1. Introduction
The Neves–Corvo mine region is located in the southeastern sector of the Rosário–Neves–Corvo antiform, a NW–SE-oriented structure (Fig. 1) of the Iberian Pyrite Belt (IPB). The IPB is part of the South Portuguese Zone (SPZ); it represents the southernmost major tectonostratigraphic segment of the Iberian Variscides, and is restricted to sedimentary and igneous rocks of Devonian and Carboniferous age (Quesada et al. Reference Quesada, Braid, Fernandes, Ferreira, Jorge, Matos, Murphy, Oliveira, Pedro, Pereira, Quesada and Oliveira2019).

Fig. 1. Geological map of the Neves–Corvo mine region with massive sulphide deposits location (orebodies surface projection from Lundin Mining data). The Graça and Corvo formations are only recognized in drill holes. Studied drill holes and geological sections are presented in Figures 2, 3, 6 and 7 (geology adapted from Oliveira et al. Reference Oliveira, Romão, Matos, Leal and Rosa2016).
The IPB is a world-class distinctive mining province with unusually large metal sulphide concentrations in giant and super-giant massive sulphide ore deposits such as Aljustrel and Neves–Corvo in Portugal and Rio Tinto, Las Cruces, Aguas Teñidas–Magdalena, Tharsis, La Zarza, Sotiel, Aznalcóllar and Los Frailes in Spain, as the most representative (Barriga et al. Reference Barriga, Carvalho and Ribeiro1997; Leistel et al. Reference Leistel, Marcoux, Thiéblemont, Quesada, Sánchez, Almodóvar, Pascual and Sáez1998; Tornos, Reference Tornos2006; Locutura, Reference Locutura and García-Cortés2011; Almodóvar et al. Reference Almodóvar, Yesares, Sáez, Toscano, González and Pons2019; Gisbert et al. Reference Gisbert, Losantos, Tornos, Pons and Videira2019). Lousal, Caveira and São Domingos in Portugal and Herrerias in Spain are smaller deposits in the IPB. These mineralizations are hosted by bimodal volcanic sequences included in the Volcano-Sedimentary Complex (VSC, Famennian – late Visean age) (Oliveira et al. Reference Oliveira, Quesada, Pereira, Matos, Solá, Rosa, Albardeiro, Díez-Montes, Morais, Inverno, Rosa, Relvas, Quesada and Oliveira2019; Quesada et al. Reference Quesada, Braid, Fernandes, Ferreira, Jorge, Matos, Murphy, Oliveira, Pedro, Pereira, Quesada and Oliveira2019). Extensional processes during Upper Devonian time led to the IPB basin formation being characterized by a considerable submarine depositional volcanism. The submarine setting record includes fossils, microfossils, felsic and mafic lavas and massive sulphide mineralizations (Barriga et al. Reference Barriga, Carvalho and Ribeiro1997; Leistel et al. Reference Leistel, Marcoux, Thiéblemont, Quesada, Sánchez, Almodóvar, Pascual and Sáez1998; Carvalho et al. Reference Carvalho, Barriga, Munhá, Barrie and Hannington1999; Tornos, Reference Tornos2006; Pereira et al. Reference Pereira, Matos, Fernandes and Oliveira2008; Rosa et al. Reference Rosa, Rosa, Matos and Relvas2010; Oliveira et al. Reference Oliveira, Rosa, Rosa, Pereira, Matos, Inverno and Andersen2013b ). During late Visean time, the tectonic regime changed to crustal compression. The syn-orogenic Baixo Alentejo Flysch Group was formed in this setting overlying the VSC. Flysch sedimentation occurred until late Moscovian time (Oliveira, 1990; Oliveira et al. Reference Oliveira, Quesada, Pereira, Matos, Solá, Rosa, Albardeiro, Díez-Montes, Morais, Inverno, Rosa, Relvas, Quesada and Oliveira2019). The compressive stage was conditioned by folding and thrust development with significant SW-directed vergence.
Since the discovery of the Neves–Corvo deposit in 1977, significant exploration investments have been made in the IPB (Leca et al. Reference Leca, Ribeiro, Oliveira, Silva, Albouy, Carvalho and Merino1983; Carvalho et al. Reference Carvalho, Barriga, Munhá, Barrie and Hannington1999; Relvas et al. Reference Relvas, Barriga, Ferreira, Noiva, Pacheco and Barriga2006). The mine is currently owned by Somincor/Lundin Mining that exploits copper, zinc and lead (tin is exhausted) in stockwork and massive sulphide ores from five orebodies: Neves, Graça, Corvo, Zambujal and Lombador. Current total reserves (Lundin Mining, 2019) are: 27.9 Mt of copper ore, with 2.2% Cu, 0.7% Zn, 0.2% Pb and 32 g t–1 Ag; and 29.7 Mt of zinc ore, with 7.5% Zn, 0.3% Cu, 1.8% Pb and 64 g t–1 Ag. In recent years, the company’s near-mine exploration program (surface and borehole, electromagnetic, constrained gravity inversion and seismic surveys) led to the discovery of the Semblana (2010) and Monte Branco (2012) massive sulphide deposits and related stockwork mineralization. The previously identified Rosa Magra stockwork (Pacheco et al. Reference Pacheco, Beliz, Carvalho, Clayton and Sevastopoulo1994; Carvalho, Reference Carvalho1995) was recently drilled and confirmed to be a northern extension of the Semblana deposit.
In Neves–Corvo, as elsewhere in the IPB, the volcanogenic massive sulphide (VMS) deposits and related hydrothermal systems occur in close association with felsic volcanic rocks and black shales, suggesting a strong genetic relationship between ore-forming processes, volcanic activity and sedimentation (Tornos, Reference Tornos2006; Rosa et al. Reference Rosa, Rosa, Matos and Relvas2010; Oliveira et al. Reference Oliveira, Rosa, Rosa, Pereira, Matos, Inverno and Andersen2013b ).
This study describes the lithological successions enveloping the Semblana and Monte Branco deposits and their extensions into the Rosa Magra and Zambujal sectors, respectively, using high-resolution stratigraphy, based on key research proxies such as palynostratigraphy of sediments, geochronology of volcanic rocks (U–Pb in zircon) and structural reconstruction of the host-rock successions. Selected log-based cross-sections were built in accordance with the regional geological setting. This approach not only represents an important contribution to the geological knowledge of the mineralized sequences recently discovered in the near-mine area, but also provides a set of conclusions and criteria with important implications for the exploration of new targets in the IPB.
2. Geological setting
The lithostratigraphic sequence of the Neves–Corvo mine area (Fig. 1; Oliveira et al. Reference Oliveira, Pereira, Carvalho, Pacheco and Korn2004; Pereira et al. Reference Pereira, Matos, Fernandes and Oliveira2008; Oliveira et al. Reference Oliveira, Relvas, Pereira, Matos, Rosa, Rosa, Munhá, Fernandes, Jorge, Pinho, Dias, Araújo, Terrinha and Kullberg2013a , b; Mendes et al. Reference Mendes, Pereira, Matos, Morais, Albardeiro, Solá, Pacheco and Araújo2018) comprises three main units defined at regional scale (in ascending order): the Phyllite–Quartzite Group (PQG), the Volcano-Sedimentary Complex (VSC) and the Mértola Formation (the lower unit of the Baixo Alentejo Flysch Group).
