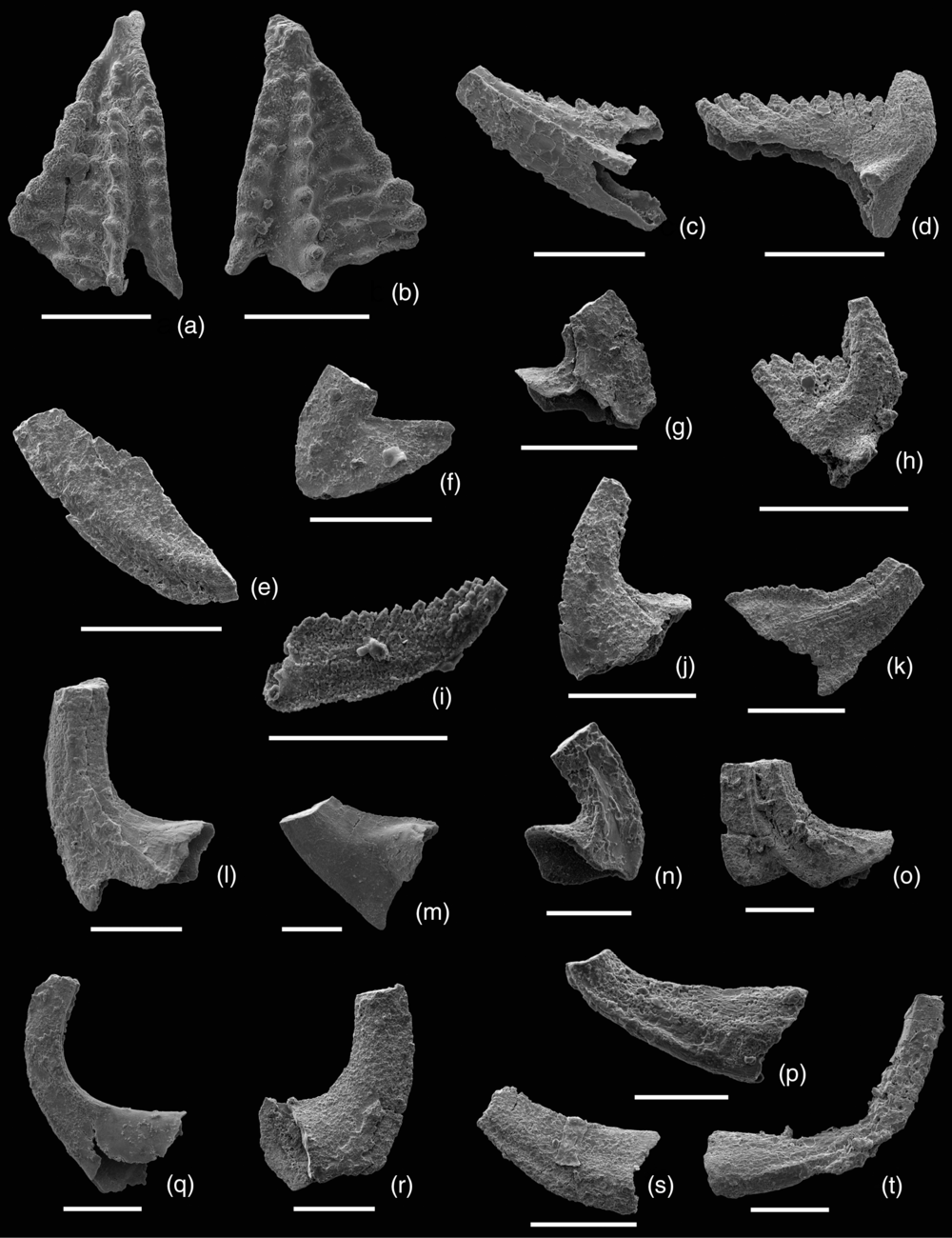
Fig. 6. Conodonts from the Upper Formation, Chiatsun Group, Nyalam County, Southern Tibet. (a–d, h) Pygodus anserinus Lamont & Lindström, Reference Lamont and Lindström1957. (a) Pygodontiform (P) element, upper view, sample T-12, NIGP 172271. (b) Pygodontiform (P) element, upper view, sample T-12, NIGP 172272. (c) Ramiform (Sb) element, outer lateral view, sample T-12, NIGP 172273. (d) Haddingodontiform element, Upper Formation, outer lateral view, sample T-12, NIGP 172274. (h) Haddingodontiform element, inner lateral view, sample T-12, NIGP 172278. (e, i) Ansella fenxiangensis (An et al. Reference An, Du, Gao, Chen and Li1981). (e) Biconvex (P) element, lateral view, sample T-12, NIGP 172275. (i) Planoconvex (S) element, inner view, sample T-12, NIGP 172279. (f, g) Oslodus semisymmetricus (Hamar, Reference Hamar1966). (f) Oistodontiform element, lateral view, sample T-12, NIGP 172276. (g) Acontiodontiform element, lateral view, sample T-12, NIGP 172277. (j, k) Dapsilodus viruensis (Fåhræus, Reference Fåhræus1966). (j) Acontiodontiform element, lateral view, sample T-12, NIGP 172280. (k) Drepanodontiform element, lateral view, sample T-12, NIGP 172281. (l–n) Protopanderodus cooperi (Sweet & Bergström, Reference Sweet and Bergström1962). (l) Symmetrical acontiodontiform element, lateral view, sample T-12, NIGP 172282. (m) Scandodiform element, inner lateral view, sample T-12, NIGP 172273. (n) Asymmetrical unicostate acontiodontiform element, inner lateral view, sample T-12, NIGP 172284. (o) Protopanderodus varicostatus (Sweet & Bergström, Reference Sweet and Bergström1962), symmetrical acontiodontiform element, lateral view, sample T-12, NIGP 172285. (p, s, t) Panderodus sp. (p) Inner lateral view of subsymmetric graciliform element, sample T-12, NIGP 172286. (s) Outer lateral view of compressiform element, sample T-12, NIGP 172289. (t) Inner lateral view of asymmetric graciliform element, sample T-12, NIGP 172290. (r) Drepandous santacrucensis Dzik, Reference Dzik1994. Drepanodontiform, inner lateral view, sample T-12, NIGP 172288. (q) Cornuodus longibasis (Lindström, Reference Lindström1955). Symmetrical cornuodontiform element, lateral view, sample T-12, NIGP 172287. Scale bars: 200 μm.
The new conodont information presented here is combined with data previously published by Harris et al. (Reference Harris, Repetski, Kauffman and Sando1987), Qiu (Reference Qiu1988), Harper et al. (Reference Harper, Zhan, Stemmerik, Liu, Donovan, Stouge, Gutiérrez-Marco, Rábano and García-Bellido2011) and Yu et al. (Reference Yu, Fang, Munnecke, Li, Li, Wang and Zhang2019). This permits a revision and a better precision of the Darriwilian conodont biozonation known from the area (Fig. 2). Some of the faunal units presented here should not be interpreted as formal biozones, but may prove to be of zonal value when more data are analysed and the precise taxonomy has been presented.
The Amorphognathus (here = Lenodus) variabilis Zone sensu Qiu (Reference Qiu1988) is not used due to the uncertain taxonomic status of the taxon from Southern Tibet (cf. Section 5.a. above). The precise stratigraphical information on the zonal boundaries is not available for all the zones, so the lower and upper boundaries of the biozones have not been precisely identified in the Nyalam succession.
The presented conodont zones are first of Australian provincial affinity (= North China Province of An, Reference An1981; Zhen & Percival, Reference Zhen and Percival2003; North American Midcontinent Province sensu lato) shifting to North Atlantic Province affinity (= South China Province of An, Reference An1981).
5.b.1. Histiodella holodentata Zone
The zone is here defined by the appearance of the nominate species. Additional species listed by Qiu (Reference Qiu1988) from this level include Paroistodus numarcuatus (Qiu Reference Qiu1988, pl. 6, figs 17, 18; = Drepanoistodus costatus (Abaimova) here), Drepanodus? gracilis (Branson and Mehl) (= Panderodus sp. here) and Juanognathus aff. J. variabilis Serpagli (not illustrated by Qiu Reference Qiu1988, but probably it represents Aurilobus leptosomatus An). Additional taxa mentioned by Qiu (Reference Qiu1988) include the form species Acodus? sp. and Drepanodus homocurvatus Lindström (? = Drepanoistodus sp.); these specimens were not illustrated and cannot be more precisely identified.
Yu et al. (Reference Yu, Fang, Munnecke, Li, Li, Wang and Zhang2019) illustrated Histiodella holodentata (i.e. Yu et al. fig. 4F, G, not fig. 4H = Histiodella wuhaiensis Wang & Qi; see Section 5.a) from this zone in the lower part of the Lower Formation. Additional taxa include Ansella jemtlandica (Löfgren), Baltoniodus sp., Drepanodus sp. (= Drepanoistodus costatus Abaimova), Loxodus sp., Panderodus gracilis, Panderodus sp. and Protopanderodus nogamii (here = Panderodus nogamii (Lee)).
5.b.2. Histiodella sp. A informal zone
This informal biozone is based on the presence of Histiodella sp. A (Fig. 5a, b) from the Lower Formation (this study), which succeeds H. holodentata (Stouge, Reference Stouge2012; Section 5.a above). Additional taxa present in this interval include Ansella jemtlandica Löfgren (Fig. 5c, d, h), Cornuodus longibasis (Fig. 5l) and Naimanodus ani (Fig. 5o). The top of the informal zone is at the appearance of Eoplacognathus suecicus (cf. Qiu, Reference Qiu1988).
Sample T-4 at c. 400 m above the base of the Lower Formation of the Chiatsun Group represents the zone (Fig. 4). Previously, Qiu (Reference Qiu1988) reported Histiodella serrata Harris (Qiu, Reference Qiu1988, pl. 4, fig. 18; here = Histiodella sp. A) from the Lower Formation and this occurrence is confirmed here.
5.b.3. Eoplacognathus suecicus Zone
The Eoplacognathus suecicus Zone is re-established because the nominate species has been reported from these strata (Qiu, Reference Qiu1988, pl. 3, figs 19, 20).
Initially, Qiu (Reference Qiu1988) introduced the zone as a subzone of the Pygodus serra Zone, but Eoplacognathus suecicus is currently a valid zone below the P. serra Zone. The base of the zone is marked by the appearance of Eoplacognathus suecicus and is recorded from the Lower Formation. As mentioned previously (Section 5.b), the evolutionary stage of the E. suecicus specimens illustrated by Qiu (Reference Qiu1988) suggests the Pygodus anitae Subzone.
5.b.4. Yangtzeplacognathus foliaceus Zone
The Y. foliaceus Subzone of the Pygodus serra Zone of Qiu (Reference Qiu1988) is probably present in the Nyalam succession based on the specimens illustrated by Qiu (Reference Qiu1988, pl. 4, figs 10, 11). Here it is elevated to the zonal level, but its precise position in the succession is unknown.
5.b.5. Pygodus serra Zone
The appearance of Pygodus serra (Fig. 5f, g, k) defines the base of the zone, and the top of the zone is concident with the appearance of Pygodus anserinus. Associated specimens from the Pygodus serra Zone include Ansella fenfangxiensis (An, Du, Gao, Chen & Kee), Cornuodus longibasis (Fig. 5l), Dapsilodus viruensis, Drepanoistodus sp. (Fig. 5i, j, m), Panderodus sp., Periodon aculeatus Hadding (cf. Qiu, Reference Qiu1988), Genus et species indet. A (Fig. 5e; ?Erraticodon sp.) and Genus et sp. indet. B (Fig. 5n; ?Triangulodus sp.).
The base of the zone is at c. 680 m or at 40 m below the top of the Lower Formation, which is at the first positive record of Pygodus serra in sample T-11 (Fig. 4).
5.b.6. Pygodus anserinus Zone
This zone is defined by the appearance of Pygodus anserinus (Fig. 6a–d, h). Associated taxa include Ansella fenxiengensis (Fig. 6e, i), Cornuodus longibasis (Fig. 6q), Dapsilodus viruensis (Fig. 6j, k), Decoriconus pesequus (Löfgren, Reference A1998), Oslodus semisymmetricus (Hamar) (Fig. 6f, g), Panderodus sp. (Fig. 6p–s), Protopanderodus cooperi (Sweet & Bergström) (Fig. 6l–m) and P. varicostatus (Sweet & Bergström) (Fig. 6o). Pygodus serra from below ranges into the zone. Additional but rare taxa include Drepanodus santacruxensis Dzik (Fig. 6r) and Drepanoistodus sp.
Pygodus anserinus is recorded from sample T-12 at 740 m, and was collected immediately above the base of the Upper Formation (Fig. 4).
The assemblage that Harris et al. (Reference Harris, Repetski, Kauffman and Sando1987) referred to the Pygodus serra Zone includes the same early variety of P. anserinus as found here (i.e. Harris et al. Reference Harris, Repetski, Kauffman and Sando1987, fig. 2N). Harris et al. (Reference Harris, Repetski, Kauffman and Sando1987) described the lithology of their sample, yielding conodonts, as a ‘red crinoid limestone’ collected from the Upper Formation. It is therefore likely that their sample was collected from the same level, or a closely similar level, to that in this study.
5.b.7. Younger Ordovician zones
The younger (Upper Ordovician) zones introduced by Qiu (Reference Qiu1988) for the Nyalam section, southern Tibet are retained here pending further revision (Fig. 2).
5.c. Correlation
The newly recorded conodonts and the revised conodont zonal succession from southern Tibet permit precise correlation with other Ordovician deposits of the lesser (Tethyan–Tibetian) Himalayas representing the north Indian passive margin of the Gondwana palaeocontinent and of several peri-Gondwana palaeoplates and/or terranes.
5.c.1. South Tibet
The lateral extension of the Ordovician strata at Nyalam includes the succession forming the peak of Mount Qomolangma (Mount Jolmo Lungma; Mount Everest) (Wager, Reference Wager and Ruttledge1934; Gansser, Reference Gansser1964; Odell, Reference Odell1967; Sakai et al. Reference Sakai, Sawada, Takigami, Orihashi, Danhara, Iwano, Kuwahara, Dong, Cai and Li2005; Corthouts et al. Reference Corthouts, Lageson and Shaw2015). The carbonate sedimentary rocks, c. 125–225 m thick, exposed at the peak of Mount Qomolangma are referred to the Ordovician Qomolangma Formation (Yin & Kuo, Reference Yin and Kuo1978a, b; Sakai et al. Reference Sakai, Sawada, Takigami, Orihashi, Danhara, Iwano, Kuwahara, Dong, Cai and Li2005; Myrow et al. Reference Myrow, Hughes, Searle, Fanning, Peng and Parcha2009; Corthouts et al. Reference Corthouts, Lageson and Shaw2015). Myrow et al. (Reference Myrow, Hughes, Searle, Fanning, Peng and Parcha2009) observed a 65-m-thick and prominent marker bed in the succession, which is comparable to the thrombolite marker bed in the Lower Formation at Nyalam (Myrow et al. Reference Myrow, Hughes, Searle, Fanning, Peng and Parcha2009).
The previously published information on the fossils is mainly based on grab samples collected from the summit of Mount Qomolangma and the fauna includes brachiopods, corals, crinoids, ostracodes, trilobites and fragments of conodonts (Gansser, Reference Gansser1964; Yin & Kuo, Reference Yin and Kuo1978a, b; Searle et al. Reference Searle, Simpson, Law, Parrish and Waters2003; Sakai et al. Reference Sakai, Sawada, Takigami, Orihashi, Danhara, Iwano, Kuwahara, Dong, Cai and Li2005; Jessup et al. Reference Jessup, Law, Searle, Hubbard, Law, Searle and Godin2006). The brachiopod and cephalopod fauna from the lower part of the Lower Formation, above the thrombolite bed in the Nyalam succession, is assigned to the Darriwilian (Middle Ordovician; Yin & Kuo, Reference Yin and Kuo1978a, b; Yin, Reference Yin1987; Harper et al. Reference Harper, Zhan, Stemmerik, Liu, Donovan, Stouge, Gutiérrez-Marco, Rábano and García-Bellido2011; Zhan et al. Reference Zhan, Harper, Jin, Liang, Liu, Stemmerik and Stouge2014) and therefore coeval and the lateral equivalent to the Lower Formation.
The carbonate strata preserved on the summit of Mount Qomolangma can probably be referred to the Histiodella holodentata Zone, perhaps extending into the H. sp. A informal zone of this study (lower Darriwilian; Middle Ordovician). The lower boundary of the Qomolangma strata is fault-bounded (Sakai, Reference Sakai1997; Carosi et al. Reference Carosi, Lombardo, Molli, Musumeci and Pertusati1998; Sakai et al. Reference Sakai, Sawada, Takigami, Orihashi, Danhara, Iwano, Kuwahara, Dong, Cai and Li2005; Corthouts et al. Reference Corthouts, Lageson and Shaw2015) and the younger Middle–Upper Ordovician carbonate succession exposed at Nyalam is probably not preserved on Mount Qomolangma as a result of structural repetitions within the succession (Sakai et al. Reference Sakai, Sawada, Takigami, Orihashi, Danhara, Iwano, Kuwahara, Dong, Cai and Li2005; Corthouts et al. Reference Corthouts, Lageson and Shaw2015).
Qiu (Reference Qiu1988) reported Yangtzeplacognathus foliaceus, Pygodus serra, P. anserinus and Yangtzeplacognathus sp. from the Chayu district (eastern Tibet) (Fig. 7). This faunal suite resembles that of the Chiatsun Group at Nyalam, and the mentioned occurrences are presumably from the area near and laterally equivalent to the Mount Qomolangma and the Lower Formation. Qiu (Reference Qiu1988) did not elaborate further on this locality.

Fig. 7. Correlation of the biozones of Tibet mentioned in the text.
5.c.2. North Tibet
Ordovician fossils from the Xianza County, North Tibet include brachiopods, bryozoans, cephalopods, graptolites, sponges and conodonts (Qiu, Reference Qiu1988; Qu et al. Reference Qu, Zhang, Zheng and Wang2002, Reference Qu, Wang, Zhang, Wang, Lü and Duan2004; Zhang et al. Reference Zhang, Qu and Zheng2003; Zheng, Reference Zheng2005; Fang et al. Reference Fang, Chen, Burrett, Wang, Qu, Zheng, Zhang, Zhang and Li2018). The region is part of the Lhasa Plate lying to the north of the Indus–Zsangpo Suture. The Middle–Upper Ordovician succession in the Xianza County comprises the Lhasai Formation overlain by the Keerduo and Gangmusang formations (Xia, Reference Xia1983). Qiu (Reference Qiu1988) reported Pygodus anserinus from the Keerduo Formation, which indicates correlation to the Upper Formation of the Nyalam succession (Fig. 7). Conodont taxa representing the Hamarodus brevirameus Zone are reported from the upper Keerduo Formation (Qiu, Reference Qiu1988), suggesting correlation with the upper part of the Upper Formation of the Nyalam succession (southern Tibet). The overlying Gangmusang Formation (Upper Ordovician) of the Xianza County (northern Tibet) has been referred to the Amorphognathus superbus Zone (Qiu, Reference Qiu1988), which suggests that the Gangmusang Formation (pars) is coeval with the lower Hungshantou Formation of the Nyalam region. Zheng (Reference Zheng2005) recorded top Ordovician conodonts from the base of the Dewukaxa Formation, Yungzhug area, North Tibet, which is younger than those known from the Hungshantou Formation in South Tibet.
5.c.3. South China
Conodont studies of Middle and Upper Ordovician strata have been undertaken during the past decades in many regions and covering many depositional environments in South China (e.g. An, Reference An1981, Reference An1987; An et al. Reference An, Du, Gao, Chen and Li1981, Reference An, Du and Gao1985; Ni & Li, Reference Ni, Li, Wang, Ni, Zeng, Xu, Zhou, Li, Xiang and Lai1987; Chen & Zhang, Reference Chen and Zhang1984, Reference Chen and Zhang1989; Chen et al. Reference Chen, Chen and Zhang1983). Newer information is provided by Wang & Bergström (Reference Wang, Bergström, Chen and Bergström1995, Reference Wang, Bergström and Serpagli1999), Wang et al. (Reference Wang, Bergström and Lane1996), Zhang (Reference Zhang1998a, b), Chen et al. (Reference Chen, Zhang, Bergström and Xu2006, Reference Chen, Bergström, Zhang, Goldman and Chen2011), Wu et al. (Reference Wu, Stouge, Zhan, Liu and Liang2016, Reference Wu, Stouge, Zhang, Wang and Song2019), Wang et al. (Reference Wang, Zhang, Wu, Zhen, Zhang and Li2017c, Reference Wang, Zhen, Bergström, Wu, Zhang and Ma2019) and summarized by Zhang et al. (Reference Zhang, Zhan, Zhen, Wang, Yuan, Fang, Ma and Zhang2019). The stratigraphic nomenclature of Zhan & Jin (Reference Zhan and Jin2007) and the formally defined conodont zonation by Zhang (Reference Zhang1998a) and Wang et al. (Reference Wang, Zhen, Bergström, Wu, Zhang and Ma2019) are used here as evidence for this correlation.
