1. Introduction
The geochemical analyses of basaltic rocks, integrated with a gamma-spectrometric (scintillometric) survey, yield significant information regarding the stratigraphy and correlation of lavas in continental flood basalts. This is of particular significance in mineralized volcanic rocks, such as the Paraná volcanic province of southern Brazil and northern Uruguay (Figs 1–3) of Cretaceous age, because of their world-class deposits (e.g. Schmitt, Camatti & Barcellos, Reference Schmitt, Camatti, Barcellos, Schobbenhaus, Queiroz and Coelho1991) of amethyst- and agate-bearing giant geodes (up to 4 m in height). These occur in the basalts and andesites of the Serra Geral Formation (Brazil) and Arapey Formation (Uruguay) from the base to the top of the volcanic stratigraphy (Gilg et al. Reference Gilg, Morteani, Kostitsyn, Preinfalk, Gatter and Strieder2003; Proust & Fontaine, Reference Proust and Fontaine2007; Hartmann, Reference Hartmann2008; Duarte et al. Reference Duarte, Hartmann, Vasconcellos, Medeiros and Theye2009; Morteani et al. Reference Morteani, Kostitsyn, Preinfalk and Gilg2010; Commin-Fischer et al. Reference Commin-Fischer, Berger, Polvé, Dubois, Sardini, Beaufort and Formoso2010). In other provinces, such as the Columbia River (Mangan et al. Reference Mangan, Wright, Swanson and Byerly1985; Milner et al. Reference Milner, Wright, Swanson and Byerly1986) and Etendeka (Marsh & Milner, Reference Marsh and Milner2007), geochemistry has been used to establish stratigraphic correlation in basalts. In the African (Etendeka) portion of the Paraná volcanic province, Milner et al. (Reference Milner, Duncan, Whittingham and Ewart1995) observed that ‘geochemistry can be used as a primary criterion for the correlation of major quartz latite units. . .’. In the Paraná volcanic province, Peate et al. (Reference Peate, Hawkesworth, Mantovani, Rogers and Turner1999) established the geochemical correlation of basalts and rhyodacites in the Urubici region, Santa Catarina state.

Figure 1. Geological map of the Serra Geral Formation of basaltic and rhyodacitic lavas. Study area indicated. Modified from Peate, Hawkesworth & Mantovani (Reference Peate, Hawkesworth and Mantovani1992).

Figure 2. Geological map of the Quaraí mining district in southernmost Brazil, Los Catalanes gemological district in northwestern Uruguay and surrounding region. Modified from the Geological Survey of Brazil (CPRM) map of Rio Grande do Sul. Location of boreholes, borehole section (A–B) and analysed samples indicated. Sample numbers are preceded by WW (e.g. 181 on the map corresponds to field number WW181).

Figure 3. Location of analysed samples in a detailed area. Correspondence between sample number in this figure and the field number: 1 = G1525, 2 = G1541, 3 = X2, 4 = X1, 5 = G1540, 6 = G1574, 7 = GARVI, 8 = LV89A, 9 = BR3, 10 = WW196, 11 = 197, 12 = BR4, 13 = BR7, 14 = LB2, 15 = LB5, 16 = BR10, 17 = B14, 18 = LB16, 19 = LB24, 20 = PB2, 21 = D5A, 22 = D5B, 23 = PT19B, 24 = PT19A, 25 = PT18, 26 = D41, 27 = PT17, 28 = PT20, 29 = D44, 30 = G1513, 31 = G12, 32 = G10, 33 = N1A, 34 = N5, 35 = D4A, 36 = LV4, 37 = LV14, 38 = E23, 39 = LV9, 40 = MCG, 41 = EG9, 42 = LV31, 43 = LV27, 44 = LV53, 45 = LV48, 46 = BU1, 47 = BU4, 48 = CTA, 49–60 = PA21-PA31, 61–65 = PA32-PA36, 66–67 = PA19-PA20, 68–71 = PA15-PA18, 72 = PA43, 73 = PA42, 74–80 = PA37–43, 81 = WW203.
Following detailed field surveys, we investigated the geochemical and scintillometric (total gamma) properties of the six tholeiitic, basaltic lavas that overlie the eolian sandstones of the Botucatu Formation in the Quaraí mining district (Brazil) and the Los Catalanes gemological district (Uruguay). Two of the flows contain world-class amethyst and agate geode deposits in Uruguay (Figs 4, 5), and similar deposits may also occur in Brazil across the border along the Rio Quaraí. Total production is approximately 600 tons per month in 400 active, underground mines in both districts. Two of the four flows were studied in more detail than the others because they are mineralized and are the most extensive flows exposed at the surface. This is the first time a long-distance correlation is attempted in the Paraná volcanic province using lava internal structure, scintillometry and geochemistry. We also evaluate the evolution of volcanism in the studied Quaraí-Los Catalanes region in the more general scenario of the Paraná volcanic province.

Figure 4. Model section of colada Catalán.

Figure 5. Model section of colada Cordillera. Location of analysed samples indicated.
2. Methodology
Three different field surveys were done for this study, all involving the observation of satellite images and the description of outcrops and rock thin-sections under the microscope. The Geological Survey of Brazil (CPRM) and the Japan Mining Engineering Center for International Cooperation made reconnaissance studies on the Brazilian portion of the studied area and on the Serra Geral Formation in general (Nakamura et al. Reference Nakamura, Wildner, Shibuya, Masuta, Murakami and Romanini2003), while L. C. Duarte (unpub. Ph.D. thesis, Univ. Federal do Rio Grande do Sul, Brazil, Reference Duarte, Hartmann, Vasconcellos, Medeiros and Theye2009) and Duarte et al. (Reference Duarte, Hartmann, Vasconcellos, Medeiros and Theye2009) focused studies in the Los Catalanes gemological district. A third field survey was done during 2008 and 2009, when geophysical (scintillometric) surveys were made with careful stratigraphic control. The portable gamma spectrometer used was a GR-320 enviSPEC from Exploration Radiation Detection Systems. The total scintillometric contributions of K, U and Th (and possibly other elements) were measured. A large number of sites were surveyed with the scintillometer with emphasis on the Quaraí mining district (n = 190), but reconnaissance work was also done in Los Catalanes gemological district (n = 94) for regional control. Duarte et al. (Reference Duarte, Hartmann, Vasconcellos, Medeiros and Theye2009) made detailed electron microprobe studies and back-scattered electron imaging of Los Catalanes rocks. Uruguay's national mining and geology board (DINAMIGE) offered advice on field problems in the gemological district.