The PQG is composed of phyllites and quartzites of the Phyllite–Quartzite Formation of middle Givetian – late Famennian age, with a total thickness in excess of 100 m with base unknown (Pereira et al. Reference Pereira, Matos, Fernandes and Oliveira2008, Reference Pereira, Matos, Batista, Sóla, Salgueiro, Oliveira, Oliveira, Inverno and Rosa2014; Mendes et al. Reference Mendes, Pereira, Matos, Morais, Albardeiro, Solá, Pacheco and Araújo2018, Reference Mendes, Pereira, Matos, Albardeiro, Morais, Solá, Salgueiro, Pacheco, Araújo, Inverno and Oliveira2020). The uppermost levels of this formation can locally present crinoids bearing limestones (Forno da Cal quarry limestones; Leca et al. Reference Leca, Ribeiro, Oliveira, Silva, Albouy, Carvalho and Merino1983; Mendes et al. Reference Mendes, Pereira, Matos, Albardeiro, Morais, Solá, Salgueiro, Pacheco, Araújo, Inverno and Oliveira2020). The VSC is divided into a Lower and an Upper VSC sequence (Fig. 2), separated by a middle–upper Tournaisian sedimentary stratigraphic hiatus (Oliveira et al. Reference Oliveira, Pereira, Carvalho, Pacheco and Korn2004, Reference Oliveira, Rosa, Rosa, Pereira, Matos, Inverno and Andersen2013b ; Pereira et al. Reference Pereira, Matos, Fernandes and Oliveira2008, Reference Pereira, Matos, Batista, Sóla, Salgueiro, Oliveira, Oliveira, Inverno and Rosa2014). The Lower VSC sequence comprises bimodal volcanic rocks (essentially felsic volcanic rocks, including coherent and abundant autoclastic rhyolites and rhyodacites, pumice breccias, and minor basalt lava flows and intrusive mafic volcanic rocks with a total thickness that can reach c. 100 m), Famennian age dark-grey shales of c. 40 m thick of the Corvo Formation (only exposed underground) and black pyritic shales of the Neves Formation, which show variable thicknesses of a few metres to c. 100 m, with interbedded massive sulphides. The Neves Formation shales are dated as being of late Famennian (Strunian) age based on the Retispora lepidophyta – Verrucosisporites nitidus (LN) Miospore Biozone, corresponding to an age of between 359 Ma (Devonian–Carboniferous boundary, International Chronostratigraphic Chart, 2020/01) and c. 360.5 Ma (Streel et al. Reference Streel, Brice and Mistiaen2006; Streel, Reference Streel2009). The well-defined Strunian age of the Neves Formation, coupled with its close association with the massive sulphide mineralization, make this unit a key stratigraphic horizon and a prime exploration guide not only in the Neves–Corvo mine region, but also in other sectors of the IPB, such as the Lousal, Caveira, Herrerias–Tharsis and Aznalcóllar areas (Gonzalez et al. 2002; Oliveira et al. Reference Oliveira, Pereira, Carvalho, Pacheco and Korn2004, Reference Oliveira, Rosa, Rosa, Pereira, Matos, Inverno and Andersen2013b ; Pereira et al. Reference Pereira, Matos, Fernandes and Oliveira2008, Reference Pereira, Matos, Rosa and Oliveira2012, Reference Pereira, Matos, Batista, Sóla, Salgueiro, Oliveira, Oliveira, Inverno and Rosa2014; Matos et al. Reference Matos, Pereira, Rosa, Rosa, Oliveira and Relvas2011; Saez et al. Reference Saez, Moreno and González2011). On top of this mineralized sequence, a commonly discontinuous unit of jaspers and exhalative carbonates (JC unit) occurs.

Fig. 2. Geological section across the Corvo orebody and the Rosa Magra stockwork mineralization, eastern region of the Neves–Corvo deposit. Section updated from Carvalho & Ferreira (Reference Carvalho and Ferreira1994).
The volcanic facies have previously been classified based on chemostratigraphic criteria (T Barrett, unpub. report, 2006, 2008) and on physical volcanology features (Rosa et al. Reference Rosa, McPhie, Relvas, Pereira, Oliveira and Pacheco2008). They range from volcaniclastic transported facies, with fiamme, quartz-feldspar crystals and lithic fragments (Rhyolite types 1 and 2) to massive-coherent and autoclastic facies (Rhyolite type 3). Rhyolite type 1 is a massive euhedral to subhedral quartz-phyric fiamme rock with quartz and crystal fragments, felsic clasts and disseminated sulphides; fiamme facies can range from breccia, crystal sandstone to mudstone. Rhyolite type 2 is characterized by abundant quartz and feldspar crystal fragments, felsic clasts, mudstone and limestone clasts and rare fiamme corresponding to a polymictic fiamme sandstone facies. Rhyolite type 3 comprises coherent rhyolites, quartz and feldspar phyric (10–20% vol) in a perlitic micropoikilitic groundmass with quartz–carbonates–chlorite-filled amygdalae and spherulite- and phyllosilicate-rich flow bands; this rhyolite type also includes jigsaw-fit and clast-rotated monomictic breccia (hyaloclastite) similar to previous coherent rhyolite facies, but more perlitic. The volcanic breccia consists of rhyolite clasts and crystal fragments matrix (Rosa et al. Reference Rosa, McPhie, Relvas, Pereira, Oliveira and Pacheco2008). Based on the geochemical signatures, Rhyolite type 1 has higher Al2O3/TiO2, Zr/TiO2 and Zr/Al2O3 than Rhyolite types 2 and 3, the last two with tholeiitic to calc-alkaline affinity (high Zr/Y). These have similar Al2O3/TiO2 and Zr/TiO2 content, but the former presents low La/Yb and Zr/Y (tholeiitic to calc-alkaline with low Zr/Y), while the latter has calc-alkaline (high Th/Yb and Zr/Y) to alkaline (low Zr/Nb and high Nb/Y) affinity.
Rhyolites types 1 and 2 occur associated with the Neves Formation black shales and the Corvo Formation shales, and both show intense hydrothermal alteration related to stockwork mineralization. Rhyolite type 3, common in the Lombador sector, occurs mostly in the hanging wall of the mineralization. However, as defended by Rosa et al. (Reference Rosa, McPhie, Relvas, Pereira, Oliveira and Pacheco2008), it can be locally found immediately below massive sulphides occasionally hosting stockwork veins and chlorite alteration. Nonetheless, this rhyolite type does not superimpose Rhyolite types 1 and 2, suggesting a different spatial distribution. The zircon U–Pb ages of these three types of rhyolites range from late Famennian to Tournaisian (c. 349–363 Ma), that is, a time span of c. 14 Ma (Solá et al. Reference Solá, Salgueiro, Pereira, Matos, Rosa, Araújo, Neto and Lains2015; Albardeiro et al. Reference Albardeiro, Solá, Salgueiro, Morais, Matos, Mendes, Batista, Pereira, Inverno, Oliveira, Rosa and Pacheco2017). The late Famennian rhyolites are related to ore-forming hydrothermal activity, and the Tournaisian rhyolites represent a later, barren, volcanic episode exclusive to the Rhyolite type 3 unit (Albardeiro et al. Reference Albardeiro, Solá, Salgueiro, Morais, Matos, Mendes, Batista, Pereira, Inverno, Oliveira, Rosa and Pacheco2017). The late Famennian zircon ages overlap (within uncertainty) the cassiterite U–Pb ages of Neves–Corvo early cassiterite mineralization (363–366 Ma; Li et al. Reference Li, Zhao, Jiang and Palmer2019).
The stockwork (stringer-type) mineralization described either in mine galleries or in exploration drill holes occurs throughout the Lower VSC and PQG sequences accompanied by intense hydrothermal alteration. The latter has textural and mineralogical patterns, and elemental and stable isotopic signatures show a well-developed zonation, varying from proximal to distal alteration facies (Relvas et al. Reference Relvas, Barriga, Ferreira, Noiva, Pacheco and Barriga2006).
