1. Introduction
In the eastern part of the Aegean Sea, the island of Rhodes is renowned for its remarkable exposures of Pleistocene marine deposits containing rich fossil assemblages reflecting environments ranging from lagoonal to upper bathyal (e.g. Moissette & Spjeldnaes, Reference Moissette and Spjeldnaes1995; Hanken, Bromley & Miller, Reference Hanken, Bromley and Miller1996; Spjeldnaes & Moissette, Reference Spjeldnaes, Moissette, James and Clarke1997; Hansen, Reference Hansen1999; Thomsen, Rasmussen & Hastrup, Reference Thomsen, Rasmussen and Hastrup2001; Moissette, Spjeldnaes & Georgiades-Dikeoulia, Reference Moissette, Spjeldnaes, Georgiades-Dikeoulia, Wyse Jackson, Butler and Spencer Jones2002; Titschack & Freiwald, Reference Titschack, Freiwald, Freiwald and Roberts2005; Cornée et al. Reference Cornée, Moissette, Joannin, Suc, Quillévéré, Krijgsman, Hilgen, Koskeridou, Münch, Lécuyer and Desvignes2006a ; Steinthorsdottir, Lidgard & Hakansson, Reference Steinthorsdottir, Lidgard and Hakansson2006; Joannin et al. Reference Joannin, Cornée, Moissette, Suc, Koskeridou, Lécuyer, Buisine, Kouli and Ferry2007; Titschack et al. Reference Titschack, Nelson, Beck, Freiwald and Radtke2008, Reference Titschack, Joseph, Fietzke, Freiwald and Bromley2013).
The lower Pleistocene (Gelasian) deposits of the Kritika Formation correspond to the first marine incursion after the formation of the Aegean chain and the deposition of Miocene–Pliocene continental/lacustrine sediments (van Hinsbergen et al. Reference van Hinsbergen, Krijgsman, Langereis, Cornée, Duermeijer and Van Vugt2007). Relatively few studies have however been carried out on the Kritika, and have been limited to general geological or localized geochemical aspects (Broekman, Reference Broekman1973, Reference Broekman1974; Lécuyer et al. Reference Lécuyer, Daux, Moissette, Cornée, Quillévéré, Koskeridou, Fourel, Martineau and Reynard2012) or to some peculiar features such as communities associated with fossil seagrasses (Moissette et al. Reference Moissette, Koskeridou, Cornée, Guillocheau and Lécuyer2007; Moissette, Reference Moissette, Ernst, Schäfer and Scholz2012) or submerged beachrock beds (Moissette et al. Reference Moissette, Koskeridou, Cornée and André2013).
The Gelasian is a key geological period characterized by the onset of alternations of glacial and interglacial episodes. The effects of this fluctuating climate resulted in significant and repeated eustatic sea-level changes (e.g. Zazo, Reference Zazo1999; Di Geronimo et al. Reference Di Geronimo, Di Geronimo, La Perna, Rosso, Sanfilippo and Hart2000; Zazo et al. Reference Zazo, Goy, Dabrio, Bardají, Hillaire-Marcel, Ghaleb, González-Delgado and Soler2003; Capraro et al. Reference Capraro, Asioli, Backman, Bertoldi, Channell, Massari, Rio, Head and Gibbard2005; Joannin et al. Reference Joannin, Cornée, Moissette, Suc, Koskeridou, Lécuyer, Buisine, Kouli and Ferry2007; Massari, Capraro & Rio, Reference Massari, Capraro and Rio2007; Caron, Bernier & Mahieux, Reference Caron, Bernier and Mahieux2009; Sgarrella, di Donato & Sprovieri, Reference Sgarrella, Di Donato and Sprovieri2012). During the Gelasian, the eastern Mediterranean climate was predominantly arid with short wet events (Vaks et al. Reference Vaks, Woodhead, Bar-Matthews, Ayalon, Cliff, Zilberman, Matthews and Frumkin2013). Marine littoral sediments were consequently deposited under warm-temperate conditions (Titschack, Bromley & Freiwald, Reference Titschack, Bromley and Freiwald2005; Lécuyer et al. Reference Lécuyer, Daux, Moissette, Cornée, Quillévéré, Koskeridou, Fourel, Martineau and Reynard2012). In Rhodes, as in other parts of the Mediterranean, local tectonic movements also played a major role in controlling environmental conditions, especially in marginal basins emplaced in forearc settings (Pirazzoli et al. Reference Pirazzoli, Montaggioni, Saliege, Segonzac, Thommeret and Vergnaud-Grazzini1989; Hanken, Bromley & Miller, Reference Hanken, Bromley and Miller1996; Duermeijer et al. Reference Duermeijer, Nyst, Meijer, Langereis and Spakman2000; Kontogianni, Tsoulos & Stiros, Reference Kontogianni, Tsoulos and Stiros2002; ten Veen & Kleinspehn, Reference ten Veen and Kleinspehn2002; Cornée et al. Reference Cornée, Moissette, Joannin, Suc, Quillévéré, Krijgsman, Hilgen, Koskeridou, Münch, Lécuyer and Desvignes2006a ).
Although the general concepts of sequence stratigraphy have been developed principally from analysis of siliciclastic sediments (for an overview, see Catuneanu, Reference Catuneanu2006), biogenic components and associations have received much more attention in carbonate than in siliciclastic deposits. The early Pleistocene of Rhodes is characterized by prevailing siliciclastics, together with abundant and very diverse carbonate skeletal remains of organisms, and may therefore provide ideal new insights into the understanding of biogenic assemblages in siliciclastic facies. They also show certain characters that are distinctive and perhaps unique, justifying further, more detailed consideration.
The aims of this paper are therefore to describe the different bio- and lithofacies, grouped into facies associations, and the depositional system of early Pleistocene nearshore environments where sediments are predominantly siliciclastic. This will lead to a better characterization of warm-temperate facies associations in shallow-water settings and to a palaeoenvironmental reconstruction with particular emphasis on bathymetrical variations, sedimentary patterns, taphonomy and sea-bottom characteristics.
2. Geological setting
The mostly marine Pleistocene deposits of Rhodes are well exposed on the north to southeast coasts of the island (Fig. 1). They have been the subject of various sedimentological, stratigraphical and palaeontological studies (e.g. Meulenkamp, de Mulder & van de Weerd, Reference Meulenkamp, De Mulder and Van De Weerd1972; Hanken, Bromley & Miller, Reference Hanken, Bromley and Miller1996; Cornée et al. Reference Cornée, Moissette, Joannin, Suc, Quillévéré, Krijgsman, Hilgen, Koskeridou, Münch, Lécuyer and Desvignes2006a , Reference Cornée, Münch, Quillévéré, Moissette, Vasiliev, Krijgsman, Verati and Lécuyer b ; Titschack et al. Reference Titschack, Joseph, Fietzke, Freiwald and Bromley2013). However, the siliciclastic sediments of the Kritika Formation have received much less attention (Broekman, Reference Broekman1973, Reference Broekman1974; Hajjaji et al. Reference Hajjaji, Bodergat, Moissette, Prieur and Rio1998; Cornée et al. Reference Cornée, Moissette, Joannin, Suc, Quillévéré, Krijgsman, Hilgen, Koskeridou, Münch, Lécuyer and Desvignes2006a ; Moissette et al. Reference Moissette, Koskeridou, Cornée, Guillocheau and Lécuyer2007; Lécuyer et al. Reference Lécuyer, Daux, Moissette, Cornée, Quillévéré, Koskeridou, Fourel, Martineau and Reynard2012). Following the works of Meulenkamp, de Mulder & van de Weerd (Reference Meulenkamp, De Mulder and Van De Weerd1972), Hanken, Bromley & Miller (Reference Hanken, Bromley and Miller1996), Nelson et al. (Reference Nelson, Freiwald, Titschack and List2001) and Titschack et al. (Reference Titschack, Joseph, Fietzke, Freiwald and Bromley2013), the Kritika deposits are assigned to the Kritika Formation. With an estimated thickness of c. 180 m (Mutti, Orombelli & Pozzi, Reference Mutti, Orombelli and Pozzi1970) and the occurrence of various types of lithofacies, with relatively abundant, diverse and well-preserved fossils, they are of interest in terms of depositional environments in a muddy, deltaic depositional setting (Moissette et al. Reference Moissette, Koskeridou, Cornée, Guillocheau and Lécuyer2007). The Kritika Formation of the Trianda Synthem (Titschack et al. Reference Titschack, Joseph, Fietzke, Freiwald and Bromley2013) is characterized by the alternation of clayey (more or less silty or sandy) and sandy beds (Figs 2–5), sometimes intercalated with conglomerates (Fig. 6a–c).