Samples collected in the three studies (Figs 2, 3) were analysed for major and trace elements in the ACME laboratory, Canada, using standard analytical procedures named ‘Group 4A’ and ‘Group 4B’ by ACME. Group 4A analyses used LiBO2 fusion/dilute nitric digestion then ICP-ES for major oxides plus minor elements; LOI is weight loss on ignition. Group 4B analyses of rare earths and refractory elements used ICP-MS. Several rock samples from the same flow were analysed to understand internal variability regarding both volcanism and hydrothermal alteration. The total number of samples analysed was 124, corresponding to coladas Mata Olho (16), Catalán (23), Cordillera (52), Muralha (22), UR-13 (4) and Coxilha (7). (Colada is the Spanish word for flow.) Samples were collected mostly from the cores of lavas, but some samples from amygdaloidal crusts were analysed to test for the presence of blocky flows.
Nineteen groundwater exploration boreholes drilled and scanned with a gamma-spectrometer by the Geological Survey of Brazil (CPRM) were selected for inclusion in this study to help decipher the stratigraphy of the lava flows in the region. Ten boreholes are shown in the figures.
3. Overview of the Paraná volcanic province
One of the largest continental flood basalt provinces in the world, the Cretaceous (c. 135 Ma: Renne et al. Reference Renne, Ernesto, Pacca, Coe, Glen, Prévot and Perrin1992; Wildner et al. Reference Wildner, Santos, Hartmann and McNaughton2006) Paraná volcanic province covers 120000 km2 (Bellieni et al. Reference Bellieni, Comin-Chiaramonti, Ernesto, Melfi, Pacca and Piccirillo1984) or only 917000 km2 (Frank, Gomes & Formoso, Reference Frank, Gomes and Formoso2009) in southern Brazil, Uruguay, Argentina and Paraguay. The total volume of lavas extruded and preserved was estimated by Frank, Gomes & Formoso (Reference Frank, Gomes and Formoso2009) at 450000 km3 with an additional volume of 122000 km3 in sills. The Serra Geral Formation has a maximum preserved thickness of 1700 m at the depocentre of the Paraná basin (Zalán et al. Reference Zalán, Wolff, Conceição, Marques, Astolfi, Vieira, Appi, Zanotto, Raja-Gabaglia and Milani1990), situated 300 km north of Ametista do Sul. Each lava unit has a thickness between 5 and 80 m (average 20 m; Peate et al. Reference Peate, Hawkesworth, Mantovani, Rogers and Turner1999) and the total number of preserved lava flows is close to 120. In a carefully measured sequence of 16 basalt lava flows along the Serra do Rio do Rastro cuesta on the eastern border of the volcanic group in the Atlantic coast (T. C. Vargas, unpub. Doctoral thesis, Univ. Federal Fluminense, Rio de Janeiro, 2006), the total lava thickness is 700 m.
The Serra Geral Formation is at the top of the Palaeozoic to Cretaceous Paraná basin, which has a total thickness of 7500 m. The climate remained arid throughout the volcanic event (Peate, Reference Peate, Mahoney and Coffin1997); desert conditions prevailed during the formation of the Botucatu Formation below the lavas (Scherer, Reference Scherer2000, Reference Scherer2002; Scherer & Lavina, Reference Scherer and Lavina2006; Holz, Soares & Soares, Reference Holz, Soares and Soares2008) and during the deposition of the Bauru Formation above the lavas (Paula-e-Silva, Kiang & Caetano-Chang, Reference Paula-e-Silva, Kiang and Caetano-Chang2009). Evidence of lava imprint on the surface of sand dunes is observed in the Quaraí region, similar to other exposures in the province (Petry et al. Reference Petry, Jerram, Almeida and Zerfass2007; Waichel et al. Reference Waichel, Lima, Lubachesky and Sommer2006; Waichel, Scherer & Frank, Reference Waichel, Scherer and Frank2008).
Rock types in the Serra Geral Formation are tholeiitic, bimodal basic-acid and include basalt, basaltic andesite, andesite, dacite, trachyte, rhyodacite and rhyolite, here referred to simply as basalt and rhyodacite, the two most abundant rock types in the volcanic province (basaltic andesite is also abundant). The acid lavas occur as a 200 m thick sequence exposed near the Atlantic coast and dip to the northwest, so they are present at depth in the depocentre. A small volume of cordierite dacites occurs in Jaguarão, Rio Grande do Sul, approximately 100 km east of Quaraí; their age is between 157±5 Ma and 139.6±7.4 Ma (Comin-Chiaramonti et al. Reference Comin-Chiaramonti, Riccomini, Slejko, De Min, Ruberti and Gomes2010), so they may be coeval with the earliest Serra Geral Formation lavas (138–126 Ma).