The Upper VSC sequence (of c. 350–400 m in total thickness) is represented, from the base upwards, by shales, siltstones and fine-grained volcanogenic rocks (Ribeira de Cobres Formation, uncertain age), dark-grey shales with siliceous-phosphate nodules (Graça Formation, early–middle Visean age), grey shales with carbonate nodules, volcanogenic sedimentary rocks and intrusive mafic volcanic rocks (Grandaços Formation, middle–late Visean age), purple and green shales (Borra de Vinho Formation, middle–late Visean age), siliceous shales and volcanogenic sedimentary rocks (Godinho Formation, middle–late Visean age) and grey and black pyritic shales with minor greywackes (Brancanes Formation, middle–late Visean age) (Oliveira et al. Reference Oliveira, Pereira, Carvalho, Pacheco and Korn2004, Reference Oliveira, Rosa, Rosa, Pereira, Matos, Inverno and Andersen2013b ; Pereira et al. Reference Pereira, Matos, Fernandes and Oliveira2008; Fig. 1).
The VSC is overlain by the Mértola Formation, the lowermost unit of the Baixo Alentejo Flysch Group (of middle–late Visean age; Oliveira, 1983; Pereira et al. Reference Pereira, Matos, Fernandes and Oliveira2008, Reference Pereira, Matos, Batista, Sóla, Salgueiro, Oliveira, Oliveira, Inverno and Rosa2014; Oliveira et al. Reference Oliveira, Rosa, Rosa, Pereira, Matos, Inverno and Andersen2013b ), which is a turbidite sequence comprising alternating dark-grey shales and greywackes of c. 3000 m thickness (in the type area, Mértola region). In the Neves–Corvo mine area, three turbidite sequences ascribed to the Mértola Formation (MT) were chronologically distinguished based on palynostratigraphy (Oliveira et al. Reference Oliveira, Pereira, Carvalho, Pacheco and Korn2004), namely MT 1 (Raistrickia nigra – Triquitrites marginatus (NM) Miospore Biozone), MT 2 (Tripartites vetustus – Rotaspora fracta (VF) Miospore Biozone) and MT 3 (VF to Bellisporites nitidus – Cingulizonates capistratus (NC) Miospore Biozone).
Figure 2 shows a representative cross-section that extends from the Corvo orebody to the Rosa Magra stockwork area, illustrating the regional tectonic style characterized by low-angle folds and thrust sheet repetitions of parts of the stratigraphic sequence, including the massive sulphide deposits and their stockwork roots. Subsequently, the thrust sheets were refolded developing an open SE-dipping antiform with vergence towards the SW (Leca et al. Reference Leca, Ribeiro, Oliveira, Silva, Albouy, Carvalho and Merino1983; Silva et al. Reference Silva, Oliveira, Ribeiro, Dallmeyer and Martinez Garcia1990; Carvalho et al. Reference Carvalho, Barriga, Munhá, Barrie and Hannington1999; Oliveira et al. Reference Oliveira, Rosa, Rosa, Pereira, Matos, Inverno and Andersen2013b , 2016). Several kilometre-long thrust planes were identified in seismic surveys carried out in this region (Carvalho et al. Reference Carvalho, Inverno, Matos, Rosa, Granado, Branch, Represas, Carabaneanu, Matias and Sousa2016). The Upper and Lower VSC sequences are separated by an important shear zone, the Neves Structural Corridor, identified through deep drilling and characterized by intense tectonic deformation and abundant quartz veinlets (Carvalho & Ferreira, Reference Carvalho and Ferreira1994; Oliveira et al. Reference Oliveira, Carvalho, Pereira, Pacheco, Fernandes and Korn1997, Reference Oliveira, Rosa, Rosa, Pereira, Matos, Inverno and Andersen2013b ).
3. Materials and methods
Ten drill holes were selected and sampled to target the Neves–Corvo eastern area (Fig. 1). Core samples collected for palynology and geochronology studies were referenced by their corresponding depths along the hole traces (Tables 1 and 2).
Table 1. Selected drill holes and samples for palynostratigraphic study.

Table 2. Selected samples for zircon U–Pb geochronology of felsic volcanic rocks.

* Poorly represented number of zircons, age interpreted as inheritance.
For the palynostratigraphy study, 282 samples of sedimentary rocks were collected from all 10 drill holes (Table 1). Standard palynological laboratory procedures (Wood et al. Reference Wood, Gabriel, Lawson, Jansonius and McGregor1996) were performed at the laboratories of LNEG (National Laboratory of Energy and Geology) to extract and concentrate the palynomorphs. The slides were examined in transmitted light using a BX40 Olympus microscope equipped with an Olympus C5050 digital camera. All samples, residues and slides are stored at LNEG facilities in São Mamede de Infesta, Portugal.
The miospore biozonal scheme adopted follows the standard Western Europe Miospore Zonation (after Clayton et al. Reference Clayton, Coquel, Doubinger, Gueinn, Loboziak, Owens and Streel1977; Streel et al. Reference Streel, Higgs, Loboziak, Riegel and Steemans1987; Pereira, Reference Pereira1999; Pereira et al. Reference Pereira, Matos, Fernandes and Oliveira2008; Higgs et al. Reference Higgs, Prestianni, Streel and Thorez2013). The selected palynomorphs from the Semblana region are depicted in online Supplementary Figure S1 (available at http://journals.cambridge.org/geo). The total number of studied samples per drill hole, and those that yielded positive results, are listed in Table 1. The palynomorph assemblages recovered from the positive samples are presented in online Supplementary Table S1 (available at http://journals.cambridge.org/geo).
Additionally, a polished thin-section of every fine-grained sediment sample was studied under the microscope.
U–Pb zircon geochronology was carried out for a group of 10 felsic volcanic rock samples recovered from 4 drill holes (Table 2). Although age results for 8 out of the 10 samples were previously available (Solá et al. Reference Solá, Salgueiro, Pereira, Matos, Rosa, Araújo, Neto and Lains2015; Albardeiro et al. Reference Albardeiro, Solá, Salgueiro, Morais, Matos, Mendes, Batista, Pereira, Inverno, Oliveira, Rosa and Pacheco2017), concordia plots, cathodoluminescence (CL) images and data tables with individual ages are presented here for the first time. The sampled rocks are all rhyolites representing different thrust sheets of the VSC sequence. Sample preparation at LNEG laboratories followed standard procedures for grain size, magnetic and heavy-density liquid separations according to Mange & Maurer (Reference Mange and Maurer1992), and the ideal zircon size fraction (63–250 μm) was used by individual hand-picking under the Zeiss Stemi SV6 binocular microscope. Due to the small volume of available rock drill core (short diameter of drill core; half/quarter cores used), some samples provided a very limited number of zircon grains. The zircon grains were mounted in epoxy-type resin, polished and coated with a golden cap for cathodoluminescence/backscattering imaging, in order to identify internal structures (inheritance and overgrowths) and for target selection.
The isotopic analysis followed the U–Pb laser ablation inductively coupled plasma mass spectrometry (ICP-MS) method performed at the Mineralogy and Geology Museum of the Senckenberg Naturhistorische Sammlungen Dresden, Germany, using a Thermo-Scientific Element 2 XR sector field ICP-MS coupled to a New Wave UP-193 Excimer Laser System (e.g. Košler & Sylvester, Reference Košler and Sylvester2003; Guillong, Reference Guillong2004; Košler, Reference Košler2007). The procedure used laser spot sizes of 15–35 μm diameter, 15 s of background acquisition followed by 35 s of data acquisition using GJ-1 standard zircon. Common-Pb correction followed Stacey & Kramers (Reference Stacey and Kramers1975) protocol and an Excel spreadsheet program developed by Axel Gerdes (Institute of Geosciences, Johann Wolfgang-Goethe, University Frankfurt) was used for raw data correction (background signal, common Pb, laser-induced elemental fractionation, instrumental mass discrimination and time-dependent elemental fractionation of Pb/Th and Pb/U).