On the Yangtze platform Histiodella sp. A and Histiodella kristinae are present in the middle to upper part of the Kuniutan Formation (An, Reference An1987; Zhang, Reference Zhang1998a). Wu et al. (Reference Wu, Stouge, Zhan, Liu and Liang2016, Reference Wu, Stouge, Zhang, Wang and Song2019) recorded Histiodella sp. A (= Histiodella cf. holodentata in their paper; see Section 5.a) from the Microzarkodina hagetiana Subzone of the Dzikodus tableheadensis Zone. Histiodella kristinae is restricted to or ranges above the Microzarkodina ozarkodella Subzone of the Dzikodus tablepointensis Zone (Zhang, Reference Zhang1998a).
Eoplacognathus suecicus is recorded from the upper to uppermost Kuniutan Formation (Zhang, Reference Zhang1998a; Wang et al. Reference Wang, Bergström, Ma, Song and Zhang2015a, Reference Wang, Zhen, Ma and Zhang2018b) where it is succeeded by Yangtzeplacognathus foliaceus and Yangtzeplacognathus protoramosus. The two taxa are either considered subzonal index species of the Pygodus serra Zone or zonal index species in the South China conodont zonal scheme (Zhang Reference Zhang1998a; Wu et al. Reference Wu, Stouge, Zhan, Liu and Liang2016; Wang et al. Reference Wang, Zhang, Wu, Zhen, Zhang and Li2017c, Reference Wang, Zhen, Bergström, Wu, Zhang and Ma2019). Pygodus serra, that is, the nominate species of the North Atlantic Pygodus serra Zone, is recorded from the uppermost Kuniutan to the lowermost part of the Miaopo Formation, and commonly occurs together with Pygodus anserinus (e.g. An et al. Reference An, Du and Gao1985; An, Reference An1987; Ni & Li, Reference Ni, Li, Wang, Ni, Zeng, Xu, Zhou, Li, Xiang and Lai1987; Zhang, Reference Zhang1998a; Chen et al. Reference Chen, Bergström, Zhang, Goldman and Chen2011; Wu et al. Reference Wu, Stouge, Zhan, Liu and Liang2016; Wang et al. Reference Wang, Zhang, Wu, Zhen, Zhang and Li2017c, Reference Wang, Zhen, Bergström, Wu, Zhang and Ma2019).
The base of the succeeding Yangtzeplacognathus jianyeensis – Pygodus anserinus Zone of Zhang (Reference Zhang1998a) is only vaguely defined as the two taxa do not have coincident first appearance datum (FADs) and the base of the zone is either at the FAD of P. anserinus or at the FAD of Yangtzeplacognathus jianyeensis (Zhang, Reference Zhang1998a; Wu et al. Reference Wu, Stouge, Zhan, Liu and Liang2016; Wang et al. Reference Wang, Zhang, Wu, Zhen, Zhang and Li2017c, Reference Wang, Zhen, Ma and Zhang2018b, Reference Wang, Zhen, Bergström, Wu, Zhang and Ma2019). According to the available data, Yangtzeplacognathus jianyeensis appears first within the top Kuniutan Formation and ranges into the Miaopo Formation where it is followed by Pygodus anserinus. Here, the appearance of Pygodus anserinus, the index species of the Pygodus anserinus Zone, is used for the correlation (i.e. the same approach as in Chen et al. Reference Chen, Bergström, Zhang, Goldman and Chen2011). Pygodus anserinus is first recorded from the lower beds in the Miaopo Formation (Chen et al. Reference Chen, Bergström, Zhang, Goldman and Chen2011; Wang et al. Reference Wang, Bergström, Ma, Song and Zhang2015a, Reference Wang, Zhen, Ma and Zhang2018b; Wu et al. Reference Wu, Stouge, Zhan, Liu and Liang2016) and below the FAD of Nemagraptus gracilis (= the index of the N. gracilis Zone (Finney & Bergström, Reference Finney, Bergström, Hughes and Richards1986) and the base of the Sandbian (Bergström et al. Reference Bergström, Chen, Gutiérrez-Marco and Dronov2009)). Pygodus anserinus also occurs at the base of the largely coeval Datianba Formation that represents a carbonate succession, lateral to the shale and/or limestone succession of the Miaopo Formation (Ding et al. Reference Ding, Chen, Zhang, Cao, Bao and Wang1993; Zhang, Reference Zhang1998a; Chen et al. Reference Chen, Bergström, Zhang, Goldman and Chen2011; Bagnoli & Qi, Reference Bagnoli and Qi2014; Wang et al. Reference Wang, Li, Zhang and Kershaw2017a, Reference Wang, Zhen, Bergström, Wu, Zhang and Ma2019). The Baltoniodus variabilis Zone sensu Wang et al. (Reference Wang, Zhang, Wu, Zhen, Zhang and Li2017c) is recorded from the top of the Kuniutan Formation and the Miaopo Formation (Wang et al. Reference Wang, Bergström, Zhang, Zhen and Wu2015b, Reference Wang, Zhang, Wu, Zhen, Zhang and Li2017c; Song et al. Reference Song, Yu, Zhang, Sun, Muir and Liu2017). The Sandbian Baltoniodus alobatus Zone is present in the top part of the Miaopo Formation (Chen et al. Reference Chen, Bergström, Zhang, Goldman and Chen2011; Wang et al. Reference Wang, Zhen, Bergström, Wu, Zhang and Ma2019).
The strata on the Jiangnan slope are composed of shale with carbonate interbeds and are assigned to the Hulo and Yenwashan formations. Chen et al. (Reference Chen, Zhang, Bergström and Xu2006) distinguished the Yangtzeplacognathus crassus, Histiodella holodentata and H. kristinae zones from the Hulo Formation at the Huangnitang global boundary stratotype section and point (GSSP), Huangnitang. However, Jing et al. (Reference Jing, Zhou and Wang2016a) and Wu et al. (Reference Wu, Stouge, Zhang, Wang and Song2019) assigned the illustrated H. holodentata specimens of Chen et al. (Reference Chen, Zhang, Bergström and Xu2006) to H. cf. holodentata (= Histiodella sp. A here). Following this, the Histiodella sp. A informal zone correlates to the H. holodentata Zone of Chen et al. (Reference Chen, Zhang, Bergström and Xu2006) from the Huangnitang GSSP section. At the Hengdu section, Zhejiang province Wu et al. (Reference Wu, Stouge, Zhang, Wang and Song2019) recognized the Histiodella sp. A informal zone (= Histiodella cf. holodentata zone of Wu et al. Reference Wu, Stouge, Zhang, Wang and Song2019) and the H. kristinae Zone from the Hulo Formation, and Wang et al. (Reference Wang, Bergström, Zhang, Zhen and Wu2015b) assigned the basal bed of the overlying Yenwashan Formation to the Yangtzeplacognathus jianyeensis – Pygodus anserinus Zone.
The range of Histiodella sp. A in the lower and middle part of the D. tablepointensis Zone on the Yangtze Platform correlates directly with the Histiodella sp. A informal zone of the Lower Formation at Nyalam (Fig. 8). The Eoplacognathus suecicus, Y. foliaceous and P. serra zones of the upper Kuniutan and lowermost Miaopo formations in South China correlate with the Eoplacognathus suecicus, Y. foliaceus and the (pars) Pygodus serra zones of the Lower Formation in the succession at Nyalam (Fig. 8). The Pygodus anserinus Zone of southern Tibet largely correlates with the Yangtzeplacognathus jianyeensis – Pygodus anserinus Assemblage Zone of the Yangtze platform. The Pygodus anserinus Zone, recognized in the Datianba Formation in South China (Bagnoli & Qi, Reference Bagnoli and Qi2014) and in the Yenwashan Formation on the Jiangnan slope (Chen et al. Reference Chen, Zhang, Bergström and Xu2006; Zhen et al. Reference Zhen, Zhang and Percival2009; Wang et al. Reference Wang, Bergström, Zhang, Zhen and Wu2015b, Reference Wang, Zhen, Bergström, Wu, Zhang and Ma2019; Wu et al. Reference Wu, Stouge, Zhang, Wang and Song2019), represents the Sandbian Stage (Middle Ordovician) (Fig. 8).

Fig. 8. Correlation of conodont biozones from the Peri-Gondwana terranes mentioned in the text. The Baltoscandian zones are from Bergström & Ferretti (Reference Bergström and Ferretti2017) and Stouge et al. (Reference Stouge, Bagnoli and Rasmussen2020). The South China zones are from Zhang (Reference Zhang1998a) and Wang et al. (Reference Wang, Zhen, Bergström, Wu, Zhang and Ma2019). The zones of North China are from Jing et al. (Reference Jing, Zhou and Wang2016a, b) and Wang et al. (Reference Wang, Li, Zhang and Kershaw2017a). The Tarim Basin biozones are from Zhang et al. (Reference Zhang, Zhan, Zhen, Wang, Yuan, Fang, Ma and Zhang2019) and the Thailand–Malaysia zones are from Agematsu & Sashida (Reference Agematsu and Sashida2009). Potential gaps in the succession are marked.
It should be added that the succession from Eoplacognathus suecicus, Yangtzeplacognathus foliaceus, Y. protoramosus to Y. jianyeensis, which is characteristic of South China (cf. Chen et al. Reference Chen, Bergström, Zhang, Goldman and Chen2011), also seems to be preserved in southern Tibet (see Section 5.b above).