Both basic and acid lavas belong to either high-Ti (commonly >2 wt% TiO2) or low-Ti (commonly <2 wt% TiO2) groups (Peate, Hawkesworth & Mantovani, Reference Peate, Hawkesworth and Mantovani1992). High-Ti rocks (Pitanga, Urubici, Paranapanema and Ribeira types) are common in the northern portion of the Serra Geral Formation and low-Ti rocks (Gramado, Esmeralda) in the southern portion. Although Gramado and Esmeralda-type lavas are abundant at the base of the volcanic stratigraphy, some occurrences are registered in the northern portion of the group. Gramado-type lavas constitute 5–10 vol.% of the volcanic formation, the third most voluminous chemical type. Overall, low-Ti lavas occur at the base, followed by high-Ti lavas (>3 wt% TiO2, e.g. Pitanga-type) which are capped by intermediate-Ti lavas (2–3 wt% TiO2). Low-Ti and high-Ti lavas occur intercalated in a few places, for example, the Serra Geral escarpment along the Atlantic Ocean coast in southern Brazil (Mantovani et al. Reference Mantovani, Marques, Sousa, Civetta, Atalla and Innocenti1985; Peate, Hawkesworth & Mantovani, Reference Peate, Hawkesworth and Mantovani1992; T. C. Vargas, unpub. Doctoral thesis, Univ. Federal Fluminense, Rio de Janeiro, 2006).
NW-oriented, vertical feeder dykes up to several hundred metres thick are abundant in the Ponta Grossa arch and also occur along the margin of the volcanic group in the Paraná basin and in the Precambrian basement (e.g. Rocha, Marques & Figueiredo, Reference Rocha, Marques and Figueiredo2005; Marques et al. Reference Marques, Ernesto, Piccirillo, Bellieni, Figueiredo and Min2005; Nardy et al. Reference Nardy, Squisato, Machado and Oliveira2005; Machado et al. Reference Machado, Nardy, Squisato and Oliveira2005). Both high-Ti and low-Ti dykes are found, commonly equivalent to nearby lavas. Most dykes and sills are basaltic but some are acid.
We focus our interest in this investigation in the basal portion of the Serra Geral Formation, specifically the Alegrete facies, which contains several basalt to andesite lava flows in the southern portion of the Paraná basin (Table 1). All lavas in the region are known to be low-Ti, Gramado-type, although Turner et al. (Reference Turner, Peate, Hawkesworth and Mantovani1999) mentioned the presence of Paranapanema (intermediate-Ti) below Gramado-type lavas in a borehole in the town of Artigas. We describe the Alegrete facies on either side of the international border and question the interpretation of Turner et al. (Reference Turner, Peate, Hawkesworth and Mantovani1999) on the diachronous effusion of different lava types in the region.
Table 1. Selected geological and geochemical characteristics of Quaraí mining district (Brazil) and Los Catalanes gemological district (Uruguay)

4. Field description
The pampas of the border region of Brazil and Uruguay are grasslands with only limited forests along Rio Quaraí and its tributary creeks. Rivers and creeks flow into Rio Uruguay, which runs from north to south at a distance of 100 km from Quaraí. The low-lying hills have typical elevations of 100–300 m above sea level, but are as low as 50 m along the Rio Uruguay. The climate is sub-tropical temperate, with average temperature between 0 and 10°C in winter and 25–35°C in summer; rainfall is approximately 1300 mm/yr, distributed evenly throughout the year.
Soil cover is 0.5–1.0 m thick and geological units are poorly exposed. Most outcrops are isolated, but continuous rock exposures occur along creeks. Colada Catalán is well exposed in road cuts along the BR-293 highway, where its internal structure can be fully observed.
The Botucatu Formation underlies the volcanic sequence and is made up of eolian sandstones formed in a large erg in the Cretaceous, possibly the largest erg in Earth history (Scherer, Reference Scherer2000). These sandstones and the underlying Guará Formation sandstones constitute the huge Guarani aquifer (Araújo, França & Potter, Reference Araújo, França and Potter1999; Soares, Soares & Holz, Reference Soares, Soares and Holz2008). The water table in the desert was probably near the surface as in the Sahara and Kalahari deserts today, so sealing by a lava flow very likely filled the aquifer in a short time, perhaps thousands of years. Filling of the erg's topographic basin (widespread ergs occur in topographic lows) with lavas sealed the aquifer and residual volcanic heat caused the intrusion of dykes and sills of liquefied sand with the formation of sand volcanoes and flows on top of the lavas. Clastic sand dykes and sills are common in the volcanic sequence (e.g. Perinoto et al. Reference Perinoto, Etchebehere, Simões and Zanardo2008), presumably as a result of fluidization processes similar to those proposed by Hurst, Cartwright & Duranti (Reference Hurst, Cartwright, Duranti, Rensbergen, Hills and Maltman2003) and Cartwright (Reference Cartwright2010).
The total thickness of the six lavas reaches 100–150 m in most of the region (Fig. 6), although Turner et al. (Reference Turner, Peate, Hawkesworth and Mantovani1999) directly observed lavas 400 m thick, particularly in borehole DDH210 outside the mining district. Total lava thickness attains almost 300 m in one borehole on the Brazilian side of the border (Fig. 7), although it is less than 100 m in most of the region (Fig. 6). A model geological section displays the variable thickness of the lavas (Fig. 8).

Figure 6. Lithological and scintillometric logs of boreholes that reached down into the Guarani aquifer; data from the Geological Survey of Brazil (CPRM), year 1986. Borehole numbers shown (e.g. IT-5).

Figure 7. (a) Lithological and scintillometric log of borehole UR-13; data from the Geological Survey of Brazil (CPRM), year 1986. (b) K2O v. Th+U of studied lava flows; clear correspondence with scintillometric measurements.

Figure 8. Model geological section of six lava flows from the studied region.
Five lavas (coladas Mata Olho, Catalán, Cordillera, Muralha and Coxilha) were recognized in the field and positioned in correct stratigraphic succession. Colada UR-13 was positioned between coladas Muralha and Coxilha based on scintillometric results from borehole UR-13 (Fig. 7); it was not observed in the field in this study, although outcrop samples were analysed by Nakamura et al. (Reference Nakamura, Wildner, Shibuya, Masuta, Murakami and Romanini2003).