For zircons older than 1.0 Ga the 207Pb/206Pb data were used instead, while for younger zircons the 206Pb/238U ratio was used. Details of the analytical protocol and data processing are provided in Frei & Gerdes (Reference Frei and Gerdes2009). Data interpretation in the form of concordia diagrams was carried out using Isoplot 4 software (Ludwig, Reference Ludwig2009; 2-sigma error ellipses and concordia ages with 95% confidence interval).
Table 2 shows the gathered concordia ages, as well as the inherited groups of ages for each sample. Online Supplementary Figure S1 and Tables S2–S11 (available at http://journals.cambridge.org/geo) display all analytical raw data supplied by the laboratory.
4. The Semblana deposit
The Semblana deposit was discovered in 2010 by Somincor/AGC. The exploration approach consisted of follow-up drilling of a strong off-hole electromagnetic conductor, highlighted in an area initially targeted based on coincident constrained inversion of ground gravity and surface transient electromagnetic subtle anomalies, and extrapolation of favourable geology down-dip of the Zambujal and Corvo orebodies, as indicated by 3D geology/structural modelling (Araújo & Castelo Branco, Reference Araújo and Castelo Branco2011; Lundin Mining, 2013, 2017).
Located 1 km NE of the Zambujal orebody (Fig. 1), the Semblana deposit was further recognized in dozens of exploration drill holes. The massive and stockwork mineralization, intersected in several drill holes, contains 7.8 Mt of inferred resources with 2.9% Cu, considering a 1% Cu cut-off grade (Lundin Mining, 2017).
The geological section of the Semblana deposit presented in Figure 3a results from the combination of a geological section across drill hole PSL48A-1, with the projection of drill holes PSN44 and PSK50B on the same plane (see Fig. 1 for location). Stratigraphic correlations are based on drill-hole logs, palynological and geochronological ages, and geophysical information (seismic reflectors, Yavuz et al. Reference Yavuz, Kinkela, Dzunic, Penney, Neto, Araújo, Ziramov, Pevzner and Urosevic2015). The massive sulphide mineralization occurs in two small ore lenses imbricated within the Neves Formation The stockwork (veins with pyrite, chalcopyrite, arsenopyrite and other sulphides + chlorite, quartz and carbonates) is hosted both by shales of the Neves Formation and by rhyolites (Fig. 3a).

Fig. 3. Geological sections across the Semblana deposit and the Rosa Magra stockwork mineralization with studied drill holes (see geological map for location). Down-dip the drill holes, sample location with palynological assemblages or obtained U–Pb geochronological data.
All the studied drill holes intersect the rather long-established units of the stratigraphic succession of the Neves–Corvo mine region (cf. Reference Oliveira, Relvas, Pereira, Matos, Rosa, Rosa, Munhá, Fernandes, Jorge, Pinho, Dias, Araújo, Terrinha and KullbergOliveira et al. 2013a , b). The identification of the lithostratigraphic units in the drill holes was based on their lithological compositions, combined with palynological and geochronological data. The shales and thinly bedded quartzites of the PQG were sampled in drill hole PSL48A-1 (six positive samples), which yielded a miospore assemblage ascribed to VH Biozone of Famennian age as indicated by the common occurrences of the taxa Apiculiretusispora verrucosa and Grandispora echinata.
Going upwards, the Lower VSC starts with the Corvo Formation, characterized by grey shales and intercalated pumice breccia, which provided a poorly preserved miospore assemblage consisting of Cristatisporites triangulatus, Emphanisporites annulatus, Geminospora sp. and Rugospora sp. that indicates a Famennian age (one sample at 1093.5 m depth from the drill hole PSK50B).
The Neves Formation black shales were identified in all the studied drill holes; 14 studied samples yielded a late Strunian palynological age, based on miospore key species (see online Supplementary Table S1). The most complete assemblage was recovered from the PSL48A-1 drill hole consisting of the key species Retispora lepidophyta and Vallatisporites verrucosus in association with the taxa Auroraspora macra, Diducites spp., Geminospora spp., Grandispora spp., Rugospora explicata, R. flexuosa and Retusotriletes spp., and Maranhites genus (prasinophyte algae).
Felsic volcanic rocks are also well represented in the Lower VSC. They consist mostly of rhyolites and some rhyodacites (geochemically classified as Rhyolite types 1, 2 and 3 as described in Section 2).
The Lower VSC Rhyolite type 2 was sampled for U–Pb age determination in drill hole PSL48A-1, whereas Rhyolite type 3 was sampled in drill hole PSN44 (Fig. 3a and Table 2). In drill hole PSL48A-1, the 1013.0 m depth sample corresponds to a pumice breccia intersected below the black shales of the Neves Formation, dated palynologically of late Strunian age (947.8 m, LN Miospore Biozone). This rhyolite was affected by intense hydrothermal alteration (chlorite+sericite±disseminated sulphides) similar to that observed in the felsic volcanic units hosting the other orebodies of the Neves–Corvo mine (Relvas et al. Reference Relvas, Barriga, Ferreira, Noiva, Pacheco and Barriga2006; Rosa et al. Reference Rosa, McPhie, Relvas, Pereira, Oliveira and Pacheco2008), and provided a U–Pb age of 360.0 ± 2.1 Ma (late Famennian, Figs 3b, 4d–f; 46 zircon grains were analysed, resulting in 37 validated measurements, 20 of which were used for concordia plot and age calculations). Inheritance (or antecrysts) of older zircon grains included Upper Devonian (c. 366–379 Ma, 7 grains), Middle Ordovician (c. 462 Ma, 1 grain) and Mesoproterozoic (c. 1399 Ma, 1 grain) ages (Figs 3b, 4). Further volcanic rock samples (1111.5 m and 1119.5 m, PSL48A-1) are effusive rhyolite hyaloclastites here classified as Rhyolite type 2 (also by Barrett, Reference Barrett2011). These rocks are strongly affected by hydrothermal alteration (chlorite+carbonates) associated with a stockwork zone. In a sample at 1119.5 m of core length, the zircon grains yield a concordia age of 361.5 ± 2.5 Ma, interpreted as the crystallization age of the rhyolitic lava. This concordia age was calculated based of 5 of the 59 total U–Pb results. The assemblage also includes Upper (c. 371–385 Ma, 18 grains) and Middle Devonian (c. 388–390 Ma, 2 grains) grains, as well as 2 Neoproterozoic (c. 528–570 Ma) inherited zircons. Only a few zircon grains from the other sample (1111.5 m) were analysed, and the age obtained (c. 370 Ma) was interpreted as inherited (Fig. 4, Table 2, 6 zircon grains were used out of 14 to calculate concordia age). The consistency between the absolute ages obtained from the felsic volcanic rocks and the palynological dating of their enveloping sedimentary rocks indicates coeval volcanic activity and sediment deposition.

Fig. 4. Concordia ages and zircon cathodoluminescense/backscattered (CL/BS) images with concordant age plotting in explanatory grains for Semblana and Monte Branco samples. n = number of zircon analyses used to give each age/total of analyses each of sample. Concordia plot of (a) PSN44-845.9, concordia age 349.5 ± 2.9 Ma; and (b) zircon analyses of sample PSN44, concordia age 351.5 ± 3.2 Ma. Concordia plot and CL image for (c) sample PSN44-928; youngest age fraction, concordia age 352.7 ± 1.9 Ma (figure inset); and (d) sample PSL48A1-1 (113.5 m), concordia age 360.0 ± 2.1 Ma. Concordia plot and BS image for (e) sample PSL48A1-1 (111.5 m), concordia age 372.1 ± 6.2 Ma; and (f) sample PSL48A1-1 (119.5 m), concordia age 361.5 ± 2.5 Ma. Concordia plot and CL image for (g) sample SCA26-684.1, concordia age 350.1 ± 1.5 Ma; (h) sample SCA26-854.65, concordia age 358.8 ± 2.1 Ma; (i) sample SCA26-868.3, concordia age 359.8 ± 1.9 Ma; and (j) sample SU22-468.8, concordia age 356.9 ± 1.9 Ma.