The Upper Ordovician succession of conodont zones from South China, that is, Baltoniodus variabilis, Baltoniodus alobatus (largely = Protopanderodus liripipus Zone of Qiu Reference Qiu1988; Fig. 8), Amorphognathus superbus and Hamarodus brevirameus, is identical to the conodont succession and zones recorded from the Upper Formation of the Chiatsun Group and the Hungshantou Formation, southern Tibet (e.g. Qiu, Reference Qiu1988). Correlation of the southern Tibet deposits with South China deposits is therefore evident for the upper Darriwilian, Sandbian and the lower part of the Katian strata (Upper Ordovician).
5.c.4. Tarim Basin
The Ordovician sedimentary rocks of the Tarim Basin are well displayed at the Yangjikan and Dawangou sections and other sections in the Kalpin–Bachu area. The strata in the Kalpin area are referred to the Lower–Middle Ordovician Qiulitag Group (Lower Qiulitag Group is composed of shallow-water carbonates, and the Upper Qiulitag Group reflects deeper-water carbonate environments), the Middle Ordovician Dawangou Formation (carbonates, upper slope deposition) and the Middle–Upper Ordovician Saergan Formation (deep-water graptolitic fine-clastic deposits), which is overlain by the Upper Ordovician Kanling, Qilang and Quilang formations. Several transgressive or regressive cycles have been recognized in the succession with a maximam transgressive peak at the Darriwilian–Sandbian transition (Saergan Formation).
The Middle–Upper Ordovician conodont fauna described from the important Yangjikan section and from the deeper-water Dawangou Formation includes the complete evolutionary lineage of the genus Histiodella (Wang & Zhou, Reference Wang and Zhou1998; Zhao et al. Reference Zhao, Zhang and Xiao2000, Reference Zhao, Zhao and Huang2006; Du et al. Reference Du, Zhao, Huang, Tan, Wang, Yang, Zhang and Xiao2005; Stouge et al. Reference Stouge, Du, Zhao, Gutierrez-Marco, Rábano and García-Bellido2011). The Histiodella holodentata Zone in the Tarim Basin (Du et al. Reference Du, Zhao, Huang, Tan, Wang, Yang, Zhang and Xiao2005) comprises the Histiodella holodentata Zone and Histiodella sp. A informal zone (Stouge et al. Reference Stouge, Du, Zhao, Gutierrez-Marco, Rábano and García-Bellido2011; Stouge, Reference Stouge2012; Jing et al. Reference Jing, Zhou and Wang2016a) and correlates with the Lower Formation of southern Tibet (Fig. 8). In addition, some of the specimens illustrated and assigned to Histiodella bellburnensis by Du et al. (Reference Du, Zhao, Huang, Tan, Wang, Yang, Zhang and Xiao2005) represent Histiodella wuhaiensis (cf. Jing et al. Reference Jing, Zhou and Wang2016a). It is possible that a hiatus occurs immediately above the H. bellburnensis Zone (e.g. Zhou et al. Reference Zhou, Chen, Wang, Wang, Li, Geng, Fang, Qiao, Zhang, Zhou and Chen1992; Fang et al. Reference Fang, Wu, Wang, Yang, Li and Zhang2019a). However, traces of the gap in the succession are not visible, and if present it is cryptic; the transition may well represent an interval of non-deposition or condensed deposition as the Eoplacognathus suecicus Zone is missing.
In the well-known Dawangou section, which serves as auxilary stratotype for the base of the Sandbian Stage and the Upper Ordovician Series (Bergström et al. Reference Bergström, Finney, Chen, Pålsson, Wang and Grahn2000; Hennissen et al. Reference Hennissen, Vandenbroucke, Chen, Tang and Verniers2010), the Yangtzeplacognathus crassus, Histiodella holodentata and Histiodella kristinae zones are recorded from the Dawangou Formation (Zhen et al. Reference Zhen, Wang, Zhang, Bergström, Percival and Cheng2011). However, the H. holodentata Zone of Zhen et al. (Reference Zhen, Wang, Zhang, Bergström, Percival and Cheng2011) from above the Y. crassus Zone is here considered to represent the Histiodella sp. A informal zone (following Jing et al. Reference Jing, Zhou and Wang2016a; see also Section 5.a). The index Histiodella kristinae occurs at a single horizon at the top of the Dawangou Formation and immediately below the base of the Saergan Formation. The overlying Saergan Formation is represented by black shale facies with low recovery of conodonts; mostly long-ranging taxa have been found, meaning that the precise extent of the conodont zones within the Saergan Formation remains uncertain. Pygodus anitae occurs on a bedding plane of the shale, within the lower Saergan Formation (Zhen et al. Reference Zhen, Wang, Zhang, Bergström, Percival and Cheng2011, p. 210; S. Leslie pers. comm. 2017). The taxon is used as subzonal index fossil of the Eoplacognathus suecicus Zone (e.g. Zhang Reference Zhang1998c; Zhang & Sturkell, Reference Zhang and Sturkell1998; Albanesi & Ortega, Reference Albanesi, Ortega and Montenari2016; Wang et al. Reference Wang, Zhen, Bergström, Wu, Zhang and Ma2019), which signifies that the lowermost part of the Saergan Formation should be assigned to the Eoplacognathus suecicus Zone. Pygodus serra and P. anserinus zones are recorded from the graptolitic Saergan Formation (Bergström & Finney, Reference Bergström and Finney1999; Chen et al. Reference Chen, Zhang, Li, Fan, Tang, Chen and Zhang2012, Reference Chen, Zhang, Wang, Goldman, Bergström, Fan, Finney, Chen, Chen, Zhang, Goldman, Bergström, Fan, Wang, Finney, Chen and Ma2015; Zhen et al. Reference Zhen, Wang, Zhang, Bergström, Percival and Cheng2011), whereas the Baltoniodus alobatus Zone is recorded from the overlying Kanling Formation at the top of the section. The base of the Pygodus anserinus Zone occurs below the FAD of Nemagraptus gracilis, that is, the taxon that defines the base of the Sandbian global Stage (Bergström & Finney, Reference Bergström and Finney1999; Bergström et al. Reference Bergström, Finney, Chen, Pålsson, Wang and Grahn2000; Zhao et al. Reference Zhao, Zhang and Xiao2000; Wang, Reference Wang2001; Chen et al. Reference Chen, Bergström, Zhang, Goldman and Chen2011, Reference Chen, Zhang, Wang, Goldman, Bergström, Fan, Finney, Chen, Chen, Zhang, Goldman, Bergström, Fan, Wang, Finney, Chen and Ma2015; Zhen et al. Reference Zhen, Wang, Zhang, Bergström, Percival and Cheng2011).
In the Bachu area the Ordovician strata are developed as shallow-water platform carbonates with an extensive Calathium-microbial reef complex in the Yijianfang Formation (Darriwilian; Zhang & Munnecke, Reference Zhang and Munnecke2016; Li et al. Reference Li, Li, Zhang and Munnecke2017; Wang et al. Reference Wang, Bergström, Song, Ma and Zhang2017b), which is overlain by the Tumuxiuke Formation. Conodonts of the Yijianfang Formation are clearly of North China affinity (Wang & Zhou, Reference Wang and Zhou1998; Xiong et al. Reference Xiong, Wu and Ye2006; Wang et al. Reference Wang, Zhen, Zhang and Wu2016, Reference Wang, Zhen, Bergström, Zhang and Wu2018a). The overlying Tumuxiuke Formation yields conodonts referred to the Sandbian (Upper Ordovician) comprising Yangtzeplacognathus jianyeensis, Baltoniodus variabilis and B. alobatus zones (Li et al. Reference Li, Huang, Wang, Wang, Zhang, Zhang, Fan and Zhang2009) and the overlying Liangliltaq Formation is referred to the Belodina confluens Zone (Katian) (Wang & Zhou, Reference Wang and Zhou1998; Wang & Qi, Reference Wang and Qi2001; Li et al. Reference Li, Huang, Wang, Wang, Zhang, Zhang, Fan and Zhang2009). The Middle Ordovician succession is incomplete to mostly missing (Wang et al. Reference Wang, Bergström, Song, Ma and Zhang2017b), whereas the Upper Ordovician strata contain conodonts of North China affinity (Jing et al. Reference Jing, Yang, Zhang, Zhang, Deng, Li, Fan and Percival2007; see also Xiong et al. Reference Xiong, Wu and Wang2015).
The deeper-water conodont zonation of the Tarim Basin correlates well with that of the Chiatsun Group and the overlying Hungshantou Formation, South Tibet (Fig. 8). The Histiodella holodentata Zone of the Yangjikan section and Histiodella sp. A informal zone of the Yangjikan and Dawangou sections correlates directly with the zone of the Lower Formation in southern Tibet. The Eoplacognathus suecicus (i.e. P. anitae Subzone) Zone and Pygodus serra Zone of the lower Saergan Formation at the Dawangou section can be correlated with the Lower Formation of southern Tibet. The lower part of the Pygodus anserinus Zone in the Dawangou section correlates precisely with the Pygodus anserinus Zone of the Upper Formation (Fig. 8).
Correlation with the higher zones from the Upper Formation and Hungshantou Formation of southern Tibet to the Yangjikan and Dawangou sections is fairly simple. Upsection in the Dawangou section, Yangtzeplacognathus jianyeensis occurs together with P. anserinus from the upper Saergan extending into the lower Kanling Formation. This common occurrence represents the Y. jianyeensis – P. anserinus Assemblage Zone as it is defined in South China (i.e. Zhang, Reference Zhang1998a). Y. jianyeensis appears together with Scabbardella altipes in the (upper) Pygodus anserinus Zone, and both taxa range into the overlying lower Kanlin Formation. The Y. jianyeensis Zone of Qui (Reference Qiu1988) therefore probably correlates with the Y. jianyeensis – P. anserinus Assemblage Zone of Zhang (Reference Zhang1998a) and with the upper Pygodus anserinus Zone from the Sandbian Stage (Upper Ordovician) (Fig. 8).
5.c.5. North China
The Ordovician succession of the North China Platform deposits is 500–1000 m thick and dominated by shallow-water carbonates. Marginal deposits on the North China Craton are thicker, up to 2600 m, and composed of mixed carbonates and siliciclastics that were deposited in slope to marginal platform environments. A significant gap corresponding to the Dapingian and lower Darriwilian strata is observed on the platform of North China and related to the so-called Huaiyuan uplift (Wang et al. Reference Wang, Zhen, Zhang and Wu2016; Zhen et al. Reference Zhen, Zhang, Wang and Percival2016).
The Darriwilian shallow-water conodont fauna of North China is dominated by conodont taxa that are characteristic of the carbonate platform of the North China Craton, which makes the precise relative age determination and international correlation of the fauna outside the palaeotropical zone difficult (An, Reference An1981; An et al. Reference An, Zhang, Xiang, Zhang, Xu, Zhang, Jiang, Yang, Lin, Cui and Yang1983; An & Zheng, Reference An and Zheng1990; Wang et al. Reference Wang, Bergström and Lane1996) (Fig. 8). Wang et al. (Reference Wang, Bergström, Zhen, Zhang, Wu and Chen2013b, c, Reference Wang, Zhen, Bergström, Zhang and Wu2018a) revised the conodont zonation based on the Zhaogezhuang section in Tanshan, Hebei Province, which represents the Middle Ordovician inner platform succession of North China. The Darriwilian (Middle Ordovician) carbonate succession on North China initiates with the appearance of Aurilobodus leptosomatus; additional taxa include Histiodella holodentata, Loxodus dissectus, Tangshanodus tangshanensis and Triangulodus changhanensis; this assemblage is assigned to the Histiodella holodentata – Tangshanodus tangshanensis Zone (e.g. Wang et al. Reference Wang, Zhen, Zhang and Wu2016, Reference Wang, Zhen, Bergström, Zhang and Wu2018a) (Fig. 8). The following zones are respectively Plectodina fragilis and Eoplacognathus suecicus – ‘Acontiodus’ linxiensis zones (An et al. Reference An, Zhang, Xiang, Zhang, Xu, Zhang, Jiang, Yang, Lin, Cui and Yang1983; An & Zheng, Reference An and Zheng1990). Associated species include Erraticodon tangshanensis and Triangulodus changsanensis extending into the zone from below and Plectodina onychodonta, a taxon that appears in the zone (Wang et al. Reference Wang, Zhen, Bergström, Zhang and Wu2018a, fig. 5). The top of the Darriwilian strata is referred to the Aurilobus serratus Zone followed by the Sandbian Scandodus handanensis Zone (An et al. Reference An, Zhang, Xiang, Zhang, Xu, Zhang, Jiang, Yang, Lin, Cui and Yang1983; An & Zheng, Reference An and Zheng1990).
The information on the shallow-water zones from the North China platform suggests that the Histiodella holodentata – Tangshanodus tangshanensis Zone correlates to the H. holodentata Zone of the Lower Formation, southern Tibet and the Eoplacognathus suecicus – ‘Acontiodus’ linxiensis Zone of the North China platform largely correlates with the Eoplacognathus suecicus Zone of the Lower Formation (Fig. 8). The following Scandodus hanndaensis Zone of North China has not been recognized from southern Tibet due to the absence of the index species. Likewise, the Plectodina fragilis and Aurilobus serratus zones have not been identified from southern Tibet.
In the deeper- to deep-water setting, the middle Darriwilian – lower Sandbian Ordovician strata that accumulated on the marginal platform and slope of the North China Plate are exposed in Inner Mongolia and North China. The Sandakan and Zhuozishan formations are exposed in the Laoshidan section, which is located on the western margin of the North China Craton. The faunal succession comprises Histiodella sp. A, Histiodella kristinae, Histiodella wuhaiensis and Histiodella bellburnensis. Jing et al. (Reference Jing, Zhou and Wang2016a) established three Histiodella-based biozones in the section, whereas Myrow et al. (Reference Myrow, Chen, Snyder, Leslie, Fike, Fanning, Yuan and Peng2015) identified the H. holodentata Zone from the Sandaokan Formation of the Subaiyingou section, which is about 30 km NE from the Laoshidan section. Unfortunately, the specimens from the Subaiyingou section are not well preserved, but their morphologies are closely similar to Histiodella sp. A (cf. Myrow et al. Reference Myrow, Chen, Snyder, Leslie, Fike, Fanning, Yuan and Peng2015, fig. 14A–C; see also Jing et al. Reference Jing, Zhou and Wang2016a, p. 53). The Histiodella holodentata Zone of Myrow et al. (Reference Myrow, Chen, Snyder, Leslie, Fike, Fanning, Yuan and Peng2015) is therefore referred to the younger Histiodella sp. A informal zone.
A similar succession is described from the condensed lower Klimoli Formation exposed at the adjecent Dashimen section (c. 12 km NW of the Laoshidan section) in the Inner Mongolia Autonomous Region (Wang et al. Reference Wang, Bergström, Zhen, Chen and Zhang2013a, c). The succession was assigned to the Histiodella kristinae Zone (Wang et al. Reference Wang, Bergström, Zhen, Chen and Zhang2013a, c); however, several of the illustrated specimens should be referred to Histiodella sp. A instead (cf. Jing et al. Reference Jing, Zhou and Wang2016a; Section 5.a above). The presence of Periodon marathonensis and Polonodus? newfoundlandensis in the same interval supports our proposed assignment to the Histiodella sp. A informal zone.
The Darriwilian conodonts are also documented from the Wuhai area of Inner Mongolia, North China (An et al. Reference An, Zhang, Xiang, Zhang, Xu, Zhang, Jiang, Yang, Lin, Cui and Yang1983; Wang et al. Reference Wang, Bergström, Zhen, Chen and Zhang2013a, b; Jing et al. Reference Jing, Zhou and Wang2016b), where Dzikodus tablepointensis, Eoplacognathus suecicus (subdivided into the Pygodus lunnensis and P. anitae subzones) and Pygodus serra (with Yangtzeplacognathus foliaceus Subzone) zones were recorded from the Klimoli Formation, and the Pygodus serra and Pygodus anserinus zones are identified in the overlying Wulalike Formation exposed at the Wolongang and the Hatuke Creek sections, respectively.
The Histiodella cf. holodentata zone of Jing et al. (Reference Jing, Zhou and Wang2016b) on the North China margin to slope deposits are directly correlated with the Histiodella sp. A informal zone of the Lower Formation in southern Tibet (Fig. 8). The overlying Pygodus serra Zone is associated with an assemblage that is similar to that recorded from southern Tibet, and thus correlates with the Pygodus serra Zone from the Lower Formation. The following Pygodus anserinus Zone from North China resembles the fauna from the Upper Formation. In North China, the Pygodus anserinus Zone is succeeded by Plectodina aculeata, a taxon that is not known from South Tibet. The younger Ordovician strata (Sandbian–Katian) from North China include genera and species that are not known from southern Tibet, and the Upper Ordovician correlation between the North China platform and the South Tibet succession is not precise.
5.c.6. Thailand and northern peninsular Malaysia
The Middle–Upper Ordovician conodont-bearing sedimentary rocks in Thailand and peninsular Malaysia were deposited on the Sibumasu peri-Gondwana Terrane (Metcalfe, Reference Metcalfe2013; summarized by Agematsu & Sashida, Reference Agematsu and Sashida2009). In northern, western and southern Thailand, the Ordovician platformal shallow-water limestone deposition prevailed and the rocks are referred to the Thung Song Group (Bunopas, Reference Bunopas1981, Reference Bunopas and Piancharoen1992; Agematsu et al. Reference Agematsu, Sashida, Salyapongse and Sardsud2006a, Reference Agematsu, Sashida, Salyapongse and Sardsud2007) or Thung Song Formation (Cocks et al. Reference Cocks, Fortey and Lee2005). The Middle and Upper Ordovician deposits on the Langkawi Islands, northern Malaysia are assigned to the Kki Bukit Formation, which is overlain by the Upper Ordovician – lower Silurian Tanjong Dendang Formation (Cocks et al. Reference Cocks, Fortey and Lee2005). The macro- and microfaunal succession changes in the Darriwilian strata and the conodont succession shows a clear shift from the Midcontinent type of fauna to the Atlantic type associated with a deepening trend upsection from the Darriwilian extending into the Upper Ordovician strata (Fortey & Cocks, Reference Fortey, Cocks, Hall and Holloway1998; Cocks & Fortey, Reference Cocks and Fortey2005; Agematsu & Sashida, Reference Agematsu and Sashida2009).
The Darriwilian shallow-water platformal deposits of the Thong Pha Phum area, west Thailand yield Aurilobodus leptosomatus and Histiodella holodentata (Agematsu et al. Reference Agematsu, Sashida, Salyapongse and Sardsud2006a, b). Plectodina onychodonta appears higher in the succession (Agematsu et al. Reference Agematsu, Sashida, Salyapongse and Sardsud2006a, b) and Panderodus nogamii is a common associated taxon (Agematsu et al. Reference Agematsu, Sashida, Salyapongse and Sardsud2006a, b, Reference Agematsu, Sashida and Ibraham2008). With the exception of Plectodina onychodonta, the conodont association recorded from the Histiodella holodenta Zone in Thailand is the same as for southern Tibet. Plectodina onychodonta is relatively long ranging, but the record of P. onychodonta occurring together with Pygodus lunnensis indicates its upper range into the lower Eoplacognathus suecicus Zone (Agematsu et al. Reference Agematsu, Sashida and Sardsud2013; cf. Jing et al. Reference Jing, Zhou and Wang2016b; Fig. 8).
The Thung Song Group strata in southern Thailand and the equivalent strata on Langkawi Island, northern Malaysia (Cocks et al. Reference Cocks, Fortey and Lee2005; Agematsu et al. Reference Agematsu, Sashida, Salyapongse and Sardsud2007) represent deeper- to deep-water depositional settings on the middle to outer shelf and slope (= Tarutao–Langkawi depositional basin of Agematsu & Sashida, Reference Agematsu and Sashida2009) and the conodont fauna is of temperate- to cool-water affinity (North Atlantic Province).
The Pygodus anserinus Zone is recorded from the Satun area of southern Thailand (Agematsu et al. Reference Agematsu, Sashida, Salyapongse and Sardsud2007); it is succeeded by the Baltoniodus (cf.) variabilis, B. alobatus and Hamarodus brevirameus zones. At the Langkawi section, northern Malaysia, the ‘Scolopodus’ striatus, Periodon sp. A, Baltoniodus alobatus and Hamarodus europaeus (= Hamarodus brevirameus in this study) zones are established (Agematsu et al. Reference Agematsu, Sashida and Ibraham2008). The Periodon specimens illustrated by Agematsu et al. (Reference Agematsu, Sashida and Ibraham2008), Periodon sp. A, probably represent the transition of Periodon marathonensis to Periodon zgierzensis. The Middle–Upper Ordovician conodont fauna and the succession from southern Thailand and Malaysia (Agematsu & Sashida, Reference Agematsu and Sashida2009) is largely similar to that in southern Tibet (Qiu, Reference Qiu1988), and correlation with the Upper Formation of the Chiatsun Group and the overlying Hungshantou Formation is relatively simple (Fig. 8).