The intermediate-Ti (~2.5 wt% SiO2) Paranapanema rocks identified by Turner et al. (Reference Turner, Peate, Hawkesworth and Mantovani1999) as flows below the low-Ti lavas are actually intrusive sills in the sedimentary rocks underlying the Serra Geral Formation volcanics (description by DINAMIGE, unpub.). The stratigraphy of borehole 410 is as follows: 0–36 m = basalt flow; 36–321 m = Botucatu Formation and underlying sandstones; 321–400 m = basaltic sill; 412–652 m = Permian sandstones; 652–783 m = basaltic sill; 783–1687 m = Permo-Carboniferous sandstones. This is consistent with surface geology, because the Botucatu Formation sandstones are exposed in Artigas town, close to the location of the borehole.
This is an important point because the main issue of Turner et al. (Reference Turner, Peate, Hawkesworth and Mantovani1999) was that near Quaraí the apparent stratigraphic relationship between the Paranapanema and Gramado types is reversed compared to elsewhere in the Paraná volcanic province. Because the Paranapanema rocks are now correctly identified as sills, no diachronous geochemical stratigraphy is registered in the region.
Colada Catalán directly overlies the sandstones in the basaltic cuesta situated in the northeastern Los Catalanes gemological district, but regionally it is the second lava (Figs 6, 7). In the Quaraí mining district, colada Mata Olho is observed to underlie colada Catalán in several boreholes (Fig. 6). Along the BR-293 highway, coladas Mata Olho, Catalán and Cordillera overstepped the sand dunes in palaeohighs as shown by the evidence of the direct contact of each lava with the Botucatu Formation sandstone. The added thickness of the three lavas indicates an estimated 100 m elevation for the Cretaceous dune (Fig. 8). This is comparable to the height of some of the modern dunes in the Kalahari desert.
The lava flows are not horizontal in the Los Catalanes gemological district because they display a basinal dip (~3°) to the north, but they are nearly horizontal in the Quaraí mining district. The lava sequence was presumably upwarped along the Rio Grande arch, whose NW-directed axis runs through Alegrete (e.g. CPRM, 2009). Many strike-slip faults cut the basaltic association in both mining districts and cause horizontal displacements of undetermined extent. The presence of a 300 m thick lava sequence in borehole UR-13 (Fig. 7), located close to thinner (<100 m) lavas requires the occurrence of nearly 200 m vertical displacements. Similar strike-slip faults and comparable vertical displacements were measured in the Ametista do Sul region, situated 300 km to the north of Quaraí, with the use of geochemical correlation of lava flows (Pinto, Hartmann & Wildner, Reference Pinto, Hartmann and Wildner2010). Nevertheless, lateral continuity of individual lavas can be observed for tens of kilometres in the Quaraí–Los Catalanes districts.
The most siliceous units (≥56 wt% SiO2, colada Catalán and colada Muralha) have the structure of aa lavas (Fig. 4), particularly the voluminous and extensive, contorted igneous layering and autobreccias. This structure is particularly well-displayed in colada Catalán; colada Muralha also shows structures of aa lava but this colada covers a smaller portion of the studied region. Similar structures were described in aa lavas by Peterson & Tilling (Reference Peterson and Tilling1980), Kilbum (Reference Kilbum1981, Reference Kilbum and Fink1990), Rowland & Walker (Reference Rowland and Walker1990), Hon, Gansecki & Kauahikaua (Reference Hon, Gansecki and Kauahikaua2003) and Bondre & Hart (Reference Bondre and Hart2008). The presence of well-exposed aa lavas in the Paraná volcanic province is here registered for the first time. The least siliceous lavas such as colada Mata Olho and colada UR-13 have the structure of pahoehoe lavas, including the absence of brecciated crusts, but poor exposure prevents more detailed description.
Colada Mata Olho was observed in some drill holes as the basal unit in direct contact with the Botucatu Formation sandstones in the Itaqui and Alegrete regions (Figs 6, 7). Integrated field work with scintillometric measurements led to the identification of colada Mata Olho in a few windows in regions covered by coladas Catalán and Cordillera. Colada Mata Olho is overlain by colada Cordillera in the Mata Olho Creek near the BR-293 highway where colada Catalán is missing. In other places, colada Catalán and colada Cordillera are in direct contact with the eolian sandstones. The thickness of colada Mata Olho varies between 10 and 15 m, as measured in four boreholes (Figs 6, 7).
Colada Catalán was given special attention because it has the structure of aa lava (Fig. 4); it has locally a basal crust and a massive core, and everywhere an upper crust and typical contorted flow banding over large distances. The crusts are made up of breccias of vesiculated lava, whereas the core has elongated amygdales. The core is thick and contains the amethyst geode deposits of colada Catalán in the Los Catalanes gemological district and in Garimpo do Vivi (Fig. 2), Quaraí mining district. Colada Catalán and colada Cordillera are present in all nine boreholes except IT-08 and IT-05 (Fig. 6), where they were probably eroded or not deposited.
An impressive 1–3 m thick autobreccia, formed during lava emplacement, marks colada Catalán in its upper crust and along most of its 50 × 50 km observed extent (possibly 200 km), with angular blocks and variable cement, as first described in Garimpo do Vivi by Augustin (Reference Augustin2007). The breccia blocks are locally immersed in silicified sandstone stockwork, which is invariably in contact with a 0.5–2.0 m thick, silicified sandstone at the top. Autobreccia lacking sandstone is more abundant and has no sandstone layer on top; the upper contact where observed is with colada Cordillera. Colada Catalán has contorted, igneous flow banding in the upper (below the breccia zone) and lower crusts. Breccia portions are observed in lateral contact with massive lava in vertical sections along the BR-293 highway as part of the same flow. The flow is approximately 30–70 m thick (Figs 6, 7) and its upper breccia portion has a distinctive appearance both in satellite images and in the field, because it consists of 100–300 m wide, 1000–2000 m long, ribbons of light grey (in satellite images) rocks alternating with green (in satellite images) grassland portions. This oustanding feature extends for more than 50 × 50 km into Brazil and possibly over an area of 200 × 100 km. The exposed rock is either a breccia or massive, flow-banded rock. The breccia is composed of angular blocks (10–50 cm large) and is clast-supported, mostly with no visible cement. On the Brazilian side of the border, a chalcedony or calcite cement is present in some places.
Colada Cordillera (20–60 m thick, Fig. 6) hosts the world-class amethyst geode deposits in the Los Catalanes gemological district, but no mineralization is known on the Brazilian side of the border. The structure of the mineralized flow (Fig. 5) consists of upper (10 m thick) and lower (5 m thick) amygdaloidal crusts with a massive core divided into two portions. The upper part of the core is 20 m thick, displays columnar jointing and is not mineralized, whereas the lower part (10 m thick) is not jointed and contains the large deposits of geodes. This structure was described in the field and confirmed by chemical analyses of the rocks and scintillometric measurements. This is a novel type of lava structure previously unknown in the Paraná volcanic province.
Colada Muralha is approximately 30–60 m thick and is mostly massive with amygdaloidal lower and upper crusts with a massive core (Fig. 6). It has the structure of aa lava, but was less studied than coladas Catalán and Cordillera because it covers a smaller area. Colada Muralha is exposed at the surface in four boreholes (Fig. 6) and was probably eroded in the others.
Colada UR-13 was first identified in borehole UR-13 (Fig. 7) which drilled all six lavas, and was then confirmed by chemical analyses of outcrop samples. Its thickness is near 60 m in the borehole (Fig. 7).
Colada Coxilha was little studied because it only occurs in one hill in the northern part of the region and in borehole UR-13 (Fig. 7); it is approximately 60 m thick and has amygdaloidal lower and upper crusts. Because colada Coxilha caps the hills in the region, the upper amygdaloidal crust has been mostly removed by erosion.
Coladas Catalán and Cordillera were strongly altered in their mineralized portions due to percolation of hydrothermal fluids. These fluids originated in the Guarani aquifer and are nearly pure water (Krüger et al. Reference Krüger, Gilg, Taubald and Frenz2009; Morteani et al. Reference Morteani, Kostitsyn, Preinfalk and Gilg2010). The highest temperature (~130 °C) attained by the fluids is delimited by the abundant presence of clinoptilolite, a zeolite, widespread in Los Catalanes mines and identified by stable isotopes (Morteani et al. Reference Morteani, Kostitsyn, Preinfalk and Gilg2010). In the Quaraí mining district, this alteration was caused by similar fluids, causing the filling of vesicles (now amygdales) with zeolites, although intense replacement of zeolites by silica minerals is also observed.
Hydrothermal alteration of all mineralized flows is concentrated in pigeonite and less so in augite; plagioclase is little altered (Duarte et al. Reference Duarte, Hartmann, Vasconcellos, Medeiros and Theye2009). Hydrothermal minerals include clays (celadonite, smectites) and zeolites, as determined by X-ray diffraction and electron microprobe analyses (Duarte et al. Reference Duarte, Hartmann, Vasconcellos, Medeiros and Theye2009).
5. Geochemical results
Six different lava flows (including two aa lavas) are identified in the study area from field stratigraphy and systematic rock geochemistry (Figs 9, 10). Selected chemical compositions are shown in Tables 2, 3; all 124 analyses are shown in Appendix 1 (see online appendix at http://www.cambridge.org/journals/geo).

Figure 9. Geochemical composition of lavas from the Quaraí mining district and Los Catalanes gemological district. (a) TAS classification; inset shows chemical composition of 355 volcanic rocks from the Paraná province (Nakamura et al. Reference Nakamura, Wildner, Shibuya, Masuta, Murakami and Romanini2003). (b) TiO2 v. P2O5 diagram; black ellipse (arrow) indicates composition of Los Catalanes gemological district surface samples from Turner et al. (Reference Turner, Peate, Hawkesworth and Mantovani1999); black filled symbols (Cat) are from Los Catalanes gemological district, unfilled (Qua) are from Quaraí mining district. (c) MgO v. TiO2 diagram indicating the low-TiO2 compositional type of the lavas. (d) TiO2 v. Zr diagram; (e) Ti/Zr v. Ti/Y diagram; three samples (within black circle) are sills from a drill core in the town of Artigas (Turner et al. Reference Turner, Peate, Hawkesworth and Mantovani1999). (f) Ba/Nb diagram; Pi – Pitanga, high-Ti lava; Pa – Paranapanema, high-Ti lava; Pi and Pa compositions from other regions.

Figure 10. Variation diagrams of several elements v. TiO2. All analyses plotted, irrespective of LOI content. Cat – samples from the Los Catalanes gemological district; Qua – samples from the Quaraí mining district.
Table 2. Selected chemical analyses of volcanic rocks from the Quaraí mining district and Los Catalanes gemological district

(< DL) – below detection limit; Qua – Quaraí mining district, LC – Los Catalanes gemological district; oxides in wt%, elements in ppm; (–) – not analysed.
Table 3. Classification and selected geochemical data of six lava flows from the Alegrete facies, Quaraí mining district and Los Catalanes gemological district

SiO2 and K2O shown for minimum and maximum LOI of samples from each lava. Oxides in wt%; (< DL) – below detection limit; Class. – classification.