Figure 1. (a) Location map of the island of Rhodes in the eastern Mediterranean and schematical outcrop map of the Neogene–Quaternary deposits of Rhodes with location of the studied sections. Modified from Meulenkamp, de Mulder & van de Weerd (Reference Meulenkamp, De Mulder and Van De Weerd1972) and Hanken, Bromley & Miller (Reference Hanken, Bromley and Miller1996). Red line: approximate location of the continental–marine transition within the Kritika Formation (open sea to the east); red arrow: main current direction. (b) Detailed (Google) map of the northern part of Rhodes indicating the location of the four studied sections.

Figure 2. Sedimentary log, relative abundance of skeletal components and facies associations in the Kritika-N section (36° 25′ 49.49″ N, 8° 12′ 11.01″ E). * – nannoplankton sample with Calcidiscus macintyrei and absence of large Gephyrocapsa.

Figure 3. Sedimentary log, relative abundance of skeletal components and facies associations in the Kritika-S section (36° 25′ 31.83″ N, 28° 12′ 12.79″ E).

Figure 4. Sedimentary log, relative abundance of skeletal components and facies associations in the Faliraki-N section (36° 21′ 29.10″ N, 28° 12′ 35.65″ E).

Figure 5. Sedimentary log, relative abundance of skeletal components and facies associations in the Faliraki-S section (36° 21′ 02.04″ N, 28° 12′ 21.45″ E).

Figure 6. Outcrop views of the main sedimentary features recognized in the Kritika Formation. (a) Alternation of sandy (1), gravelly (2) and marly (3) layers (Kritika-S section). (b) Conglomeratic sandstone bed showing cross-stratification (Faliraki-N section). (c) Sandstone bed (2) with cross-stratification on top of a marly level (1) (Kritika-N section). (d) Block of conglomeratic beachrock (2) covered by a thick, poorly indurated, carbonate crust (3), between two marly layers (1) (Faliraki-N section). (e) Thin carbonate crust (3) developed on top of a dismembered beachrock bed (2) overlaying a sandy level (1) (Faliraki-S section).
The scarcity of biostratigraphic markers within the shallow-water siliciclastic deposits of the Kritika Formation makes it difficult to estimate its precise age. It was first referred to the late Pliocene on the basis of ostracodes (Sissingh, Reference Sissingh1972) and sporomorphs (Benda, Meulenkamp & van de Weerd, Reference Benda, Meulenkamp and van de Weerd1977). Using mostly calcareous nannofossils, but also planktonic foraminifers and ostracodes, the age of the Kritika deposits was estimated by biostratigraphic correlation with the Pliocene–Pleistocene boundary section occurring at Vrica, southern Italy (Thomsen, Rasmussen & Hastrup, Reference Thomsen, Rasmussen and Hastrup2001). The radiometric dating of a volcaniclastic layer contained in the overlying Lindos Bay Clay Formation yielded an age of 1.89 ± 0.09 Ma, which is consistent with planktonic foraminiferal and magnetostratigraphic data and indicates the base of the Pleistocene or Gelasian and the Olduvai subchron (Cornée et al. Reference Cornée, Münch, Quillévéré, Moissette, Vasiliev, Krijgsman, Verati and Lécuyer2006b ).
3. Material and methods
Four detailed sections (Fig. 1) of the sedimentary succession were measured and sampled: two close to the type locality of Kritika (Kritika-N and Kritika-S) and two near Faliraki (Faliraki-N and Faliraki-S). A total number of 147 samples were collected from the outcrops for palaeontological analysis (Figs 2–5).
Depending on the type of material, samples of c. 500 g (loose sediment, clay or sand) or several kilograms (sandstones, rhodoliths, shells) were collected from the studied sections. The samples were later treated in the laboratory by first soaking them in diluted hydrogen peroxide for 24 hours to facilitate disaggregation, and then wet sieved through strainers with five different mesh sizes (2, 1, 0.5, 0.25 and 0.125 mm). When dried, the residues were sorted into different floral and faunal groups; a semi-quantitative estimation of their respective amounts was then made and converted into a graphical representation displayed next to the lithological columns (Figs 2–5).
An average of 150 foraminifer specimens were randomly picked from each fraction >0.125 mm. In the case of very low density of specimens (usually in sandy sediments), the entire dried residue was microscopically examined. For the molluscs and the bryozoans, all specimens from the 2–1 mm fractions were extracted, identified and counted using a binocular microscope. For grain sizes 0.5 and 0.25 mm, and when the residues were abundant, only a small percentage quantity was analysed. The residues of fraction 0.125 mm contained no identifiable molluscs and only an insignificant number of bryozoan fragments which were merely scanned for the presence/absence of some minute species.
Benthic foraminifers, molluscs and bryozoans dwell in a wide range of marine (and even fresh- or brackish-water) bottom settings and are therefore valuable palaeoenvironmental indicators. Ecological information, especially on depth, hydrodynamism, salinity and substrates, was extracted from the abundant available literature dealing with benthic foraminifers (e.g. Jorissen, Reference Jorissen1988, Reference Jorissen and Sen Gupta1999; Albani & Serandrei Barbero, Reference Albani and Serandrei Barbero1990; Cimerman & Langer, Reference Cimerman and Langer1991; Jorissen, Fontanier & Thomas, Reference Jorissen, Fontanier, Thomas, Hillaire-Marcel and de Vernal2007; Sgarrella, di Donato & Sprovieri, Reference Sgarrella, Di Donato and Sprovieri2012), molluscs (e.g. Pérès & Picard, Reference Pérès and Picard1964; Pérès, Reference Pérès1967; Riedl, Reference Riedl1983; Carthew & Bosence, Reference Carthew and Bosence1986; Barash & Danin, Reference Barash and Danin1988, Reference Barash and Danin1992; Koukouras & Russo, Reference Koukouras and Russo1991; Morton, Britton & Frias Martins, Reference Morton, Britton and Frias Martins1998; Zenetos, Vardala-Theodorou & Alexandrakis, Reference Zenetos, Vardala-Theodorou and Alexandrakis2005) and bryozoans (e.g. Gautier, Reference Gautier1962; Harmelin, Reference Harmelin1976; Zabala, Reference Zabala1986; Rosso, Reference Rosso1996a , Reference Rosso b ; Novosel, Požar-Domac & Pasarić, Reference Novosel, Požar-Domac and Pasarić2004; Rosso et al. Reference Rosso, Di Martino, Sanfilippo, Di Martino, Ernst, Schäfer and Scholz2012).
The Mediterranean bionomical classification/zonation established by Pérès & Picard (Reference Pérès and Picard1964) and revised by Bellan-Santini et al. (Reference Bellan-Santini, Bellan, Bitar, Harmelin and Pergent2002) has been used to define most of the communities encountered in the deposits of the Kritika Formation. The sedimentological interpretations are based on studies by Wright and Burchette (Reference Wright, Burchette and Reading1996), Selley (Reference Selley2000), and Merzeraud (Reference Merzeraud2009). As a result of repetitive bio- and lithofacies successions at the metre-scale in the field, a number of genetic sequences (Homewood et al. Reference Homewood, Guillocheau, Eschard and Cross1992; Loucks & Sarg, Reference Loucks and Sarg1993; Cross & Lessenger, Reference Cross, Lessenger, Gradstein, Sandvik and Milton1998; for an overview, see Merzeraud, Reference Merzeraud2009) have been recognized. Nevertheless, it is sometimes difficult to precisely locate base-level turnarounds from sedimentological criteria only, especially in homogenous facies such as the siliciclastic sediments of the Kritika Formation. This is the reason why we used both sedimentological features and biological content to more accurately define the genetic sequences. Fall-to-rise or rise-to-fall turnarounds can be deduced by using sedimentological criteria (e.g. erosional surfaces, facies changes, firmgrounds), but also from variations in fossil assemblages. For instance, some of these turnarounds occur in homogeneous silty-clayey deposits, but the marine or brackish-water origin of many of them was ascertained only by means of palaeontological criteria. Correlations between neighbouring sections (hundreds of metres) are based on the occurrence of a few index beds complemented by sequence stratigraphic interpretations. Nevertheless, long-distance correlations (kilometres) remain difficult in the Kritika Formation because of a lack of both precise biostratigraphic indices and complete sections in field exposures.
4. Results
4.a. Stratigraphy
From the eight samples studied for calcareous nannoplankton record only one, from the uppermost marly level of the Kritika-N section (Fig. 2), yielded high coccolith abundance and good preservation. The occurrence of Calcidiscus macintyrei and the absence of large Gephyrocapsa in this sample suggests an age older than 1.66 Ma (Zone NN19/NN18; Raffi et al. Reference Raffi, Backman, Fornaciari, Pälike, Rio, Lourens and Hilgen2006; G.-E. López-Otálvaro, pers. comm. 2012). Taking into account this new information, together with field observations and the isotopic, magnetostratigraphic and biostratigraphic dating of the overlying Lindos Bay clay Formation (Cornée et al. Reference Cornée, Münch, Quillévéré, Moissette, Vasiliev, Krijgsman, Verati and Lécuyer2006b ), the uppermost part of the Kritika Formation is now confidently assigned a Gelasian age (2.58–1.80 Ma; Gibbard et al. Reference Gibbard, Head and Walker2009). Nevertheless, the precise ages of the studied sections remain unknown, especially for its lowermost part.
4.b. Biodiversity
The most abundant organisms (Figs 2–5) are the coralline red algae (mostly rhodoliths, but also some crustose and geniculate forms), the benthic foraminifers, the molluscs (mainly bivalves and gastropods, but some scaphopods and polyplacophores as well) and the bryozoans. Although less abundant (and diverse), numerous other groups of organisms are represented: green algae (charophyceans, dasycladaceans, Halimeda); planktonic foraminifers; sponges (calcareous and siliceous spicules); cnidarians (solitary and colonial scleractinians, alcyonarian spicules); annelids (serpulids mostly, but also some tube-worms); brachiopods; crustaceans (decapods, ostracodes and a few barnacles); echinoderms (regular and irregular echinoids, ophiuroids); and fishes (rare to very rare teeth and bone fragments, a few otoliths). Trace fossils also occur, either in the form of bioturbations in soft-bottoms or borings in hard substrates.
The majority of the collected fossils lived in marine environments. However, some are typically brackish and even terrestrial or freshwater organisms.
The fossil communities which are the object of this study are represented by about 80 species of benthic foraminifers, 330 species of molluscs (60 bivalves, 260 gastropods, 8 polyplacophores and 2 scaphopods) and 190 species of bryozoans. These groups will eventually be studied by the authors in more detailed publications.
4.c. Facies associations
Based on the type of sediments, but mostly their organic content, 14 facies (lithologically and faunistically distinct units, labelled 1–14) grouped into four facies associations have been recognized in the studied sections (Figs 2–5).
In some parts of the sections, two facies may be barely discernible from one another because of rapid ecological successions. Early successional forms (shallow-water facies association) may therefore be preserved underneath the skeletons of late successional species (deeper-water facies association) or vice versa. This is especially the case for the beachrock crusts and some of their surrounding levels.
4.c.1. Facies association 1 (Facies 1–3)
The deposits of this facies association are almost or completely fossil free. Very few worn-out gastropod and ostracode fragments may sporadically occur. Three different facies have been identified.
-
1. Facies 1. Associated with the conglomeratic beds. It is completely barren.
-
2. Facies 2. Its sandy sediments are also fossil free.
-
3. Facies 3. It corresponds to clayey and almost fossil-free levels (e.g. base of the Faliraki-N section).
4.c.2. Facies association 2 (Facies 4–6)
This association is characterized by the occurrence of only a few groups of organisms, none of them very abundant. The most common are the benthic foraminifers, the bivalves, the ostracodes and, less frequently, the gastropods. Other groups are occasionally represented by a few fragments: annelids, bryozoans, echinoids, decapods, barnacles and fishes.
Freshwater elements are always rare: charophyte oogonia and small gastropods (Melanopsis, Planorbis, Theodoxus, Valvata). Very few terrestrial hygromiid gastropod shells have even been found in two samples of the Faliraki-S section. Brackish-water organisms are generally better represented, among them benthic foraminifers (with high occurrences in some samples of species such as Ammonia tepida and Aubignyna perlucida), gastropods (e.g. Cyclope neritea, Potamides, Ventrosia ventrosa), bivalves (e.g. Loripes lacteus, Cerastoderma edule, Abra alba) and ostracodes (e.g. Candona, Cyprideis, Aurila). Remains of normal marine organisms may occasionally occur in this facies association: dasycladacean and coralline algae, seagrass leaves, planktonic foraminifers, fragments of erect bryozoan colonies and echinoid spines. On the basis of lithological and palaeontological criteria, three facies have been recognized within this facies association.
Facies 4. Argillaceous, more or less silty or sandy deposits with rare freshwater elements (charophyte oogonia and gastropods), common brackish-water faunas (predominantly benthic foraminifers and ostracodes) and even very rare terrestrial gastropods. Extremely rare normal marine organisms, notably planktonic foraminifers, occur mostly in the Kritika-N and Faliraki N sections.
Facies 5. Occasional sands with very rare freshwater elements (charophyte oogonia) and common brackish-water faunas (benthic foraminifers, bivalves and ostracodes). Typical ‘lagoonal’ species such as Aubignyna perlucida dominate the benthic foraminifer fauna.
Facies 6. Dark lignitic clays. They occur only in the Faliraki-N section and contain mostly brackish-water but also freshwater bivalves and gastropods (Planorbis planorbis, Planorbarius corneus).
4.c.3. Facies association 3 (Facies 7–10)
This association occurs in argillaceous and sandy deposits (Fig. 6a–c), predominantly where hard substrates are abundant: beachrock slabs (Fig. 6d, e) and bivalve shells (Fig. 7e). It is moderately rich. A higher number of groups (and species) of skeletal organisms occur than in Facies association 2, each generally represented by more fragments.

Figure 7. Outcrop photographs showing some of the main biosedimentary features of the Kritika Formation. (a) Rhizomes of the seagrass Posidonia oceanica within a coarse siliciclastic bed (Kritika-S section). (b) Large colony of the hermatypic coral Cladocora caespitosa positioned on top of a beachrock slab (Faliraki-S section; hammer blade for scale). (c) Detailed view of a layer with abundant coralline algal rhodoliths, bivalve shells and small gravels (Faliraki-S section; hammer blade for scale). (d) Detailed view of the upper surface of a poorly indurated organogenic crust on top of a beachrock bed. A gastropod shell (Turritella vermicularis), branching bilaminate bryozoans (br), bivalves (b) and gravels are observable (Kritika-N section). (e) Sandy bed with numerous Glycymeris bivalve shells (Faliraki-N section; hammer for scale). (f) Marly firmground with vertical to subvertical burrows infilled by sand from the overlaying bed (Kritika-S section; lens cap for scale).
Only marine organisms are represented in this association. Coralline algae, benthic foraminifers, annelids (serpulids and Ditrupa tube-worms), gastropods, bivalves, bryozoans, decapods and ostracodes are much more abundant than in Facies association 2. A few planktonic foraminifers, solitary corals, scaphopods, alcyonarian spicules and polyplacophores also occur. Apart from numerous eurybathic organisms, some species indicate infralittoral environments. Among the most characteristic are some benthic foraminifers (miliolids, Ammonia beccarii, Elphidium crispum, E. macellum, Haynesina depressula, Porosononion subgranosum), bivalves (Lentidium mediterraneum, Lucinella divaricata, Gouldia minima, Ervilia castanea, Spisula subtruncata), gastropods (Bittium spp., Cerithium spp.) and bryozoans (Calpensia nobilis, Cryptosula pallasiana, Hagyosinodos latus, Chlidonia pyriformis, Savignyella lafontii). Some of the less well-preserved benthic foraminifers (miliolids, elphidiids and ammoniids) are brownish stained and to some degree abraded and polished. Four facies have been identified within this facies association.
Facies 7. Pure argillaceous deposits (Fig. 6a) are relatively rare in this facies association. They are characterized by benthic foraminifers, bivalves (with localized shell accumulations), gastropods, bryozoans, ostracodes, echinoids, rarer planktonic foraminifers, solitary corals, scaphopods and annelids. Among the bivalves, the more characteristic species are Loripes lacteus, Divaricella divaricata, Nucula nucleus and Tellina distorta. Benthic foraminifers are represented by high abundance of Miliolidae, together with Ammonia beccarii and Porosononion subgranosum.
Facies 8. Sandy, more or less clayey, deposits (Fig. 6a–c) are widespread. They contain abundant calcareous algae, benthic foraminifers, annelids, gastropods, bivalves (occasionally forming shell beds) and bryozoans. Scaphopods, decapods, ostracodes and echinoids are much less common.
Two main shallow-water mollusc associations have been recognized in this facies. The first contains the following characteristic species: Cerastoderma edule, Donax trunculus, Mytilus sp. and Lentidium mediterraneum (bivalves) and Cerithium vulgatum, Conus ventricosus and Neverita josephinia (gastropods). A second association, comprising only bivalves (occasionally accompanied by the tube-worm Ditrupa arietina), yielded Spisula subtruncata, Glycymeris violascens, Anomia ephippium, Parvicardium papillosum, Donax venustus, Tellina pulchella and Dosinia lupinus.
Facies 9. Characterized by remains of the marine phanerogam Posidonia oceanica preserved in fine-grained sands and silty/sandy clays (Figs 6a, 7a). In both Kritika sections they are mostly associated with crustose calcareous algae (thin films on seagrass leaves), benthic foraminifers, molluscs, bryozoans and ostracodes (Moissette et al. Reference Moissette, Koskeridou, Cornée, Guillocheau and Lécuyer2007). Benthic foraminifers are notably represented by Ammonia beccarii, Asterigerinata planorbis, Elphidiidae and Miliolidae.
Facies 10. With its abundant and diverse fossil communities, it is associated with the sandstones of the beachrock beds (Moissette et al. Reference Moissette, Koskeridou, Cornée and André2013; Fig. 6d, e). The first generation of carbonate crusts that developed directly on top of the drowned beachrock slabs is typical of a rocky bottom environment: crustose coralline algae; benthic foraminifers (elphidiids, miliolids, Ammonia beccarii); serpulid worms; bivalves (Chama, Spondylus, Ostrea, Chlamys, Barbatia, Lima, Anomia); gastropods (Cerithiidae, Haliotis, Xenophora, Vermetus, Gibbulla, Tricolia) and encrusting bryozoans (Calpensia nobilis, Cryptosula pallasiana, Hagyosinodos latus).
4.c.4. Facies association 4 (Facies 11–14)
This association is the richest; it is often found within deposits where hard substrates are abundant: calcareous crusts on beachrock slabs, sandstones, rhodoliths and bivalve shells. Many groups of skeletal organisms are represented. The most common, diverse and always present include benthic foraminifers, bivalves, gastropods and bryozoans. They are generally accompanied by coralline red algae (especially rhodoliths, but also abundant crustose forms and sparse geniculate segments and branching forms found in some of the loose sediment beds), cnidarians (solitary and colonial), annelids, ostracodes and echinoids (regular and irregular) and sporadically include brachiopods, scaphopods, pteropods and planktonic foraminifers.
A number of deeper-water species occur among the main components of this facies association. The benthic foraminifers Gavelinopsis praegeri, Asterigerinata planorbis and Neoconorbina terquemi, the bivalves Diplodonta rotundata, Pseudoamussium clavatum and Corbula gibba, the gastropod Obtusella macilenta and the bryozoans Tervia irregularis, Tessaradoma boreale and Hippomenella mucronelliformis are probably the most common and characteristic. Four main facies have been identified within this facies association.
Facies 11. It corresponds to sandy, more or less clayey, and sometimes gravelly or pebbly (Fig. 8c) deposits and contains abundant and diverse invertebrate and benthic foraminifer communities, often accompanied by rarer coralline algae. The molluscs (especially the bivalves) and the bryozoans are always the most obvious and distinctive elements of the fauna.
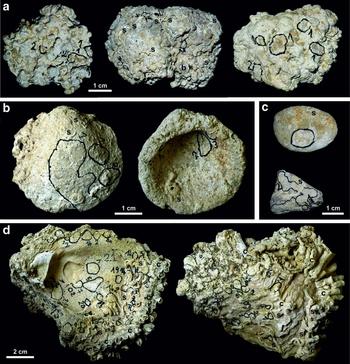
Figure 8. Examples of ‘benthic islands’ from the soft-bottom environments of the Kritika Formation. (a) Coralline algal rhodoliths colonized by bryozoan colonies (circled and numbered with black ink), serpulid worms (s) and a small cemented oyster valve (b) (Kritika-N section). (b) Glycymeris bivalve shells intensely encrusted by calcareous algae (whitish background), bryozoan colonies (circled and numbered with black ink), serpulid worms (s) and occasionally bored (small circular holes) by clionid sponges (Faliraki-N section). (c) Small pebbles used as substrates by encrusting calcareous algae (whitish background), bryozoan colonies (circled and numbered with black ink) and serpulid worms (s) (Kritika-N section). (d) Internal (left) and external (right) surface of a large oyster shell forming attachment base for numerous epibionts: Cladocora caespitosa coral colony (c), serpulid worms (s) and bryozoan colonies (circled and numbered with black ink) (Faliraki-N section). Each number near a black circle corresponds to a different species of bryozoan.
One of the soft-bottom mollusc associations of this facies is particularly common, comprising the bivalves Timoclea ovata, Parvicardium minimum, Plagiocardium papillosum and Hiatella arctica and the gastropods Emarginula fissura, Petalochoncus intortus, Turboella incospicua, Rissoa ventricosa and Hinia serraticosta. It also includes rhodoliths, serpulids and erect bryozoans.
Small branching coralline algae (maërl) also occur in one sandy level of the Kritika-S section where they are accompanied by numerous benthic foraminifers, bivalves (such as Tellina donacina and Chlamys flexuosa) and bryozoans.
The foraminiferal fauna is mainly dominated by Miliolidae. The remaining fauna consists mainly of plano-convex species and Elphidium spp. (among which E. crispum is the dominant species).
Facies 12. Algal nodules (Fig. 7c) are concentrated in several soft-sediment (clayey or sandy) levels (Figs 2–5). The rhodoliths (ranging from a few centimetres to 10 cm across) are commonly encrusted by invertebrates such as bivalves, serpulid worms and bryozoans (Fig. 8a). Numerous other organisms are found in the enclosing sediment: common benthic foraminifers (Cibicidoides lobatulus, Asterigerinata planorbis, Miliolidae), bivalves (especially Venus casina and Tellina donacina), gastropods, tube worms and erect and free-living bryozoans, together with rarer brachiopods, decapod crustaceans and echinoids.
Facies 13. It is characterized by accumulations of abundant large bivalve shells (mostly oysters) occurring within silty, clayey and sandy deposits. These shells are generally encrusted by numerous bryozoans and other encrusters such as calcareous red algae, serpulid worms and smaller bivalves (Fig. 8d). The enclosing sediment contains mostly benthic foraminifers (several plano-convex species such as A. planorbis and Neoconorbina terquemi, and miliolids), gastropods, bryozoans (erect and free-living), ostracodes, decapods and echinoids (spines).
Facies 14. It corresponds to the second generation of calcareous crusts that developed after the first generation (Facies 10) on top of the drowned beachrock slabs (Figs 6d, e, 7b, d). It is characterized by encrusting (coralline algae, molluscs and serpulids) and erect organisms (Cladocora caespitosa, a colonial scleractinian coral, and bryozoans). Small build-ups locally developed topographic relief upon the hard-bottom beds in the Faliraki-N section (Moissette et al. Reference Moissette, Koskeridou, Cornée and André2013). The skeletal community of the knoll-shaped build-ups is dominated by calcareous red algae growing in close connection with serpulid worms and other encrusting organisms such as bryozoans, bivalves and vermetid gastropods.
4.d. Description of the sections
4.d.1. Kritika-N section
The lowest part of this section is exposed about 2 km south of Rhodes Town near the western coastal road leading to the village of Kritika (and then to Ialisos and the airport; Fig. 1). Its total thickness is c. 100 m (the conglomerates at the base of the exposure are 8 m thick, but only the uppermost part is shown in Fig. 2). This section displays the widest variety of facies and the highest diversity of skeletal components. Shell levels and rhodolith-rich horizons are conspicuous features, together with the carbonate crusts overlying two beachrock beds (Fig. 7d). Based on facies associations, 13 genetic sequences, each bounded by maximum flooding surfaces, have been recognized. Sediments were repeatedly deposited in continental to marine, deeper-water settings.
4.d.2. Kritika-S section
This exposure extends along the road starting 1.5 km south of the base of the previous section and reaches the top of the hill, near the southern suburbs of Rhodes Town (Fig. 1); it is c. 80 m thick (Fig. 3). Compared to the previous section, the variety of facies is also high but the organic diversity is slightly lower. Mollusc shells and rhodoliths are abundant, but only one beachrock bed (with its associated carbonate crust) was observed. Several firmground levels (Fig. 7f) are recognizable in the lower half of the section. In the upper part, two beds display soft deformation with ball and pillow structures. Ten genetic sequences have been recognized. Sediments were also repeatedly deposited in continental to marine, deeper-water settings.
4.d.3. Faliraki-N section
This section was measured and sampled on the right side of the eastern coastal road leading from Rhodes Town and Kallithea to the village of Faliraki (Fig. 1). With a thickness of about 35 m, this section is the thinnest of the four (Fig. 4). It consists mostly of continental, fluviatile and brackish-water deposits (including a thin lignite horizon) with a few marine levels adjacent to a beachrock bed (Fig. 6e). The diversity of skeletal components is much lower than in the previous two sections. Three genetic sequences have been recognized. Sediments were dominantly deposited in continental to brackish-water environments.
4.d.4. Faliraki-S section
The lowest part of this section is exposed about 2 km north of Faliraki (and 1 km south of Faliraki-N; Fig. 1) on the right side of the eastern coastal road (Fig. 6d).This exposure is almost 50 m thick (Fig. 5). Despite the occurrence of two beachrock beds (with their associated carbonate crusts), the observed organic diversity is the lowest of the four studied sections. A few rhizoliths are observed near the top of the section, and three genetic sequences have been recognized. Sediments were also dominantly deposited in continental to brackish-water environments.
5. Discussion
Apart from a few fossil-free levels, most of the sampled beds contain more or less rich skeletal assemblages (Figs 2–5). The conglomeratic levels are devoid of organic remains, which is also the case for most pure sandy beds. The majority of clay beds contain relatively poor communities. On the contrary, rich assemblages of organisms occur in sandy or clayey deposits with mollusc shells and/or red algal rhodoliths (Fig. 7c–e).
A number of sandstone beds, dismembered into polygonal blocks, also occur in all four sections of the Kritika Formation (Fig. 6d, e). They have been interpreted as submerged former beachrock beds (Moissette et al. Reference Moissette, Koskeridou, Cornée and André2013). The carbonate crusts that developed directly on top of the beachrock slabs yielded abundant algal and invertebrate communities (Moissette et al. Reference Moissette, Koskeridou, Cornée and André2013; Fig. 7b, d).
Seagrass meadows are recorded in both sections of the Kritika locality by the occurrence of beautifully preserved leaves and rhizomes (Fig. 7a) of the Mediterranean endemic marine phanerogam Posidonia oceanica (Moissette et al. Reference Moissette, Koskeridou, Cornée, Guillocheau and Lécuyer2007). Relatively rich fossil communities are associated with these remains.
5.a. Palaeoenvironmental interpretation of the facies associations
A synthesis of the results obtained by the study of benthic foraminifers, molluscs and bryozoans, together with the main sediment characteristics, allows the palaeoenvironments of the lower Pleistocene Kritika Formation to be reconstructed. These nearshore siliciclastic sediments were emplaced predominantly in marine embayments adjacent to a rising coast with fluvial/deltaic influence. In these environments, changes in benthic communities occurred along a depth gradient from the coastal area to c. 50–60 m.
5.a.1. Facies association 1
These typically fossil-free deposits (Facies 1–3) may contain very few, worn-out, probably reworked or transported fragments of gastropods and ostracodes. The predominance of conglomerates and sands indicate continental, partly fluviatile environments.
5.a.2. Facies association 2
Brackish-water settings (typically lagoonal) are suggested by rare remains of gastropods and more abundant benthic foraminifers, bivalves and ostracodes. These euryhaline organisms are mostly associated with clayey, more or less silty or sandy deposits. The presence of freshwater settings (most probably lacustrine) are indicated by the occurrence of a few green algal charophyte oogonia and by a number of gastropod taxa (i.e. Planorbis and Theodoxus). These elements were transported, especially during flood season, from nearby lakes or rivers and deposited into brackish or marine environments. The rare to very rare marine fossils also occurring in this facies association were either brought by marine currents (seagrass leaves, planktonic foraminifers, small bryozoan segments) or reworked (echinoid spines) from older deposits.
Facies 4 (argillaceous, more or less silty or sandy deposits) and less distinctively Facies 5 (more sandy sediments) are typical of brackish-water environments where the abundance of benthic foraminifers such as Ammonia tepida (>90 % in some samples) or Aubignyna perlucida is characteristic of estuarine to lagoonal settings. A. tepida is a typical lagoonal species commonly occurring in muddy sands (e.g. Jorissen, Reference Jorissen1988) and A. perlucida is found in water depths of 10–25 m on the Black Sea shelf, where salinities vary over the range 11–16 ‰ (Yanko, Reference Yanko1990). Gastropods such as Cyclope neritea, Potamides, Pirenella conica and Ventrosia ventrosa or bivalves such as Loripes lacteus, Cerastoderma edule and Abra alba are typical of brackish-water mollusc communities (Bellan-Santini et al. Reference Bellan-Santini, Bellan, Bitar, Harmelin and Pergent2002) that are equivalent to the ‘biocénose lagunaire euryhaline et eurytherme’ (LEE, euryhaline and eurythermal biocoenosis) described by Pérès & Picard (Reference Pérès and Picard1964) from the present-day Mediterranean (Michez et al. Reference Michez, Dirberg, Bellan-Santini, Verlaque, Bellan, Pergent, Pergent-Martini, Labrune, Francour and Sartoretto2011). The occurrence of some dark lignitic levels (Facies 6) may also indicate accumulation of organic matter in coastal lagoon sediments, resulting in episodes of hypoxia and even anoxia.
5.a.3. Facies association 3
Fossiliferous argillaceous deposits and sandy beds, often coarse-grained and poor in organic matter, contain abundant and fragmented remains of molluscs and bryozoans (Crisia, Chlidonia, Savignyella). They are usually accompanied by benthic foraminifers (Ammonia beccarii, elphidiids, miliolids) and a few echinoids, coralline algae and crustaceans (decapods and ostracodes). These organisms, together with their higher diversity and better preservation state, are characteristic of shallow-water, generally high-energy, fully marine infralittoral settings, especially in sandy to muddy soft bottoms adjacent to shorelines. This facies association notably displays affinities with various biocoenoses of Pérès & Picard (Reference Pérès and Picard1964).
Facies 7 is dominated by terrigenous sediments with poorer benthic communities (mostly foraminifers, molluscs, bryozoans, ostracodes and echinoids) than in the more sandy settings of Facies 8. A few bivalves such as Loripes lacteus, Lucinella divaricata, Nucula nucleus and Tellina distorta indicate that the sedimentation occurred in a nearshore and stressed sedimentary environment (Pérès & Picard, Reference Pérès and Picard1964; Simboura & Zenetos, Reference Simboura and Zenetos2002; Dimitriadis & Koutsoubas, Reference Dimitriadis and Koutsoubas2008). This reduced diversity is characteristic of soft substrates in settings of increased turbidity (and probably also dissolved organic matter). These features most likely indicate that this shallow-water area was under the influence of a muddy river delta. The benthic foraminiferal fauna is represented by a high abundance of the epiphyte Miliolidae, likely reflecting high-energy environments that inhibit the growth and accumulation of small, fragile tests.
Several biocoenoses of Pérès & Picard (Reference Pérès and Picard1964) have been recognized in Facies 8, notably the biocoenosis termed ‘sables fins bien calibrés’ (SFBC, well-sorted fine sands) which is characteristic of sandy bottoms in the shoreface (at depths of 5–20 m). It is dominated by shallow infaunal bivalve suspension feeders (Spisula subtruncata, Glycymeris violascens, Parvicardium papillosum, Donax venustus, Tellina pulchella and Dosinia lupinus) and is often accompanied by the tube-worm Ditrupa arietina (Dominici, Reference Dominici2001; Urra et al. Reference Urra, Gofas, Rueda and Marina2011). The other mollusc association (with Cerastoderma edule, Donax trunculus, Lentidium mediterraneum, Cerithium vulgatum and Neverita josephinia) indicates depths of c. 0–10 m and may correspond to the very shallow biocoenosis of Pérès & Picard (Reference Pérès and Picard1964) named ‘sables fins superficiels’ (SFS, fine superficial sands). The biocoenosis termed ‘sables grossiers et fins graviers brassés par les vagues’ (SGBV, coarse sands and fine gravels mixed by the waves), characteristic of sandy bottoms in the shoreface (2–10 m), is also represented in this facies. It is dominated by shallow infaunal suspension feeders with bivalves such as Ervilia castanea, Donacila cornea and Dosinia lupinus (Koukouras & Russo, Reference Koukouras and Russo1991; Morton, Britton & Frias Martins, Reference Morton, Britton and Frias Martins1998; Koskeridou, Thivaiou & Giamali, Reference Koskeridou, Thivaiou and Giamali2013). All the dominant benthic foraminiferal taxa are infralittoral species common in shallow marine environments that denote normal salinity conditions. In particular, Quinqueloculina spp. and A. beccarii are considered by Jorissen (Reference Jorissen1988) as inhabitants of a nearshore zone (7.5–25 m water depth) with coarse substrates and which is poor in organic matter. Furthermore, these taxa show a significant negative correlation with the percentage of organic matter.
