1. Introduction
Fault rocks such as pseudotachylytes or fault gouges are classically thought to be a record of seismic slip events (e.g. Sibson, Reference Sibson1975; Lin, Reference Lin1996, Reference Lin2008; Chester & Chester, Reference Chester and Chester1998) and are consequently considered as ‘earthquake fossils’ (see Lin, Reference Lin2008), whose finding can help to shed light on brittle processes occurring at various depths within the crust (e.g. Sibson, Reference Sibson1975; Allen, Reference Allen2005; Di Toro et al. Reference Di Toro, Nielsen and Pennacchioni2005a, b, Reference Di Toro, Hirose, Nielsen, Pennacchioni and Shimamoto2006).
Tectonic pseudotachylytes, formed by frictional melting during coseismic localized slip along a fault (i.e. typically at 1 cm s−1 to 1 m s−1 slip rates; e.g. Philpotts, Reference Philpotts1964; Sibson, Reference Sibson1975) are frequently associated with cataclasites and/or mylonites (e.g. Chu et al. Reference Chu, Hwang, Shen and Yui2012; Rowe et al. Reference Rowe, Kirkpatrick and Brodsky2012; Kirkpatrick & Rowe, Reference Kirkpatrick and Rowe2013; Wang et al. Reference Wang, Li, Janssen, Sun and Si2015), indicating that, despite being the result of frictional mechanisms, they can also form below the brittle–ductile transition within the crust. This evidence can be explained assuming episodic rapid slip events within a general framework of slow ductile deformation (e.g. Handy & Brun, Reference Handy and Brun2004). At lower crustal conditions, this process can be also repeated, with alternating brittle and ductile deformation phases (e.g. Angiboust et al. Reference Angiboust, Kirsch, Oncken, Glodny, Monie’ and Rybacki2015; Menegon et al. Reference Menegon, Pennacchioni, Malaspina, Harris and Wood2017; Behr et al. Reference Behr, Kotowsky and Ashley2018; Hawemann et al. Reference Hawemann, Mancktelow, Wex, Camacho and Pennacchioni2018; Menant et al. Reference Menant, Angiboust, Monié, Oncken and Guigner2018).
Generally speaking, the relative paucity of pseudotachylyte veins in the geological record has fed a long-lasting debate (e.g. Sibson & Toy, Reference Sibson, Toy, Abercombie, McGarr, Kanamori and Di Toro2006), on whether this should be ascribed to: (i) the substantial rarity of frictional melting episodes, (ii) the occurrence of mechanisms different from frictional melting during seismic events in subduction environments, such as dehydration embrittlement, especially in a fluid-rich context such as the subduction channel, resulting in the development of different types of fault rocks (FRs) (e.g. mineral veins; Angiboust et al. Reference Angiboust, Agard, Raimbourg, Yamato and Huet2011), or (iii) the intrinsic difficulty of pseudotachylyte preservation (e.g. Kirkpatrick et al. Reference Kirkpatrick, Shipton and Persano2009; Price et al. Reference Price, Johnson, Gerbi and West2012; Kirkpatrick & Rowe, Reference Kirkpatrick and Rowe2013). In addition, pseudotachylyte occurrences are not uniformly distributed in the various lithotypes. According to the original estimates reported by Sibson & Toy (Reference Sibson, Toy, Abercombie, McGarr, Kanamori and Di Toro2006), less than 3 % of the total known pseudotachylytes are hosted in mafic blueschist/eclogite facies rocks, most of them being rather hosted within quartzo-feldspathic crystalline rocks (e.g. Sibson & Toy, Reference Sibson, Toy, Abercombie, McGarr, Kanamori and Di Toro2006; Kirkpatrick et al. Reference Kirkpatrick, Dobson, Mark, Shipton, Brodsky and Stuart2012; Rowe et al. Reference Rowe, Kirkpatrick and Brodsky2012; Di Vincenzo et al. Reference Di Vincenzo, Rossetti, Viti and Balsamo2013; Wang et al. Reference Wang, Li, Si, Sun and Huang2014, Reference Wang, Li, Janssen, Sun and Si2015; Ferré et al. Reference Ferré, Geissmann, Chauvet, Vauchez and Zechmeister2015; Zheng et al. Reference Zheng, Li, Sun, Wang, Zhang, Li and Cao2016; Stewart & Miranda, Reference Stewart and Miranda2017; Wex et al. Reference Wex, Mancktelow, Hawemann, Camacho and Pennacchioni2017). However, in recent years the study of FRs hosted within subducted ophiolites that experienced high-pressure/low-temperature (HP/LT) metamorphism has become a significant focus of research, and findings of pseudotachylytes in blueschist/eclogite rocks have significantly increased in number (e.g. Austrheim & Boundy, Reference Austrheim and Boundy1994; Austrheim & Andersen, Reference Austrheim and Andersen2004; Rowe et al. Reference Rowe, Moore and McKeiman2005; John & Schenk, Reference John and Schenk2006; Andersen et al. Reference Andersen, Mair, Austrheim, Podladchikov and Vrijmoed2008, 2014; John et al. Reference John, Medvedev, Rüpke, Andersen, Podladchikov and Austrheim2009; Deseta et al. Reference Deseta, Andersen and Ashwal2014a, b; Magott et al. Reference Magott, Fabbri and Fournier2016, Reference Magott, Fabbri and Fournier2017; Scambelluri et al. Reference Scambelluri, Pennacchioni, Gilio, Bestmann, Plümper and Nestola2017).
In this paper, we present the first evidence of FR relics, interpreted as pseudotachylyte injections, for a metaophiolite unit (Diamante–Terranova unit; DIATU) exposed in northern Calabria. This finding can contribute to shedding additional light on the factors influencing the genesis and preservation in the geological record of pseudotachylytes, to better understanding the ductile and brittle deformation mechanisms acting under the HP/LT conditions experienced by the DIATU during the subduction–exhumation process, and the palaeoseismic activity of Calabria, possibly witnessing for a long-lived activity (at least since the Eocene and currently ongoing) for this part of the orogenic chain.
