1. Introduction
The Early Jurassic marine reptile remains are rarely reported outside of Europe (Bardet et al. Reference Bardet, Falconnet, Fischer, Houssaye, Jouve, Pereda Suberbiola, Pérez-Garcia, Rage and Vincent2014). The records include localities in North America (Nicholls, Reference Nicholls1976; McGowan, Reference McGowan1978; Dennison et al. Reference Dennison, Smith and Tipper1990; Martindale et al. Reference Martindale, Them, Gill, Marroquín and Knoll2017), Greenland (Marzola et al. Reference Marzola, Mateus, Milàn and Clemmensen2018), South America (Chong Diaz & Gasparini, Reference Chong Diaz and Gasparini1976; Gasparini, Reference Gasparini1979, Reference Gasparini1985, Reference Gasparini and Westermann1992; Gasparini et al. Reference Gasparini, Vignaud and Chong2000; Otero et al. Reference Otero, Soto-Acuña, Rubilar-Rogers, Rubilar-Rogers, Otero, Vargas and Sallaberry2015; Pardo Pérez et al. Reference Pardo Pérez, Otero, Suárez, Rubilar-Rogers, Otero, Vargas and Sallaberry2015; Otero & Sepúlveda, Reference Otero and Sepúlveda2020), Australia (Bartholomai, Reference Bartholomai1966; Thulborn & Warren, Reference Thulborn and Warren1980; Kear, Reference Kear2012), New Zealand (Sachs & Grant-Mackie, Reference Sachs and Grant-Mackie2003; Zammit, Reference Zammit2011), Madagascar (Buffetaut et al. Reference Buffetaut, Termier and Termier1981) and Asia (Hoffet & Le Maitre, Reference Hoffet and Le Maitre1939; Tanimoto & Okura, Reference Tanimoto and Okura1989; P Vignaud, unpub. PhD thesis, University of Poitiers, 1995) (summarized in Table 1). However, the majority of those reported ichthyosaurian and plesiosaurian specimens are too fragmentary and/or poorly preserved for the generic and specific determination and are therefore of reduced interest. In this regard, the most promising is the Fernie Formation of Canada that yielded articulated plesiosaurian and ichthyosaurian skeletal remains (Nicholls, Reference Nicholls1976; McGowan, Reference McGowan1978; Martindale et al. Reference Martindale, Them, Gill, Marroquín and Knoll2017). Although most of the marine reptile specimens from the Fernie Formation was identified as indeterminate plesiosaurians and ichthyosaurians, one of them, an articulated forelimb, was positively referred to a well-known European genus Ichthyosaurus De la Beche & Conybeare, Reference De la Beche and Conybeare1821, demonstrating the wide distribution of this genus in the Sinemurian (McGowan, Reference McGowan1978). More recently, another ichthyosaurian genus, Temnodontosaurus, was identified in the Hettangian–Sinemurian strata of the Atacama Desert, South America (Otero & Sepúlveda, Reference Otero and Sepúlveda2020), further highlighting the cosmopolitan distribution of at least some Early Jurassic marine reptiles. Nonetheless, our knowledge of the diversity and distribution of Early Jurassic (especially Pliensbachian and Toarcian) plesiosaurians and ichthyosaurians is largely restricted to extensively studied localities of Western Europe, in particular, the UK and Germany (e.g. McGowan & Motani, Reference McGowan and Motani2003; Benson et al. Reference Benson, Ketchum, Noé and Gómez-Pérez2011, Reference Benson, Evans and Druckenmiller2012; Fig. 1a); every new record of diagnostic marine reptiles in the Lower Jurassic Series outside of this region is therefore of great interest.
Table 1. Marine reptile occurrences in the Lower Jurassic Series outside of Europe (excepting Siberia)
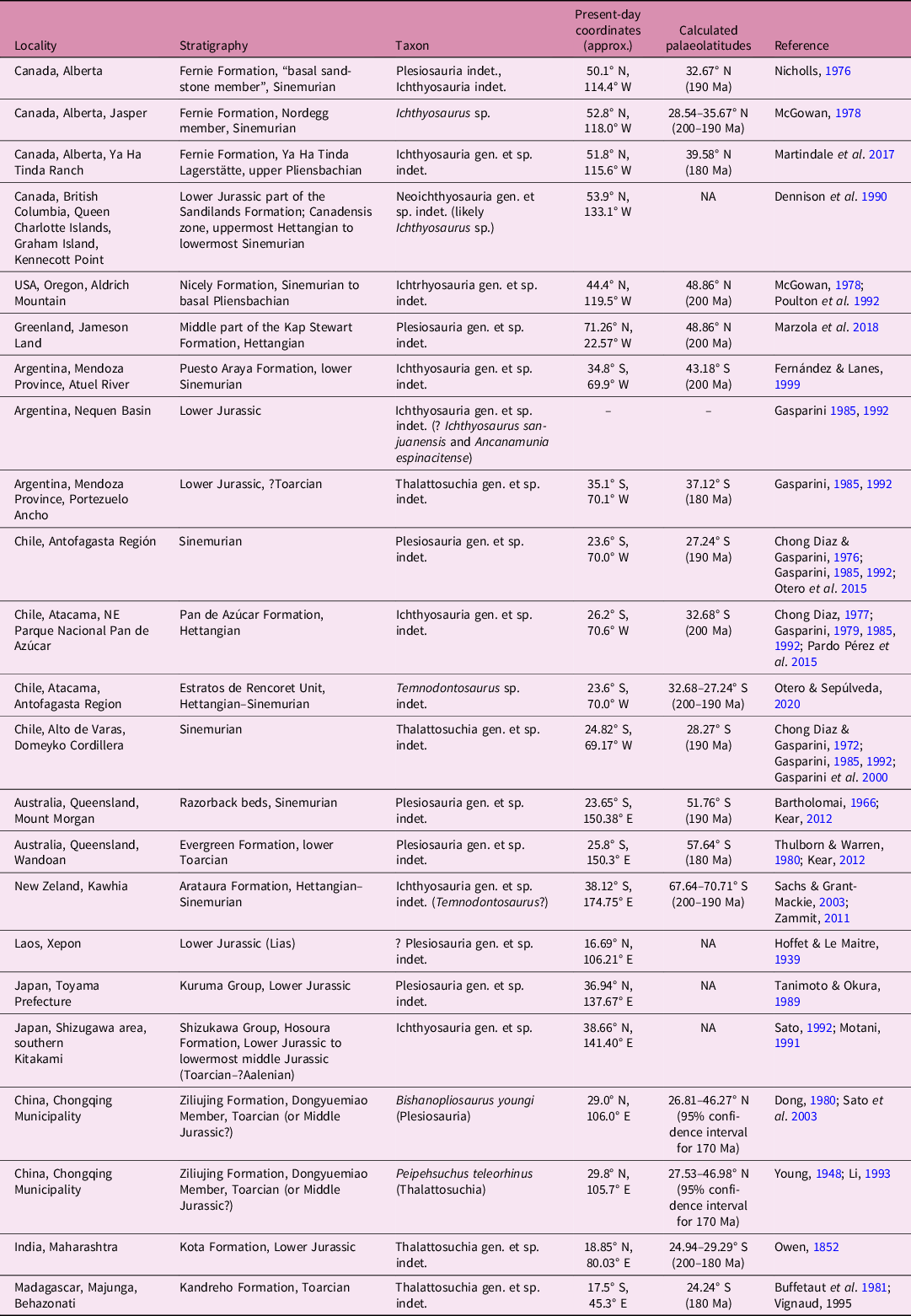
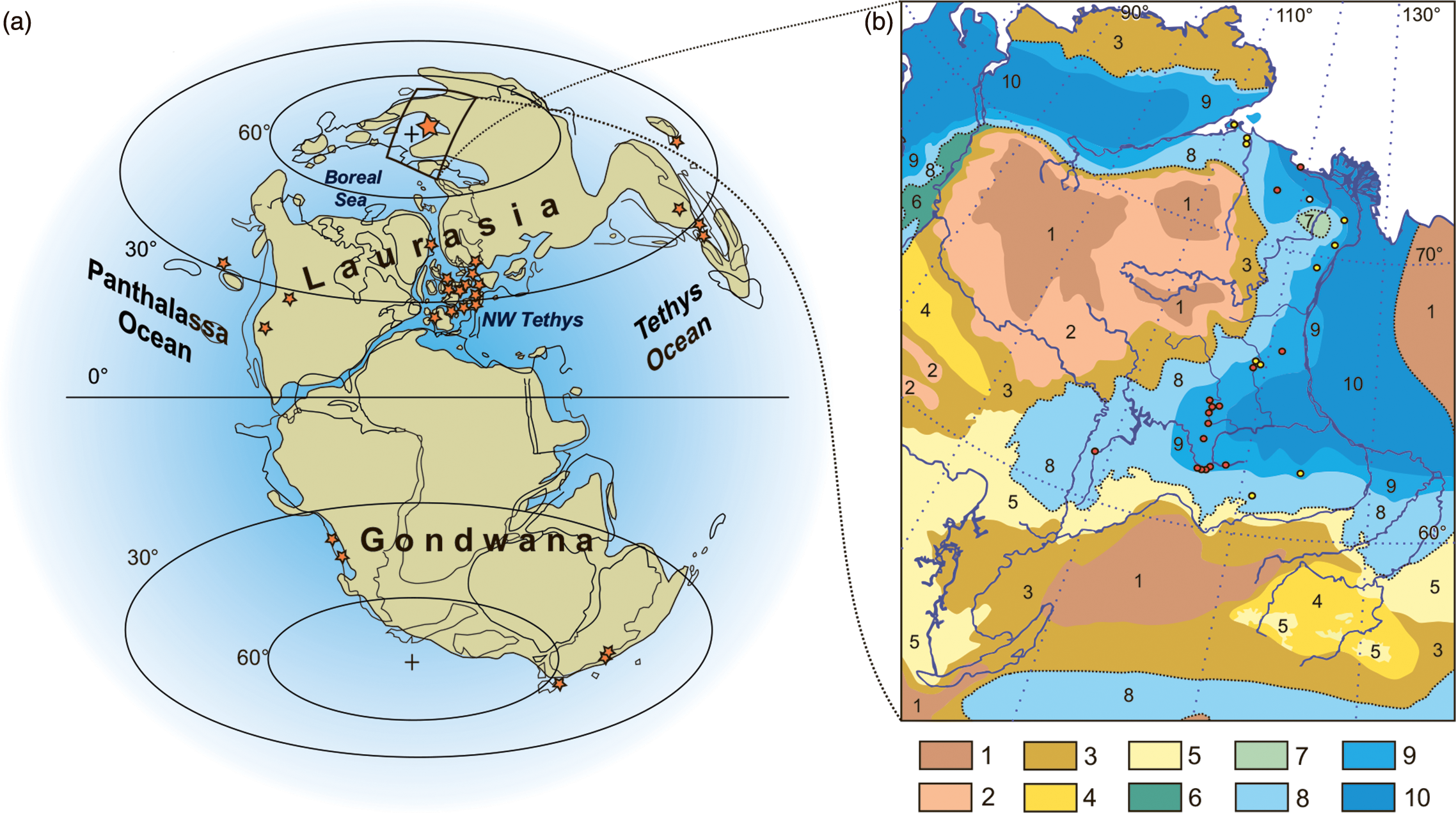
Fig. 1. Palaeogeographical and palaeoenvironmental contexts. (a) Global palaeogeography during the Pliensbachian–Toarcian interval (modified from Dera et al. Reference Dera, Neige, Dommergues and Brayard2011) showing the localities of Early Jurassic plesiosaurians and ichthyosaurians worldwide (shown by asterisks, for details see Table 1). (b) Palaeoenvironments in Eastern Siberia during early Toarcian time shown on a contour map with present-day latitudes and longitudes, rivers and coastline (modified from Devyatov et al. Reference Devyatov, Nikitenko and Shurygin2011); the localities of marine reptiles are shown by coloured dots with red (dark) dots indicating the localities from which materials are described here and yellow (pale) dots indicating other localities known from the literature (summarized in Rogov et al. Reference Rogov, Zverkov, Zakharov and Arkhangelsky2019). Palaeoenvironments for (b): 1, medium and low mountains; 2, highlands and uplands; 3, areas where denudation prevails over accumulation; 4, areas where accumulation prevails over denudation; 5, accumulation plains and lowlands with rivers and lakes; 6, coastal plains; 7, submarine elevations and/or islands; 8, sea and/or nearshore, depth 0–20 m; 9, sea, middle sublittoral zone, depth 20–80 m; 10, relatively deep sea, depth > 80 m.
In 1948, a Soviet palaeontologist and stratigrapher Vladimir V. Menner described plesiosaurian remains (including vertebrae, ribs, fragmentary propodial bones and girdle elements) from the outcrop near the confluence of the Iligir (Ulegir) and Vilyuy rivers, Eastern Siberia. Menner (Reference Menner1948) referred all the materials to Eretmosaurus Seeley, Reference Seeley1874, a genus originally described from the Sinemurian of England. He suggested that most of the materials belong to a single new species, that is, Eretmosaurus rzasnickii Menner, Reference Menner1948, named in honour of a geologist Adolf G. Rząśnicki, who collected the material. Following the conclusions of Rząśnicki (Reference Rząśnicki1918), Menner suggested that the age of materials is Middle Jurassic. However, subsequent study of the locality and re-examination of ammonite collection of Rząśnicki resulted in the conclusion that the specimens originate from the Toarcian part of the section (Krymholz & Tazikhin, