1. Introduction
Most of Earth’s largest earthquakes occur in subduction zones that constitute the most active plate boundaries and are usually densely populated. Thrust or reverse earthquakes take place more frequently than other types of tectonic events at the convergent plate boundaries. Earthquakes of this type are responsible for most of the recent loss of life and property through natural disasters in such regions, and therefore have attracted considerable attention since the 2004 Sumatra–Andaman Mw 9.0 earthquake (e.g. Ammon et al. Reference Ammon, Ji, Thio, Robinson, Ni, Hjorleifsdottir, Kanamori, Lay, Das, Helmberger, Ichinose, Polet and Wald2005; Titov et al. Reference Titov, Rabinovich, Mofjeld, Thomson and Gonzalez2005; Vigny et al. Reference Vigny, Simons, Abu, Bamphenyu, Satirapod, Choosakul, Subarya, Socquet, Omar, Abidin and Ambrosius2005; Subarya et al. Reference Subarya, Chlieh, Prawirodirdjo, Avouac, Bock, Sieh, Meltzner, Natawidjaja and McCaffrey2006; Banerjee et al. Reference Banerjee, Pollitz, Nagarajan and Bürgmann2007). Similar to thrust earthquakes, strike-slip events also pose a seismic and tsunami threat to subduction zone areas, but little is known about the rupture structures associated with them. The strike-slip faulting along a plate boundary structure during the Palu Mw 7.5 earthquake on 28 September 2018 provides an unusual opportunity to investigate the characteristics of these events.
The focal mechanism solutions and observations from geodetic, seismic and geological data (Bao et al. Reference Bao, Ampuero, Meng, Fielding, Liang, Milliner, Feng and Huang2019; Fang et al. Reference Fang, Xu, Wen, Wang, Xu, Zhao and Yi2019; Socquet et al. Reference Socquet, Hollingsworth, Pathier and Bouchon2019; Zhang et al. Reference Zhang, Chen and Feng2019; Wu et al. Reference Wu, Ren, Liu, Chen, Guo, Yin, Ran, Li and Yang2020) indicate that the earthquake occurred on the left-lateral, north–south-striking Palu–Koro fault, a main plate boundary structure that connects to the Minahassa subduction zone to the north, and accommodates the relative motion between the Makassar block and the North Sula block (Fig. 1a). One remarkable feature of the 2018 event is that this strike-slip earthquake caused an unexpected destructive tsunami (Heidarzadeh et al. Reference Heidarzadeh, Muhari and Wijanarto2019; Omira et al. Reference Omira, Dogan, Hidayat, Husrin, Prasetya, Annunziato, Proietti, Probst, Paparo, Wronna, Zaytsev, Pronin, Giniyatullin, Putra, Hartanto, Ginanjar, Kongko, Pelinovsky and Yalciner2019; Ulrich et al. Reference Ulrich, Vater, Madden, Behrens, Van Dinther, Van Zelst, Fielding, Liang and Gabriel2019). Tsunamis usually accompany earthquakes that occur on thrust or reverse faults in subduction zones, but not for such an earthquake type (Prasetya et al. Reference Prasetya, De Lange and Healy2001; Carvajal et al. Reference Carvajal, Araya-Cornejo, Sepúlveda, Melnick and Haase2018; Mikami et al. Reference Mikami, Shibayama, Esteban, Takabatake, Nakamura, Nishida, Achiari, Rusli Marzuki, Marzuki, Stolle, Krautwald, Robertson, Aránguiz and Ohira2019). Another striking feature of the 2018 event, suggested by seismic and space geodesy observations, is that the rupture propagated at supershear speeds on most of the rupture segments (Bao et al. Reference Bao, Ampuero, Meng, Fielding, Liang, Milliner, Feng and Huang2019; Socquet et al. Reference Socquet, Hollingsworth, Pathier and Bouchon2019), something which commonly occurs on faults with simple geometry (Bouchon & Vallée Reference Bouchon and Vallée2003; Vallée et al. Reference Vallée, Landès, Shapiro and Klinger2008; Bouchon et al. Reference Bouchon, Karabulut, Bouin, Schmittbuhl, Vallée, Archuleta, Das, Renard and Marsan2010; Konca et al. Reference Konca, Leprince, Avouac and Helmberger2010). Our previous study reported the primary features of the coseismic surface ruptures associated with the earthquake (e.g. coseimic slip sense, the coseismic displacement distribution, the seismogenic fault), based on a limited number of field investigations carried out immediately after the earthquake (Wu et al. Reference Wu, Ren, Liu, Chen, Guo, Yin, Ran, Li and Yang2020). However, that previous study is preliminary, and the assessment of the fault geometry, the identification of rupture segments, and details of the fault’s seismotectonic behaviour remain unclear because of a lack of detailed field investigations, which are important for understanding rupture propagation and termination and assessing the kinematics, rupturing process and the seismic hazard of an interplate strike-slip fault. In this study, a thorough investigation and detailed mapping of the coseismic surface rupture structures in the field allowed us to understand what may have caused the puzzling tsunami and further discuss the role of rupture geometry in a supershear event.