2. Geological setting
In northern Calabria, the exposed thrust-sheet pile includes some slivers of HP/LT metaophiolites. These are embedded between Palaeozoic continental crust and related Mesozoic cover rocks (Calabria–Peloritani Terrane) on the top, and Triassic–Miocene continental margin successions (Apennine Platform units; Adria plate) at the bottom (e.g. Bonardi et al. Reference Bonardi, Cavazza, Perrone, Rossi, Vai and Martini2001; Iannace et al. Reference Iannace, Vitale, D’Errico, Mazzoli, Di Staso, Maiacone, Messina, Reddy, Somma, Zamparelli, Zattin and Bonardi2007; Vitale & Ciarcia, Reference Vitale and Ciarcia2013; Fedele et al. Reference Fedele, Tramparulo, Vitale, Cappelletti, Prinzi and Mazzoli2018; Vitale et al. Reference Vitale, Ciarcia, Fedele and Tramparulo2019). The Diamante–Terranova unit (Cello et al. Reference Cello, Invernizzi and Mazzoli1996; Fedele et al. Reference Fedele, Tramparulo, Vitale, Cappelletti, Prinzi and Mazzoli2018 and references therein), cropping out mainly in the Diamante area (on the Tyrrhenian side of northern Calabria; Fig. 1), is characterized by Jurassic metabasalts and a Cretaceous metasedimentary cover. It experienced a tectonic burial within a subduction channel, following the subduction of the Ligurian oceanic lithosphere beneath the Calabria–Peloritani Terrane (CPT).
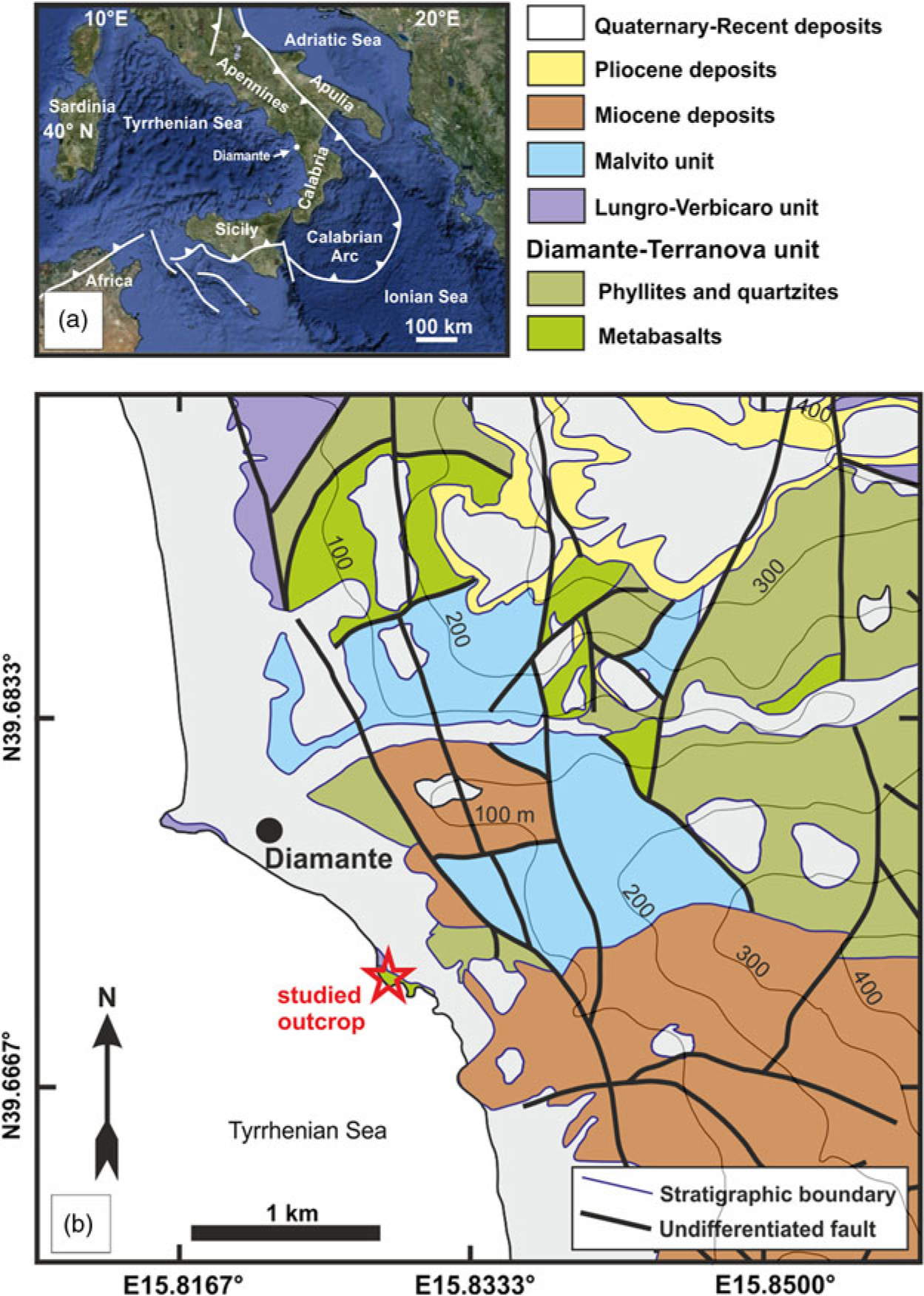
Fig. 1. (a) Satellite image showing the Diamante location in the framework of the southern Apennines – Calabria Peloritani Terrane system (with the position of the inferred orogenic front). (b) Simplified geological map (after Fedele et al. Reference Fedele, Tramparulo, Vitale, Cappelletti, Prinzi and Mazzoli2018) of the Diamante area showing the location where the investigated fault rocks (FRs) crop out (red star).
The DIATU metabasalt rocks suffered a metamorphic cycle peaking at T = 350–390 °C and P = 0.8–1.1 GPa during the Eocene (46.7 ± 0.4 Ma; Shimabukuro et al. Reference Shimabukuro, Wakabayashi, Alvarez and Chang2012), developing a mineral assemblage mainly consisting of glaucophane, lawsonite, chlorite and pumpellyite, with accessory quartz, titanite, albite and apatite (Fedele et al. Reference Fedele, Tramparulo, Vitale, Cappelletti, Prinzi and Mazzoli2018). At the outcrop scale, metabasalts generally appear as well-foliated rocks defined by alternating blue and green layers (Fig. 2a) consisting of glaucophane+lawsonite- and lawsonite+chlorite+pumpelliyte-dominated assemblages, respectively. The tectonic evolution was accompanied by a complex ductile and brittle polyphase deformation (Fedele et al. Reference Fedele, Tramparulo, Vitale, Cappelletti, Prinzi and Mazzoli2018). The early ductile deformation stage, defined by three deformation phases (D1–D2–D3), occurred close to the HP/LT metamorphic peak, during the tectonic exhumation of the DIATU within the subduction channel. The widespread occurrence of syn-kinematic lawsonite crystals associated with the ductile deformation allowed Fedele et al. (Reference Fedele, Tramparulo, Vitale, Cappelletti, Prinzi and Mazzoli2018) to estimate the pure and simple shear strain components by means of a vorticity analysis. The D1–D2 deformation was characterized by ductile non-coaxial strain with a dominant flattening component, developing an S1 foliation transposed into a main S2 crenulation cleavage (Fig. 2b). The late folding (D3) developed only a weak S3 crenulation cleavage (Fig. 2c). The subsequent deformation stages (D4–D5–D6) occurred at shallower crustal levels without metamorphic recrystallization.

Fig. 2. (a) Representative field photograph of the glaucophane–lawsonite-bearing Diamante blueschist metabasalts. Plane polarized light view photomicrographs of the Diamante blueschist metabasalts: (b) S1 and S2 foliations; (c) F3 fold and related S3 foliation. (d) Field photograph of a quartz+epidote pod embedded within the Diamante metabasalts; (e) pod hosting a non-planar fracture filled with a meander-like fault rock layer. (f) Representative crossed polarized light view photomicrograph of a quartz (Qtz)+epidote(Ep) pod.
Metabasalts of the DIATU embed quartz+epidote pods up to 10 cm thick, originally developed as veins during the D1 deformation stage, and subsequently folded and boudinaged (Fig. 2d). At the mesoscale observation, pods host meander-like thin dark seams of very fine-grained material enclosed within fractures, locally displaying tabular, curvilinear and meander-like shapes (Fig. 2e), identified as FR after optical and scanning electron microscope observations (see below).
3. Microstructural observations of the DIATU pods
Several samples of the quartz+epidote pods, embedded within the DIATU metabasalts and hosting the investigated FRs, were collected and subjected to detailed microstructural and petrographic investigations. Selected samples were then investigated using optical microscope and a JEOL JSM-5310 Scanning Electron Microscope (SEM) at the laboratories of the DiSTAR (Napoli). Backscattered electron (BSE) images were acquired during SEM observations, and the mineral phases were qualitatively analysed by EDS (energy dispersive spectrometry) using an Oxford Instruments Microanalysis Unit equipped with an INCA X-act detector. Measurements were performed with an INCA X-stream pulse processor using a 15 kV primary beam voltage, 50–100 μA filament current, variable spot sizes and 50 s of acquisition time. Observations at both the optical microscope and at the SEM revealed the existence of several different structures within the investigated pod samples, including relics of fine-grained FRs and various types of late structures such as quartz-mylonites, boudins and minor thrust faults. These are described in detail in the following sections.
3.a. Host rock
Pods generally consist of variably comminuted (up to a few mm in size) broken crystals of epidote with subordinate lawsonite and glaucophane (Figs 2f, 3c–f, 4a–d, 5a–c and 6a–c) embedded by quartz and lesser calcite, chlorite and albite (Fig. 3d). The abundant quartz suggests that pods are veins related to fluid-induced brecciation (hydrofracturing). The average crystal size of broken epidote crystals is only locally observed to quite regularly decrease towards the contact with the FR relics (Fig. 3c), whereas more frequently no systematic variation of the grain size can be observed (Fig. 3e, f).

Fig. 3. Representative backscattered electron (BSE) scanning electron microscope (SEM) images of the investigated quartz+epidote pod samples hosting fault rock (FR) relics recovered in the DIATU metabasalts. (a) Red false colours highlighting Ti concentrations discriminating titanite (Ttn) from survivor clasts within a FR at the contact with a large fractured epidote crystal (with fractures filled with chlorite (Chl) and quartz (Qtz)] of the host pod (sample DIA17a). (b) Close-up view of the previous picture, showing high concentrations of survivor clasts (mainly crystals of epidote, Ep and Chl) embedded in a FR matrix dominated by titanite. (c) Vein showing a decrease in grain size approaching the contact with the FR, which features both a glaucophane-rich and a foliated facies (sample DIA17b; Cal = calcite). (d) Close-up view of the previous picture showing clasts of Ep within a cement made of Cal and albite (Ab). (e, f) Focus on a contact [note the jagged indented outline, especially in (f)] between the quartz+epidote pod host rock and a foliated FR (sample DIA17b; Gln = glaucophane).
3.b. Fault rock relics
Relics of FRs typically appear as yellowish-brown to brown-dark films when observed under the optical microscope in plane polarized light view (Figs 4, 6). FRs show different degrees of foliation development, including: (i) no foliation (Fig. 4a), (ii) a weakly developed foliation (Fig. 4b–d) and (iii) a well-developed foliation (Fig. 6). Types (i) and (ii) contain survivor clasts more or less homogeneously dispersed in a finer matrix. FRs show variably coloured streaks, generally mantling asperities of the host rock (Figs 4, 6). Locally, FRs form flow folds that can be observed both in the main vein (Fig. 4c) and in small injection veins intruding in the host rock (Fig. 4d). More frequently, FRs show a well-marked foliation that is particularly evident at the SEM, defined by layers of different mineral composition (different tones of grey) and alignment of survivor clasts (Fig. 5a–f). These latter, generally formed by broken crystals of epidote, occur in variable amounts, occasionally reaching extremely abundant concentrations (approximately ∼50 vol. %; Fig. 3a, b) and locally forming lens/ribbon-shaped aggregates (Fig. 5c–e). Less common lawsonite survivor clasts are occasionally boudinaged and show evidence for growth of glaucophane fibres in the necks (Fig. 5e).