6. Chronostratigraphy
The Histiodella holodentata (= H. tableheadensis Stouge, Reference Stouge1984), Histiodella kristinae and H. bellburnensis formal zones were first defined in the Table Head and Cow Head groups, Newfoundland, Canada (Stouge, Reference Stouge1984, Reference Stouge2012). Stouge et al. (Reference Stouge, Du, Zhao, Gutierrez-Marco, Rábano and García-Bellido2011) added the informal Histiodella sp. A as potential zonal index taxon to this scheme based on the sections in Tarim, China. Histiodella holodentata is the index species of the Histiodella holodentata Subzone and, together with Histiodella sp. A, are contained in the (pandemic) Periodon macrodentata evolutionary Zone. Histiodella kristinae and Histiodella bellburnensis are the index fossils for the two subzones of the younger Periodon zgierzensis Zone (Stouge, Reference Stouge2012; Feltes et al. Reference Feltes, Albanesi, Bergström, Albanesi and Ortega2013, Reference Feltes, Albanesi and Bergström2016; Jing et al. Reference Jing, Zhou and Wang2016a; Serra et al. Reference Serra, Albanesi, Ortega and Bergström2015, Reference Serra, Feltes, Henderson and Albanesi2017a, b, Reference Serra, Feltes, Albanesi and Goldman2019) (Fig. 9).

Fig. 9. Chronostratigraphy and stage slices are those of Bergström et al. (Reference Bergström, Chen, Gutiérrez-Marco and Dronov2009). Darriwilian graptolite zones are based on Zhang et al. (Reference Zhang, Zhan, Zhen, Wang, Yuan, Fang, Ma and Zhang2019); conodont zonation of South Tibet is from Qiu (Reference Qiu1988) and this study. The eustatic sea-level is from Haq & Schutter (Reference Haq and Schutter2008). The relative sea temperature is based on Trotter et al. (Reference Trotter, Williams, Barnes, Lécuyer and Nicoll2008) and Rasmussen et al. (Reference Rasmussen, Ullmann, Jakobsen, Lindskog, Hansen, Hansen, Eriksson, Dronov, Frei, Korte, Nielsen and Harper2016). The MDICE information is from Schmitz et al. (Reference Schmitz, Bergström and Wang2010), Zhang et al. (Reference Zhang, Shen and Algeo2010), Munnecke et al. (Reference Munnecke, Zhang, Liu and Cheng2011), Ma et al. (Reference Ma, Wang, Zhang, Song and Fang2015) and Zhang & Munnecke (Reference Zhang and Munnecke2016). The star indicates possible glaciation. For details see text.
The Histiodella holodentata Subzone of the Periodon macrodentatus evolutionary Zone correlates with the North American Levisograptus dentatus graptolite zone and Histiodella sp. A informal zone with the Holmograptus spinosus graptolite Zone (Maletz, Reference Maletz2009; Stouge, Reference Stouge2012; Albanesi & Ortega, Reference Albanesi, Ortega and Montenari2016; Serra et al. Reference Serra, Feltes, Henderson and Albanesi2017a, b, Reference Serra, Feltes, Albanesi and Goldman2019), which largely corresponds to the Nicholsonograptus fasciculatus graptolite Zone of South China (Wu et al. Reference Wu, Stouge, Zhang, Wang and Song2019; Zhang et al. Reference Zhang, Zhan, Zhen, Wang, Yuan, Fang, Ma and Zhang2019). The Histiodella kristinae and H. bellburnensis subzones of the Periodon zgierzensis Zone corresponds to the Nicholsonograptus fasciculatus graptolite Zone and pars the Pterograptus elegans graptolite Zone (Maletz, Reference Maletz2009; Stouge, Reference Stouge2012; Albanesi & Ortega, Reference Albanesi, Ortega and Montenari2016; Serra et al. Reference Serra, Feltes, Henderson and Albanesi2017a, b; Wu et al. Reference Wu, Stouge, Zhang, Wang and Song2019; Zhang et al. Reference Zhang, Zhan, Zhen, Wang, Yuan, Fang, Ma and Zhang2019). This is equivalent to Stage slice (Dw2) of Bergström et al. (Reference Bergström, Chen, Gutiérrez-Marco and Dronov2009) and suggests that the main part of the strata of the Lower Formation accumulated during middle Darriwilian time (Fig. 9).
The Eoplacognathus suecicus Zone (Bergström, Reference Bergström, Sweet and Bergström1971) is part of the cool- and deep-water Atlantic conodont zonation. Here it is largely referred to the Pterograptus elegans graptolite Zone, although the direct evidence is weak for this interval; the match is also hampered by the development of gaps or characterized by condensed deposition within successions on the Peri-Gondwana plates (cf. Schmitz et al. Reference Schmitz, Bergström and Wang2010; Chen et al. Reference Chen, Bergström, Zhang, Goldman and Chen2011; Ma et al. Reference Ma, Wang, Zhang, Song and Fang2015; Zhang & Munnecke, Reference Zhang and Munnecke2016).
The Pygodus serra Zone of the Atlantic conodont scheme was originally defined in the Kårgärde Limestone (Kårgärde quarry), Dalarna, Sweden (Bergström, Reference Bergström, Sweet and Bergström1971) and the zone is subdivided into four subzones (Bergström, Reference Bergström, Ebbestad, Wickström and Högström2007b). Pygodus serra is a pandemic species in coeval cold- and deep-water deposits and has been recorded from many localities worldwide (e.g. Bergström, Reference Bergström, Sweet and Bergström1971, Reference Bergström, Ebbestad, Wickström and Högström2007b). The Pygodus serra Zone corresponds to the Didymogratus murchisoni and Jiangxigraptus vagus (pars =‘Hustedograptus teretiusculus’) graptolite zones (Bergström, Reference Bergström, Sweet and Bergström1971, Reference Bergström2007a, b). In South China, the succession of the Yangtezeplacognathus species is well established and the presence of the same Yangtzeplacognathus species recorded from South Tibet (Qiu, Reference Qiu1988; Section 5.b above) suggests that the same faunal succession is preserved in the Chiatsun Group (Fig. 9). The base of the Pygodus serra Zone defines the base of Stage Slice Dw3 of the upper Darriwilian strata (Middle Ordovician; Bergström et al. Reference Bergström, Chen, Gutiérrez-Marco and Dronov2009) (Fig. 9).
The Pygodus anserinus Zone, first introduced by Bergström (Reference Bergström, Sweet and Bergström1971) from the Furudal Limestone (Fjäcka locality), Dalarna, Sweden, is currently subdivided into two bio-subzones (i.e. Sagittodontina kielcensis Subzone and Amorphognathus inaequalis Subzone; cf. Bergström & Ferretti, Reference Bergström and Ferretti2017), where the lower part of the zone is partly characterized by the overlapping ranges of Pygodus serra and Pygodus anserinus (Bergström, Reference Bergström, Sweet and Bergström1971, Reference Bergström, Ebbestad, Wickström and Högström2007b). The Pygodus anserinus Zone correlates with the upper part of the Hustedograptus teretiusculus graptolite Zone and the lower part of the Nemagraptus gracilis graptolite Zone (stage slice Dw3 to upper Darriwilian to Stage slice Sa1, lower Sandbian; Bergström, Reference Bergström, Sweet and Bergström1971, Reference Bergström2007a, b; Bergström et al. Reference Bergström, Chen, Gutiérrez-Marco and Dronov2009).
This described correlation indicates that the deposition of the carbonates of the Lower Formation of the Chiatsun Group occurred during middle Darriwilian time (Dw2), whereas the deeper-water sedimentation of the Upper Formation of the Chiatun Group started during late Darriwilian time (Dw3; Fig. 9).
7. Palaeogeographic, palaeoceanographic and palaeoclimatic implications
7.a. Palaeogeography
A large amount of research on the Ordovician palaeogeography of the eastern Peri-Gondwana terranes has been published over the last couple of decades (e.g. Burrett et al. Reference Burrett, Long, Stait, McKerrow and Scotese1990, Reference Burrett, Zaw, Meffre, Lai, Khositanont, Chaodumrong, Udclachon, Ekins and Halpin2014; Metcalfe, Reference Metcalfe, Hall and Holloway1998, Reference Metcalfe and Weis2001, Reference Metcalfe2013; Huang et al. Reference Huang, Zhu, Otofuji and Yang2000; Li & Powell, Reference Li and Powell2001; Cocks & Torsvik, Reference Cocks and Torsvik2013; Torsvik & Cocks, Reference Torsvik and Cocks2013, Reference Torsvik and Cocks2016; Zhen et al. Reference Zhen, Zhang, Wang and Percival2016; Fang et al. Reference Fang, Chen, Burrett, Wang, Qu, Zheng, Zhang, Zhang and Li2018, Reference Fang, Burrett, Li, Zhang, Zhang, Chen and Wu2019b; Yu et al. Reference Yu, Fang, Munnecke, Li, Li, Wang and Zhang2019). Although there are some disagreements, most palaeogeographic reconstructions suggest that, during Middle Ordovician time, the Tarim Basin and the North China Plate were situated at low latitudes and largely confined to the tropical zone. South China was situated at c. 30° south, some distance from Gondwana (Torsvik & Cocks, Reference Torsvik and Cocks2016), the elongated Sibumasu Terrane occupied a low-latitudinal position (Agematsu & Sashida, Reference Agematsu and Sashida2009) with NW Thailand in close proximity to Australia, and the southern Thailand–Malaysia region was closer to the Indian margin. The India platform, with South Tibet, extended from c. 25° to c. 35° south during Darriwilian time (e.g. Zhu et al. Reference Zhu, Zhuang, Zhou, Pan and Feng2006; Torsvik & Cocks, Reference Torsvik and Cocks2016).
7.b. Geochemistry
The important positive middle Darriwilian isotope carbon excursion (MDICE) represents the most clearly defined event in the δ13Ccarb profile of the Middle Ordovician (middle Darriwilian, Dw2) (Bergström et al. Reference Bergström, Chen, Gutiérrez-Marco and Dronov2009; Ainsaar et al. Reference Ainsaar, Kaljo, Martma, Meidla, Männik, Nolvak and Tinn2010; Calner et al. Reference Calner, Lehnert, Wu, Dahlqvist and Joachimski2014), and may support our biostratigraphy-based correlation. In South China (Schmitz et al. Reference Schmitz, Bergström and Wang2010; Zhang et al. Reference Zhang, Shen and Algeo2010; Munnecke et al. Reference Munnecke, Zhang, Liu and Cheng2011; Ma et al. Reference Ma, Wang, Zhang, Song and Fang2015), the rapidly rising limb culmination in the peak of the MDICE is recorded from the upper Dzikodus tableheadensis Zone, that is, starting at the Microzarkodina ozarkodella Subzone. The Eoplacognathus suecicus Zone represents the turnover in the MDICE and the Y. foliaceus and Y. protoramosus zones or subzones of the Pygodus serra Zone are recorded from the gradually falling limb. In the Tamir Basin, the rising limb (Liu et al. Reference Liu, Li, Wang, Liu, Luo and Shao2016a, b; Zhang & Munnecke, Reference Zhang and Munnecke2016; Fang et al. Reference Fang, Wu, Wang, Yang, Li and Zhang2019a) matches the Histiodella kristinae and H. bellburnensis zones and the falling limb was initiated within the upper E. suecicus Zone (Fig. 9). At some places in both South China and the Tamir Basin the curve is incomplete, perhaps due to a hiatus (Schmitz et al. Reference Schmitz, Bergström and Wang2010; Chen et al. Reference Chen, Bergström, Zhang, Goldman and Chen2011; Fang et al. Reference Fang, Wu, Wang, Yang, Li and Zhang2019a) or condensed deposition (Ma et al. Reference Ma, Wang, Zhang, Song and Fang2015).
7.c. Palaeoclimate and sea-level changes
The Darriwilian Age represents a transitional interval from a late Early Ordovician Greenhouse world to colder conditions, part of the long-term global cooling trend and expansion of the Gondwanan icesheets that preceded the global Hirnantian (Late Ordovician) Ice Age (Trotter et al. Reference Trotter, Williams, Barnes, Lécuyer and Nicoll2008; Finnegan et al. Reference Finnegan, Bergmann, Eiler, Jones, Fike, Eisenman, Hughes, Tripati and Fisher2011; Turner et al. Reference Turner, Armstrong, Wilson and Makhlouf2012; Young et al. Reference Young, Saltzman, Foland, Linder and Kump2009, Reference Young, Saltzman, Ausich, Desrochers and Kaljo2010). The long-term global cooling of the Ordovician sea-surface temperature culminated in the oceanic cooling associated with the Hirnantian glaciation (Trotter et al. Reference Trotter, Williams, Barnes, Lécuyer and Nicoll2008).
The long-term and progressive cooling was punctuated by short-term glaciations and warming pulses commencing in the Dapingian Age and continuing into the Darriwilian Age (Middle Ordovician) (e.g. Trotter et al. Reference Trotter, Williams, Barnes, Lécuyer and Nicoll2008; Cherns & Wheeley, Reference Cherns, Wheeley and Bassett2009; Zhang et al. Reference Zhang, Shen and Algeo2010; Turner et al. Reference Turner, Armstrong, Wilson and Makhlouf2012; Cherns et al. Reference Cherns, Wheeley, Popov, Ghobadi Pour, Owens and Hemsley2013; Pohl et al. Reference Pohl, Donnadieu, Le Hir, Ledant, Dumas, Alvarez-Solas and Vandenbroucke2016; Rasmussen et al. Reference Rasmussen, Ullmann, Jakobsen, Lindskog, Hansen, Hansen, Eriksson, Dronov, Frei, Korte, Nielsen and Harper2016; Young et al. Reference Young, Gill, Edwards, Salzman and Leslie2016; Fang et al. Reference Fang, Wu, Wang, Yang, Li and Zhang2019a; Albanesi et al. Reference Albanesi, Barnes, Trotter, Williams and Bergström2020). The short-term pulses promoted change from a relatively warm-water to a cool-water depositional environment on the shelf margin and slope, and promoted condensed deposition on the shelf margin and slope (Haq & Schutter, Reference Haq and Schutter2008). The early (Dw1–2) Darriwilian short-term cooling is thought by some to have triggered increased marine productivity and promoted the start of the Great Ordovician Biodiversification Event (GOBE; e.g. Webby et al. Reference Webby, Paris, Droser and Percival2004; Young et al. Reference Young, Saltzman, Foland, Linder and Kump2009, Reference Young, Saltzman, Ausich, Desrochers and Kaljo2010, Reference Young, Gill, Edwards, Salzman and Leslie2016; Rasmussen et al. Reference Rasmussen, Ullmann, Jakobsen, Lindskog, Hansen, Hansen, Eriksson, Dronov, Frei, Korte, Nielsen and Harper2016; Edwards et al. Reference Edwards, Saltzman, Royer and Fike2017). This short cooling pulse was succeeded by the middle Darriwilian warm ‘interglacial’ period, and was associated with relatively warm water and a sea-level rise (Dw2; Trotter et al. Reference Trotter, Williams, Barnes, Lécuyer and Nicoll2008; Turner et al. Reference Turner, Armstrong, Wilson and Makhlouf2012; i.e. the Tableheadian transgression of Stouge et al. Reference Stouge, Bagnoli and Rasmussen2020). The younger middle–late Darriwilian (late Dw2) short-term cooling is manifest in the global MDICE event (Cherns & Wheeley, Reference Cherns, Wheeley and Bassett2009; Cherns et al. Reference Cherns, Wheeley, Popov, Ghobadi Pour, Owens and Hemsley2013; Fang et al. Reference Fang, Wu, Wang, Yang, Li and Zhang2019a). It caused a lowering of sea-surface temperatures, intensifying the cool oceanic circulation and upwelling along continental margins (Haq & Schutter, Reference Haq and Schutter2008). The depositional environment changed from warm water to cool water with condensed deposition in several regions (cf. Lindström et al. Reference Lindström, Chen and Zhang1991; Ma et al. Reference Ma, Wang, Zhang, Song and Fang2015 for South China), which had a significant effect on the deeper-water conodont faunal assemblages of the Peri-Gondwana palaeoplates.
7.d. Conodont provinces
The Middle Ordovician conodont faunas from the Peri-Gondwana terranes have their own provincial character. An (Reference An1981) distinguished the North China conodont fauna from the South China conodont fauna based on the faunas from the two terranes. These faunas show similarities to the North American Midcontinent Province (includes the so-called Australian/Australasian Province) and the North Atlantic Province, respectively. It has long been recognized that the North Atlantic conodont province was associated with temperate to cool water, and the North American Midcontinent conodont province comprised warm shallow-water taxa (e.g. Lindström, Reference Lindström and Bruton1984; Bergström, Reference Bergström, McKerrow and Scotese1990; Bagnoli & Stouge, Reference Bagnoli and Stouge1991; for a detailed review see Zhen & Percival, Reference Zhen and Percival2003).
During the Darriwilian Age the North China and South China faunas (provinces) were distinct and well developed, but the conodont fauna assemblages on several of the Peri-Gondwana terranes changed during middle–late Darriwilian time. The middle Darriwilian shallow to warm-water taxa Aurilobus leptosomatus, Loxodus dissectus and Plectodina onychodonta, occurring with Histiodella holodentata, are recorded from the North China platform (An et al. Reference An, Zhang, Xiang, Zhang, Xu, Zhang, Jiang, Yang, Lin, Cui and Yang1983; Wang et al. Reference Wang, Zhen, Zhang and Wu2016), Tarim Basin (Zhao et al. Reference Zhao, Zhang and Xiao2000; Du et al. Reference Du, Zhao, Huang, Tan, Wang, Yang, Zhang and Xiao2005; Wang et al. Reference Wang, Qi and Bergström2007), Sibumasu Terrane (i.e. western Thailand; Agematsu et al. Reference Agematsu, Sashida, Salyapongse and Sardsud2006a, b, Reference Agematsu, Sashida and Ibraham2008; Agematsu & Sashida, Reference Agematsu and Sashida2009) and the Indian margin (southern Tibet; Qiu, Reference Qiu1988; Yu et al. Reference Yu, Fang, Munnecke, Li, Li, Wang and Zhang2019). This faunal assemblage clearly resembles that of Australia (Webby et al. Reference Webby, Percival, Edgecombe, Cooper, Vandenberg, Pickett, Pojeta, Playford, Winchester-Seeto, Young, Zhen, Nicoll, Ross and Schallreuter2000; Zhen & Percival, Reference Zhen and Percival2003) and was a low-latitude warm shallow-water platformal conodont assemblage that occupied a nearly equatorial position during Middle Ordovician time, similar to the macrofauna (Fortey & Cocks, Reference Fortey, Cocks, Hall and Holloway1998; Agematsu & Sashida, Reference Agematsu and Sashida2009; Cocks & Torsvik, Reference Cocks and Torsvik2013; Burrett et al. Reference Burrett, Zaw, Meffre, Lai, Khositanont, Chaodumrong, Udclachon, Ekins and Halpin2014; Torsvik & Cocks, Reference Torsvik and Cocks2016; Fang et al. Reference Fang, Chen, Burrett, Wang, Qu, Zheng, Zhang, Zhang and Li2018, Reference Fang, Burrett, Li, Zhang, Zhang, Chen and Wu2019b; Yu et al. Reference Yu, Fang, Munnecke, Li, Li, Wang and Zhang2019; this study).
During the middle–late Darriwilian time, the South China platform hosted many species of conodont genera, including Baltoniodus, Dzikodus, Eoplacognathus, Histiodella, Lenodus, Microzarkodina, Periodon, Pygodus and Yangtzeplacognathus, an assemblage of mixed provincial affinity, that is, representatives of the temperate- to cool-water North Atlantic Province and the low-latitude warm-water Midcontinent Province. This assemblage was succeeded by the Late Ordovician temperate- to cool-water genus Amorphognathus with several species and the genus Hamarodus. Likewise, the middle–late Middle Ordovician conodont faunal association and succession of South Thailand – northern Malaysia (Agematsu et al. Reference Agematsu, Sashida, Salyapongse and Sardsud2007; Agematsu & Sashida, Reference Agematsu and Sashida2009) and at Nyalam, South Tibet, were of similar mixed provincial affinity. Notably, the conodont succession for some of these terranes, starting with Histiodella holodentata, followed by Histiodella sp. A and associated with Dzikodus and Microzarkodina species, and succeeded by the appearance of Eoplacognathus suecicus, Yangtzeplacognathus spp., Pygodus spp., Hamarodus and finally Amorphognathus, is largely similar. In contrast, the shallow-water conodont fauna from the North China platform and partly the Tarim Basin were remarkly different and characteristic of the Australian conodont faunas (province).
7.e. Synthesis
The short-term late Dw2 glacial event coincided with a lowering of sea-surface temperatures and increased glaciation leading to intensified oceanic circulation and upwelling at continental margins. In this scenario, it is possible to relate the palaeobiogeographical change of the conodont fauna and ranges of the taxa and distribution of bioprovinces to faunal migrations and extinctions of some taxa in the Peri-Gondwana region.
During middle Darriwilian time (Dw 2) the climatic and global oceanic conditions changed to relatively warm milieux together with a rise in sea level – the Tableheadian transgression – and provided favourable conditions permitting the spread of the warm-water conodont fauna from tropical areas into higher-latitude (subtropical) regions. The warm-water taxa including species of Histiodella and Dzikodus migrated to South China and southern Tibet, but not all species in these lineages were present. For example, in South China the Histiodella lineage was incompletely represented and H. holodentata and H. bellburnensis are not recorded; the lineage is represented by Histiodella sp. A and H. kristinae. The lineage is even less complete on the Sibumasu Terrane. The succeeding global cooling and short-term glacial event (e.g. Trotter et al. Reference Trotter, Williams, Barnes, Lécuyer and Nicoll2008; Cherns et al. Reference Cherns, Wheeley, Popov, Ghobadi Pour, Owens and Hemsley2013; Albanesi et al. Reference Albanesi, Barnes, Trotter, Williams and Bergström2020; Fang et al. Reference Fang, Wu, Wang, Yang, Li and Zhang2019a) in the late Dw2 forced warm-water taxa to retreat from the South China Plate (at higher southern latitudes) and probably also from South Tibet to the tropical North China and Tarim terranes, where Histiodella bellburnensis (the last representative of the lineage; Du et al. Reference Du, Zhao, Huang, Tan, Wang, Yang, Zhang and Xiao2005; Stouge et al. Reference Stouge, Du, Zhao, Gutierrez-Marco, Rábano and García-Bellido2011; Jing et al. Reference Jing, Zhou and Wang2016a) persisted.
The change in the conodont fauna from the top of middle Darriwilian strata (Dw2) and across the MDICE into upper Darriwilian strata (Dw3) is significant. The new fauna that appeared along with the subsequent cooler-water regressive–transgressive transition heralded the appearance of the significant new cool-water genera Eoplacognathus, Oslodus, Sagittodontina and Yangtzeplacognathus, and later Pygodus. The relative warm-water genera Dzikodus and Histiodella and the temperate genera Lenodus and Microzarkodina, which were all characteristic of the Dw1 and Dw2 conodont fauna in South China, evolved either into another genus (Dzikodus to Pygodus), disappeared or became extinct (e.g. Histiodella, Microzarkodina). Other genera such as the temperate genera Ansella, Baltoniodus and the pandemic Periodon, and most of the simple cone genera (i.e. Dapsilodus, Drepanodus, Drepanoistodus and Protopanderodus) persisted, but all the genera changed species (e.g. Ansella jemtlandica to Ansella fenfangxiensis, Baltoniodus medius to B. prevariabilis and Periodon zgirenzensis to Periodon aculeatus).
The evidence for this significant faunal turnover of the conodont taxa is principally from South Tibet, South China and south Thailand – north Malaysia, whereas the shallow-water faunal assemblages on the North China and the Tarim platforms were less severely affected by climate change. At these places some cool-water taxa did appear on the platform (e.g. Eoplacognathus suecicus) during the highstand of the warm-water transgression, but the Australian conodont provincial aspect remained intact into Late Ordovician time on these terranes.
8. Conclusions
Carbonate samples collected from strata exposed in the Nyalam section, southern Tibet produced a low-yielding conodont fauna, which provides new significant information. The new data, together with light revision of specimens illustrated from previous publications (Harris et al. Reference Harris, Repetski, Kauffman and Sando1987; Qiu, Reference Qiu1988), permitted an update of the previous Middle–Upper Ordovician conodont zonation of southern Tibet, and constrains the age of the Lower and lower Upper formations of the Chiatsun Group to middle and late Darriwilian time (Dw2–3; Middle Ordovician), respectively. The southern Tibet conodont succession of Middle and Late Ordovician age is representative of the Indian continental carbonate platform, but may differ in detail from lateral equivalent successions.
In terms of global stratigraphy, the newly investigated conodont material is confidently referred to the Histiodella holodentata Subzone and Histiodella sp. A informal subzone of the global Periodon macrodentata evolutionary Zone, the North Atlantic Eoplacognathus suecicus, Yangtzeplacognathus foliaceus and Pygodus serra zones and the lower Pygodus anserinus Zone. The lower Pygodus anserinus Zone is defined in the lower Upper Formation and represents the top of the Darriwilian Stage i.e. the interval immediately below the FAD of Nemagraptus gracilis, the index for the Upper Ordovician Series (Sandbian Stage). This relative dating using conodonts confirms, but also constrains, the previous broad correlations based on brachiopod and cephalopod assemblages, for example (e.g. Wang, Reference Wang1974; Zhan et al. Reference Zhan, Harper, Jin, Liang, Liu, Stemmerik and Stouge2014; Fang et al. Reference Fang, Chen, Burrett, Wang, Qu, Zheng, Zhang, Zhang and Li2018, Reference Fang, Burrett, Li, Zhang, Zhang, Chen and Wu2019b).
The carbonate rocks of the Lower Formation of the Chiatsun Group in the Nyalam region, southern Tibet are the lateral equivalent platform deposits of those exposed on the summit of Mount Qomolangma (Mount Everest), Eastern Tibet (Yin & Kuo, Reference Yin and Kuo1978a, b; Sakai et al. Reference Sakai, Sawada, Takigami, Orihashi, Danhara, Iwano, Kuwahara, Dong, Cai and Li2005; Myrow et al. Reference Myrow, Hughes, Searle, Fanning, Peng and Parcha2009; Harper et al. Reference Harper, Zhan, Stemmerik, Liu, Donovan, Stouge, Gutiérrez-Marco, Rábano and García-Bellido2011; Zhan et al. Reference Zhan, Harper, Jin, Liang, Liu, Stemmerik and Stouge2014; Corthouts et al. Reference Corthouts, Lageson and Shaw2015; Yu et al. Reference Yu, Fang, Munnecke, Li, Li, Wang and Zhang2019). It is confirmed that the sedimentary package forming the peak of Mount Qomolangma is early–middle Darriwilian (Middle Ordovician) in age, but the succession at Mount Oomolangma (Mount Everest) is incomplete in comparison to the Nyalam succession (e.g. Sakai et al. Reference Sakai, Sawada, Takigami, Orihashi, Danhara, Iwano, Kuwahara, Dong, Cai and Li2005; Corthouts et al. Reference Corthouts, Lageson and Shaw2015).
The Darriwilian succession at Nyalam, southern Tibet reflects an upwards deepening trend during middle–late Darriwilian time. The overall deepening from shallow warm-water carbonate platform deposition to deeper-water deposition is associated with the arrival of the temperate- to cool-water North Atlantic Province Eoplacognathus–Yangtzeplacognathus fauna and the appearance of the pandemic Pygodus serra – Pygodus anserinus conodont fauna. Significantly, the upper Darriwilian red crinoid packstone at the base of the Upper Formation is associated with the appearance of Pygodus anserinus. A similar and coeval deepening and faunal development is recorded on the margin to slope of North China, South China, Tarim Basin and Sibumasu terranes or plates.
The overall deepening in the SE Asian region allowed the relatively warm-water taxa, for example, Dzikodus and Histiodella, to extend their palaeogeographical range into higher latitudes and peaked in the Histiodella kristinae Zone (middle Darriwilian). The late-middle Darriwilian short-term cooling event and associated sea-level drop (e.g. Haq & Schutter, Reference Haq and Schutter2008; Trotter et al. Reference Trotter, Williams, Barnes, Lécuyer and Nicoll2008; Cherns & Wheeley, Reference Cherns, Wheeley and Bassett2009; Cherns et al. Reference Cherns, Wheeley, Popov, Ghobadi Pour, Owens and Hemsley2013; Albanesi et al. Reference Albanesi, Barnes, Trotter, Williams and Bergström2020; Stouge et al. Reference Stouge, Bagnoli and Rasmussen2020), probably astronomically forced (Zhang et al. Reference Zhang, Shen and Algeo2010; Fang et al. Reference Fang, Wu, Wang, Yang, Li and Zhang2019a), is marked by the global MDICE (Cherns et al. Reference Cherns, Wheeley, Popov, Ghobadi Pour, Owens and Hemsley2013). The event caused the disappearance – possibly the extinction – of the temperate characteristic genera Lenodus and Microzarkodina, and the deeper-water low-latitude genera Dzikodus and Histiodella. The appearance of the new genera Eoplacognathus, Pygodus, Yangtzeplacognathus and Sagittodontus followed, and this major change – especially evident in South China and southern Tibet – is the dramatic faunal response to the global late-middle Darriwilian (Dw2) short-term cooling and lowstand event. Minor events like this during the Ordovician Period have received far less attention than the major Hirnantian extinction, one of the ‘big five’, and the understanding and significance of the late Darriwilian event is still at a premature stage.
Acknowledgements
We wish to thank the Danish Innovation Center in Shanghai for the initiation, organization and financial support of the expedition. This project was also partially supported by the National Natural Science Foundation of China (40825006, 41221001 and 41290260), the State Key Laboratory of Palaeobiology and Stratigraphy (LPS), mainly for the laboratory studies, and the Carlsberg Foundation (Denmark). The authors are grateful to Dr Annalisa Ferretti and an anonymous referee for their constructive comments and helpful suggestions, which improved the manuscript. This paper is a contribution to the IGCP 653 project ‘The onset of the Great Ordovician Biodiversification Event’.
Conflict of interest
None.