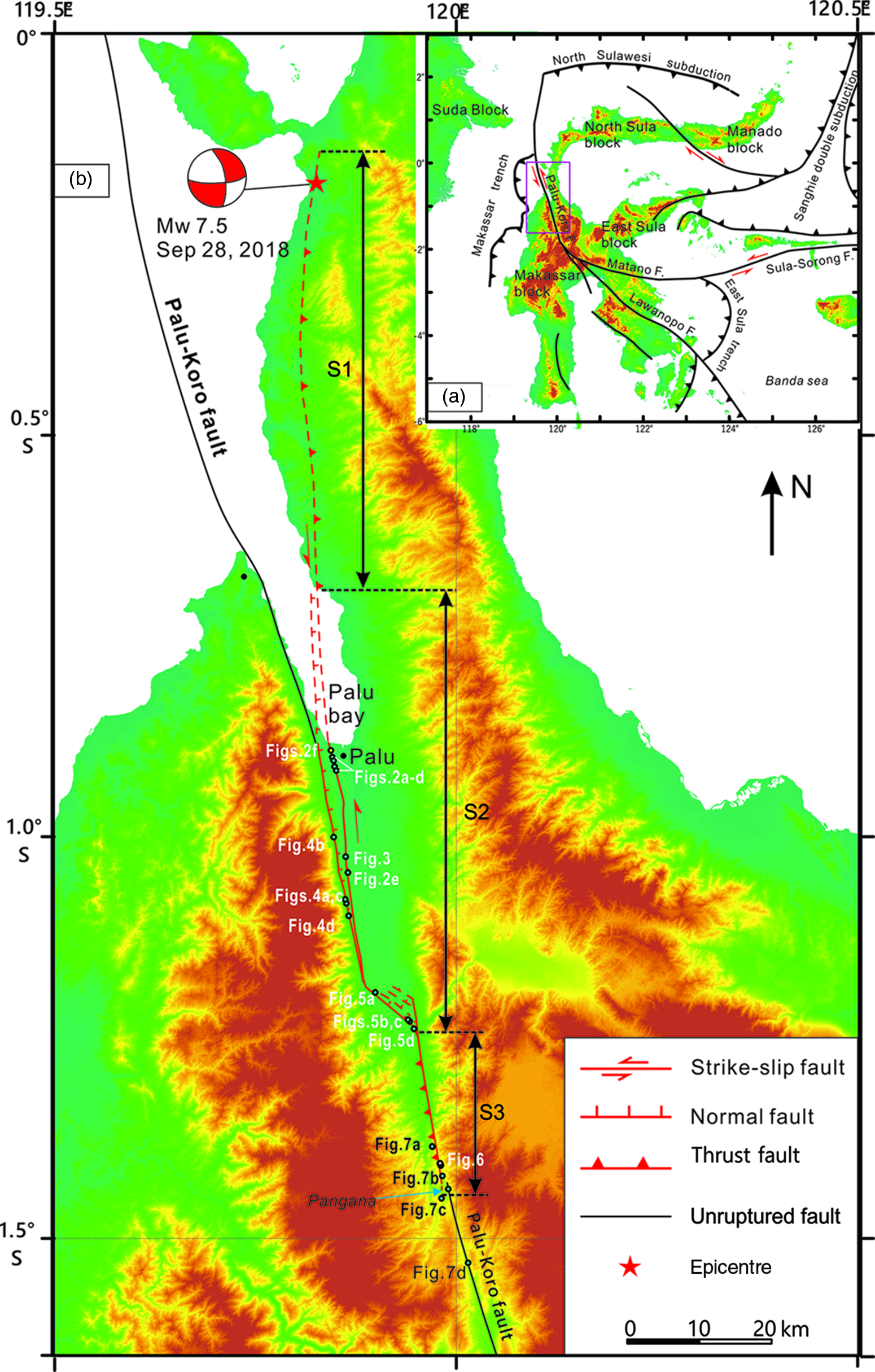
Fig. 1. Tectonic setting and surface ruptures associated with the Mw 7.5 Palu earthquake. (a) The island of Sulawesi, where the 28 September 2018 Mw 7.5 earthquake occurred, is located at the junction of several blocks. The Palu–Koro fault, which produced the 2018 earthquake, is the main plate boundary structure in the Sulawesi area. (b) The extent of the surface ruptures of the 2018 Palu earthquake. Solid red lines: rupture sections mapped in the field; dashed red lines: rupture sections suggested by interferometric synthetic aperture radar (InSAR) and seismic studies (e.g. Bao et al. Reference Bao, Ampuero, Meng, Fielding, Liang, Milliner, Feng and Huang2019; Fang et al. Reference Fang, Xu, Wen, Wang, Xu, Zhao and Yi2019; Socquet et al. Reference Socquet, Hollingsworth, Pathier and Bouchon2019). S1–S3: northern, middle and southern segments, respectively. Note that the middle segment of the rupture zone consists of two strands. The geological traces of the unruptured faults are shown in black. The epicentre is indicated by a red star. The epicentre and the focal mechanism are from US Geological Survey (2018).
To better understand the characteristics of the surface ruptures and related faulting behaviour of such a strike-slip earthquake at convergent plate boundaries, we carried out additional detailed field investigations along the surface ruptures of the 2018 Palu earthquake three and a half months after the earthquake. Based on the previous fieldwork, we investigated geometric and geomorphic features of the surface rupture in greater detail, and discuss the rupture mechanism of a supershear interplate earthquake.
2. Tectonic setting
The Palu–Koro fault and its southeast continuation (the Matano fault) are the main active structures in Sulawesi, eastern Indonesia, a triple junction where the Australian, Philippine Sea, Pacific and Sunda plates (eastern part of Eurasia) meet (Walpersdorf et al. Reference Walpersdorf, Rangin and Vigny1998 a; Socquet et al. Reference Socquet, Simons, Vigny, McCaffrey, Subarya, Sarsito, Ambrosius and Spakman2006). The Palu–Koro fault obliquely crosses the Sulawesi island and divides it into two parts: the Sula block to the northeast and the Makassar block to the southwest (Fig. 1a). The crustal blocks’ relative motion accommodates the convergence between the plates in the junction area (Fitch Reference Fitch1972; Silver et al. Reference Silver, McCaffrey and Smith1983; Walpersdorf et al. Reference Walpersdorf, Rangin and Vigny1998a; Stevens et al. Reference Stevens, McCaffrey, Bock, Genrich, Endang, Subarya, Puntodewo, Fauzi and Vigny1999; Kreemer et al. Reference Kreemer, Holt, Goes and Govers2000; Vigny et al. Reference Vigny, Perfettini, Walpersdorf, Lemoine, Simons, van Loon, Ambrosius, Stevens, Mc Caffrey, Morgan, Bock, Subarya, Manurung, Kahar, Abidin and Abu2002; Socquet et al. Reference Socquet, Simons, Vigny, McCaffrey, Subarya, Sarsito, Ambrosius and Spakman2006). Sinistral transpression through the Sula–Sorong fault in the east is transformed by left-lateral strike-slip along the Matano fault and the Palu–Koro fault to the west and the north, then absorbed at the Minahassa Trench, where the Sunda plate subducts southward beneath the Sula block (e.g. Hamilton Reference Hamilton1979; Silver et al. Reference Silver, McCaffrey and Smith1983; Rangin et al. Reference Rangin, Le Pichon, Mazzotti, Pubellier, Chamot-Rooke, Aurelio, Walpersdorf and Quebral1999) (Fig. 1a).
The ∼300 km long, left-lateral Palu–Koro fault connects the north Sulawesi subduction zone in the north and shows a transforming behaviour. Much of its trace north of the Palu gulf is under water, and runs through the 2018 epicentral area. To the south, the fault trace is well expressed in the Palu valley, and becomes more linear southward. In addition to evidence for late Quaternary geomorphic features associated with strike-slip faulting, the major fault bounding the Palu valley is marked by faceted escarpments including a several metres high scarp, which suggests that the recent motion of the fault contains a substantial normal component (e.g. Bellier et al. Reference Bellier, Sébrier, Seward, Beaudouin, Villeneuve and Putranto2006). Slip on the Palu–Koro fault accommodates rotation of the Sula block at a rate of between ∼35 mm a−1 determined by geological studies (Bellier et al. Reference Bellier, Sebrier, Beaudouin, Villeneuve, Braucher, Bourlès, Siame, Putranto and Pratomo2001) and ∼42 mm a−1 inferred from GPS (the global positioning system) observation (Walpersdorf et al. Reference Walpersdorf, Vigny, Subarya and Manurung1998 b; Stevens et al. Reference Stevens, McCaffrey, Bock, Genrich, Endang, Subarya, Puntodewo, Fauzi and Vigny1999; Socquet et al. Reference Socquet, Simons, Vigny, McCaffrey, Subarya, Sarsito, Ambrosius and Spakman2006). Estimates from plate kinematics and palaeomagnetic studies suggest a similarly high slip rate of ∼30–50 mm a−1 across the fault (Silver et al. Reference Silver, McCaffrey and Smith1983; Surmont et al. Reference Surmont, Laj, Kisse, Rangin, Bellon and Priadi1994). According to the empirical relations between displacement and magnitude for strike-slip earthquakes, the recurrence interval for magnitude M>7 earthquakes on the Palu–Koro fault is ∼100 years if it slips at a rate of 40 mm a−1 (Socquet et al. Reference Socquet, Simons, Vigny, McCaffrey, Subarya, Sarsito, Ambrosius and Spakman2006). The characteristic earthquake model and such a recurrence interval can account for the low seismicity in the past 100 years.
3. Observations
Both seismic inversion results (Bao et al. Reference Bao, Ampuero, Meng, Fielding, Liang, Milliner, Feng and Huang2019; Zhang et al. Reference Zhang, Chen and Feng2019; Li et al. Reference Li, Zhao, Tan and Xu2020) and geodetic observations (Fang et al. Reference Fang, Xu, Wen, Wang, Xu, Zhao and Yi2019; Socquet et al. Reference Socquet, Hollingsworth, Pathier and Bouchon2019) suggest the rupture of the 2018 Palu Mw 7.5 earthquake nucleated near the epicentre located ∼80 km north of the city of Palu, and propagated mainly unilaterally southward along the Palu–Koro fault for ∼150 km. The coseismic slip was mainly left-lateral strike-slip with some vertical component. Based on our field investigation, combined with knowledge of previous studies from seismic and geodetic observations, the 2018 earthquake rupture zone can be divided into three segments: the northern, the middle and the southern (Fig. 1b), separated by bends or steps identified in our work and previous studies (Fang et al. Reference Fang, Xu, Wen, Wang, Xu, Zhao and Yi2019; Socquet et al. Reference Socquet, Hollingsworth, Pathier and Bouchon2019).
3.a. The northern segment
The northern segment of the 2018 rupture did not follow the previously mapped trace offshore, but broke on land within the Sulawesi neck (Fig. 1b). This segment is constrained based mainly on seismic and geodetic studies (Fang et al. Reference Fang, Xu, Wen, Wang, Xu, Zhao and Yi2019; Socquet et al. Reference Socquet, Hollingsworth, Pathier and Bouchon2019), due to the extraordinary difficulty of carrying out field investigation in such a remote, mountainous area and the fact that there is almost no way to gain access to the fault. The rupture exhibits a much-smoothed displacement gradient, which indicates that along this segment the fault probably did not break the surface. A coseismic lateral slip of up to ∼2 m was measured by geodetic studies (Fang et al. Reference Fang, Xu, Wen, Wang, Xu, Zhao and Yi2019). Uplift occurred to the east of the fault (Socquet et al. Reference Socquet, Hollingsworth, Pathier and Bouchon2019), which suggests a thrust slip component on this segment, especially in the area of the northern portion close to the epicentre. The maximum thrust slip observed around 0.35° S on this segment is also ∼2 m (Fang et al. Reference Fang, Xu, Wen, Wang, Xu, Zhao and Yi2019). The rupture trace along this segment is less straight than it appears along the southern segments and propagated southwards for ∼60 km before reaching Palu bay.