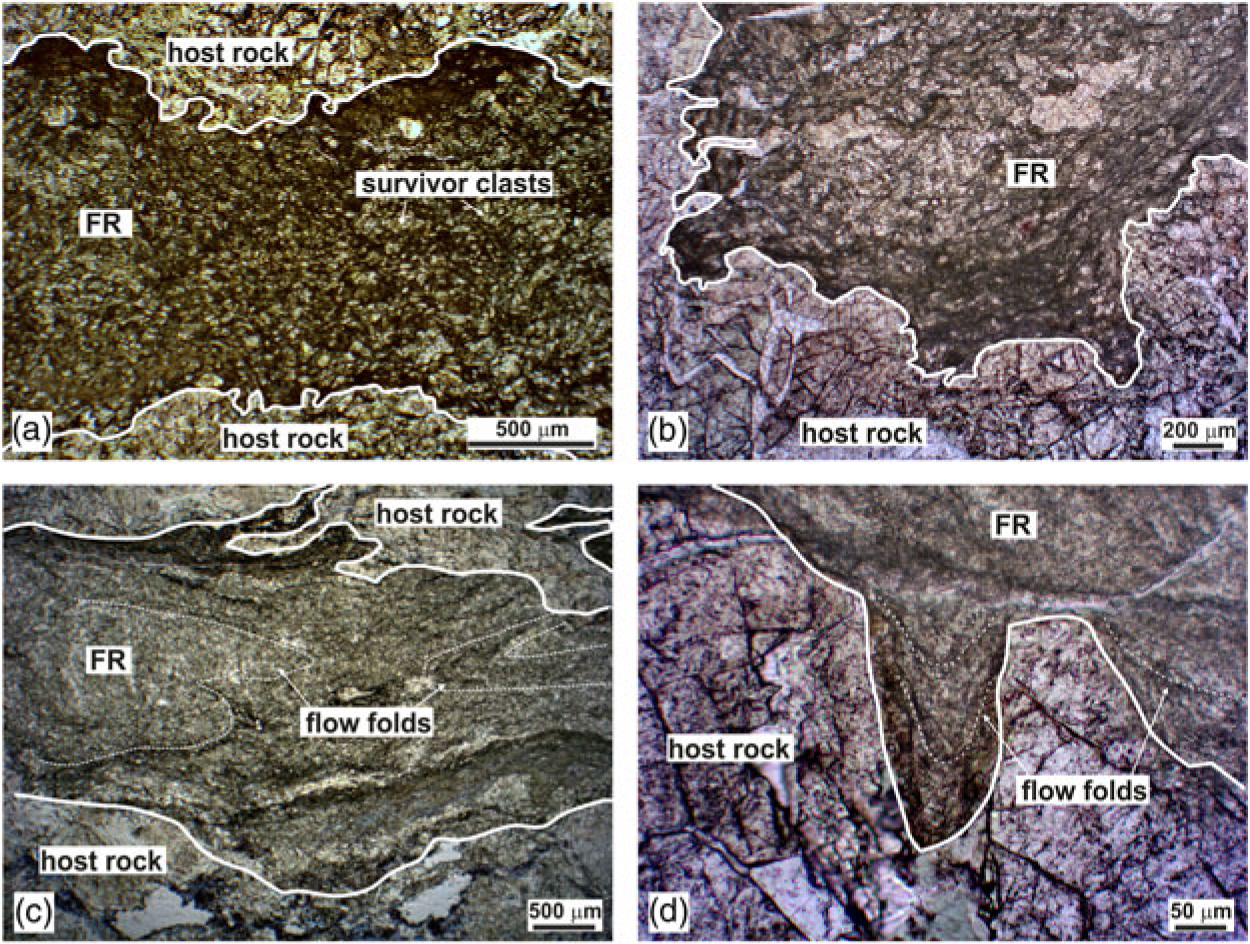
Fig. 4. Representative plane polarized light view photomicrographs of the investigated quartz+epidote pod samples hosting fault rock (FR) relics recovered in the DIATU metabasalts (sample DIA17), showing (a) high concentrations of survivor clasts; (b) amoeboid injections within host rock; (c), weakly foliated appearance with flow folds; and (d) small injection veins within the host rock.
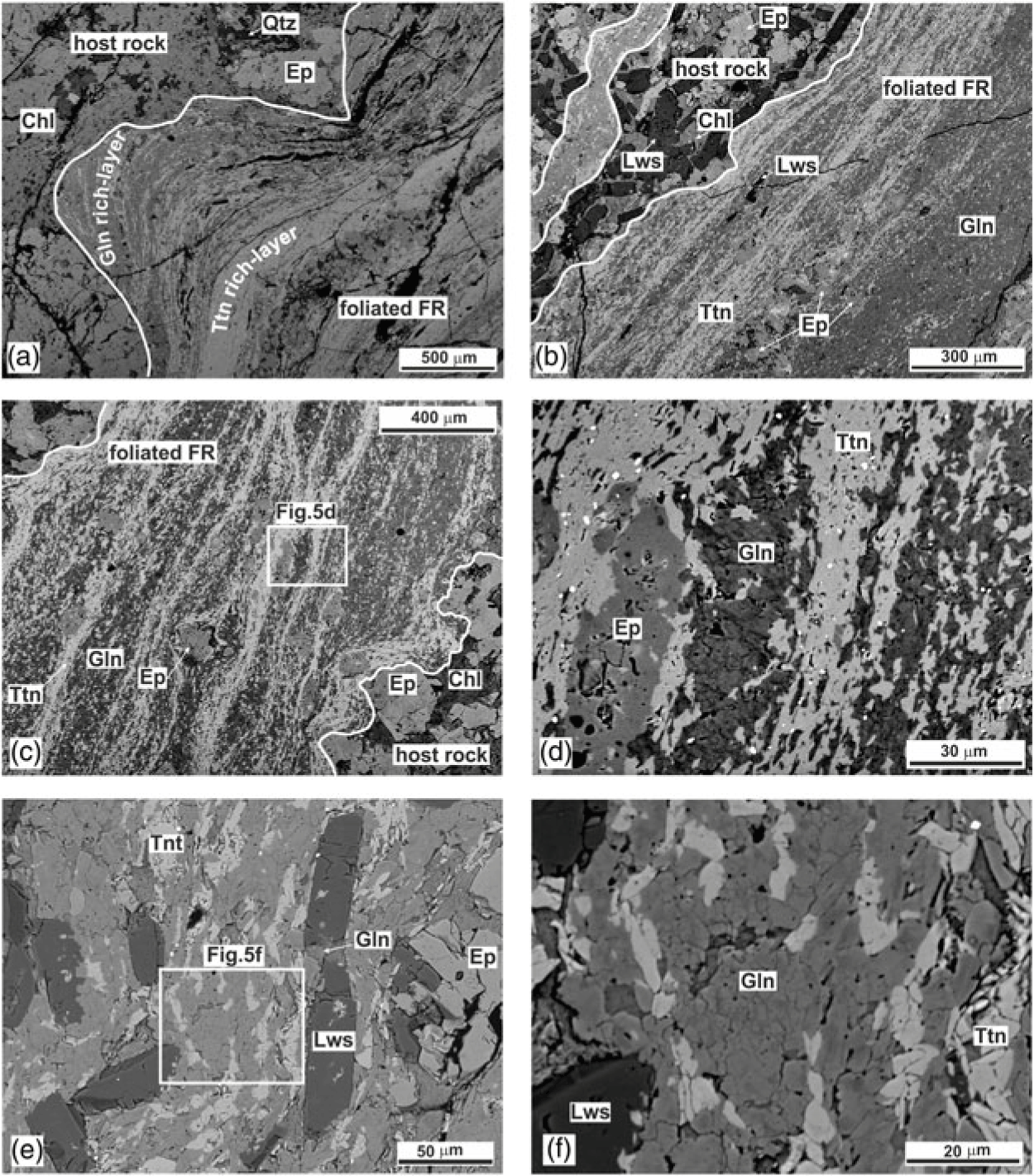
Fig. 5. Representative BSE SEM images illustrating some characteristic textural features of the investigated fault rock (FR) relics hosted in the quartz+epidote pod samples recovered in the DIATU metabasalts. (a) Foliated FR consisting of very small crystals of titanite (Ttn, light grey), and glaucophane (Gln, darker grey), forming a festoon-like structure mantling host rock asperities [the same structure is shown in (c) at the optical microscope] (DIA17b; Chl = chlorite, Qtz = quartz). (b) Foliated FR embedding layers of the host rock made of crystals of lawsonite (Lws), epidote (Ep) and Gln (DIA17b). (c) Foliated FR showing sparse Ep survivor clasts (sample DIA17) close to the contact with the host rock (right end), with white rectangle indicating the area of the enlarged view of (d), showing the FR microcrystalline texture. (e) Foliated FR showing lawsonite (Lws) survivor clasts (sample DIA17), with white rectangle indicating the area of the enlarged view of (f), where the microcrystalline texture of the FR is again evidenced.

