1. Introduction
The recognition of silicic large igneous provinces (LIPs) in the geological record has led to the reassessment of many continental margin volcanic provinces worldwide (Bryan et al. Reference Bryan, Riley, Jerram, Stephens, Leat, Menzies, Klemperer, Ebinger and Baker2002). The Chon Aike Province of Patagonian South America (Pankhurst et al. Reference Pankhurst, Leat, Sruoga, Rapela, Márquez, Storey and Riley1998) and the Antarctic Peninsula (Riley & Leat, Reference Riley and Leat1999) represents one of the world's largest silicic LIPs (Bryan et al. Reference Bryan, Riley, Jerram, Stephens, Leat, Menzies, Klemperer, Ebinger and Baker2002) and is, in part, contemporaneous with the Karoo flood basalt province (e.g. Riley et al. Reference Riley, Millar, Watkeys, Curtis, Leat, Klausen and Fanning2004), although clear magmatic and tectonic links are tenuous.
The silicic volcanic rocks of the Chon Aike Province were emplaced mainly in the form of subaerial ignimbrites (Pankhurst et al. Reference Pankhurst, Leat, Sruoga, Rapela, Márquez, Storey and Riley1998), and Pankhurst et al. (Reference Pankhurst, Riley, Fanning and Kelley2000) identified three distinct volcanic episodes at c. 185 Ma (V1), c. 168 Ma (V2) and c. 155 Ma (V3). The 168 Ma (V2) event is well represented on the Antarctic Peninsula and has been described in detail from the Oscar II Coast region of eastern Graham Land (Fig. 1) where the Mapple Formation has been defined (Riley & Leat, Reference Riley and Leat1999) and has been detailed geochemically (Riley et al. Reference Riley, Leat, Pankhurst and Harris2001).

Figure 1. Geological map of the northern Antarctic Peninsula showing the main outcrop area of Jurassic Antarctic Peninsula Volcanic Group rocks (from Hathway, Reference Hathway2000).
In this paper the Mapple Formation of the Oscar II Coast is correlated with other isolated volcanic exposures from elsewhere on the east coast of the Antarctic Peninsula. The correlations are based on recent fieldwork observations and a review of archive field data, combined with newly acquired and published geochronological data. The outcome is a more robust stratigraphy for the east coast of the Antarctic Peninsula, with a better understanding of how the silicic volcanic rocks are related to other Mesozoic and older successions.
2. Volcanic rocks of the Antarctic Peninsula
With the exception of Cenozoic volcanic rocks associated with the slowing and cessation of subduction, Thomson & Pankhurst (Reference Thomson, Pankhurst, Oliver, James and Jago1983) assigned all volcanic rocks on the Antarctic Peninsula to the Antarctic Peninsula Volcanic Group (APVG). This grouping took no account of tectonic setting, eruption age or chemistry, but grouped basalt–rhyolite compositions of Mesozoic age from fore-, intra- or back-arc settings.
More recently, Leat & Scarrow (Reference Leat and Scarrow1994), Riley & Leat (Reference Riley and Leat1999), Pankhurst et al. (Reference Pankhurst, Riley, Fanning and Kelley2000), Riley et al. (Reference Riley, Leat, Pankhurst and Harris2001) and Hunter et al. (Reference Hunter, Riley, Cantrill, Flowerdew and Millar2006) have attempted to divide the APVG into groups and formations based on their age, chemistry and tectonic setting.
Many of the igneous rocks exposed on the western side of the Antarctic Peninsula are Cretaceous in age and have dominantly intermediate compositions, but extensive, thick (at least 1 km), dominantly silicic volcanic rocks (and plutonic rocks), which occur irregularly along the eastern side of the Antarctic Peninsula, are typically Middle Jurassic in age and have very different isotopic and geochemical characteristics from the Cretaceous intermediate rocks on the west coast. The isotopic variation across the Antarctic Peninsula can be attributed to variable amounts of magma–crust interaction within the arc (Hole, Pankhurst & Saunders, Reference Hole, Pankhurst, Saunders, Thomson, Crame and Thomson1991), such that the crustal input increases from the west to the east coast (e.g. Saunders & Tarney, Reference Saunders and Tarney1982). The compositional variation may, however, suggest different mechanisms of magma generation in the Jurassic in comparison with the Cretaceous, or certainly some change in the subduction regime, as suggested by Rapela et al. (Reference Rapela, Pankhurst, Llambías, Labudía and Artabe1996) for southern South America.
The silicic volcanic rocks of the east coast are associated with granitoid plutons of Early–Middle Jurassic age (Pankhurst, Reference Pankhurst1982; Leat, Scarrow & Millar, Reference Leat, Scarrow and Millar1995) and thick sequences of sedimentary rocks, all of which have a characteristic continental affinity. The volcanic rocks of the west coast are typically mafic–intermediate in composition and characterized by thick volcaniclastic sequences, particularly in the fore-arc and intra-arc settings. Rare rhyolitic centres have also been identified in Palmer Land (e.g. Leat & Scarrow, Reference Leat and Scarrow1994), but these are Cretaceous in age and are not the thick plateau, caldera-fed ignimbrites typical of the east coast. Geochemically the volcanic successions of the west coast are lower in elements such as Nb, Zr and Y, which is more characteristic of a juvenile volcanic arc than the more mature continental margin crust and lithosphere of the east coast.