At ∼0.7° S, the rupture entered offshore and propagated southward for ∼21 km within Palu bay. Around the part between 0.7° S and 0.8° S, the fault behaves transpressionally, displaying comparable strike-slip with dip-slip components (Fang et al. Reference Fang, Xu, Wen, Wang, Xu, Zhao and Yi2019). Therefore, this point (∼0.7° S) acts as a boundary between the northern and middle segments.
3.b. The middle segment
Significant normal-slip components are observed in the offshore part to the south. Along this portion within Palu bay, left-lateral strike-slip of up to 6 m with normal slip of up to 2 m was observed geodetically (Ulrich et al. Reference Ulrich, Vater, Madden, Behrens, Van Dinther, Van Zelst, Fielding, Liang and Gabriel2019). The coseismic rupture section south of Palu bay is the main surface rupture of the 2018 event and shows a very sharp trace both in field and in satellite images. By combining field studies with analysis of satellite images acquired after the earthquake, we mapped the surface rupture in detail. In the middle segment, the 2018 earthquake broke two distinct subparallel strands, 1–1.3 km apart: a strike-slip strand in the east and a normal strand in the west (Fig. 1b). A similar phenomenon was also identified geodetically (Socquet et al. Reference Socquet, Hollingsworth, Pathier and Bouchon2019). The ruptures propagated from the bay, then extended southward on land, which suggests that similar fault geometry possibly occurred offshore in the bay and the on-land segment is a continuation of the offshore extent. This point is also supported by the similar motion and slip magnitude on both sections. From a geomorphic perspective, Palu bay and the Palu valley seem to belong to a NWN-striking rift caused by the pull-apart structure in the Palu area. The eastern strand traverses a flat, alluvial floodplain on which Palu city is situated, and exhibits almost pure strike-slip motion along the main stretch of this rupture strand. This strand is located up to 1.5 km east of the mapped fault trace and does not appear to lie on pre-existing scarps along a large portion of the fault. From the southern shoreline of Palu bay to the southern end of this segment, the strike-slip strand is mostly simple, narrow and continuous (Fig. 2; Figs S2 and S3 in the Supplementary Material available online at https://doi.org/10.1017/S0016756822000012). The rupture generally displays as a zone consisting of multiple subparallel fault strands with a width varying from several metres to tens of metres (Fig. 2c and d). It is also displayed as a ∼1 m wide opening with left-lateral slip (Fig. 3a; Fig. S3f in the Supplementary Material available online at https://doi.org/10.1017/S0016756822000012) or defined as a series of NWN-striking, right-stepping en echelon tension fissures in some places. Coseismic offsets were measured across linear surface landmark features that cross the fault, such as roads, fences, walls, canals, crop rows, ditches, treelines and field boundaries (Fig. 2). Most of the offset features we selected to measure are nearly perpendicular to the fault strike; azimuthal corrections can be neglected. To the features not strictly perpendicular to the fault, the pierce points of the offset markers have been projected on a vertical plane striking perpendicularly to the fault trace; such azimuthal corrections can ensure a reliable value of the offset amounts. The left-lateral slips are commonly 3–5 m, with a maximum value of 5.5 ± 0.1 m measured at the site (1.025° S) near Mesjid Alkhairaat, 15 km south of Palu bay, based on offset of field boundaries (Fig. 3). The maximum coseismic horizontal displacement is obtained from measurements both in the field and on the unmanned aerial vehicle (UAV) images. The maximum coseismic offset was previously reported to be ∼6.2 m by our group, based on a preliminary result during the first field investigation, a value obtained only from the UAV image of an offset vehicle track (Fig. 2f). We re-examined that rupture section and found that surface ruptures at that site have a complicated pattern, which makes the accurate matching of offset piercing lines difficult. Measurements of several offset linear geomorphic markers across this fault section yield the coseismic horizontal displacements from 2 to 4 m, which suggest the coseismic lateral slip should be in the range 2–4 m along this section. Thus, we infer that the maximum offset of 6.2 m was somewhat misinterpreted.

Fig. 2. Coseismic left-lateral slip along the middle segment of the 2018 surface rupture zone. (a) Offsets of a street and a small canal near JI Asam II in Palu city, with sinistral displacement of c. 3.0 ± 0.2 m, view to the east; (b), another site in Palu city showing typical strike-slip offsets of the 2018 surface rupture zone (west view), sinistral displacement of ∼4.2 ± 0.2 m; (c, d) surface breaks exhibiting as a ∼5–10 m wide zone constituted by several parallel strands (indicated by light purple arrows and dark purple arrows), views to the west and east, respectively; (e) coseismic left lateral offsets of the field edges south of Palu city, view to the south. (f) An oblique UAV image showing the coseismic surface rupture at the site where the maximum coseismic left-lateral offset was measured in the previous study (Wu et al. Reference Wu, Ren, Liu, Chen, Guo, Yin, Ran, Li and Yang2020). The red arrows show the locations and strike of the fault trace, the yellow arrows indicate the offset features. See Figure 1 for locations.