Facies 9 is interpreted as corresponding to the biocoenosis ‘herbier à Posidonia oceanica’ (HP, Posidonia oceanica meadows; Pérès & Picard, Reference Pérès and Picard1964), which is represented by the well-preserved remains of this seagrass species found in some levels of both Kritika sections (Moissette et al. Reference Moissette, Koskeridou, Cornée, Guillocheau and Lécuyer2007). Although no direct evidence of seagrass meadows was found in the Faliraki sections and in other levels of the Kritika sections, their former presence can be deduced by the co-occurrence, especially within poorly sorted terrigenous sediments, of characteristic fossil components such as geniculate coralline red algae, discoidal benthic foraminifers, infaunal and epibyssate bivalves, small gastropods, encrusting and articulated bryozoans and ostracodes (Hajjaji et al. Reference Hajjaji, Bodergat, Moissette, Prieur and Rio1998; James & Bone, Reference James and Bone2007; Moissette et al. Reference Moissette, Koskeridou, Cornée, Guillocheau and Lécuyer2007; Reuter et al. Reference Reuter, Piller, Harzhauser, Kroh, Rögl and Ćorić2011; Moissette, Reference Moissette, Ernst, Schäfer and Scholz2012; O’Connell, James & Bone, Reference O’Connell, James and Bone2012; Reich et al. Reference Reich, Di Martino, Todd, Wesselingh and Renema2015). In these infralittoral settings (at depths of 0–20 m), baffling and trapping by seagrass leaves and rhizomes took place together with a limited carbonate production (Pérès & Picard, Reference Pérès and Picard1964; Canals & Ballesteros, Reference Canals and Ballesteros1997; Perry & Beavington-Penney, Reference Perry and Beavington-Penney2005; James et al. Reference James, Bone, Brown, Cheshire, Swart, Eberli and McKenzie2009). The Posidonia oceanica meadows are generally an extension at greater depths of the biocoenoses of ‘fine superficial sands’ and ‘muddy sands in sheltered waters’ (Bellan-Santini et al. Reference Bellan-Santini, Bellan, Bitar, Harmelin and Pergent2002).
Facies 10 represents the first generation of carbonate crusts (with crustose coralline algae, serpulid worms, encrusting bivalves and bryozoans) that developed directly on top of the drowned beachrock slabs (Fig. 6d, e) and is typical of a nearshore (c. 20 m) rocky bottom environment (Moissette et al. Reference Moissette, Koskeridou, Cornée and André2013). This association of algae and invertebrates is a possible analogue of the pre-coralligenous biocoenosis of Pérès and Picard (Reference Pérès and Picard1964). In some places, a photophilic seaweed or seagrass cover is indicated by the presence of minute grazing gastropods in small sandy pockets (e.g. Gibbulla sp., Tricolia spp.), chitons and bivalves (Barbatia sp., Chlamys spp., Lima sp.).
5.a.4. Facies association 4
The organisms found in this association may have lived at water depths of c. 20–60 m. Several communities have been recognized in this deeper, relatively quiet marine upper circalittoral environment. A number of biocoenoses of Pérès & Picard (Reference Pérès and Picard1964) have also been recognized within this facies association.
Facies 11. Among the soft-bottom biocoenoses, the ‘Détritique côtier’ (DC, coastal detritic bottoms) is well-represented in the Kritika Formation. It is characterized by coarse sands with abundant gravels, together with mollusc shells (whole and fragmented), bryozoans and calcareous red algae (notably rhodoliths, but also small unattached maërl branches). This biocoenosis displays a high faunal abundance and diversity; its shallowest occurrence in the Mediterranean is at depths of c. 30 m (Pérès & Picard, Reference Pérès and Picard1964; Bellan-Santini et al. Reference Bellan-Santini, Bellan, Bitar, Harmelin and Pergent2002). Together with bivalve shells and rhodoliths, free-living and erect rigid bryozoans (among them the deeper-water species Tervia irregularis) are relatively common in both Kritika sections. Benthic foraminifers such as Gavelinopsis praegeri, Asterigerinata planorbis, Neoconorbina terquemi and Elphidium crispum, together with abundant Buliminidae and Bolivinidae, may also characterize this biocoenosis. The high occurrence of the plano-convex forms may point to the presence or nearness of an area with subaquatic vegetation. The relatively high abundance of Buliminidae and Bolivinidae further indicates the influence of deeper water, high food input and low oxygen levels. Within this soft-bottom biocoenosis, a mollusc association with bivalves (Timoclea ovata, Parvicardium minimum, P. papillosum and Hiatella arctica) and gastropods (Emarginula fissura, Petalochoncus intortus, Turboella inconspicua, Rissoa ventricosa and Hinia serraticosta) ressembles the ‘Timoclea paleocommunity’ of Dominici (Reference Dominici2001) and points to a depth of c. 30–60 m. Lunulitiforms are free-living discoidal bryozoans adapted to live on the surface of soft, more or less sandy sea bottoms. Species belonging to this group have an optimum depth range of c. 30–60 m (Cook & Chimonides, Reference Cook and Chimonides1983; Bone & James, Reference Bone and James1993; O’Dea, Reference O’Dea2009). Colonies belonging to two lunulitiform species occur, occasionally in great numbers, in some of the sandy and shelly levels of sections Kritika-N and Kritika-S.
Within approximately the same depth range, when the terrigenous material (silt and clay) is more abundant another biocoenosis develops, the ‘fonds détritiques envasés’ (DE, muddy detritic bottoms). Among the molluscs, the gastropods Apporhais pespelecani and Turritella communis and the bivalve Timoclea ovata are characteristic elements of this community (Desbruyères, Guille & Ramos, Reference Desbruyères, Guille and Ramos1972/1973; Zenetos et al. Reference Zenetos, Christianidis, Pancucci, Simboura and Tziavos1997). Posidonia oceanica seagrass meadows may also have colonized patches of soft substrates at depths down to c. 40 m (Pérès & Picard, Reference Pérès and Picard1964; Moissette et al. Reference Moissette, Koskeridou, Cornée, Guillocheau and Lécuyer2007).
Facies 12. Concentrations of coralline algal rhodoliths can be found within the ‘Détritique côtier’ (DC, coastal detritic bottoms) of Pérès & Picard (Reference Pérès and Picard1964), especially in the ‘prâline faciès’ where comparable assemblages can be found. The bivalves Venus casina and Tellina donacina are part of a typical association of this facies. The rhodolithic nodules of the Kritika Formation (Fig. 7c) developed either on small pebbles or on biogenic nuclei such as fragments of mollusc shells or calcareous algae. They usually provided substrates for encrusting serpulid worms and bryozoans (Fig. 8a). Periodical rotation is necessary for rhodolith survival and growth; this generally occurs on high-energy sea bottoms, but is also due to overturning by various vagile benthic organisms.
Facies 13. Accumulations of large articulated and disarticulated oyster valves probably also took place in coastal detritic bottoms. The relative stability of the bivalve shells allowed the extensive colonization (Fig. 8d) of these ‘benthic islands’ (Taylor & Wilson, Reference Taylor and Wilson2003) by numerous organisms (not only encrusting, but also erect forms) in an otherwise soft-bottom habitat. In turn, the skeletal remains of the epibionts were dispersed throughout the enclosing sediment where they could be used as hard substrates by other organisms.
Facies 14. In the absence of suitable rocky bottoms in the Kritika Formation, the coralligenous biocoenosis (Pérès & Picard, Reference Pérès and Picard1964) is only represented by the second generation of beachrock carbonate crusts (with abundant calcareous red algae, bryozoans and Cladocora caespitosa colonial corals) and the small build-ups (locally occurring in the Faliraki-N section). Both were established at a depth of c. 20–40 m (Moissette et al. Reference Moissette, Koskeridou, Cornée and André2013). This is notably indicated by the accompanying mollusc fauna with bivalves (Chlamys multistriata, C. varia, Chama gryphoides, C. placentina, Pseudochama gryphina, Ostrea edulis, Spondylus gaederopus, Hiatella rugosa and Striarca lactea) and gastropods (turritellids, naticids, vermetids and herbivorous species such as Alvania cancellata, Rissoa spp., Bittium spp., Cerithiopsis tubercularis, Jujubinus spp., Diodora gibberula and Nassarius incrassatus).
5.b. Environmental parameters recorded by facies associations
5.b.1. Sedimentation
The lower Pleistocene deposits of the Kritika Formation developed in coastal areas of prevailing terrigenous input. In this environment, abundant invertebrate (especially mollusc) communities could thrive under conditions of high sediment accumulation and turbidity (often accompanied by reduced/fluctuating salinity and increased nutrient influx). Among them a number of infaunal bivalves (i.e. Abra alba, Cerastoderma spp., Corbula gibba, Dosinia lupinus, Lentidium mediterraneum) could dominate, taking advantage of these turbid-water deltaic settings which are unbearable to other suspension- and deposit-feeding organisms (Scarponi & Kowalewski, Reference Scarponi and Kowalewski2007; Weber & Zuschin, Reference Weber and Zuschin2013). In less turbid marine conditions, rather patchy seagrass meadows could also flourish together with their associated skeletal communities (Moissette et al. Reference Moissette, Koskeridou, Cornée, Guillocheau and Lécuyer2007).
Despite pronounced turbidity and highly mobile siliciclastic sediments, some hard substrates also occurred. Apart from several drowned beachrock slabs, which provided optimum sites for the main carbonate factory to develop within the Kritika Formation (Moissette et al. Reference Moissette, Koskeridou, Cornée and André2013), the only other partially stable hard substrates were the pebbles, the bivalve shells and the red algal rhodoliths used as ‘benthic islands’ (Fig. 8) by hard-bottom communities on the otherwise soft-sediment sea floor (Taylor & Wilson, Reference Taylor and Wilson2003).
5.b.2. Hydrodynamism
In the infralittoral Facies association 3, some benthic foraminiferal specimens (mostly belonging to Quinqueloculina, Triloculina, Elphidium, Textularia and Ammonia) are brownish coloured, highly polished and severely abraded, representing high-energy conditions. These reworked specimens are indicative of transport from nearshore shallow depths and/or mixing due to winnowing associated with high-energy environments. Among the benthic foraminifers, E. crispum and Porosononion subgranosum are also particularly frequent; they reach their highest numbers in present-day high-energy sandy substrates. The small infaunal bivalve Ervilia castanea is characteristic of shallow-water coarse sand habitats (Facies 8) exposed to wave action (Morton, Britton & Frias Martins, Reference Morton, Britton and Frias Martins1998). The dense populations of this species in the studied material, especially in the Kritika-N section, indicate that it was a very successful colonizer of such energetic environments. Deeper-water planktonic foraminifers and pteropods were also transported shorewards by coastal currents and deposited into shallower, narrow-shelf environments, especially during storms.
5.b.3. Depth zonation and sea-level changes
The Kritika Formation was deposited in continental to marine shallow-water, wave-dominated, muddy to sandy deltaic environments (Broekman, Reference Broekman1973, Reference Broekman1974; Moissette et al. Reference Moissette, Koskeridou, Cornée, Guillocheau and Lécuyer2007, Reference Moissette, Koskeridou, Cornée and André2013). Based on sedimentary structures (ripple marks, hummocky cross-stratification, planar and oblique bedding, firmgrounds, beachrock beds, rhizoliths, etc.) and facies associations, 13 genetic sequences have been recognized in the Kritika sections (Fig. 9) and four in the Faliraki sections (Fig. 10). The thickness of each sequence falls within the range c. 4–30 m. The main changes in thickness depend mostly on the presence or absence of conglomeratic beds. Both the overall depositional stacking pattern and the distribution of skeletal organisms do not change significantly from the bottom to the top of each of the sections, indicating that there is no major unconformity within the sections. In contrast, facies content displays strong variations inside each sequence. The maximum flooding surfaces are located within marine, marly or sandy bioclastic sediments with red algae and planktonic foraminifers (Facies association 4), or within marly to sandy deposits with hummocky cross-stratification, oblique bedding, shell accumulations (Facies association 3). In the Kritika-S section, maximum flooding surfaces are also underlined by firmgrounds (base of sequences J and I). The continental deposits are mainly composed of fluviatile, conglomeratic to sandy deposits with very few fossils from continental to lacustrine/fluviatile environments (Facies association 1). Emersions are locally recorded by beachrock beds and rhizoliths. Changes from lagoonal to infralittoral deposits are recorded by Facies associations 2 and 3, respectively.

Figure 9. High-resolution sequence stratigraphic model of the Kritika Formation in the Kritika area.

Figure 10. High-resolution sequence stratigraphic model of the Kritika Formation in the Faliraki area.
5.b.4. Nutrients
Facies association 2 generally contains a distinct, low-diversity and particularly thin-shelled foraminiferal fauna, which exhibits species-specific tolerance to the input of fresh water and/or nutrients delivered by terrestrial runoff. Indeed, the high occurrence (>80 %) of Aubignyna perlucida in some of the samples attests to freshwater influx and high concentrations of organic matter (Jorissen, Reference Jorissen1988). Severe episodes of dysoxia are not observed in the Kritika Formation, except for a very limited but intense episode in the Kritika-N section in which the benthic foraminiferal taxa Fursenkoina acuta, Bolivina and Bulimina are present in high abundance. These species are indicative of increased amounts of organic matter and decreased oxygen levels. The fine-grained character of the sediment promotes the deposition and preservation of organic matter and the occurrence of oxygen deficiency at or just below the sediment–water interface. Moreover, the high frequency of Ammonia beccarii in Facies association 3, a species highly resistant to environmental stresses, appears to be related to episodic hypoxia events in the benthos.
A number of infaunal bivalves (Abra alba, Corbula gibba, Lucinella divaricata, Nucula sulcata, Nuculana pella and Ringicula auriculata) can tolerate long periods of hypoxia and even anoxia (Diaz & Rosenberg, Reference Diaz and Rosenberg1995; Dominici, Reference Dominici2001; Nerlović, Doğan & Hrs-Brenko, Reference Nerlović, Doğan and Hrs-Brenko2011; Riedel, Zuschin & Stachowitsch, Reference Riedel, Zuschin and Stachowitsch2012). They are especially abundant in shallow muddy bottoms where water stratification, combined with nutrient-enriched terrestrial runoff, induces periods of low oxygen concentration.
5.b.5. Salinity
The abundance and diversity of skeletal organisms in most samples implies normal marine conditions during the deposition of the major part of the Kritika Formation. However, freshwater influx is also recorded by the occurrence in some levels of rare fluviatile/lacustrine organisms and by more common euryhaline taxa (benthic foraminifers, ostracodes and molluscs), indicating the presence of brackish-water environments such as lagoons and river mouths (Facies association 2). Isotopic analyses carried out on invertebrate carbonate shells (Lécuyer et al. Reference Lécuyer, Daux, Moissette, Cornée, Quillévéré, Koskeridou, Fourel, Martineau and Reynard2012) demonstrated that in the coastal environments of the Kritika conditions with fluctuating salinity were mostly caused by seasonal changes in freshwater inputs.
5.b.6. Temperature
Apart from numerous warm-temperate species characteristic of the present-day Mediterranean and near-Atlantic regions, a few thermophilic, subtropical–tropical taxa also occur in the studied deposits. Among them are the gastropod Turritella vermicularis (Fig. 7d) and the bryozoans Metrarabdotos (represented in many samples and even abundant in some of them; Cheetham, Reference Cheetham1967; Cheetham, Sanner & Jackson, Reference Cheetham, Sanner and Jackson2007) and rarer Biflustra savarti, Exechonella antillea and Thalamoporella.
Stable carbon and oxygen isotope compositions of gastropod and bivalve carbonate shells also indicate slightly higher mean seawater temperatures (22.0 ± 1.5 °C) than those typically found offshore present-day Rhodes (Lécuyer et al. Reference Lécuyer, Daux, Moissette, Cornée, Quillévéré, Koskeridou, Fourel, Martineau and Reynard2012).
5.b.7. Taphonomy
The quality of fossilization in the studied deposits is generally good with well-preserved aragonitic skeletons (corals, molluscs) and even moulds of the leaves and calcified casts of the rhizomes of Posidonia oceanica seagrass (Moissette et al. Reference Moissette, Koskeridou, Cornée, Guillocheau and Lécuyer2007). The excellent state of preservation of many fossils is chiefly due to the predominance of fine-grained siliciclastic material. This also explains why numerous organisms are preserved in situ, entombed in terrigenous sediments.
With the exception of the hard bottoms of the beachrock beds, covered by thick biogenic carbonate crusts, siliciclastic soft substrates prevail throughout the Kritika Formation. However, mixed with this terrigenous material are variable proportions of mollusc shells and coralline algal rhodoliths, together with lithic gravels (and even pebbles). The skeletal remains, whole or fragmented, and the gravels occurring in these types of environments are often used as hard substrates by numerous benthic organisms in a process named ‘taphonomic feedback’ (Kidwell, Reference Kidwell1986). Within soft-sediment habitats, these ‘benthic islands’ (Fig. 8) served as attachment for sessile organisms, especially encrusters, that otherwise could not have colonized such environments (Kidwell, Reference Kidwell1986; Taylor & Wilson, Reference Taylor and Wilson2003). The periodical overturning by waves, currents or vagile animals of these encrusted substrates may also encourage the settlement of other encrusters and therefore the formation of nodules (such as rhodoliths) with their characteristic concentric growth. The main consequences are an increase in: (1) stability and topographic complexity of the sea floor; (2) surfaces available for colonization; (3) biodiversity; (4) carbonate production; and (5) protection against physical stress, mechanical destruction and predation. This often results in changes in the composition, structure and dynamics of marine benthic communities and their ecological successions (Kidwell, Reference Kidwell1986). In the clayey and sandy deposits of the Kritika Formation, large oyster shells are often heavily encrusted by numerous bryozoans (with as many as 80 colonies per host bivalve) belonging to quite a lot (10–30) of species (Fig. 8d). They are typically accompanied by other encrusters such as calcareous algae, serpulids and smaller bivalves. There are apparently no significant differences between encrustation of convex and concave surfaces, indicating that these oyster valves were occasionally subjected to overturning by wave or current action, or by biological activities (Wilson, Reference Wilson, Knight and McLean1986). The circumrotatory growth of rhodoliths is another example of nodules formed by numerous generations of encrusting calcareous algae, often coupled with serpulid tubes and bryozoan colonies (Fig. 8a). Even small-sized substrates will be beneficial to some epizoan organisms such as free-living (lunulitiform) bryozoan colonies, which are adapted to soft sediments, but need minute shell fragments (or large sand grains) for attachment during initial stages of growth.
The fidelity of death assemblages to living communities depends on many parameters such as transport, fracturing and abrasion, dissolution, sedimentation rate and diagenesis. The remains of successive, non-contemporaneous, populations of skeletal organisms may be found together within a single bed. This phenomenon is known as time-averaging (Kidwell, Reference Kidwell1998) and is less pronounced in nearshore settings where sedimentation rates are high and within-habitat time-averaged assemblages consequently predominate. This is generally the case for the early Pleistocene siliciclastic sequences sampled in Rhodes, where shallow-water autochthonous and parautochthonous assemblages are commonly observed.
5.c. Vertical and lateral facies association distribution
High-resolution correlations were conducted within each studied areas. Nevertheless, it is presently not possible to correlate the Kritika and the Faliraki areas, separated by c. 6 km of unidentified deposits.
In the Kritika area (Fig. 9) the main maximum flooding surfaces are located at the boundaries of sequences G and at the bottom of sequences C and L, allowing genetic sequences to be grouped into five lower-resolution main sequences. These surfaces are located in infralittoral to upper circalittoral deposits. Sequences A–D are mostly characterized by repetitive superimpositions of lagoonal and marine deposits. The transgressive part of sequence E is typified by a generalization of fluviatile deposits over the whole area, indicative of a major regressive event. From sequence E–G, coarse-grained terrestrial deposits alternating with marine deposits in the north change into marine to brackish deposits in the south. From sequences H–M, terrestrial, lagoonal and marine deposits in the north change into lagoonal and marine deposits in the south. As a whole, terrestrial deposits are preserved in the north while lagoonal and marine deposits are dominant in the south, indicating apparent sources from the north of Rhodes. The repetitive pattern of genetic sequences also suggests a partly eustasy-controlled sedimentation under rather constant subsidence during the deposition of the deltaic complex. Indeed, the Gelasian is the time when significant sea-level fluctuations began to occur with amplitude of several tens of metres (Miller et al. Reference Miller, Mountain, Wright and Browning2011), as recorded in the deposits of the Kritika Formation. Nevertheless, in this littoral setting, the deposition of the fluviatile sediments of sequence E at the marine–continental transition is anomalous.
In the Faliraki area (Fig. 10) the investigated sections are characterized by dominant terrestrial to lagoonal deposits, with restricted marine intervals. Genetic sequences are organized in a similar way to the sequences in the Kritika area, also indicative of a subsiding coastal area.
Apart from subsidence, the presence of ball and pillow structures interpreted as seismites in the Kritika-S section (Fig. 3) and the abrupt development of terrestrial deposits over marine deposits within sequence E may indicate limited tectonic pulses. Such pulses were probably linked to the geodynamical location of Rhodes, at the front of the Aegean subduction (Hanken, Bromley & Miller, Reference Hanken, Bromley and Miller1996; Cornée et al. Reference Cornée, Moissette, Joannin, Suc, Quillévéré, Krijgsman, Hilgen, Koskeridou, Münch, Lécuyer and Desvignes2006a). From stable isotope analysis of carbonate shells, Lécuyer et al. (Reference Lécuyer, Daux, Moissette, Cornée, Quillévéré, Koskeridou, Fourel, Martineau and Reynard2012) proposed a near-constant temperature of 22.0 ± 1.5 °C for marine waters in sequences F2 and F3 (Faliraki-N section). The abrupt changes in salinity through these sequences were therefore interpreted as the result of tectonic forcing during the deposition of the Kritika Formation. Our new sequence stratigraphic framework indicates at least 13 repetitive terrestrial to marine genetic sequences, also suggesting repetitive salinity changes. As a consequence, in order to confirm the role of tectonics new geochemical investigations in other sequences are necessary to determine whether palaeotemperatures changed or remained constant during the deposition of the Kritika Formation.
Since no major unconformity was recorded in the Kritika siliciclastics, it is possible to use facies association analyses to propose a depositional model for these deltaic deposits (Fig. 11). Offshore, the Kritika Formation was deposited on a muddy to sandy, low-angle ramp interrupted by low-relief shoals (decimetre- to metre-sized). Hard substrate favourable for sessile communities were restricted, limited to conglomeratic bottoms or submerged beachrock slabs. As a whole, most of the flora and fauna developed in/on soft substrates, under constant turbid conditions in mostly marine areas. Moreover, storm events could transport lagoonal to littoral skeletal communities into more distal marine areas. Landward, deposits changed into lagoonal and then terrestrial siliciclastics. Beachrock beds could develop at the continental–marine transition.

Figure 11. Simplified reconstruction sketch of the facies association zonation in the Kritika Formation at a given time (not to scale).
5.d. Palaeogeography
The palaeogeography of the Kritika Formation is poorly constrained. In the Kritika area, terrestrial deposits are well developed in the north and become progressively rare towards the south. This is indicative of proximal depositional areas to the north, changing into lagoonal to marine, more distal areas to the south, as recorded by some palaeocurrent directions (Moissette et al. Reference Moissette, Koskeridou, Cornée, Guillocheau and Lécuyer2007). In the Faliraki area, prevalent palaeocurrents are also directed to the south or to the southeast. There, lagoonal to terrestrial deposits are dominant, as in the upper part of both Kritika sections. Elsewhere, along the SE coast of Rhodes, the Kritika Formation displays changing, terrestrial to marine, depositional settings (Fig. 1a). In central and NW Rhodes, Gelasian deposits are either absent or terrestrial (Meulenkamp, de Mulder & van de Weerd, Reference Meulenkamp, De Mulder and Van De Weerd1972; Hanken, Bromley & Miller, Reference Hanken, Bromley and Miller1996; van Hinsbergen et al. Reference van Hinsbergen, Krijgsman, Langereis, Cornée, Duermeijer and Van Vugt2007). As a whole, the Kritika Formation was part of a deltaic complex which originated in the north, indicating that Gelasian Rhodes was fully part of the Asian landmass (Meulenkamp, de Mulder & van de Weerd, Reference Meulenkamp, De Mulder and Van De Weerd1972).
6. Conclusions
Rich and generally well-preserved warm-temperate fossil communities of coralline algae, benthic foraminifers and invertebrates (mostly molluscs and bryozoans) characterize the mostly siliciclastic deposits of the lower Pleistocene Kritika Formation of Rhodes.
Fourteen different facies (grouped into four facies associations) have been identified in the studied sections. Their analysis indicates that deposition took place mostly in warm-temperate marine settings at a maximum depth of c. 50–60 m. Continental, lacustrine and lagoonal environments have also been recognized. Among the brackish-water and marine facies associations, several communities resemble the biocoenoses defined by Pérès & Picard (Reference Pérès and Picard1964) for the present-day Mediterranean.
Based on sedimentary structures and fossil assemblages, 13 genetic sequences have been documented in the Kritika Formation, allowing a sequence stratigraphic model to be proposed for these muddy to sandy deltaic deposits. The general organization of these sediments also suggests that Gelasian Rhodes was connected to the Asian landmass.
Despite the fact that global depositional stacking patterns and distribution of skeletal organisms do not change significantly within each of the studied sections, facies and fossil content display strong variations within each of the cycles. This suggests a partly eustasy-controlled, repetitive sedimentation, a general subsidence of Rhodes during the deposition of the Kritika Formation and a constant range of palaeoenvironmental conditions. Nevertheless, the lack of precise biostratigraphic indices does not allow a clear differentiation between the roles of eustasy and tectonics.
In these muddy deltaic environments, terrestrial runoff induced intermittent periods of increased sedimentation rates, freshwater influx and nutrient levels, resulting in episodic low oxygen concentrations in bottom waters. The main consequences are repeated changes in benthic communities, some of them later beautifully preserved (even in life position) within generally fine-grained terrigenous sediments.
In a sedimentary system dominated by siliciclastics, carbonates occur primarily as coralline algal crusts (especially developed on submerged beachrock slabs) and nodules (rhodoliths), and less abundantly as mollusc (especially bivalve) shells and bryozoan colonies. The rhodoliths, bivalve shells and many gravels and pebbles provided habitat for numerous associated organisms and served as benthic islands for epibionts. One of the major consequences is an increase in abundance and diversity of skeletal invertebrates over that of purely soft-bottoms at similar depths.
Acknowledgements
This research was partially supported by UMR 5276 CNRS (University of Lyon 1) and CNRS INSU SYSTER (‘Forearc vertical movements: frontal versus oblique subduction’). Financial support was also provided by the National and Kapodistrian University of Athens (Research Project 70/4/5849). We are grateful to G.-E. López-Otálvaro for analyses of calcareous nannoplankton and to G. Merzeraud for help with the sedimentological interpretations. We also thank Jürgen Titschack and an anonymous reviewer for their constructive comments on earlier versions of this paper.