The two most intensely mineralized lava flows in the Los Catalanes gemological district are colada Catalán and colada Cordillera, although up to four mineralized flows were indicated in other studies (e.g. Morteani et al. Reference Morteani, Kostitsyn, Preinfalk and Gilg2010). The chemical analyses of the four mineralized flows published by Morteani et al. (Reference Morteani, Kostitsyn, Preinfalk and Gilg2010) correspond to colada Catalán and colada Cordillera (inset in Fig. 9d), so fewer mineralized flows are known than previously considered.
All six studied lavas are of the low-Ti, Gramado-type (Fig. 9c, e), based on Peate, Hawkesworth & Mantovani (Reference Peate, Hawkesworth and Mantovani1992); three Paranapanema samples (Fig. 9e) from Turner et al. (Reference Turner, Peate, Hawkesworth and Mantovani1999) were actually collected from sills in a borehole in the town of Artigas. Most lavas in the Serra Geral Formation (Bellieni et al. Reference Bellieni, Comin-Chiaramonti, Ernesto, Melfi, Pacca and Piccirillo1984) are basalts and basaltic andesites (>90 vol.%) and rhyodacites; the inset of Figure 9a shows the classification of 355 volcanic rocks from the Serra Geral Formation collected by CPRM (Nakamura et al. Reference Nakamura, Wildner, Shibuya, Masuta, Murakami and Romanini2003) and analysed at ACME (Canada). In some regions, such as the Ametista do Sul region (Gilg et al. Reference Gilg, Morteani, Kostitsyn, Preinfalk, Gatter and Strieder2003; Pinto, Hartmann & Wildner, Reference Pinto, Hartmann and Wildner2010), only basalts occur.
The first lava of the Alegrete facies is a basalt; the other five lavas have the compositions of andesite and basaltic andesite (Tables 1, 2, 3). This classification is based on the SiO2 content of the rocks (Fig. 9a) that have <2 wt% LOI (loss on ignition); strong SiO2 and K2O depletion occurs in the rocks at >2% LOI so they are not used for classification. The inverse correlation of SiO2 and K2O with LOI was caused by hydrothermal alteration (smectite, zeolites), so we selected for classification the lava composition at the lowest LOI content of the several samples from the same lava. Hydrothermal alteration is highest in the mineralized rocks. For example, colada Catalán is part of the group of Gramado-type lavas with the highest SiO2 content and is an andesite, but this can only be recognized in little-altered rocks. These have SiO2 = 57.5 wt% at LOI = 0.6 wt% but hydrothermal alteration reduced the SiO2 to 50 wt% with corresponding LOI = 7.6 wt%; from SiO2 content alone, colada Catalán would be incorrectly classified as a basalt. Normalization of the major analyses to 100% free of volatiles before plotting would artificially increase the contents of the other oxides. The contents of most oxides and trace elements are little affected by alteration (Figs 9, 10). Similar geochemical mobility of SiO2 and MgO (and Rb) was observed by Wood, Gibson & Thompson (Reference Wood, Gibson and Thompson1976) in Iceland basalts.
All chemical analyses are used in variation diagrams of least mobile elements (Figs 9b, c, 10a–f), in spite of variably low to high LOI content. Some elements, such as Y and Nb, display very small deviations from a linear relationship; Rb and Sr have large deviation from linearity, whereas Th and U have small deviations. This indicates low geochemical mobility of most elements during hydrothermal alteration of the studied basalts.
Titanium and phosphorous are among the least-mobile elements. The TiO2 v. P2O5 plots (Fig. 9) discriminate the six different lavas in the region because each has a characteristic composition. The four lavas from the Los Catalanes gemological district are (in stratigraphic order) colada Mata Olho, colada Catalán, colada Cordillera and colada Muralha, and they correlate with the four lavas in the Quaraí mining district in the same stratigraphic order. As mentioned, coladas UR-13 and Coxilha were only studied to the north of the international border.
Chemical analyses of volcanic rocks from the Los Catalanes gemological district published by Turner et al. (Reference Turner, Peate, Hawkesworth and Mantovani1999) include borehole and outcrop samples. Surface samples collected in the district (U2, U3, U6, U7) are chemically similar (Fig. 9b) to colada Cordillera.
6. Scintillometric results
The scintillometric results provide a fingerprint for the stratigraphic succession of the six lavas and are a direct consequence of the variation in the chemistry of the rocks (Table 4; Figs 6, 7a, b). The siliceous coladas Catalán, Muralha and UR-13 have higher cps, K2O >1.5 wt% and U + Th >7 ppm. Chemical analyses (n = 3) of Botucatu Formation sandstones by Morteani et al. (Reference Morteani, Kostitsyn, Preinfalk and Gilg2010) yielded average 0.32 wt% K2O.
Table 4. Average scintillometric results (cps – counts per second, K + U + Th) from six lavas in boreholes and outcrops, Alegrete facies, Quaraí mining district and Los Catalanes gemological district

(–) – not measured; n – number of measurements; av – average; sd – standard deviation; cps – counts per second. Borehole cps estimated from continuous profiling.
Colada Mata Olho has 49±3.2 cps (counts per second) in outcrops, the lowest among the six lavas, and this corresponds to 51.0 wt% SiO2. Colada Catalán has 123±10.3 cps and 57.5 wt% SiO2, whereas Colada Cordillera has 62±4.7 cps and 52.5 wt% SiO2. Colada Muralha has 94±4.6 cps and 56.0 wt% SiO2 and colada UR-13 has estimated 45 cps in one borehole and 53.0 wt% SiO2, whereas colada Coxilha has 85 cps in one borehole and 54.5 wt% SiO2. Total cps values are mostly a result of K2O content of the rock (Table 3; Fig. 7b) with minor contribution from Th and U.
The chemical stratigraphy of the six lavas is supported by an equivalent scintillometric stratigraphy (Table 4). Colada Mata Olho directly overlies the eolian sandstones and has the lowest scintillometry (~49 cps) of the six lavas, only higher than the sandstones (~43 cps). Colada Catalán is a stratigraphic guide based on its high value of 123 cps, succeeded by colada Cordillera with low scintillometry (62 cps). Colada Muralha is also a stratigraphic guide, because of its high cps value near 94.
7. Discussion
The onset of volcanism is here evaluated in the Quaraí–Los Catalanes regions of Brazil and Uruguay, in a geological environment comparable to the Etendeka portion of the province (Jerram et al. Reference Jerram, Mountney, Holzforster and Stolhofen1999). This study sets forth the chemical and scintillometric stratigraphy of six lavas from the Alegrete facies (Serra Geral Formation) of southernmost Brazil and northwesten Uruguay, integrating the Quaraí mining district and Los Catalanes gemological district. The stratigraphic succession can be identified in the field and confirmed by geochemistry, because the lavas have contrasting compositions from basalt to basaltic andesite and andesite. Accordingly, while the total counts per second (eK + eU + eTh) vary within wide limits from 49 cps in basalt to 123 cps in andesite (43 cps in sandstone), individual flows have distinctive cps ranges, dependent upon their chemistry. The origin of the lavas is now evaluated in the broader scene of the Serra Geral Formation.
Diversified origins of intraplate continental basalts were operative in different provinces (e.g. Silver et al. Reference Silver, Behn, Kelley, Schmitz and Savage2006). Although an origin of the Serra Geral Formation by decompression melting of a plume head was proposed (e.g. Richards, Duncan & Courtillot, Reference Richards, Duncan and Courtillot1989; Peate et al. Reference Peate, Hawkesworth, Mantovani and Shukowski1990; White & McKenzie, Reference White and McKenzie1995; Ewart et al. Reference Ewart, Milner, Armstrong and Duncan1998), conductive heating of the subcontinental mantle is also a possible mechanism (e.g. Turner et al. Reference Turner, Peate, Hawkesworth and Mantovani1999; Coltice et al. Reference Coltice, Phillips, Bertrand, Ricard and Rey2007), because the basaltic lavas originated in the lithospheric mantle (e.g. Ernesto et al. Reference Ernesto, Marques, Piccirillo, Molina, Ussami, Comin-Chiaramonti and Bellieni2002). Overall, the low-Ti and high-Ti groups have compositional ranges equivalent to other continental flood basalt provinces, for example, Deccan, Karoo and Siberia (e.g. Wooden et al. Reference Wooden, Czamanske, Fedorenko, Arndt, Chauvel, Bouse, King, Knight and Siem1993; Kumar et al. Reference Kumar, Chavan, Sawant, Raju, Kanakdande, Patode, Deshpande, Krishnamacharyulu, Vaideswaran and Balaram2010). The Paraná volcanic province evolved along similar paths to the other provinces (e.g. Garland, Turner & Hawkesworth, Reference Garland, Turner and Hawkesworth1996) from early basalts with lithospheric chemical signatures to younger basalts with more plume-type signatures (Carlson, Reference Carlson1991). The high-Ti Pitanga-type lavas are the most closely related to a plume origin, whereas the low-Ti Gramado-type composition indicates intense interaction with the continental crust (e.g. Garland, Turner & Hawkesworth, Reference Garland, Turner and Hawkesworth1996). Turner et al. (Reference Turner, Peate, Hawkesworth and Mantovani1999) evaluated the evolution of the Gramado-type magmas in the Los Catalanes gemological district, and Hergt, Peate & Hawkesworth (Reference Hergt, Peate and Hawkesworth1991) discussed the origin of low-Ti magmas in several provinces. Fractional crystallization of olivine, clinopyroxene, plagioclase and Fe–Ti oxides was significant for the evolution of the magmas. An evolution of the low-Ti basalts in the southern Paraná volcanic province from lithospheric to asthenospheric transition was envisaged by Peate & Hawkesworth (Reference Peate and Hawkesworth1996). The six low-Ti, Gramado-type lavas in the region are closer to lithospheric mantle lavas than the high-Ti, Pitanga and Paranapanema lavas higher up in the stratigraphy (Figs 9f, 11) (e.g. Peate et al. Reference Peate, Hawkesworth, Mantovani, Rogers and Turner1999).
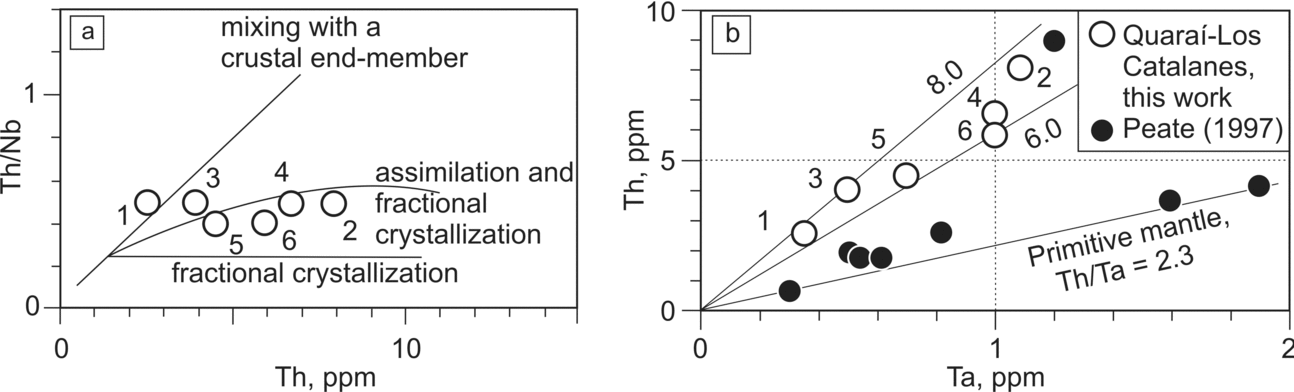
Figure 11. (a) Th/Nb v. Th diagram displaying the compositions of the six studied lava flows from Quaraí–Los Catalanes; (b) Th v. Ta diagram, displaying the composition of the six lava flows from this study and the compositions of the chemical magma types of the Paraná magmatic province as defined by Peate, Hawkesworth & Mantovani (Reference Peate, Hawkesworth and Mantovani1992). Colada numbers in both diagrams are: 1 – Mata Olho; 2 – Catalán; 3 – Cordillera; 4 – Muralha; 5 – UR-13; 6 – Coxilha.