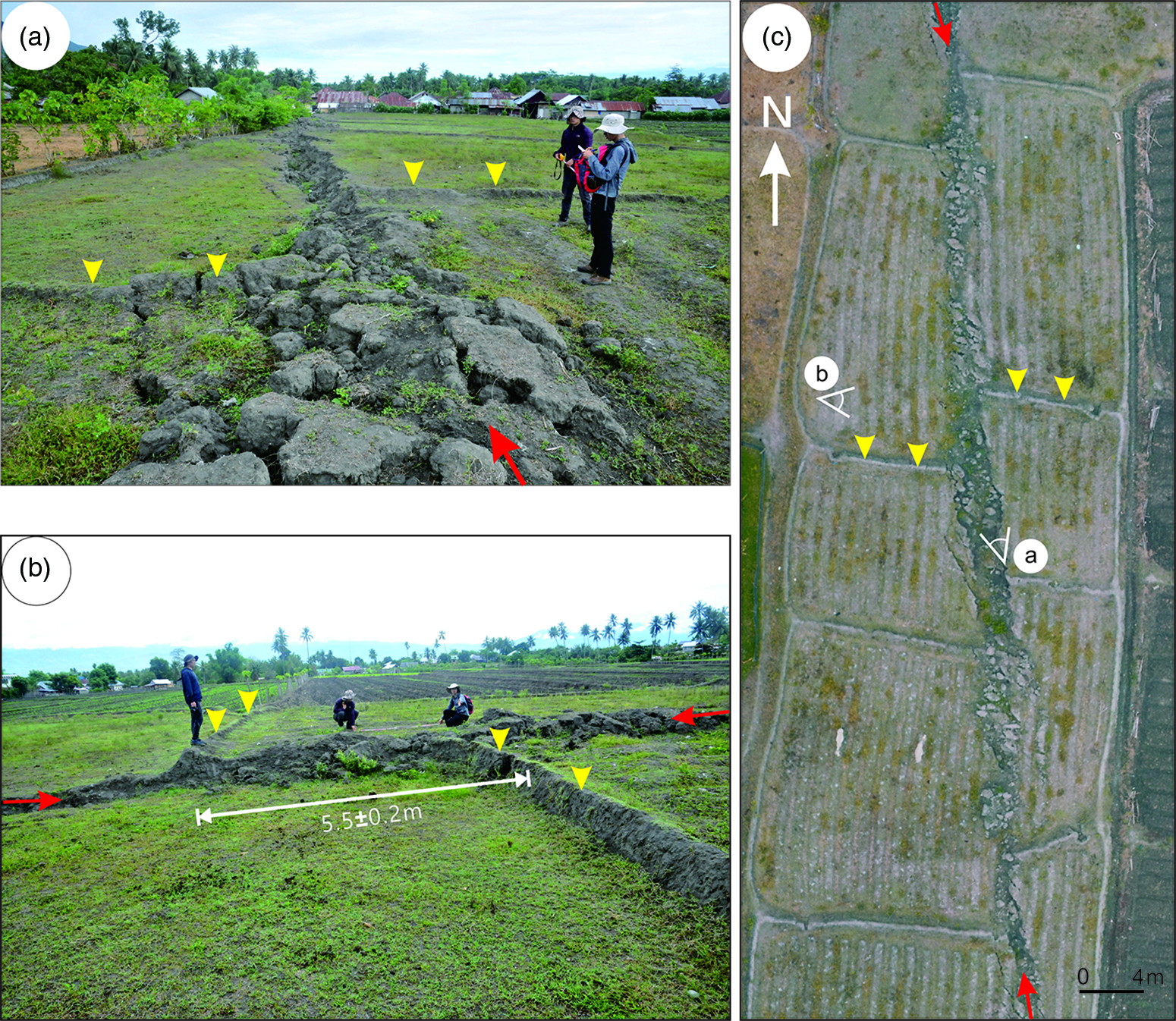
Fig. 3. Photos and UAV image showing maximum coseismic left-lateral slip along the middle segment of the 2018 surface rupture zone. (a) Surface break exhibiting as a 1–2 m wide crack with ∼5 m sinistral displacement (north view, see the location in (c)); (b) the maximum strike-slip displacement observed in the field (east view, see the location in (c)); (c) an oblique UAV image of a surface rupture section. The red arrows show the locations and strike of the fault trace; the field edges are indicated by the yellow arrows. See Figure 1 for locations.
The western strand runs at the base of the sharp range front west of the Palu valley, with prominent triangular facets several hundred metres high (Fig. 4a; see Supplementary Material for more figures). The surface break is discontinuous, and consists of open cracks and small scarps showing mainly normal faulting with a vertical, down-to-the-east offset of generally 30–150 cm (Fig. 4 b–d). Along this normal strand, the surface rupture closely follows the previously mapped fault trace. Fresh opening is usually observed at the base of a pre-existing escarpment (Fig. 4c–d; Fig. S4b–d in the Supplementary Material available online at https://doi.org/10.1017/S0016756822000012). Continuous ground cracking ∼5 km long has been mapped along the mountain front in the central part of this rupture strand, following a pre-existing fault trace defined by scarps and lineaments. In other areas, surface ruptures are generally discontinuous and short, especially approaching the southern end. The two rupture strands run alongside each other for c. 35 km on land before they merge into a single rupture zone at the southern limit of the Palu valley. The junction is characterized by a bend where the azimuth of the strike rotates by ∼20° counterclockwise, which could be considered as a segment boundary (Fig. 1b). Within this ∼8 km long extensional bend, faulting is distributed throughout a 1 km wide zone of surface cracking, each crack showing several to tens of centimetres of vertical displacement (Fig. 5; Fig. S5 in the Supplementary Material available online at https://doi.org/10.1017/S0016756822000012).

Fig. 4. Geomorphic expression and typical surface breaks of the 2018 earthquake along the western strand bounding the western edge of the Palu valley. (a) An oblique UAV image showing morphology by the active fault in front of the triangular facets on the Palu–Koro fault north of Buper Vatujulai; (b) west view of 2018 break with a down-to-the-east throw of 50 cm, following a pre-existing fault scarp (note that the ‘new track’ is a track created after the earthquake because the old one did not work due to the wide crack); (c) a canal was vertically offset ∼150 cm during the earthquake and formed a small waterfall (view is to the west); (d) west view of a 120 cm high scarp formed during the 2018 earthquake. Red arrows show the locations of the fault trace and the fault scarps; the scarp heights are also shown. Locations are shown in Figure 1.

Fig. 5. Coseismic deformation and related tectonic geomorphology along the bend between the middle and southern segments. (a) North view of the new vertical offset superimposed on a pre-existing fault scarp around Masjid Nurul Hidayah; (b) a fence was vertically offset ∼50 cm during the earthquake and formed a small scarp northeast of Mushollah (view is to the south); (c) the road was also vertically offset the same amount during the earthquake (view is to the southwest); (d) a more than 200 m long rupture with a ∼20 cm high scarp observed across the farmland (view is to the east). Red arrows show the locations of the fault trace and the fault scarps. Locations are shown in Figure 1.
3.c. The southern segment
South of the bend, the rupture bends back to a strike similar to the overall orientation of the Palu–Koro fault (Fig. 1), and coseismic slip decreases rapidly, as indicated by discontinuous, small scarps along the fault. Then the rupture zone enters a narrow valley between high mountains, where the surface ruptures are obscured by active drainage, vegetation, landslides and steep topography. Although observations along much of the stretch of this segment are limited because of the mountainous environment, the characteristics of the rupture are still clearly exhibited. The 2018 rupture zone along the southern segment is mostly single-stranded, and displays a left-lateral slip with a minor vertical motion (Figs 1b and 6). In contrast to the middle segment, a clear reverse component has been identified along this segment, with a vertical displacement as large as 0.4 m (Fig. 6b and c; Fig. S6a–c in the Supplementary Material available online at https://doi.org/10.1017/S0016756822000012). The ratio between average horizontal displacement and reverse slip is about 1:1 along the southern segment (Fig. 7b; Fig. S6a in the Supplementary Material available online at https://doi.org/10.1017/S0016756822000012). The surface rupture accompanying the 2018 earthquake follows pre-existing 1–2 m high scarps along the southernmost section (Fig. 6b). Slip along this segment is typically low, with a maximum observed left-lateral and vertical displacement of c. 50 cm and 40 cm, respectively. The rupture on the southern segment was arrested by the ∼1.2-km wide Pangana basin, which acted as a step-over (Fig. 1b). Around the southern end, the rupture is characterized by a series of 10–30 cm wide extensional fissures (Fig. 7c). Based on the absence of surface breaks south of the step-over (Fig. 7d; Fig. S6d–f in the Supplementary Material available online at https://doi.org/10.1017/S0016756822000012), we conclude that the coseismic surface rupture of the 2018 event did not extend onto the fault segment in the south.

Fig. 6. Coseismic deformation along the southern segment of the surface rupture zone. (a) An oblique UAV image of a rupture section along the southernmost part of the surface ruptures in Pangana basin; (b) coseismic fault scarps (indicated by red arrows) and pre-existing fault scarps (indicated by white arrows). North view, see the location in (a); (c) the fault plane (indicated by the two red arrows) disclosed on the southern wall of the trench excavated across the surface rupture (see the location in (a)); U1–U3 are young sediment units. Red arrows show the locations of the fault trace. See Figure 1 for locations.

Fig. 7. Coseismic deformation along the southern segment of the surface rupture zone and tectonic geomorphology of the Palu–Koro fault south of the 2018 rupture. (a) A ∼60 cm high fault scarp formed during the earthquake at the northern part of Pangana basin, view to the northeast. (b) The coseismic left-lateral and vertical displacements of the field edges close to the southern end of the rupture zone were ∼50 cm and 40 cm, respectively (view is to the east). (c) Open cracks along the fault south of the Pangana basin, where the rupture terminated (south view); (d) the geomorphic expression of the fault that was not ruptured during the 2018 event (south view). Red arrows show the locations of the fault trace. See Figure 1 for locations.
4. Discussion
Our field investigation, combined with previous studies on the surface ruptures of the 2018 Mw 7.5 earthquake, reveals that the earthquake ruptured a ∼150 km long part of the Palu–Koro fault, and displayed a predominantly left-lateral strike-slip motion with some dip-slip component on the surface. The maximum strike-, normal and thrust slips are ∼5.5 m, ∼2 m and ∼2 m, respectively.
4.a. The surface rupture zone of the 2018 event shows two unique features regarding a plate-boundary strike-slip fault
According to the field observations, two remarkable features characterize the 2018 surface ruptures. One is that the rupture was dominated by sinistral motions with a normal component in the middle segment, in contrast to thrust components in the northern and southern segments. This feature illustrates that the Palu–Koro fault acts as a transform fault connecting the two subduction zones in the north and south (Fig. 8a). The thrust components in the northern and southern parts are consistent with the shortening across them, which reflects a transpressional stress regime (Fig. 8a). Thrust slip on the northern part of the Palu–Koro fault has been documented by GPS results, which indicate an 8 mm a−1 shortening across the fault (Stevens et al. Reference Stevens, McCaffrey, Bock, Genrich, Endang, Subarya, Puntodewo, Fauzi and Vigny1999). This thrust slip has also been observed in geological investigation, and can be considered as corresponding to an early (post-Oligocene stress) regime (Bellier et al. Reference Bellier, Sébrier, Seward, Beaudouin, Villeneuve and Putranto2006). Reverse faulting in the southern part of the Palu valley has been observed for the first time in our fieldwork. Evidence from geomorphic expression and trench result is reasonable. These two segments act as transpressional structures that accommodate the present-day convergence of the Sula block with the Sunda plate in the north and with the Australian plate in the south (Fig. 8a). The normal faulting in the middle segment is related to an east-trending extension, suggesting that the transtensional regime corresponds to the fault activity in this area. It accommodates the relative motion between the Makassar block, which rotates anticlockwise, and the Sula block, which rotates clockwise (Socquet et al. Reference Socquet, Simons, Vigny, McCaffrey, Subarya, Sarsito, Ambrosius and Spakman2006).