Fig. 6. Representative plane polarized light view photomicrographs illustrating some characteristic textural features of the investigated foliated fault rock (FR) relics hosted in the quartz+epidote pod samples recovered in the DIATU metabasalts (sample DIA17b). (a) Low-magnification view of a foliated FR seam. (b, c) Festoon-like structures of foliated FR (flow fold) filling embayments of the host rock (with higher concentrations of epidote survivor clast layers in (c)). (d) Foliated FR with very abundant layers of epidote survivor clasts. (e) Close-up view of a mantled epidote survivor clast indicating a dextral sense of shear.
High-magnification BSE SEM images of foliated FRs reveal the presence of very fine crystals (<10 µm) of glaucophane and titanite, typically forming regular alternations of monomineralic layers (respectively darker grey and lighter grey; e.g. Fig. 5f). Crystals generally display a relatively well-formed euhedral habit, although we also observed some subhedral to anhedral shapes (Fig. 5f).
Crystal aggregates typically form thin anastomosing seams wrapping epidote survivor clasts and mantling host rock asperities, locally resulting in festoon-like structures (flow folds) within host rock embayments (Fig. 5a) or amoeboid injection structures (Fig. 4b). The dark levels within foliated FRs observed with the optical microscope (e.g. Fig. 6c) proved to be enriched in titanite crystals when analysed at the SEM (Fig. 5a). Survivor clasts of epidote are commonly mantled by fine-grained fragments, occasionally showing a δ symmetric shape (Passchier & Trouw, Reference Passchier and Trouw1996), indicating a simple shear component during the emplacement of the FR (Fig. 6e).
3.c. Late structures
Several late deformation structures, clearly following the development of the FRs, can be locally observed both in the pod and FRs. Quartz is locally deformed, with fine to coarse crystals (up to 200 µm) showing elongate shapes and a typical curved mylonitic foliation (Fig. 7a). The largest of such quartz crystals also display undulose extinction and the development of subgrains (Fig. 7b).

Fig. 7. Representative photomicrographs illustrating some characteristic late structures observed in the investigated quartz+epidote pod samples hosting fault rock (FR) relics relics recovered in the DIATU metabasalts (sample DIA17). Crossed polarizers view of a quartz (Qtz)-mylonite showing (a) sigmoidal foliation and (b) formation of subgrains (high-interference colour crystals are epidote crystals). Plane polarized light view of (c) boudinaged FR with glaucophane (Gln) fibres growing in the neck, (d) reverse-fault deforming the foliated FR (Cal = calcite).
FRs are locally boudinaged, with necks separated by glaucophane fibres and pinch and swell structures (Fig. 7c). In addition, FRs are also observed to be deformed by small reverse faults with slickenside fibres of calcite and quartz (Fig. 7d).
4. Discussion
4.a. The DIATU fault rock relics: pseudotachylytes or ultracataclasites?
Though appearing only in very small occurrences within the investigated quartz+epidote pods, the studied relics of FRs possibly represent the repository of some interesting clues regarding the deformation mechanisms affecting the DIATU rocks during the subduction–exhumation process. Their nature as injection vein relics of FRs is evidenced by their peculiar mineral composition consisting of very fine-grained glaucophane and titanite crystals, indicative of bulk source rock composition different from that of the host quartz+epidote pods (where such mineral phases are largely subordinate). Such FR injections could be interpreted as representing (i) mylonites, (ii) melt-originated pseudotachylytes or (iii) fluidized ultracataclasites. Since no shear plane was observed (FRs are clearly injected within fractures) and no ductile mechanism can explain the high concentration of titanite within the FRs with respect to the metabasalt photolith, hypothesis (i) can be easily discarded.
Although there is a substantial genetic difference between tectonic pseudotachylytes and ultracataclasite veins (including crush-origin pseudotachylyte-like veins; e.g. Lin, Reference Lin1996, Reference Lin2008; Wenk et al. Reference Wenk, Johnson and Ratschbacher2000; Janssen et al. Reference Janssen, Wirth, Lin and Dresen2010a, b; Lin et al. Reference Lin, Yamashita and Tanaka2013) representing the injection of a fault gouge into fractures cross-cutting the slip plane of a fault zone, assessing whether the observed FRs should be considered as pseudotachylyte or fluidized ultracataclasites is not straightforward.
A first-level difficulty is simply related to the overall similar appearance of the two types of rocks, including the fact that both have been demonstrated to form veins with similar aspect ratios (∼0.2; Rowe et al. Reference Rowe, Kirkpatrick and Brodsky2012), and that amorphous material can also occur in ultracataclasites (likely formed by mechanochemical effects during the comminution process; e.g. Ozawa & Takizawa, Reference Ozawa and Takizawa2007; Pec et al. Reference Pec, Stünitz, Heilbronner, Dury and Capitani2012). Furthermore, pseudotachylytes and ultracataclasites are commonly associated with and gradational to each other (as well as with ultramylonites), so they might be indistinguishable from each other even at the microscope scale.
This finally brings us to the core of the problem, as the presence of (metastable) glass and/or ultrafine-grained material makes both pseudotachylytes and ultracataclasites extremely prone to obliteration (though sometimes glass can be well preserved; e.g. Andersen & Austrheim, Reference Andersen and Austrheim2006; Andersen et al. Reference Andersen, Mair, Austrheim, Podladchikov and Vrijmoed2008) via recrystallization, alteration, hydration and deformation (see Kirkpatrick & Rowe (Reference Kirkpatrick and Rowe2013) for an exhaustive synthesis). As a consequence, the most diagnostic features of both pseudotachylytes (i.e. chilled margins, spherulites, dendritic/skeletal crystals suggesting rapid quenching of a melt) and ultracataclasites (i.e. ultrafine rock fragments marking a clear crush origin) are not always clearly recognizable. The latter is likely the case of the investigated DIATU FR relics. In fact, they have recrystallized in blueschist facies conditions, as indicated by: (i) presence of glaucophane; (ii) host quartz+epidote pods formed in similar HP/LT conditions with respect to enclosing metabasalts (i.e. T = 350–390 °C and P = 0.8–1.1 GPa, corresponding to a depth of ∼30 km; Fedele et al. Reference Fedele, Tramparulo, Vitale, Cappelletti, Prinzi and Mazzoli2018); (iii) some FRs being locally boudinaged with glaucophane fibres in the neck (Fig. 6c). In addition, the host quartz+epidote pods likely also experienced a complex deformation history, as testified by the occurrence of ductile and brittle late structures. Pods clearly pre-date the FR relics, as the latter are hosted within them as injection veins (Figs 3e, f, 4c–f, 5a–c). A subsequent ductile deformation stage is also evident, as recorded by dynamic recrystallization of quartz crystals (Fig. 4a, b). The evidence that FR veins do not cut the quartz-mylonites suggests that the ductile process was also subsequent to the FR injections. Hence, this complex history of recrystallization and deformation has likely overprinted the primary textural features of the investigated FRs. However, a possibly diagnostic feature is represented by the common presence of a clear distinction between titanite- and glaucophane-dominated layers (e.g. Figs 3e, f, 5a–d). This could be achieved through flow differentiation during melt injection, consistent with the common presence of flow structures (e.g. Figs 4e, f, 5a, 6b, c), suggesting non-equilibrium melting processes or lack of melt homogenization (e.g. Chu et al. Reference Chu, Hwang, Shen and Yui2012; Kirkpatrick & Rowe, Reference Kirkpatrick and Rowe2013; Deseta et al. Reference Deseta, Andersen and Ashwal2014a, b). Flow banding seems evident even at the optical microscope scale, as also highlighted by the common wrapping of epidote survivor clasts, represented not only by isolated clasts (e.g. Figs 3b, 5d), but also by boudinaged levels (Fig. 6d, e).
In this framework, the occurrence of flow streaks with different colour and composition could be related to viscous flow of molten material characterized by a non-uniform chemical composition (e.g. Toyoshima, Reference Toyoshima1990). Pseudotachylyte streaks frequently form tight to isoclinal folds, with a geometry of similar-type folds (flow folds; Berlenbach & Roering, Reference Berlenbach and Roering1992; O’Hara, Reference O’Hara2001; Theunissen et al. Reference Theunissen, Smirnova and Dehandschutter2002). In the analysed FRs, the flow foliation is curved in the central part of the vein, and becomes parallel to the vein margin, as observed in some lateral ‘subsidiary’ injection veins (Fig. 4e, f) or in some embayments within the host rock (Figs 3f, 5a, 6b, c).
Although foliation is a feature commonly ascribed to pseudotachylytes, it should however be noted that in some cases it has also been reported for ultracataclasites (e.g. Magloughlin & Spray, Reference Magloughlin and Spray1992; Chester & Chester, Reference Chester and Chester1998; Cashman & Cashman, Reference Cashman, Cashman, Snoke and Barnes2006; Kirkpatrick & Rowe, Reference Kirkpatrick and Rowe2013; Wang et al. Reference Wang, Li, Janssen, Sun and Si2015), so it cannot be considered to be a fully diagnostic feature for melt-originated FRs. However, well-developed flow folds, such as those observed in the analysed FRs, are never reported for ultracataclasites, which are more likely to display a homogeneous mineral composition, rather than a well-developed compositional banding like that observed for the DIATU FRs.
Relic survivor clasts (as well as their size distribution; e.g. Ray, Reference Ray2004; Lin, Reference Lin2008; Price et al. Reference Price, Johnson, Gerbi and West2012) can be of some use in discriminating between pseudotachylytes and ultracataclasites, but similarly do not provide unequivocal clues in this sense. The presence of embayed clasts, testifying to incomplete melting of fragments from the source rock, is generally taken as supporting a melting-related origin for these FRs (e.g. Kirkpatrick & Rowe, Reference Kirkpatrick and Rowe2013). The most common survivor clasts observed in the investigated FRs are instead from the host quartz+epidote pods, showing rounded and embayed morphologies (e.g. Figs 3b, 5d, e, f). This feature is in contrast to the typical angular shape expected for a clast within an ultracataclasite. The corroded appearance of the epidote crystal in Figure 5d could thus be taken as evidence of a partially molten survivor clast that reacted with an infiltrating melt. Similarly, the lawsonite crystals of Figure 5e, as well as the few subhedral/anhedral crystals of glaucophane and titanite surrounded by euhedral crystals in the FR vein of Figure 5f, can be interpreted as partially melted relics dragged from the source metabasalt.
In the light of the above evidence, we think that the DIATU FRs can be more confidentially interpreted as pseudotachylytes, rather than ultracataclasites. In any case, it should be noted that even if the investigated FRs (or at least some of them) are considered to have originated from a granular flow rather than from a quenching melt, their textural features are still consistent with a process related to high slip rates. The genesis of ultracataclasite injections has indeed been ascribed to at least three different mechanisms: (i) slow deposition of clay minerals-rich materials transported by hydrothermal fluids; (ii) slow intrusion along fractures during aseismic periods (e.g. Lin, Reference Lin1996, Reference Lin2008, Reference Lin2011; Bjørnerud, Reference Bjørnerud2010); and (iii) fast intrusion during seismic slip (e.g. Otsuki et al. Reference Otsuki, Monzawa and Nagase2003; Rowe et al. Reference Rowe, Moore and McKeiman2005, Reference Rowe, Kirkpatrick and Brodsky2012; Ujiie et al. Reference Ujiie, Yamaguchi, Kimura and Toh2007; Brodsky et al. Reference Brodsky, Rowe, Meneghini and Moore2009; Bjørnerud, Reference Bjørnerud2010; Lin, Reference Lin2011; Lin et al. Reference Lin, Yamashita and Tanaka2013). In the DIATU case, mechanism (i) seems unlikely, as a slow flow within a hydrothermal fluid would probably have enriched the veins in clay minerals due to density sorting. Similarly, a slow flow such as is required for mechanism (ii) also seems unconvincing, as it could not allow the sharp separation between glaucophane and titanite observed in some of the investigated FRs. On this basis, the only viable mechanism is (iii), and so we conclude that the investigated FR relics have to be considered as evidence of processes acting at seismic rates.