Rhyolite type 3 samples were collected and selected for U–Pb dating in drill hole PSN44 at depths of 845.9, 848.5 and 928 m, the first two being volcanic hyaloclastite and the latter a felsic effusive massive volcanic rock. In all cases, shear planes affect the volcanic rocks, showing minor signs of hydrothermal alteration (sericite+quartz). Emplacement/crystallization U–Pb zircon ages of these rhyolites (Figs 4a–c, 5) are very similar for the three samples: 349.5 ± 2.9, 351.5 ± 3.2 and 352.7 ± 1.9 Ma U–Pb zircon ages (Tournaisian; Table 2). These three concordia ages were gathered based on 9 of 25 grains (27 mounted grains), 6 out of 18 (20 mounted zircons) and 33 out of 63 (67 mounted), respectively. Inherited zircon ages are within Upper Devonian age range: c. 363–369 Ma (3 grains), c. 360–363 Ma (2 grains) and c. 361–383 Ma (17 grains), respectively (Figs 4, 6c, d).

Fig. 5. U–Pb crystallization ages and selected inherited zircon ages of Semblana and Monte Branco rhyolites showing two well-defined age groups. Semblana drill holes: PSL48A-1 and PSN44; Monte Branco drill holes: SCA26 and SU22.

Fig. 6. Geological section of the Monte Branco deposit with studied drill holes (see location in Fig. 1). SDA44-1 hole is projected into the section. Along the drill holes, sample location with palynological assemblages or obtained U–Pb geochronological data.
The consistency of geochronological results suggests that the sampled rhyolites represent a younger volcanic episode of Tournaisian age. The fact that these younger rhyolites occur interbedded in (or within) the Neves Formation black shales of Strunian age suggest that they occur in a particularly complex tectonic setting where a number of repetitions were piled up, including massive sulphides (see geological section in Fig. 3a where younger rhyolites are emplaced between thrust fault planes).
In the geological section shown in Figure 3a, the horizon of volcanic rocks with ages of c. 351 Ma (Tournaisian) could well be coeval with the base of the Graça Formation.
As previously mentioned, the Semblana mineralization is hosted by the Neves Formation black shales and consists of massive sulphides and related stockwork. The Tournaisian felsic volcanic rocks only contain sulphide disseminations and veinlets parallel to the cleavage with subtle quartz+sericite alteration. Dominantly formed by pyrite, this late and weak mineralization may represent a post-Tournaisian syn-tectonic sulphide remobilization stage (Solá et al. Reference Solá, Salgueiro, Pereira, Matos, Rosa, Araújo, Neto and Lains2015; Albardeiro et al. Reference Albardeiro, Solá, Salgueiro, Morais, Matos, Mendes, Batista, Pereira, Inverno, Oliveira, Rosa and Pacheco2017).
The established units that compose the Upper VSC sequence (cf. Oliveira et al. Reference Oliveira, Pereira, Carvalho, Pacheco and Korn2004, Reference Oliveira, Relvas, Pereira, Matos, Rosa, Rosa, Munhá, Fernandes, Jorge, Pinho, Dias, Araújo, Terrinha and Kullberg2013a , b) were also identified in all the studied drill holes. Their ages were established based on miospore content (Fig. 3a, online Supplementary Table S1). The Graça Formation provided miospore assemblages of the Knoxisporites triangulates – Knoxisporites stephanephorus (TS) Biozone of early Visean age (drill hole PS50K at 687.0 and 637.7 m depths) and of the NM Biozone of middle–late Visean age (drill hole PSN44 at 604.8, 704.7 and 714.2 m depths). The Grandaços Formation also yielded a miospore assemblage of NM Biozone, as indicated by the recurring presence of the guide species Raistrickia nigra (drill holes PS50K and PSN44). Two samples recovered from the Godinho Formation and one sample from the Mértola Formation (drill hole PSN44) yielded assemblages of the NM Miospore Biozone. The same ages for the Grandaços, Godinho and Mértola formations were obtained in other sectors of the Neves–Corvo mine region (Oliveira et al. Reference Oliveira, Pereira, Carvalho, Pacheco and Korn2004).
Overall, the tectonic setting of the Semblana deposit consists of a complex PQ/Lower VSC parautochthonous block, tectonically overlain by the Upper VSC. The lithostratigraphic units are repeated by NW-trending low-angle thrusts forming a pile of thrust sheets with tectonic SW-directed transport. The tectonic deformation is apparently more intense in the PQG and Lower VSC units than in the Upper VSC because of the rheological contrast. A metre-sized zone with fractured core and gauge (called the Neves Structural Corridor) separates the Lower VSC from the Upper VSC in the Neves–Corvo mine. This contains a dense, sheared, deformed and white mottled quartz veining. This main thrust plane is evident in dozens of drill hole intersections and in seismic geophysical surveys (Lundin Mining database, Araújo & Castelo Branco, Reference Araújo and Castelo Branco2011; Carvalho et al. Reference Carvalho, Inverno, Matos, Rosa, Granado, Branch, Represas, Carabaneanu, Matias and Sousa2016), suggesting that the Neves Structural Corridor may have a wider regional expression than previously anticipated.
5. The Rosa Magra stockwork
Located 1.1 km ENE of the Corvo massive sulphide orebody and 600 m NW of the Semblana deposit (Fig. 1), the Rosa Magra stockwork zone was originally discovered by Somincor/Lundin Mining after resorting to gravity surveys (Pacheco et al. Reference Pacheco, Beliz, Carvalho, Clayton and Sevastopoulo1994). More recently, it was investigated by seismic surveys and drilled (Araújo & Castelo Branco, Reference Araújo and Castelo Branco2011).
The geological framework of the Rosa Magra stockwork zone was assessed on the basis of drill hole logging. Two drill holes were selected and sampled: PSC56 and PSC46 (Fig. 3b). These drill holes intersected the regional stratigraphic sequence, exhibiting successions and palynological ages that are very similar to those of the Semblana sector (Fig. 3, online Supplementary Table S1). No U–Pb zircon age determinations were undertaken in this area. Regarding the volcanism, it is worth noting the occurrence of felsic coherent rhyolites at the footwall of the Graça Formation, showing flow banding and spherulitic textures, occasionally with minor sedimentary intercalations (e.g. PSC56 hole, between depths 1060.9 and 1059.7 m). Here, the volcanic hanging-wall contact appears to be intrusive, which implies that its coeval character with the Graça Formation black shales is possible but needs to be positively demonstrated by absolute dating.
The Rosa Magra stockwork mineralization is hosted by rhyolites intercalated in the Neves Formation sediments of late Strunian age, and interpreted to correspond to a distal sulphide stringer zone, characterized by mild hydrothermal alteration (quartz and sericite alteration facies). The composition of the secondary phyllosilicate was not addressed in this study.
As in the Semblana deposit, the tectonic setting of the Rosa Magra stockwork zone consists of a parautochthonous package of tectonic thrust sheets affecting the PQ Formation and the Lower VSC units. The upper block comprises all the units of the Upper VSC, and both blocks are again separated by a structural corridor, reinforcing the correlation with the Semblana sector.
The Semblana deposit and the Rosa Magra stockwork zone consequently show a similar geological setting, the latter being envisaged as the northwards extension of the former. Favourable geological correlation between the Rosa Magra and the Corvo mine sector is also suggested (Fig. 2).
6. Zambujal – South Semblana sector
Located east of the Zambujal deposit and 350 m south of the Semblana deposit, the geological framework of this area was investigated via SU22 and PSS42A-2 drill holes (Fig. 7; see map location in Fig. 1). This sector, where the Neves–Corvo NE-dipping normal limb of the antiform is exposed, shows a complex tectonic setting characterized by several stacked thrust sheets.

Fig. 7. Geological section east of the Zambujal deposit and the Semblana south area, with projection of studied drill holes SU22 and PSS42A-2 (see location in Fig. 1). Along the drill holes, sample location with palynological assemblages or obtained U–Pb geochronological data.
At the base of the stratigraphic sequence, the upper section of the PQ and Corvo formations are dated as being of Famennian age (VH Miospore Biozone identified in shales of the PSS42A-2 drill hole). In drill hole SU22, the Neves Formation yielded a Strunian age (LN Miospore Biozone). The massive sulphide mineralization intersected in PSS42A-2 drill hole (917–919 m) is hosted by the Neves Formation black shales (Strunian age, established by correlation), occurring stratigraphically on top of felsic volcanic rocks with disseminated and stockwork sulphides that ntersected above the Corvo Formation pumice volcanic rocks. This setting is consistent with the geological interpretation proposed for the Semblana deposit (see Fig. 3a). It represents a basal piled-up repetition, limited by thrusts sheets and dominated by the Lower VSC sequence (Neves and Corvo formations and volcanic rocks) and PQ sediments (Fig. 7). An intermediate tectonically displaced block can also be considered, represented by the allochthonous Mértola Formation and the Upper VSC formations (Fig. 7). This interpretation is consistent with the palynological data defined by the identification of the NM Miospore Biozone of middle–late Visean age (PSS42A-2 samples) in the Godinho and Grandaços formations, and Visean-age sediments identified in the PSS42A-2 drill hole section at depths 470–810 m. On the top portion of the section an uppermost piled-up repetition is identified formed by the Upper VSC sequence formations. A normal upwards sequence is observed comprising the Upper VSC Grandaços Purple Shales, Godinho and Brancanes formations and the Mértola Formation flysch (including sediments cropping out). In PSS42-A drill hole of middle–late Visean age (NM Miospore Biozone) was identified in Grandaços, Godinho and Mértola formations.
A U–Pb 356.9 ± 3.5 Ma age was obtained from a very limited number of zircon grains separated from a felsic volcanic rock sample collected in hole SU22 (468 m; Figs 4j, 7). This age was obtained from three grains in a universe of six grains.
7. The Monte Branco deposit
Located in the Cerro do Lobo mine waste facility, approximately 1 km SE of the Zambujal deposit and 800 m south of the Semblana, the Monte Branco deposit was identified as being of interest by Somincor/Lundin Mining in the 1980s because of its geophysical anomalies (gravity, seismic and electromagnetic surveys). Drilling carried out by Somincor in 1983 and 1994 had already intersected a copper-rich stockwork zone and a small lens of massive sulphide mineralization grading 5.3% Zn. Further exploration in 2011/2012 by surface drill holes led to the identification of six small discontinuous and tectonically sheared ore lenses lying at depths ranging between 540 and 700 m and of a Cu-rich stockwork hosted in the Neves and PQ formations (Lundin Mining, 2017).
In this study, the interpretations of the stratigraphic relations and tectonic structure were based on the detailed logging of drill holes SD20, SCA26 and SDA44-1. The stratigraphic succession appears incomplete in all the drill holes as a consequence of strong tectonic repetition and imbrication (Fig. 6). In spite of these complications, although tectonically dismembered, all the regional lithostratigraphic units (except for the Graça Formation) were identified including the product of the two abovementioned felsic volcanic episodes (Fig. 5). Their lithologies and palynological ages are similar to those recorded in the Semblana and Rosa Magra sectors. The PQ Formation was dated late Famennian based on the Miospore VH Biozone assemblage (Fig. 6; online Supplementary Table S1).
Drill hole SCA26 intersected a few Lower VSC volcanic rocks as follows: a pumice breccia with U–Pb zircon age of 358.8 ± 2.1 Ma (16 out of 47 grains were used to calculate the concordia age, in a universe of 50 mounted grains), earliest Tournaisian, 854.6 m depth and 359.8 ± 1.9 Ma (concordia age was calculated from 37 grains in 70 sample results from 71 mounted zircons), late Famennian, 868.3 m depth (Fig. 4h, I; Table 2); rhyolite lavas (hyaloclastite envelope, locally with jigsaw-fit texture); and polymictic breccia. Inherited zircon grains in these two dated samples yield Upper Devonian (c. 366–378 Ma and c. 382 Ma, 15 and 2 grains, respectively), Lower Devonian (c. 395 Ma, 3 grains), Neoproterozoic (c. 573–626 Ma, 2 grains) and Mesoproterozoic (c. 1324 Ma, 1 grain) ages. In the same drill hole, a fine-grained Rhyolite type 3 was logged at 684.1 m depth of age 350.1 ± 1.5 Ma (22 grains were used to calculate concordia ages out of 36 U–Pb ages from 37 mounted zircons), late Tournaisian, with an Upper Devonian (c. 374 Ma), a Neoproterozoic (545 Ma) and Mesoproterozoic (c. 1024 Ma) inherited zircon grains (Figs 4g, 5; Table 2).
The massive sulphide orebody consists of two ore lenses, Monte Branco West (MBW) and Monte Branco East (MBE), both hosted by the Neves Formation (late Strunian age, Biozone LN; see Fig. 6; online Supplementary Table S1) and felsic volcanic rocks. The stockwork mineralization occurs within a PQ Formation tectonic slice that overthrusts the VSC felsic volcanic rocks.
The tectonic setting is dominated by the existence of several thrust sheets. The thrust planes correspond to shear zones, with tectonic transport to the SW. The structural complexity is demonstrated by the overthrust of PQ Formation sediments and associated stockwork over the VSC felsic volcanic rocks (drill hole SCA26 at MB West) and by the intensely sheared units (Fig. 6, SDA44-1 drill hole and insert). By using the two intercalations of shales and greywackes (flysch sediments ascribed to the Mértola Formation) as marker units (see Monte Branco East, Fig. 6), two main tectonic sheets can be recognized: the upper block, encompassing all the Upper VSC units in a normal stratigraphic sequence; and the lower block, which is considerably more disturbed. The two blocks are separated by a strongly sheared zone, interpreted to correspond to the upper limit of the Neves Structural Corridor. This structural setting mimics the tectonic framework previously described in the Neves–Corvo mine area (Reference Oliveira, Rosa, Rosa, Pereira, Matos, Inverno and AndersenOliveira et al. 2013b , 2016). These kilometres-long thrust fault zones have a regional character, and can also be positively identified in seismic surveys (Carvalho et al. Reference Carvalho, Inverno, Matos, Rosa, Granado, Branch, Represas, Carabaneanu, Matias and Sousa2016).
8. Geology of the Semblana, Rosa Magra and Monte Branco sectors in the context of the Neves–Corvo mine region
Despite the complex geological setting of the Semblana, Rosa Magra and Monte Branco mineralizations, their hosting VSC units share the lithological composition and ages of those of the Neves–Corvo mine region (only the Graça Formation is not represented in the Monte Branco sequence due to the intense tectonism). The sulphide mineralization in both Semblana and Monte Branco is massive and stockwork, whereas in Rosa Magra it is largely dominated by stockwork mineralization. In all cases it is also possible to palynologically identify stratigraphic gaps corresponding to the Upper Devonian LL and LE Miospore biozones and the early Carboniferous, Tournaisian VI, HD, BP and PC Miospore biozones, the latter corresponding to a sedimentary hiatus of c. 12–14 Ma between the Lower VSC and Upper VSC, consistent with what was described for the Neves–Corvo mine region (Oliveira et al. Reference Oliveira, Pereira, Carvalho, Pacheco and Korn2004, Reference Oliveira, Rosa, Rosa, Pereira, Matos, Inverno and Andersen2013b ). All these gaps may be related to the erosion of uplifted basin blocks in close association with extensional tectonism that affected the entire IPB during its early opening stage (Oliveira, 1990; Oliveira et al. Reference Oliveira, Pereira, Carvalho, Pacheco and Korn2004, Reference Oliveira, Relvas, Pereira, Matos, Rosa, Rosa, Munhá, Fernandes, Jorge, Pinho, Dias, Araújo, Terrinha and Kullberg2013a ).
The ages reported for the VSC rhyolite units (Rhyolites types 1, 2 and 3) intersected by drill holes at the Semblana and Monte Branco areas are shown as weighted average plots (Fig. 5). The obtained ages range from 349.5 ± 2.9 to 361.5 ± 2.5 Ma. A preliminary interpretation leads to the identification of two different age groups: an older age of c. 354–364 Ma and a younger age of c. 347–355 Ma, including outward error limits (Fig. 5). Weighted average filtering indicates respective ages of 359.6 Ma (late Famennian) and 350.9 Ma (late Tournaisian) as the approximate emplacement and/or crystallization ages for the two different volcanic events (Fig. 5).
The U–Pb zircon ages recorded in the studied sectors suggest long-lasting volcanic activity. As well as the emplacement/crystallization concordia ages, almost all samples exhibit antecrystic ages (e.g. PSN44). It is particularly clear that the youngest Tournaisian episode includes an older inheritance. In other words, the autocrystic zircons of the older volcanic episode or pulse appear to be part of the antecrysts population of the younger episode. This has implications for the interpretation of the tectonic setting, the spatial distribution of the volcanic centres and the evolution of volcanic activity through time. Inherited zircon ages also demonstrate that magmatism might have started at c. 395–390 Ma or even as early as c. 416 Ma, consistent with the age data recorded in other areas of the Neves–Corvo mine region (Solá et al. Reference Solá, Salgueiro, Pereira, Matos, Rosa, Araújo, Neto and Lains2015; Albardeiro et al. Reference Albardeiro, Morais, Solá, Matos, Rosa, Batista, Pacheco, Araujo, Inverno, Salgueiro, Marques, Mendes, Pereira and Oliveira2018).
Figure 8 is an overall chronostratigraphic chart, where all the lithostratigraphic units are included together with the corresponding palynological and U–Pb zircons ages obtained in this study. Straightforward correlation with the stratigraphy and volcanism of the Neves–Corvo mine region can be immediately established.
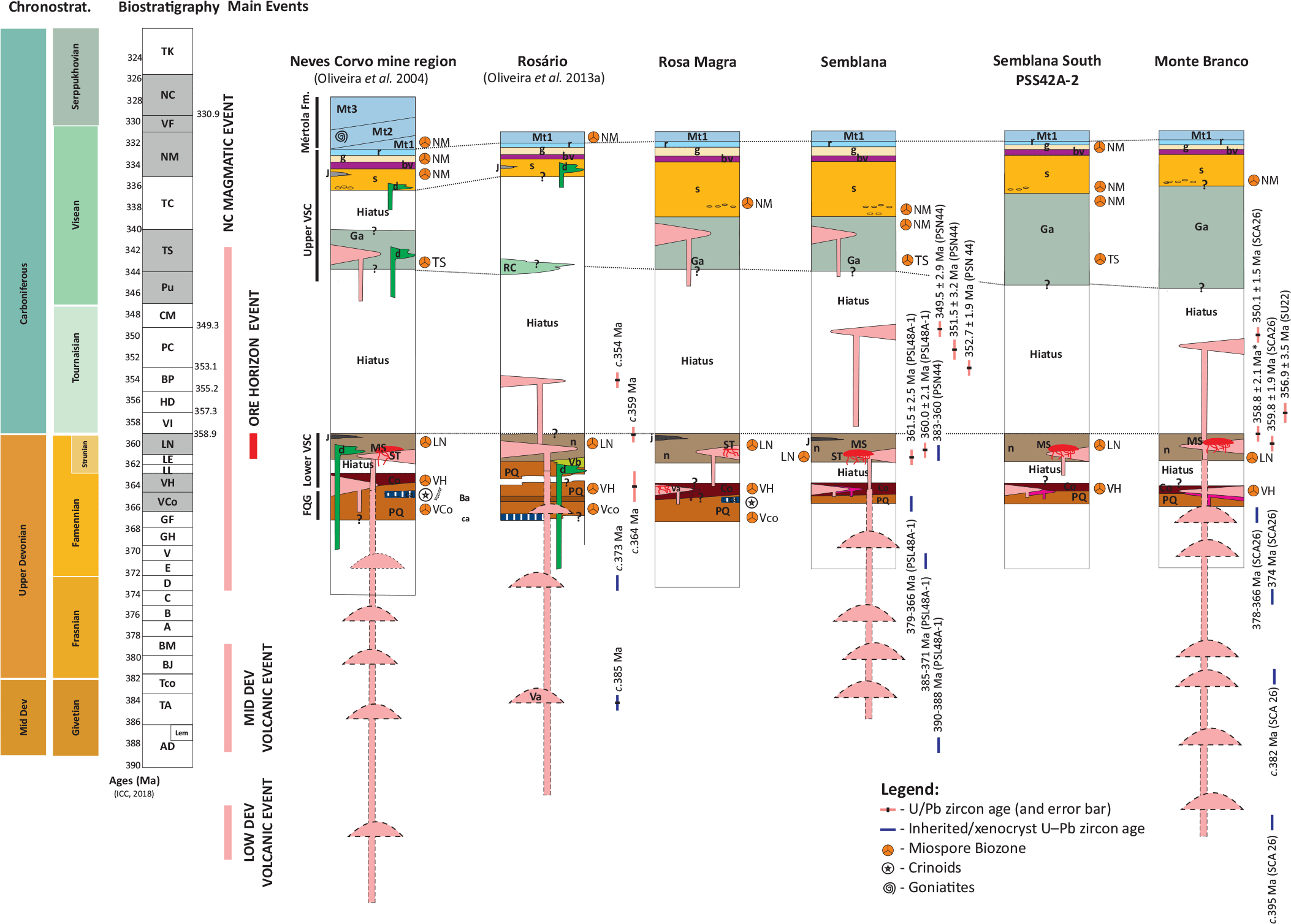
Fig. 8. Schematic representation of the overall current knowledge concerning the stratigraphy and volcanism of the Semblana, Monte Novo and Rosa Magra mineralizations in the general context of the Neves–Corvo mine region (Rosario–Neves–Corvo Antiform). The Devonian–Carboniferous boundary is at 358.9 ± 0.4 Ma according to the International Chronostratigraphic Chart 2020/01. Baixo Alentejo Flysch Group: MT – Mértola Formation (MT1, MT2 and MT3); Volcano-Sedimentary Complex: r – Brancanes Formation; g – Godinho Formation; bv – Borra de vinho Formation; s – Grandaços Formation; RC – Ribeira Cobres Formation; Va – felsic volcanic rocks; Vb – mafic volcanic rocks; MS – massive sulphides; ST – stockwork; n – Neves Formation; Co – Corvo Formation; d - dolerites; J - jaspers; PQ – Phyllite Quartzite Formation; Ba – Barrancão Member; ca – limestones.