3. The Chon Aike Province
The Chon Aike province of Patagonia (Fig. 2) extends from the Atlantic Coast to the Chilean side of the Andes (Pankhurst et al. Reference Pankhurst, Leat, Sruoga, Rapela, Márquez, Storey and Riley1998) and is correlated with the Jurassic silicic volcanic rocks of the Antarctic Peninsula (Riley & Leat, Reference Riley and Leat1999). In eastern Patagonia, the volcanic rocks are predominantly flat-lying and undeformed where they overlie crystalline basement rocks of Precambrian to earliest Jurassic age and Lower Jurassic rift-related sedimentary rocks. In contrast, silicic volcanic rocks of the Andean Cordillera form relatively narrow outcrops, which are locally deformed, tilted and strongly affected by hydrothermal alteration. The province is dominated by phenocryst-poor ignimbrites, which vary in degree of welding from high-grade rheomorphic ignimbrites, to the volumetrically dominant, non-welded lithic-rich ignimbrites. They are sourced from multiple caldera centres (e.g. Aragón, Rodriguez & Benialgo, Reference Aragón, Rodriguez and Benialgo1996). Volumetrically minor rhyolite lavas, fallout deposits, debris flow deposits and epiclastic deposits are interbedded with the ignimbrites.
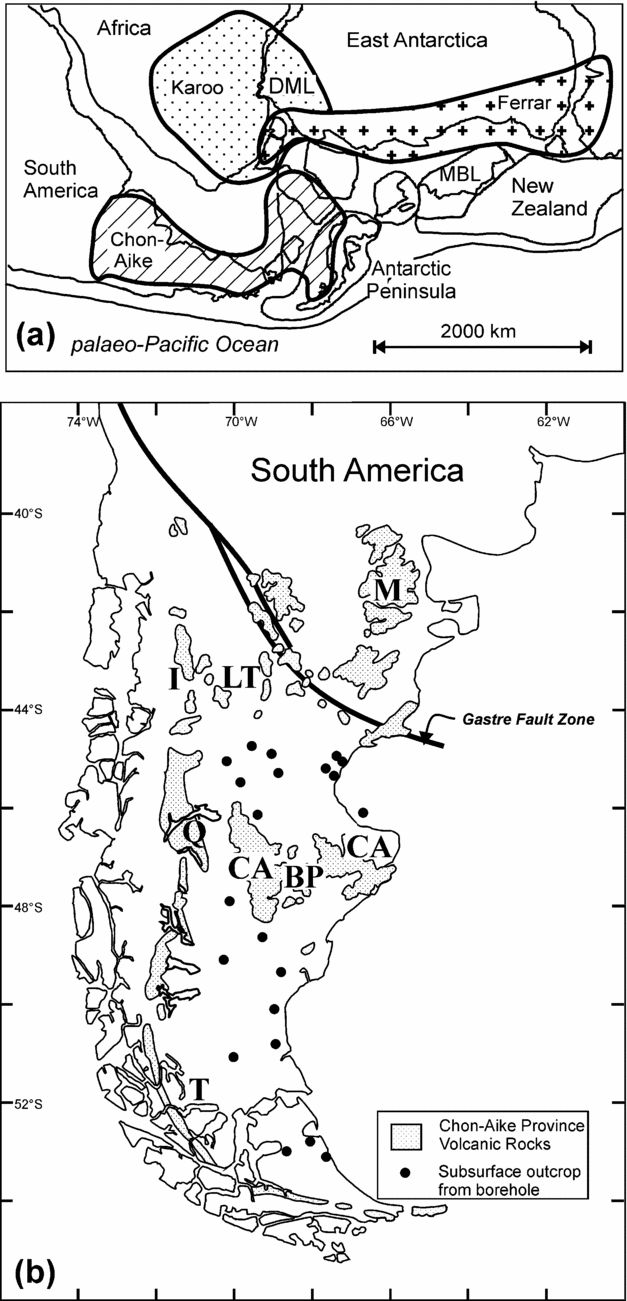
Figure 2. (a) Reconstruction of pre-break-up western Gondwana showing the major magmatic provinces (Karoo, Ferrar and Chon-Aike), after Storey et al. (Reference Storey, Alabaster, Hole, Pankhurst, Wever, Storey, Alabaster and Pankhurst1992). MBL – Marie Byrd Land; DML – Dronning Maud Land. (b) The Jurassic Chon-Aike volcanic province of Patagonia showing extent of outcrop and subsurface exposures. Individual formations: M – Marifil; LT – Lonco Trapial; I – Ibañez; Q – El Quemado; CA – Chon-Aike; BP – Bajo Pobre; T – Tobífera (after Pankhurst et al. Reference Pankhurst, Leat, Sruoga, Rapela, Márquez, Storey and Riley1998).
The province is chemically bimodal (Pankhurst et al. Reference Pankhurst, Leat, Sruoga, Rapela, Márquez, Storey and Riley1998) but is dominated by rhyolite, with only rare intermediate (basaltic andesite and/or andesite) compositions. The eruptive ages of silicic volcanic rocks from Patagonia and the Antarctic Peninsula have been defined by U–Pb ion microprobe dating (Pankhurst et al. Reference Pankhurst, Riley, Fanning and Kelley2000) and 40Ar–39Ar geochronology (Féraud et al. Reference Féraud, Alric, Fornari, Bertrand and Haller1999; Pankhurst et al. Reference Pankhurst, Riley, Fanning and Kelley2000). The age data indicate that volcanism continued for as long as 30 Ma, from the Early Jurassic to Late Jurassic, but occurred in three main phases. The first phase of volcanism (188–178 Ma; V1 of Pankhurst et al. Reference Pankhurst, Riley, Fanning and Kelley2000) has a peak eruptive age of 184 ± 2 Ma, and brackets the peak of flood basalt volcanism of the Karoo and Ferrar provinces at 183 ± 1 Ma (e.g. Encarnación et al. Reference Encarnación, Fleming, Elliot and Eales1996; Riley et al. Reference Riley, Curtis, Leat, Watkeys, Duncan, Millar and Owens2006). The Chon Aike and Tobífera formations of southernmost Patagonia, however, yield ages in the interval 167–171 Ma (V2) and overlap with the Mapple Formation of the Antarctic Peninsula (Pankhurst et al. Reference Pankhurst, Riley, Fanning and Kelley2000). The final episode, V3 (157–153 Ma), is confined to the Andean volcanic outcrops of Argentina and Chile, although small granite bodies of this age occur both in western Patagonia and in the western Antarctic Peninsula, and may be subvolcanic equivalents. The three eruptive phases define an age progression of magmatism from an intraplate to continental margin setting, away from the locus of flood basalt volcanism in the Karoo province (Pankhurst et al. Reference Pankhurst, Riley, Fanning and Kelley2000; Fig. 2). Concomitant with the age progression is a change in rhyolite composition from intraplate (higher Nb, Zr; V1) to increasingly calc-alkaline (lower Nb, Zr; V2 and V3) geochemical signatures. The Middle Jurassic silicic volcanic rocks of the Mapple Formation (Antarctic Peninsula) and those of the Chon Aike Formation (South America) are thought to have been generated as a result of anatexis of Grenvillian-age hydrous mafic lower crust of andesitic composition, linked to pre-Middle Jurassic subduction, and superimposed fractional crystallization (Pankhurst & Rapela, Reference Pankhurst and Rapela1995; Riley et al. Reference Riley, Leat, Pankhurst and Harris2001).