4.b. A genetic model for the DIATU pseudotachylytes
A model summarizing the overall deformation evolution explaining the genesis of the DIATU pseudotachylyte veins is suggested in Figure 8, based on the evidence presented in the previous section. The earliest deformation stage was mainly in the brittle–ductile regime, during HP/LT metamorphism (Fig. 8a), characterized by low strain rates (e.g. 10−12/10−10 s−1; Spray, Reference Spray2010), with the development of the glaucophane+lawsonite+chlorite+pumpelliyte metabasalt assemblage and the epidote veins. The subsequent activation of a thrust fault (Fig. 8b), defined by very high slip rates (∼10−1/106 s−1; Spray, Reference Spray2010), caused the transition to a dominant brittle deformation style, producing quartz+epidote veins by means of hydraulic fracturing, accompanied by melting, along the fault plane, of glaucophane and titanite in the metabasalts, locally producing pseudotachylyte injection veins. The evidence that the quartz-mylonites do not host pseudotachylytes and the absence of multiple generations of pseudotachylytes suggest that, at least in the analysed rocks, there was no multiple alternation of brittle (fast slip events) and ductile (normal strain rate) deformation stages.

Fig. 8. Cartoon showing the reconstructed evolution for the investigated DIATU pseudotachylytes (PST). Gln = glaucophane; Lws = lawsonite; Ep = epidote; Qtz = quartz.
The peculiar bimineralic composition of the DIATU pseudotachylytes supports the idea that melting must have been extremely selective, as it did not occur either in the epidote veins or in the green lawsonite+chlorite+pumpelliyte-dominanted layers of the metabasalt, but it only affected the glaucophane-dominated (including diffuse titanite accessory crystals) metabasalt layers. This could be explained assuming a decrease of effective normal stress over the fault plane, which hence lowered the frictional heat (Passchier & Trouw, Reference Passchier and Trouw1996), due to the presence of high volumes of fluids. Such fluids can be related to the release of dehydration water by the H2O-rich green layers of the host metabasalt (also including abundant lawsonite), which was also responsible for the development of the aforementioned quartz+epidote veins and fluid-induced brecciation processes. On the other hand, the relatively low porosity and lower water content of glaucophane-rich layers likely allowed the build-up of the effective normal stress, eventually leading to melt generation.
In the successive deformation stages, melt formerly solidified as glass recrystallized under HP/LT conditions to very tiny crystals of glaucophane and titanite. Recrystallization did not erase the original flow banding, which is still evident in the form of differently coloured layers, flow folds, festoon-like structures and minor injections within the host rock. The following deformation phases, characterized by dominant flattening and minor simple shear (Fedele et al. Reference Fedele, Tramparulo, Vitale, Cappelletti, Prinzi and Mazzoli2018), produced (i) the observed pods, from folding and boudinage of the previous quartz+epidote veins, (ii) the quartz-mylonites, from the ductile deformation of the quartz cement of the veins and (iii) the boudinage of the pseudotachylyte veins, with the growth of glaucophane crystals in the necks. Since the studied pseudotachylytes formed during the ductile deformation phases D1–D3 of the HP/LT metamorphic event, a model assuming that fault zone shear melting was preceded and/or followed by mylonitic deformation (Passchier & Trouw, Reference Passchier and Trouw1996; Takagi et al. Reference Takagi, Goto and Shigematsu2000), commonly invoked to suggest a genetic linkage between pseudotachylytes and associated ductile structures, seems far from convincing in the DIATU case. It is more likely that brittle rapid slip events occurred within a general framework of slow deformation characterized by a ductile strain. As a final remark, the observation that pseudotachylyte relics aare totally lacking in the DIATU metabasalt source rocks can possibly give some interesting clues to our understanding of the factors influencing the preservation in the geological record of such delicate FR. We indeed suggest that the selective preservation of the DIATU pseudotachylytes simply reflects the effects of significant tectonic obliteration acting concurrently with recrystallization, which must have completely erased any pseudotachylyte relic originally preserved in the DIATU metabasalts. Such obliteration did not occur in the stiffer quartz+epidote aggregates of the host pods because these must have efficiently shielded the tiny and vulnerable pseudotachylyte veins. This might suggest that the relative rarity of pseudotachylytes is largely dependent on the need for favourable conditions allowing their preservation.
4.c. Palaeotectonic implications
The present recognition of pseudotachylyte relics in the quartz+epidote pods embedded within the DIATU metabasalts offers the opportunity to increase our understanding of the seismogenetic processes active along the subduction interface, and potentially within the subducting lithospheric plate.
In the southern Apennines – CPT system, the occurrence of pseudotachylyte veins has so far been reported only in southern Calabria, within the Hercynian basement of the Serre Massif, namely in greenschist/amphibolite-facies foliated meta-tonalites (Copanello locality; Caggianelli et al. Reference Caggianelli, de Lorenzo and Prosser2005) and in high-grade mafic and felsic granulite-facies rocks (Curinga; Altenberger et al. Reference Altenberger, Prosser, Grande, Günter and Langone2013). As for the latter, Altenberger et al. (Reference Altenberger, Prosser, Grande, Günter and Langone2013) hypothesized that pseudotachylytes resulted from deep-seated seismic events (c. 30 km in depth), occurring during the activity of the Curinga–Girifalco Fault (Schenk, Reference Schenk1980; Langone et al. Reference Langone, Gueguen, Prosser, Caggianelli and Rottura2006), within the geodynamic framework of the Eocene piling-up of CPT slivers. Our results provide evidence that pseudotachylyte veins formed at similar depth synchronously with the HP/LT metamorphic ductile deformation affecting the subducted thrust sheets during the Eocene closure of the Ligurian Ocean (e.g. Vitale & Ciarcia, Reference Vitale and Ciarcia2013; Vitale et al. Reference Vitale, Ciarcia, Fedele and Tramparulo2019). The recognized pseudotachylyte veins thus testify to a long-lasting intermediate-depth seismicity in the CPT/Ligurian domain, active at least from the Eocene until the presently ongoing Ionian subduction beneath Calabria, where deep to shallow earthquakes frequently occur (e.g. Castello et al. Reference Castello, Selvaggi, Chiarabba and Amato2006). Similar evidence for early Eocene (55–46 Ma) intermediate-depth seismicity, based on the recognition of pseudotachylyte veins, has recently been reported by Scambelluri et al. (Reference Scambelluri, Pennacchioni, Gilio, Bestmann, Plümper and Nestola2017) for the oceanic gabbros and mantle peridotites of the Lanzo Massif, belonging to the northern sector of the Ligurian domain (though developed at much higher depths of 60–80 km, corresponding to eclogite-facies conditions of 550–620 °C at 2–2.5 GPa; e.g. Pelletier & Müntener, Reference Pelletier and Müntener2006; Debret et al. Reference Debret, Nicollet, Andreani, Schwartz and Godard2013).