Figure 9 depicts a schematic geological model for the Semblana and Rosa Magra sectors during Famennian time, when a horst and graben structure, controlled by deep faults, seems to have played a prime role. These graben structures may have functioned as lower-order basins, which would have favoured the deposition in anoxic conditions of the Neves Formation black shales. Additionally, the associated faults coupled with high heat flow conditions induced by the volcanism would have enabled hydrothermal fluid circulation responsible for the focusing of stockwork feeder zone and massive sulphide-forming processes. The felsic volcanism was certainly more abundant in the Semblana sector than in the Rosa Magra sectors, especially during the critical period of 360–359 Ma. The hydrothermal flow represented in Figure 9 is conceptual and represents fluid percolation along VSC and PQ basement rocks. The proposed scenario is based on previous research on the Neves–Corvo VSC hydrothermal event (Relvas et al. Reference Relvas, Tassinari, Munhá and Barriga2001, Reference Relvas, Barriga, Ferreira, Noiva, Pacheco and Barriga2006) and PQ sequence leaching models (Relvas et al. Reference Relvas, Tassinari, Munhá and Barriga2001; Jorge et al. Reference Jorge, Relvas and Matos2006; Rosa et al. Reference Rosa, McPhie, Relvas, Pereira, Oliveira and Pacheco2008; Tornos & Heinrich, Reference Tornos and Heinrich2008). The volcanic activity at c. 350 Ma (Rhyolite type 3, Figs 5, 9) is also represented by sericitic hydrothermal alteration and sulphide dissemination (Semblana), mainly along cleavage planes. Admittedly, this younger (c. 350 Ma) volcanic activity could have played a role in the Neves–Corvo metallogenesis, in terms of either magmatic heat, hydrothermal fluid and/or metal contributions, or simply as a driver of syn-tectonic sulphide remobilization (Solá et al. Reference Solá, Salgueiro, Pereira, Matos, Rosa, Araújo, Neto and Lains2015; Albardeiro et al. Reference Albardeiro, Solá, Salgueiro, Morais, Matos, Mendes, Batista, Pereira, Inverno, Oliveira, Rosa and Pacheco2017). The preliminary data related to the Semblana and Rosa Magra sectors suggest that this younger hydrothermal episode was far less developed than that associated with the Strunian age mineralization event.

Fig. 9. Conceptual geological model for the Semblana deposit and Rosa Magra mineralization, during late Famennian – Tournaisian time.
The Semblana–Rosa Magra high-resolution stratigraphic model can be correlated with other IPB volcanic and sedimentary sequences where VMS deposits are present. In Portugal, the studied setting defined by Neves Formation Strunian-age black shales and late-Famennian-age felsic volcanism can be correlated with the dark-grey to black shales and Famennian-age felsic volcanic rocks defined at Lousal and Caveira mines (Matos et al. Reference Matos, Pereira, Rosa, Rosa, Oliveira and Relvas2011, Reference Matos, Pereira, Rosa and Oliveira2014; Dias et al. Reference Dias, Oliveira, Matos, Ressurreição, Pereira, Machado, Pais and Manupella2016; Oliveira et al. Reference Oliveira, Quesada, Pereira, Matos, Solá, Rosa, Albardeiro, Díez-Montes, Morais, Inverno, Rosa, Relvas, Quesada and Oliveira2019). These mines and Neves–Corvo are located in the same VSC NW–SE-aligned lineament that includes other deposits such as Montinho and Sesmarias, which is one of the key mineral exploration areas in the Portuguese IPB sector (Matos et al. Reference Matos, Carvalho, Represas, Batista, Sousa, Ramalho, Marques, Morais, Albardeiro, Gonçalves and Dias2020). In Spain, similar models have been proposed for the Herrerias, Tharsis and Aznalcollar mine sites located in the southern and eastern IPB areas (Saez et al. Reference Saez, Moreno and González2011; Almodóvar et al. Reference Almodóvar, Yesares, Sáez, Toscano, González and Pons2019). In the latter, the local setting is dominated by stacking between thrust fault zones and structures, conditioned by significant S–SW vergence (Almodóvar et al. Reference Almodóvar, Sáez, Pons, Maestre, Toscano and Pascual1998). This tectonic setting is similar to the studied section of Semblana–Rosa Magra and Monte Branco.
9. Concluding remarks
The main results from this research are as follows:
-
1. The newly studied sectors, Semblana–Rosa Magra and Monte Branco show a stratigraphic sequence similar to that previously defined for the Neves–Corvo mine region.
-
2. Geological sections supported by geological logging, palynostratigraphy of sediments and geochronology of felsic volcanic rocks are presented, showing a more complex structural setting defined by thrusting and duplexing as observed in the Neves–Corvo NE-dipping normal limb.
-
3. The sulphide mineralization in both Semblana and Monte Branco is massive, occurring in small lenses and stockwork, whereas in Rosa Magra it is largely dominated by stockwork mineralization.
-
4. Although only the Semblana deposit has economic potential (Cu ore grades) depending on further exploitation, the present study suggests a favourable geological correlation to the west, between the Rosa Magra stockwork and the Corvo mine sector.
-
5. The VSC felsic volcanic units (Rhyolite types 1, 2 and 3) zircon ages range from 349.5 ± 2.9 to 361.5 ± 2.5Ma, and two main volcanic events were proposed at 359.6 Ma (late Famennian) and 350.9 Ma (late Tournaisian) based on the weighted average of ages.
-
6. The main stage of massive sulphide deposition took place during late Strunian time (c. 360.0–361.5 Ma), clearly associated with the black shales of Neves Formation and rhyolites of the older volcanic event (359.6 ± 1.6 Ma), implying that felsic volcanism, mineralization and shale sedimentation are essentially coeval.
-
7. Stratigraphic gaps in the palynological record occur within the Strunian (Upper Devonian) and in the Tournaisian (c. 12–14 Ma) strata, between the Lower and Upper VSC sequences, reflecting probably erosion and uplift mechanisms linked with extensional tectonics. Nevertheless, the younger volcanic event exclusive of Rhyolite type 3 is of late Tournaisian age (350.9 ± 2.3 Ma).
-
8. The younger volcanic activity at c. 350 Ma is also represented by rhyolites with sericitic hydrothermal alteration and sulphide dissemination (Semblana), mainly along cleavage planes. Although apparently barren, this volcanism could also have played a role in the Neves–Corvo metallogenesis, either in terms of magmatic heat, hydrothermal fluid and/or metal contributions, or simply as a driver of syn-tectonic sulphide remobilization.
-
9. A schematic geological model is proposed, where horst/graben structures hosted anoxic sediment deposition and associated massive sulphides, which ore-forming processes might have been triggered by the high heat flow induced by the felsic volcanism during Famennian–Tournaisian times.
Acknowledgements
The research was sponsored by AGC-Lundin Mining/LNEG project IPBVECTORS (Geologic, stratigraphic and lithogeochemical characterization of the geological units of the Algaré structure, Rosário Antiform and the Semblana massive sulphide mineralization) and by the EXPLORA project (Definition of new geological, geophysical and geochemical knowledge vectors applied to Neves-Corvo northern region), Op ALT20-03-0145-FEDER-000025, funded by Alentejo 2020, Portugal 2020 and European Union (ERDF). We thank Lundin Mining for access to the corporate geochemical databases. Somincor/Lundin Mining and AGC consultants Tim Barrett and Brian Marten are acknowledged for fruitful discussions and constructive reviews. We are grateful to Scott Napier, Bob Carmichael and the exploration team on the discovery of Semblana deposit. We also thank Professor Ulf Linnemann and Andreas Gartner (Geochronology Lab, Dresden Museum, Germany) for the zircon geochronological study supervision. We also thank João Fernandes, Rogério Raposo and Rosa Irene Sousa for sample preparation and laboratory support at LNEG facilities. Editors, Professor Jorge Relvas and an anonymous referee are acknowledged for valuable comments that improved the manuscript.
Supplementary material
To view supplementary material for this article, please visit https://doi.org/10.1017/S0016756820001284