Fig. 8. (a) The mode of the Palu–Koro fault and the main faults surrounding the island of Sulawesi. The Palu–Koro fault behaves as a transform fault accommodating the collisions and rotation between different blocks. (b) A three-dimensional model of the Palu–Koro fault in the Palu valley. The two rupture strands are linked at depth. Cross-sections are constructed based on geological reports from Hamilton (Reference Hamilton1979) and Watkinson & Hall (Reference Watkinson and Hall2017). U1: Quaternary layers; U2: Cenozoic volcanics and rocks; U3: schist.
The second notable feature is that the middle segment (the Palu segment) exhibits an unusual geometry that consists of two distinct, roughly parallel strands showing almost pure strike-slip and normal faulting (Fig. 8b). Such an extraordinary structure can be explained by slip partitioning between coseismic strike-slip and normal faulting along an oblique slip fault zone. Coseismic oblique slip along a fault zone partitioned on two or more faults with different motions in a single event is rarely observed (King et al. Reference King, Klinger, Bowman and Tapponnier2005; Klinger et al. Reference Klinger, Xu, Tapponnier, Van der Woerd, Lasserre and King2005). The same partitioning occurred during the 2001 Kunlun earthquake between coseismic strike-slip and normal faulting on two parallel fault strands along a 70 km long rupture segment (King et al. Reference King, Klinger, Bowman and Tapponnier2005). Previous studies have pursued a mechanism for slip partitioning (Bowman et al. Reference Bowman, King and Tapponnier2003; King et al. Reference King, Klinger, Bowman and Tapponnier2005). Bowman et al. (Reference Bowman, King and Tapponnier2003) proposed that slip partitioning can be explained as a result of upward propagation of oblique shear at depth. King et al. (Reference King, Klinger, Bowman and Tapponnier2005) suggested that the likely mechanical cause of such partitioning is the rupture travelling faster at depth than near the surface, leaving the surface deformation to catch up. The slip partition on the 2018 earthquake rupture corresponds to the faulting characteristics of the segment of the Palu–Koro fault as a transtensional structure.
4.b. Coseismic slip partitioning contributed much to the supershear speed
Consequently, the above-stated two focal issues of the 2018 earthquake rupture could possibly be resolved now by understanding the geometry and faulting characteristics of the rupture. One is that the Mw 7.5 earthquake may have ruptured at a supershear speed, according to the results from seismic inversion, geodetic observations and joint analysis (Bao et al. Reference Bao, Ampuero, Meng, Fielding, Liang, Milliner, Feng and Huang2019; Socquet et al. Reference Socquet, Hollingsworth, Pathier and Bouchon2019; Ulrich et al. Reference Ulrich, Vater, Madden, Behrens, Van Dinther, Van Zelst, Fielding, Liang and Gabriel2019). Our mapping of the surface rupture of the Mw 7.5 earthquake south of Palu bay shows that these two segments display a geometrically simple trace with linear, smooth, straight and narrow features, all of which are consistent with the typical geometric characteristics associated with supershear earthquakes (Bouchon et al. Reference Bouchon, Karabulut, Bouin, Schmittbuhl, Vallée, Archuleta, Das, Renard and Marsan2010). Through this unique scenario of coseismic slip partitioning along the middle segment, the vertical component is accommodated onto a secondary fault (Fig. 8b), which enables the main fault to rupture a simple, smooth segment with a pure strike-slip motion, thus making supershear propagation more likely. Furthermore, normal faulting marks an extension that can possibly lower the friction strength on the major fault plane, hence favouring supershear faulting. Although there is an 8 km long bend that may have prevented the rupture from propagating southward, the mature properties and simple geometry (e.g. narrow, straight) of the fault segment south of the bend enabled the rupture to propagate at a supershear velocity. In addition, a maximum left-lateral displacement of 5.5 ± 0.1 m during the Palu earthquake occurs on the middle segment, which encourages a condition to localize the supershear rupture propagation there. Only one of these three segments is the northern segment that does not show mature properties of geometrically simple, smooth structures. Studies of relations between earthquake slip and fault properties suggest that rupture speeds are controlled by the along-strike changes of fault maturity (Bruhat et al. Reference Bruhat, Fang and Dunham2016; Huang et al. Reference Huang, Ampuero and Helmberger2016; Perrin et al. Reference Perrin, Manighetti, Ampuero, Cappa and Gaudemer2016). Rupture propagation speeds are faster along the most mature sections, while along the most immature sections ruptures travel at subshear velocities. From this point, conditions of the northern segment likely enabled only a subshear speed. Thus, the 2018 rupture might not have propagated at a supershear speed initially as argued by Bao et al. (Reference Bao, Ampuero, Meng, Fielding, Liang, Milliner, Feng and Huang2019), but only at a subshear speed in the northern segment, while in the middle and southern segments it propagated at a speed much faster than that which they proposed, 4.1 m s−1.