The occurrence of pseudotachylyte veins is also reported for other Ligurian oceanic unit rocks such as those of the Alpine Corsica Schistes Lustrés (Austrheim & Andersen, Reference Austrheim and Andersen2004; Deseta et al. Reference Deseta, Andersen and Ashwal2014a, b; Magott et al. Reference Magott, Fabbri and Fournier2016, Reference Magott, Fabbri and Fournier2017), a sort of analogue of the metamorphic Ligurian oceanic units exposed in the southern Apennines – CPT realm. These pseudotachylyte veins occur within thrust sheets of partially metamorphosed mantle peridotite and lower crust metagabbro enclosed by serpentinite and blueschist- to eclogite facies schists (i.e. in a broadly similar context to that for the Lanzo Massif). This reinforces the idea, proposed here for the DIATU case, that recognition of medium- to high-depth pseudotachylyte veins is strongly dependent on the presence of favourable geological conditions allowing their preservation. Interestingly, the Schistes Lustrés and the Lanzo Massif pseudotachylyte veins are so far the only two reported cases for pseudotachylyte occurrences from exhumed subducted ophiolites in the circum-Mediterranean orogenic chains.
The genesis of the Schistes Lustrés pseudotachylyte veins has been widely investigated (Austrheim & Andersen, Reference Austrheim and Andersen2004; Andersen et al. Reference Andersen, Mair, Austrheim, Podladchikov and Vrijmoed2008, Reference Andersen, Austrheim, Deseta, Silkoset and Ashwal2014; Deseta et al. Reference Deseta, Andersen and Ashwal2014a, b) and ascribed to thermally activated processes (i.e. ‘thermal runaway’ or ‘thermally-induced shear instability’, controlled by rheology; e.g. Braeck & Podladchikov, Reference Braeck and Podladchikov2007; John et al. Reference John, Medvedev, Rüpke, Andersen, Podladchikov and Austrheim2009; Spray, Reference Spray2010; Prieto et al. Reference Prieto, Florez, Barrett, Beroza, Pedraza, Blanco and Poveda2013). A similar mechanism is unlikely for the DIATU pseudotachylyte veins, which formed at much shallower depths with respect to the ∼40–80 km range of the Alpine Corsica ones (based on the 1.4–2.6 GPa and 480–550 °C estimates for the metamorphic peak; Ravna et al. Reference Ravna, Andersen, Jolivet and De Capitani2010; Vitale Brovarone et al. Reference Vitale Brovarone, Beyssac, Malavieille, Molli, Beltrando and Compagnoni2013 and references therein). It therefore seems likely that, in the DIATU case, brittle-controlled processes such as dehydration embrittlement (related to mineral composition; e.g. Green, Reference Green1995; Jung et al. Reference Jung, Green and Dobrzhinetskaya2004; Green & Jung, Reference Green and Jung2005; Jung et al. Reference Jung, Katayama, Jiang, Hiraga and Karato2006; Okazaki & Hirth, Reference Okazaki and Hirth2016) were prevailing. This would also be consistent with their relatively low H2O content (likely not exceeding 3 wt %, since glaucophane is the only hydrous phase), in contrast to the water-rich nature of the Schistes Lustrés pseudotachylytes (H2O up to 15 wt %). In this regard, a parallel with the pseudotachylytes from the northern Ligurian domain rocks of the Lanzo Massif is no longer supported, since the genesis of the latter has been rather ascribed to the release of differential stresses accumulated in strong dry metastable rocks, likely as a consequence of the much higher depths of formation with respect to the shallower DIATU pseudotachylytes (Scambelluri et al. Reference Scambelluri, Pennacchioni, Gilio, Bestmann, Plümper and Nestola2017).
5. Concluding remarks
Relics of FRs in the HP/LT DIATU metabasalts cropping out in northern Calabria are hosted within pods mostly made of epidote+quartz crystals. At the mesoscale they appear as dark seams of millimetre- to centimetre-sized widths, locally characterized by internal foliation and displaying tabular, curvilinear and meander-like shapes.
Microstructural observations by optical and scanning electron microscopes show that the FRs form injection veins, marked by flow folds, festoon-like and amoeboid structures. Injection veins are made by fine-grained crystals of glaucophane and titanite enclosing survivor clasts of epidote and more rarely lawsonite. These FRs show a wide variety of textures: some of them are massive with survivor clast dispersed in the matrix; more frequently they appear as foliated, with a foliation marked by alternating layers of glaucophane and titanite, or levels of survivor clasts with different grain sizes. Such textural features have been interpreted as indicative of an origin from seismic slip processes, which more likely produced a melt phase (successively quenched and recrystallized), rather than a granular cataclastic flow. The investigated FR injections are thus retained to represent pseudotachylyte (rather than ultracataclasite) veins, generated by partial melting of the glaucophane-rich layers of the DIATU metabasalts.
The DIATU pseudotachylytes were subsequently boudinaged with growth of glaucophane fibres in the necks, or cut by small reverse faults. Also the pods were subsequently deformed by ductile strain, with the development of a mylonitic foliation within the quartz crystals. This suggests that the pseudotachylytes formed in HP/LT conditions at middle crustal depths during the Eocene burial of the DIATU within the subduction channel. Such a result is consistent with similar structures found in the continental CPT crystalline slivers in southern Calabria, related to the Eocene crustal thickening and developed at similar depths.
No pseudotachylyte relic was found in the DIATU metabasalt source rock, suggesting that preservation of such delicate FR was possible only where this was shielded by the stiffer quartz+epidote pods.
Our study confirms that the brittle processes associated with the formation of pseudotachylytes can be active also in a ductile environment, as indicated by the subsequent mylonite formation recorded by the DIATU quartz+epidote pods. Brittle mechanisms are triggered by specific physico-chemical conditions such as occurrence of fluids and pulsating high-strain rates, typical of subduction zones.
The DIATU pseudotachylytes witness also for high-magnitude earthquakes associated with the Eocenesubduction of this sector of the Ligurian Ocean.
Author ORCIDs
Vitale Stefano 0000-0003-1952-0463
Acknowledgements
The authors wish to thank Roberto de Gennaro for skilled assistance during SEM observations. The official revisions from two anonymous reviewers and the editorial handling from the Executive Editor Olivier Lacombe greatly contributed to improving the quality of the paper, increasing its consistency and readability.