This is in agreement with the interpretation of Turner et al. (Reference Turner, Peate, Hawkesworth and Mantovani1999) for the lavas of the Los Catalanes gemological district. Although high-Ti and low-Ti lavas occur intercalated in some sections of the Paraná volcanic province (e.g. T. C. Vargas, unpub. Doctoral thesis, Univ. Federal Fluminense, Rio de Janeiro, 2006), the incompatible-element depletion of the Alegrete facies lavas suggests that less-depleted mantle was tapped for the effusion of younger lavas higher in the sequence. The process of magma generation in the mantle was varied along the Paraná volcanic province, because some low-Ti lavas occur among the dominant high-Ti lavas of the northern portion and some high-Ti lavas occur among the low-Ti lavas of the southern portion of the province (Marques et al. Reference Marques, Ernesto, Piccirillo, Bellieni, Figueiredo and Min2005).
High-Ti, Paranapanema-type lavas commonly overlie the low-Ti Gramado-type lavas in the volcanic province. The Paranapanema sills present in the borehole in the town of Artigas were interpreted as lava flows by Turner et al. (Reference Turner, Peate, Hawkesworth and Mantovani1999); these sills may have generated lavas higher up in the section and are now eroded so that only low-Ti lavas are present in the Alegrete facies. A possible evolution of the lavas in the studied region (Fig. 11a) is the mixing of a mantle-generated magma with a crustal end-member until the Th/Nb ratio of the magma reached 0.5; then fractional crystallization produced variation in magma compositions, which were tapped in sequentially different positions from the magma chamber. High-Ti magmas tend to have Th/Ta ratios (Fig. 11b) closer to primitive mantle than the low-Ti magmas of this investigation. This broad genetic interpretation of the Serra Geral Formation leads to a better understanding of the low-Ti, Gramado-type, lavas in the Quaraí–Los Catalanes region.
The most distinctive flow in the field both in Uruguay and Brazil is colada Catalán; it extends for 200 km along a N–S line between Itaqui (boreholes), Quaraí (surface exposures) and the southern limit of the Los Catalanes gemological district. The E–W distance covered by this aa lava is 100 km, the largest andesite flow identified in the Paraná volcanic province. This distance is superseded by transatlantic correlation of silicic flows in the Paraná–Etendeka province which indicates runout distances exceeding 500 km (Milner et al. Reference Milner, Duncan, Whittingham and Ewart1995).
Equivalent lava stratigraphy is seen in the Los Catalanes gemological district and Quaraí mining districts. The first four flows correlate between Los Catalanes and Quaraí, although the lavas present above these four lavas in Quaraí were not studied in Los Catalanes. This is an important geological parameter in the search for major amethyst geode deposits in the Quaraí mining district, because the mineralized coladas Catalán and Cordillera in Uruguay have physical continuity into Brazil where they have similar characteristics and possibly similar world-class geode deposits. For instance, Garimpo do Vivi is in colada Catalán and at the end of 2009 started the production of amethyest geodes.
Establishment of a regional lava stratigraphy not only unifies our thinking about the genesis of giant geode deposits in both districts, but will also improve exploration in both districts and hopefully, help extend them to the large area covered by the identified flows.
8. Conclusions
The characterization and correlation of tholeiitic lavas of the Cretaceous Alegrete facies (Serra Geral Formation) from both the Quaraí mining district (Brazil) and Los Catalanes gemological district (Uruguay) lead to significant conclusions.
(1) World-class amethyst and agate geode deposits are expected to be present in the Quaraí mining district, because lava stratigraphy is the same as the Los Catalanes gemological district.
(2) Six lavas are characterized in the region, based on field relations, rock geochemistry and scintillometry; chemical variation of the volcanic rocks is 51.0–57.5 wt% SiO2, so rock types range from basalt (colada Mata Olho) to andesite (colada Catalán) and basaltic andesite (coladas Cordillera, Muralha, UR-13 and Coxilha).
(3) Coladas Catalán and Cordillera have world-class amethyst geode deposits in Los Catalanes; Garimpo do Vivi in the Quaraí mining district is in colada Catalán.
(4) Colada Catalán, and locally colada Muralha, has the structure of aa lava and flowed over 200 × 100 km, the most extensive lava identified in the southern Paraná volcanic province.
(5) Total gamma-ray spectrometry in outcrops and boreholes yields a consistent stratigraphy in the Quaraí mining district.
(6) The lavas may have formed by melting of the lithospheric mantle, followed by intrusion into the lower crust and extensive crustal assimilation; geochemical evidence is strong for significant crystal fractionation before individual flows were released from the magma chamber.
Acknowledgements
The Geological Survey of Brazil (CPRM) did the drilling for underground water and the scintillometric profiling of boreholes. CPRM also undertook extensive field work in the area over two decades. Nakamura et al. (Reference Nakamura, Wildner, Shibuya, Masuta, Murakami and Romanini2003) provided many unpublished chemical analyses of Serra Geral Formation lavas, in joint research with CPRM. Financial support for field work, scintillometric survey and chemical analyses was given by ‘Conselho Nacional do Desenvolvimento Científico e Tecnológico do Brasil (CNPq)’ through grants to LAH. Antônio Flávio Uberti Costa is acknowledged for support and expertise in geophysics. Javier Techera (DINAMIGE) was helpful with field geology in the Los Catalanes gemological district. Paul E. Potter is thanked for manuscript revision.