4.c. The Palu earthquake tsunami is owed to a normal slip on the fault within Palu bay
The other issue is that the Mw 7.5 Palu earthquake generated a devastating tsunami, which is commonly associated with earthquakes occurring on thrust or reverse faults in subduction zones (Carvajal et al. Reference Carvajal, Araya-Cornejo, Sepúlveda, Melnick and Haase2018; Mikami et al. Reference Mikami, Shibayama, Esteban, Takabatake, Nakamura, Nishida, Achiari, Rusli Marzuki, Marzuki, Stolle, Krautwald, Robertson, Aránguiz and Ohira2019; Omira et al. Reference Omira, Dogan, Hidayat, Husrin, Prasetya, Annunziato, Proietti, Probst, Paparo, Wronna, Zaytsev, Pronin, Giniyatullin, Putra, Hartanto, Ginanjar, Kongko, Pelinovsky and Yalciner2019; Ulrich et al. Reference Ulrich, Vater, Madden, Behrens, Van Dinther, Van Zelst, Fielding, Liang and Gabriel2019). However, a strike-slip earthquake can also generate a tsunami when the submarine faulting has a component of vertical displacement, or when it triggers a landslide. The post-earthquake bathymetry within Palu bay defines a trans-tensional graben along the submarine channel that is indicated to be bounded by the Palu–Koro fault (Frederik et al. Reference Frederik, Adhitama, Hananto, Sahabuddin, Irfan, Moefti, Putra and Riyalda2019). Based on the bathymetric data, no apparent evidence of recent slumping and lateral spreading was observed on the bay floor, suggesting that a submarine landslide as the cause of the tsunami can be excluded. Although the 2018 rupture was not clearly shown due to the limit resolution of the bathymetric data, a significant submarine normal faulting was observed in seismic and geodetic studies (Fang et al. Reference Fang, Xu, Wen, Wang, Xu, Zhao and Yi2019; Ulrich et al. Reference Ulrich, Vater, Madden, Behrens, Van Dinther, Van Zelst, Fielding, Liang and Gabriel2019). As opposed to the viewpoint that dip-slip is the main cause of the tsunami, some researchers ascribe the tsunami to submarine landslides. Most proponents of the landslide-induced tsunami hypothesis obtained their results from numerical simulations based on data of remote-sensing images. Some studies indicate that tsunami waves were generated by coastal landslide sources (Takagi et al. Reference Takagi, Pratama, Kurobe, Esteban, Aránguiz and Ke2019; Aránguiz et al. Reference Aránguiz, Esteban, Takagi, Mikami, Takabatake, Gómez, González, Shibayama, Okuwaki, Yagi, Shimizu, Achiari, Stolle, Robertson, Ohira, Nakamura, Nishida, Krautwald, Goseberg and Nistor2020); however, they cannot explain the tsunami-wave records (Liu et al. Reference Liu, Higuera, Husrin, Prasetya, Prihantono and Diastomo2020). Detailed landslide simulations are unable to consider the physical model of a landslide tsunami in order to investigate the possibility of a submarine landslide source. Hence, they underestimate tsunami excitation due to underwater fault movements but solely attributed to massive underwater landslides. Coseismic deformation studies reveal a primarily horizontal strike-slip motion, with a vertical slip component of 1–3 m along the Palu bay section (e.g. Jamelot et al. Reference Jamelot, Gailler, Heinrich, Vallage and Champenois2019; Socquet et al. Reference Socquet, Hollingsworth, Pathier and Bouchon2019; Yolsal-Çevikbilen & Taymaz Reference Yolsal-Çevikbilen and Taymaz2019), suggesting a tectonic tsunami source. Our field investigation also suggests possible rupture on a normal fault strand in Palu bay, which is probably the continuation of the part observed in the Palu valley, and could have a similar expression. Our observations support the findings from seismic and geodetic data that a left-lateral strike slip of up to ∼5.5 m with normal slip of up to ∼2 m occurred along the fault part around Palu bay (Fang et al. Reference Fang, Xu, Wen, Wang, Xu, Zhao and Yi2019; Ulrich et al. Reference Ulrich, Vater, Madden, Behrens, Van Dinther, Van Zelst, Fielding, Liang and Gabriel2019). Normal slip of the fault in Palu bay may have induced a large portion of the tsunami waves, and those coastal landslides may make some contribution to the tsunami but not be the main tsunami source. Thus, we can speculate that the considerable normal slip favours tsunami genesis.
5. Conclusions
By mapping the surficial breaks through field investigations complemented with detailed analysis of high-resolution satellite images, we document geometric and geomorphic features of the surface ruptures of the Palu Mw 7.5 earthquake along the Palu–Koro fault. Our investigation shows that the fault exhibits a complex transforming behaviour, which demonstrates the features of the Palu–Koro fault as a strike-slip fault connecting subduction zones. The transtensive behaviour of the middle segment of the rupture, which contains a substantial normal slip, accounts for the generation of the large tsunami. Our results for the surface rupture geometry support the Palu earthquake as a supershear event.
Supplementary material
To view supplementary material for this article, please visit https://doi.org/10.1017/S0016756822000012
Acknowledgements
This study was funded by the National Nonprofit Fundamental Research Grant of China, IGCEA (IGCEA1901), the National Research and Development Program of China (2017YFC1500101) and the National Natural Science Foundation of China (42072250). We thank Professor Pavel Adamek (Nanyang Technological University) and Dr Murat Tamer for comments and English grammar corrections. We also thank editor Olivier Lacombe and anonymous reviewers for thoughtful comments that significantly improved the manuscript.
Declaration of Competing Interests
The authors declare no competing interests.