4. Age relationships and stratigraphy
The crystalline basement crops out sporadically along the east coast, with the greatest concentration occurring near the Adie Inlet region (Fig. 1). Elsewhere along the east coast, particularly to the north of Cape Disappointment (Fig. 3), the local basement is the ?Permian–Triassic quartzose metasedimentary Trinity Peninsula Group. The Trinity Peninsula Group succession is at least 3 km thick, and facies analysis indicates deposition as submarine fans along a continental margin (Smellie, Roberts & Hirons, Reference Smellie, Roberts and Hirons1996). The Trinity Peninsula Group is variably deformed, from gentle folding without cleavage development to tight polyphase folding with well-developed cleavage.

Figure 3. Simplified geological map of the Oscar II Coast area.
Sedimentary rocks of the Botany Bay Group unconformably overlie the metasedimentary rocks of the Trinity Peninsula Group. The Botany Bay Group is dominated by non-marine conglomerates, interpreted as the products of debris flow and braided stream deposition on alluvial fans (Farquharson, Reference Farquharson1984). Interbedded with the alluvial deposits are sandstone and mudstone packages, characterized by abundant plant fossils. Sedimentation associated with the Botany Bay Group is, in part, contemporaneous with Middle Jurassic silicic volcanism (Hunter et al. Reference Hunter, Cantrill, Flowerdew and Millar2005), although there is uncertainty regarding the age of the lower part of the succession, which may well be Early–Middle Jurassic in age based on plant fossil evidence (Rees, Reference Rees1993a,Reference Reesb).
4.a. Mapple Formation
The Oscar II Coast region (Fig. 3) exhibits the greatest range and most widespread concentration of silicic volcanic rocks in the Antarctic Peninsula (Fleet, Reference Fleet1968). Rhyolitic volcanic rocks conformably overlie or are interbedded with up to 600 m of siltstones and mudstones of the Botany Bay Group. Riley & Leat (Reference Riley and Leat1999) defined the Mapple Formation from the Mapple Glacier region where there is a maximum observed thickness of 1000 m. Because of within-flow facies variations and significant faulting, it is difficult to correlate units, so a type section for the Mapple Formation was not defined. However, in general terms the Mapple Formation is dominated by ignimbrite units and crystal tuffs (about 80 %). The volcanic rocks are almost entirely subaerial, although rare chert horizons and development of siliceous nodules within some horizons may imply localized subaqueous emplacement (Reedman et al. Reference Reedman, Howells, Orton and Campbell1987). Eruptive centres have proved very difficult to identify, although close proximity to source can be locally inferred from mass flow deposits and co-ignimbrite breccias.
The ignimbrite and crystal tuff units are typically phenocryst-poor, but with a mineral assemblage characterized by embayed quartz, K-feldspar, plagioclase, biotite, magnetite, apatite, titanite, rutile and zircon.
The Mapple Formation was metamorphosed and deformed, probably during the Cretaceous Palmer Land compressional event (Vaughan, Pankhurst & Fanning, Reference Vaughan, Pankhurst and Fanning2002). Metamorphic grade ranges up to greenschist facies in the western part of the outcrop. Associated deformation generated open folds and low-angle faults. The low-angle faults localized movement on mud- to sand-grade epiclastic deposits between the more competent ignimbrites and lava flows. Steeply dipping cleavage is developed in epiclastic and many mudflow deposits, and increases in intensity westward.
The Mapple Formation was deposited in Middle Jurassic times in the interval 168–171 Ma, based on four U–Pb zircon ages (Pankhurst et al. Reference Pankhurst, Riley, Fanning and Kelley2000). Mapple Formation volcanism overlapped with part of the extensive Chon Aike Formation (168–169 Ma; Pankhurst et al. Reference Pankhurst, Riley, Fanning and Kelley2000) of South America. Pankhurst et al. (Reference Pankhurst, Riley, Fanning and Kelley2000) included that part of the Chon Aike and the Mapple Formation in their V2 volcanic episode, which was assigned an age of 162–172 Ma, with a peak in the interval 167–172 Ma.
5. Mapple Formation: correlated successions
Six separate volcanic sections or multiple successions along the north and east coast of the Antarctic Peninsula are described here. They are all considered to be coeval with the Mapple Formation based on their lithology, volcanology, geochronology and stratigraphic relationships. The correlations are further supported by recent field observations and newly acquired U–Pb geochronology.
5.a. D'Urville Monument and Cape Alexander, Joinville Island
5.a.1. Occurrence
Joinville Island is located at the northeastern tip of the Antarctic Peninsula (Fig. 1) and is a geological continuation of Graham Land, dominated by sedimentary successions of the Trinity Peninsula Group and Botany Bay Group. Relatively minor outcrops of volcanic rocks have also been identified along the southern coast of Joinville Island (Elliot, Reference Elliot1967; this study). Part of the Lower Jurassic–Cretaceous Nordenskjöld Formation is also exposed on the southeast tip of Mount Alexander (Fig. 1). However, no stratigraphic relationship is observed with the Botany Bay Group or the volcanic rocks, as the area is extensively intruded by diorite–tonalite plutons of uncertain age. Similar pluton compositions on the adjacent Danger Islands have been dated at c. 85 Ma (Hamer, Reference Hamer1984).
The volcanic rocks are best exposed at D'Urville Monument (Elliot, Reference Elliot1967) and Mount Alexander (this study). At D'Urville Monument (Fig. 1), flow-banded, devitrified rhyolite lavas are dominant and are accompanied by acid tuffs, which are host to locally derived lithic fragments. At Mount Alexander, a volcanic succession, at least 300 m thick, is exposed. Moderately to strongly welded ignimbrites are the dominant lithology, accompanied by fine-grained, feldspar-phyric rhyolitic lavas. A separate succession of unfossiliferous, but presumed Botany Bay Group, mudstones and siltstones are interbedded with silicic volcanic rocks, including tuffs, hyaloclastite and flow-banded lavas, which further documents contemporaneous sedimentation and volcanism.
5.a.2. Age
Two silicic volcanic rocks (R.8308.1, R.8309.1) which are interbedded with sedimentary rocks of the Botany Bay Group have been dated using U–Pb zircon analysis (Table 1) obtained with the NORDSIM ion microprobe (Swedish Natural History Museum), following the method of Whitehouse & Kamber (Reference Whitehouse and Kamber2005). Sample R.8308.1 crops out near the exposed base of the succession and is a rhyolitic autoclastic breccia with feldspar–quartz–phyric rhyolite clasts, which are partly silicified. The zircon separates are typically clear, prismatic and small (< 100 μm length), are non-luminescent under cathodo-luminescence and no inherited zircon was identified. They have very high U concentrations (~ 7500 ppm) and are highly cracked, to the extent that locating an ion probe spot away from the cracks was difficult and explains why many analyses have suffered Pb loss and have high common Pb contents. Because of the high U concentrations, calibration with the 91500 zircon (81 ppm U) standard is likely to be invalid and explains why some analyses are reversely discordant. The 207Pb/206Pb ages are, however, considered reliable. The three points without common Pb correction (spots 2, 3 and 4; Table 1) yield a weighted mean of their 207Pb/206Pb ages of 171 ± 11 Ma, while three points (spots 5, 8 and 9; Table 1) with significant common Pb corrections and recent Pb loss yield a weighted mean of their 207Pb/206Pb ages of 144 ± 37 Ma. These six analyses combined yield a weighted mean of 168 ± 11 Ma (Fig. 4a), which is considered to be the eruptive age. All other data are considered meaningless in view of their large uncertainty and are so discounted from the age calculation.
Table 1. U–Pb ion microprobe zircon data

All U–Pb zircon geochronology was carried out using a Cameca 1270 ion-microprobe housed at the NORDSIM facility, Swedish Museum of Natural History. Analytical method followed that described by Whitehouse & Kamber (Reference Whitehouse and Kamber2005). Pb/U ratios, Th/U and elemental concentrations were calibrated against the 1065 Ma 91500 zircon standard (Wiedenbeck et al. Reference Wiedenbeck, Alle, Corfu, Griffin, Meirer, Oberli, von Quadt, Roddick and Spiegel1995). Concordia ages were calculated using Isoplot version 3.1 (Ludwig, Reference Ludwig2003) and the decay constants of Steiger & Jäger (Reference Steiger and Jäger1977). All uncertainties include the uncertainty of the standard calibration.
1Percentage of 206Pb attributed to common Pb, which is calculated from the measured 204Pb. Bracketed values are not corrected. Common Pb is corrected for the volcanic zircon analyses where f 206% is greater than 0.5. It is assumed that the common Pb comes from surface contamination and the present day terrestrial Pb values of Stacey & Kramers (Reference Stacey and Kramers1975) are used to perform this correction.

Figure 4. Concordia diagrams for (a) volcanic R.8308.1, (b) volcanic R.8309.1 and (c) sandstone R.6648.2b. Ages on (a) and (b) are interpreted as eruption ages, while those on (c) represent ages for detrital zircon populations. See text for further details regarding age calculations.
Sample R.8309.1 is a flow-banded rhyolite lava cropping out stratigraphically higher than R.8308.1, but still close to the exposed base of the Botany Bay Group succession. The sample is partly devitrified, fine-grained with feldspar and quartz phenocrysts, and displays chaotic flow banding. The zircon population from R.8309.1 is very similar to that from R.8308.1. Grains are small (< 100 μm length), prismatic and non-luminescent under cathodo-luminesence. They have U concentrations an order of magnitude lower than R.8308.1, and therefore the standard calibration is considered valid. The four analyses (Fig. 4b) yield a weighted mean of their 206Pb/238U ages of 162.4 ± 1.8 Ma, which is considered to be the eruptive age.
The two new ages and stratigraphy of the volcanic rocks from Joinville Island are entirely consistent with the volcanism being a sequence correlatable with the Mapple Formation of the Oscar II Coast.
5.b. Kenney Glacier Formation, Hope Bay
5.b.1. Occurrence
The volcanic rocks at Hope Bay (Fig. 5) overlie the Early–Middle Jurassic sedimentary rocks (Mount Flora Formation: Botany Bay Group; Elliot & Gracanin, Reference Elliot, Gracanin, Oliver, James and Jago1983) first described by Halle (Reference Halle1913), who assigned a Middle Jurassic age based on comparisons with the Jurassic flora of rocks from Yorkshire. The volcanic rocks are characterized by rhyolitic/dacitic ignimbrites, lavas and rare, fine-grained volcanic breccias and have been assigned the name ‘Kenney Glacier Formation’ (Birkenmajer, Reference Birkenmajer1992, Reference Birkenmajer1993). Birkenmajer (Reference Birkenmajer1993) described an angular unconformity between the Kenney Glacier Formation and the underlying sedimentary Mount Flora Formation, although Farquharson (Reference Farquharson1984) suggested some uncertainty over the contact between the two. Elsewhere along the east coast, a conformable relationship has been observed and described between the Botany Bay Group and the Antarctic Peninsula Volcanic Group. Both Farquharson (Reference Farquharson, Oliver, James and Jago1983) and Elliot & Gracanin (Reference Elliot, Gracanin, Oliver, James and Jago1983) describe several ignimbrite and lapilli tuff layers which punctuate the upper half of the sedimentary rocks of the Mount Flora Formation. A similar relationship is observed at other localities and implies a gradual shift between terrestrial–shallow water sedimentation and entirely volcanic conditions, with contemporaneous sedimentation and volcanism at some point.

Figure 5. Simplified geological map of the Hope Bay–Botany Bay area. The inset stratigraphic logs are from the Camp Hill (1) and Hope Bay (2) areas (Hunter et al. Reference Hunter, Riley, Cantrill, Flowerdew and Millar2006).
5.b.2. Age
Pankhurst et al. (Reference Pankhurst, Riley, Fanning and Kelley2000) dated (U–Pb zircon) a welded acidic tuff (R.609.1; ignimbrite) from the Mount Flora Formation. It is one of several volcanic units interbedded with the sedimentary rocks near the top of the Mount Flora Formation. A concordant age of 162.2 ± 1.1 Ma was reported, which also gives a maximum age of the overlying but broadly contemporaneous Kenney Glacier Formation.
5.c. Camp Hill, Botany Bay, Bald Head
5.c.1. Occurrence
The volcanic rocks of Camp Hill conformably overlie a thick (780 m) sequence of terrestrial sedimentary rocks (fluviatile–deltaic) of the Botany Bay Group (Camp Hill Formation; Farquharson, Reference Farquharson1984). The volcanic succession is approximately 50 m in thickness and crops out at Church Point, to the east of Camp Hill and at Bald Head (Fig. 5). The volcanic rocks are distinct from the Mapple Formation rhyolites seen elsewhere in eastern Graham Land, in being dominated by agglomerates near the base of the exposed section, overlain by a thick sequence of garnetiferous andesite lavas, tuffs and volcaniclastics near the upper part of the succession (Farquharson, Reference Farquharson1984). The Camp Hill Formation itself lacks volcanic units, with the exception of a single accretionary lapilli horizon which occurs approximately half-way up the section (Farquharson, Reference Farquharson1984). The lapilli are set in a matrix of devitrified glass shards and provide the only evidence of contemporaneous volcanism and sedimentation at Botany Bay.
5.c.2. Age
Several workers have attempted to date the sedimentary and volcanic succession at Botany Bay. Millar, Milne & Whitham (Reference Millar, Milne and Whitham1990) dated (Sm–Nd) primary igneous garnets from an andesitic sill, which cuts the base of the volcanic succession, and obtained an age of 152 ± 8 Ma, although the Sm and Nd concentrations are unusually high for garnet and may suggest some inclusions were present. Pankhurst et al. (Reference Pankhurst, Riley, Fanning and Kelley2000) have dated (U–Pb zircon) an andesite sill cutting the same Camp Hill succession dated by Millar, Milne & Whitham (Reference Millar, Milne and Whitham1990) but determined an age of 166.9 ± 1.6 Ma, confirming that the Sm–Nd age does not precisely date the intrusion of the sills, or that magmatism continued until at least 152 Ma, or that the dated zircons are inherited. More recently, Hunter et al. (Reference Hunter, Cantrill, Flowerdew and Millar2005) dated detrital zircons from the single lapilli tuff horizon interbedded with the sedimentary Camp Hill Formation, which yielded an interpreted maximum eruption age of 167.1 ± 1.1 Ma. The zircon geochronology overwhelmingly suggests a Middle Jurassic age for the volcanic rocks at Botany Bay, correlative with the Mapple Formation, and younger than the Early Jurassic age suggested by Rees (Reference Rees1993a), based on the fossil flora assemblage from the Camp Hill Formation.
5.d. Mount Tucker, Tower Peak
5.d.1. Occurrence
The sedimentary Tower Peak Formation (Botany Bay Group) is predominantly debris flow fed conglomerates which reach a maximum thickness of 124 m (Farquharson, Reference Farquharson1984; Fig. 6). The upper half of the exposed sequence is punctuated by volcanic horizons, including a 21 m thick massive crystal-lithic tuff, a 6 m thick altered tuff unit containing abundant accretionary lapilli and rare agglomerate horizons. The Tower Peak Formation at Tower Peak and Mount Tucker (Fig. 6) is conformably overlain by a sequence of agglomerates and garnet-bearing andesite lavas (~ 750 m thick; Aitkenhead, Reference Aitkenhead1975), akin to the succession observed at Camp Hill. The volcanic rocks are also in faulted contact with metasedimentary rocks of the Trinity Peninsula Group.

Figure 6. Simplified geological map of the Cape Longing–Sobral Peninsula area. The inset stratigraphic log is from the Tower Peak area (Hunter et al. Reference Hunter, Riley, Cantrill, Flowerdew and Millar2006).
5.d.2. Age
Millar, Milne & Whitham (Reference Millar, Milne and Whitham1990) dated (Sm–Nd) three garnet-bearing conglomerate samples which overlie the crystal-lithic tuff from the Tower Peak Formation. The four garnet fractions from Tower Peak have a restricted range in Sm/Nd ratio and do not yield a precise age, but fall on an errorchron indicating an age of 165 ± 57 Ma. The combined Tower Peak and Camp Hill Sm–Nd data yielded an age of 156 ± 6 Ma (Millar, Milne & Whitham, Reference Millar, Milne and Whitham1990).
Hunter et al. (Reference Hunter, Cantrill, Flowerdew and Millar2005) have also dated volcanic rocks from the upper part of the Tower Peak Formation. They used zircon separates from a conglomerate, which is host to volcanic fragments. The zircons yielded a concordia age of 168.9 ± 1.3 Ma, which was interpreted to date deposition of the sedimentary Tower Peak Formation and again confirmed contemporaneous sedimentation with volcanic activity of the Mapple Formation.
5.e. Jason Peninsula
5.e.1. Occurrence
Jason Peninsula, with an area of approximately 3000 km2, extends eastward from Graham Land into the Larsen Ice Shelf (Fig. 7). Its geology is characterized by relatively flat-lying ignimbrites, crystal tuffs and lava flows. They are associated, in part, with rare basaltic and basaltic andesite lavas and sills (Saunders, Reference Saunders1982) at Standring Inlet and Stratton Inlet (Fig. 7). At Standring Inlet, a 30 m (minimum) succession of spheroidally weathered basaltic lavas is associated with finely bedded basaltic volcaniclastic rocks. The succession at Stratton Inlet is interpreted as two separate 20 m thick basaltic sills. A succession, at least 450 m thick, of silicic volcanic rocks is exposed on Jason Peninsula, but it is intensely frost-shattered and any stratigraphical interpretations are difficult (Smellie, Reference Smellie, Ulbrich and Campos1991). The ignimbrites locally contain carbonized wood (petrified tree trunks) and subaerial deposition is inferred (del Valle et al. Reference del Valle, Lirio, Lusky, Morelli and Nunez1997). The volcanic rocks on Jason Peninsula are severely altered, having undergone extreme silicification, with SiO2 values up to 85 wt %. They are also strongly iron-stained following the oxidation of finely disseminated Fe-sulphides.

Figure 7. Simplified geological map of the Jason Peninsula–Churchill Peninsula area. The inset stratigraphic log is generalized from the field area. GLVG – Graham Land Volcanic Group.
The silicic volcanic rocks are presumed to be overlain by fossiliferous sedimentary rocks (Riley et al. Reference Riley, Crame, Thomson and Cantrill1997), which are seen to crop out at the eastern extremity of Jason Peninsula, at Cape Framnes (Fig. 7). The sedimentary rocks were interpreted to be a distal outcrop and equivalent of the Latady Group (Hunter & Cantrill, Reference Hunter and Cantrill2006) exposed further south, and the fossil assemblage was assigned a Kimmeridgian–early Tithonian age (Riley et al. Reference Riley, Crame, Thomson and Cantrill1997).
5.e.2. Age
There are no recorded U–Pb (zircon) ages for the Jason Peninsula silicic rocks, although del Valle et al. (Reference del Valle, Lirio, Lusky, Morelli and Nunez1997) summarized K–Ar (whole rock) analyses, which give ages in the range 156–191 Ma. These K–Ar results fail to constrain the true eruption age or to demonstrate whether the basaltic lavas and sills are contemporaneous with the rhyolites.
Riley et al. (Reference Riley, Leat, Kelley, Millar and Thirlwall2003) used Ar–Ar geochronology on whole rock and plagioclase phenocrysts from the basaltic rocks of Jason Peninsula (Stratton Inlet and Standring Inlet). Six ages in the range 168–175 Ma were obtained and overlap with the age of the Mapple Formation (168–171 Ma), indicating essentially coeval rhyolitic and basaltic magmatism. Detrital zircons from the Kimmeridgian–early Tithonian sedimentary rocks at Cape Framnes (Riley et al. Reference Riley, Crame, Thomson and Cantrill1997) have been analysed (Table 1) by NORDSIM ion microprobe (Swedish Natural History Museum), and three distinct age populations can be identified at 179 ± 6, 166 ± 4 and 147 ± 5 Ma (Fig. 4b). Although few in number, the ages of the populations are of significance for accurately dating the local volcanic events. The 166 ± 4 Ma zircon population is interpreted to be derived from the local silicic volcanic rocks on Jason Peninsula, while the 179 ± 6 Ma population may record a volcanic episode correlating to the V1 event of Pankhurst et al. (Reference Pankhurst, Riley, Fanning and Kelley2000). The youngest detrital zircon age (147 ± 5 Ma) overlaps with the Kimmeridgian–early Tithonian age indicated by the mixed fossil assemblage described by Riley et al. (Reference Riley, Crame, Thomson and Cantrill1997) and indicates contemporaneous Late Jurassic sedimentation and volcanism.
5.f. Churchill Peninsula
5.f.1. Occurrence
Beyond the area of outcrop of the Mapple Formation on the Oscar II Coast (Fig. 1), the greatest concentration of silicic volcanic rocks in eastern Graham Land is the Churchill Peninsula region (Fig. 7), where volcanic rocks are seen primarily in association with felsic–intermediate plutons. No stratigraphic relationship is observed with the Botany Bay Group, although a small outcrop of hornfelsed terrestrial mudstones and siltstones is in faulted contact with the silicic volcanic rocks. The volcanic rocks of the Churchill Peninsula region are, however, occasionally seen to unconformably overlie granitic and migmatitic gneisses of uncertain age, although possibly correlative with the Permian migmatites of Adie Inlet (Millar, Pankhurst & Fanning, Reference Millar, Pankhurst and Fanning2002) or Triassic gneisses of the Bowman Coast (Flowerdew, Reference Flowerdew2008).
The volcanic rocks of the region are dominated by thick sequences (up to 400 m) of crystal tuffs and crystal-lithic tuffs, with rare ignimbrite horizons (Fig. 8a). Individual tuff units tend to be thick (~ 30–60 m) and monotonous, and have an apparent porphyritic texture. The tuff units are also interbedded with rare lava flows (up to 4 m thick) and pitchstone units (Fig. 8b), accretionary lapilli tuffs (Fig. 8c) and breccia/agglomerate units. The volcanic rocks were deposited subaerially, although Marsh (A. F. Marsh, unpub. Ph.D. thesis, Univ. Birmingham, 1968) interpreted some successions as being deposited subaqueously. However, recent fieldwork failed to find any convincing evidence to support subaqueous emplacement. The general style of volcanism, with thick, monotonous, crystal tuffs, suggests an intracaldera-type succession, particularly in the area to the north of the Churchill Peninsula.

Figure 8. (a) Eutaxitic texture in welded silicic ignimbrite from Churchill Peninsula, Mapple Formation. Lens cap is 58 mm diameter. (b) Rhyolitic pitchstone lava, Lyttleton Ridge, Churchill Peninsula area. (c) Accretionary lapilli in planar bedded silicic ash fall unit from Churchill Peninsula, Mapple Formation. Lens cap is 58 mm diameter.
Alteration in the silicic volcanic rocks of the area is intense in places. The rocks at the southern extremity of the Churchill Peninsula (Fig. 7) are akin to those of the Jason Peninsula and have undergone silicification, pyritization and oxidation of the iron sulphides. Also, within the main outcrop area of the crystal tuffs, distinct bands of intensely altered rocks are observed and are interpreted to mark the location of intense hydrothermal activity.
5.f.2. Age
There is only one published age from the silicic volcanic rocks of the Churchill Peninsula area. Pankhurst (Reference Pankhurst1982) reported a five-point Rb–Sr isochron for a rhyolitic tuff from Gulliver Nunatak (Fig. 7), which yielded an age of 174 ± 2 Ma, essentially contemporaneous with the Mapple Formation volcanic rocks further north.
Granitic plutonism, coeval with the silicic volcanism, has also been identified from the Mapple Formation outcrop area (Riley & Leat, Reference Riley and Leat1999; Riley et al. Reference Riley, Leat, Pankhurst and Harris2001). The granitoids are isotopically identical to the silicic volcanic rocks (Riley et al. Reference Riley, Leat, Pankhurst and Harris2001) and fall within the same age interval. Granite and granodiorite plutons from the Churchill Peninsula area have also been dated by Pankhurst (Reference Pankhurst1982) at 167 ± 2, 169 ± 3 and 173 ± 6 Ma and suggest they are the subvolcanic equivalents of the Mapple Formation volcanic rocks.
6. Discussion
6.a. Stratigraphic relationships and correlations
The widespread occurrence of Jurassic volcanic rocks across the Antarctic Peninsula has been recognized for decades, but the identification of distinct episodes of volcanism relating to widespread regional events has been less easy to establish. Pankhurst et al. (Reference Pankhurst, Leat, Sruoga, Rapela, Márquez, Storey and Riley1998) correlated components of Antarctic Peninsula volcanism with the extensive Jurassic silicic volcanism of Patagonia. This study has allowed widespread correlations to be made across the northern Antarctic Peninsula using newly acquired geochronology (U–Pb) and recent fieldwork.
Age comparisons between the Mapple Formation (Cape Disappointment area; Fig. 3) and exposures at Hope Bay, Joinville Island, Botany Bay, Tower Peak, Churchill Peninsula and Jason Peninsula are made, and demonstrate that volcanism can be assigned to a common event, in the interval 162–172 Ma and with a peak at c. 168 Ma. There is therefore a very strong case based on both age and field characteristics for correlating these exposures.
The field characteristics of the Mapple Formation described by Riley & Leat (Reference Riley and Leat1999) from the Cape Disappointment area are similar to the silicic volcanic rocks from the other localities, wherein the succession is dominated (typically > 80 %) by ash flow tuffs, in the form of crystal tuffs, crystal lithic tuffs or ignimbrites. Individual units vary in thickness from < 0.5 m to up to 100 m and there is a wide variation in the degree of welding (rare rheomorphic ignimbrites exist) and lithic content. The Mapple Formation and its correlatives are also characterized by rhyolitic, frequently flow-laminated lava flows, volcanic breccias (including avalanche and mass flow deposits), and rare, but relatively widespread, bedded, air fall tuffs, characterized by rim-type lapilli.
The volcanic rocks exposed at Tower Peak and Camp Hill (Botany Bay) have also been dated in the interval c. 167–169 Ma, but are compositionally and volcanologically very different from the Mapple Formation rocks and their correlatives. Basaltic and intermediate compositions are very rare in the Mapple Formation, but the successions at Tower Peak and Camp Hill are dominated by andesitic lavas and agglomerates, which reach a maximum thickness of ~ 750 m. Interestingly, the Botany Bay Group sedimentary rocks that underlie the andesitic lavas and agglomerates at Camp Hill and Tower Peak both contain volcanic horizons that are more typical of Mapple Formation volcanic rocks (e.g. rhyolitic crystal tuffs, accretionary lapilli tuffs), which are also common to the sequences exposed at Hope Bay, Joinville Island and Cape Disappointment.
The basalts and basaltic andesites described from Jason Peninsula are unlikely to be directly related to the Tower Peak and Camp Hill andesites, which are more intermediate in composition and are characteristically garnet-bearing. However, both occurrences indicate that there was at least some basic–intermediate magmatism associated with the predominantly silicic volcanism of the east coast of the Antarctic Peninsula. A similar scenario exists in the Patagonian Chon Aike Province (Pankhurst et al. Reference Pankhurst, Leat, Sruoga, Rapela, Márquez, Storey and Riley1998), where the component formations are all silicic in character, with the exception of the Lonco Trapial and Bajo Pobre formations, which are dominated by andesite and basaltic andesite lavas, agglomerates and breccias. The intermediate lavas from Patagonia yielded significantly younger ages (c. 152 Ma; Pankhurst et al. Reference Pankhurst, Riley, Fanning and Kelley2000) than the nearby silicic volcanic rocks. Even without the uncertainty of age surrounding the intermediate magmatism, there is clear potential for separating the successions at Tower Peak and Camp Hill, which are dominated by andesites, from the dominantly silicic Mapple Formation and its correlatives. It is proposed that the dominantly andesitic lavas and agglomerates exposed at Camp Hill and Tower Peak be given the formal stratigraphic name of the Mount Tucker Formation. To provide a type section would be misleading, given the nature of the volcanic rocks exposed at Mount Tucker and elsewhere, however, the general field characteristics of the Mount Tucker Formation are:
(1) The volcanic rocks are intermediate in composition (typically andesite).
(2) The succession (up to 750 m thick) is dominated by agglomerates, with rare interbedded lava flows.
(3) The volcanic rocks overlie or are interbedded with terrestrial sedimentary rocks of the Botany Bay Group.
(4) The volcanic rocks are typically garnet-bearing, as are the conglomerates underlying the volcanic rocks.
In the case of the silicic volcanic successions described from elsewhere along the east coast of the Antarctic Peninsula, it is stratigraphically acceptable to refer to them as Mapple Formation, unless they have been formally named elsewhere (e.g. Kenney Glacier Formation; Birkenmajer, Reference Birkenmajer1993).
6.b. Graham Land Volcanic Group
As discussed previously, the term ‘Antarctic Peninsula Volcanic Group’ (APVG), introduced by Thomson & Pankhurst (Reference Thomson, Pankhurst, Oliver, James and Jago1983), takes no account of tectonic setting, eruption age or chemistry, but groups together rocks of basalt–rhyolite compositions of Mesozoic age from fore-, intra- or back-arc settings. Recently, Hunter et al. (Reference Hunter, Riley, Cantrill, Flowerdew and Millar2006) defined the Ellsworth Land Volcanic Group, which comprises the Early Jurassic silicic and basaltic volcanic formations from the southern Antarctic Peninsula. These formations are clearly distinct from the Graham Land volcanic rocks described here and the Cretaceous arc-related sequences on the west coast of the Antarctic Peninsula. Hunter et al. (Reference Hunter, Riley, Cantrill, Flowerdew and Millar2006) defined the Ellsworth Land Volcanic Group largely on the basis of tectonic setting and age of the volcanic sequences, and similar criteria will be used here to define the Graham Land Volcanic Group. The key characteristics are:
(1) Age: in the interval 162–172 Ma, with the maximum eruption episode at c. 168 Ma.
(2) Composition: typically silicic (rhyolite–rhyodacite), although rare intermediate units occur.
(3) Association: coeval and cogenetic with granitoids, which are presumed to be subvolcanic equivalents of the rhyolites.
(4) Isotope characteristics: volcanic rocks and coeval granitoids falling within very restricted ranges (87Sr/86Sr168 = 0.7066–0.7070; ɛNd168 = −2 to −3).
(5) Lithology: characterized by pyroclastic deposits: crystal tuffs, lithic tuffs, lapilli tuffs and ignimbrites. Lavas are rare.
(6) Source: typically caldera-fed, thick (> 1 km) successions, extensive over large areas (plateau ignimbrites).
(7) Relationship: the onset of volcanism is characterized by contemporaneous deposition of terrestrial sedimentary rocks.
As defined, the Graham Land Volcanic Group would include the Mapple Formation and its silicic correlatives at Hope Bay (Kenney Glacier Formation), Joinville Island, Churchill and Jason peninsulas, and also the intermediate volcanic rocks of the Mount Tucker Formation at Botany Bay and Tower Peak. The rocks are restricted to the north and east coasts of Graham Land and probably include volcanic rocks exposed further south from Churchill Peninsula, such as the rhyolites (A. F. Marsh, unpub. Ph.D. thesis, Univ. Birmingham, 1968) exposed at Cape Robinson (Fig. 1).
All of the volcanic units described here are correlated with the Chon Aike Province of Patagonian South America and specifically with the V2 event of Pankhurst et al. (Reference Pankhurst, Riley, Fanning and Kelley2000). These volcanic units were erupted along the continental margin of Gondwana and were the result of melting of subduction-modified crustal underplate and lower crust (Riley et al. Reference Riley, Leat, Pankhurst and Harris2001).
The volcanic rocks of the Graham Land Volcanic Group are distinct from the volcanic successions which crop out along the west coast of the Antarctic Peninsula, where volcanism is typically mafic–intermediate in composition and characterized by thick volcaniclastic sequences, particularly in the fore–intra-arc setting. Rare rhyolitic centres have also been identified in Palmer Land (Leat & Scarrow, Reference Leat and Scarrow1994) and eastern Ellsworth Land (Flowerdew et al. Reference Flowerdew, Millar, Vaughan and Pankhurst2005), but these are Cretaceous in age and are not the characteristic thick plateau, caldera-fed ignimbrites of the east coast, but are smaller, central volcano-fed outflow facies.
The volcanic rocks of the Ellsworth Land Volcanic Group (Hunter et al. Reference Hunter, Riley, Cantrill, Flowerdew and Millar2006), which crop out further south along the eastern Antarctic Peninsula, are in many respects similar to the East Coast Volcanic Group defined here. Both groups are dominated by silicic pyroclastic rocks forming thick (> 1 km), caldera-fed successions. However, the Ellsworth Land Volcanic Group rocks have an average eruption age of 183 Ma (Hunter et al. Reference Hunter, Riley, Cantrill, Flowerdew and Millar2006) and are isotopically distinct from the Mapple Formation and its correlatives and are thought to relate to the wider V1 event of Pankhurst et al. (Reference Pankhurst, Riley, Fanning and Kelley2000).
Acknowledgements
Catrin Thomas, James Wake, and the air operations staff at Rothera Base and HMS Endurance are thanked for their field support. Thoughtful and constructive reviews by David Elliot, Bob Pankhurst and an anonymous reviewer all helped to improve the final manuscript. Kerstin Lindén and Lev Ilyinsky are thanked for their assistance at the NORDSIM facility. This is NORDSIM contribution number 244.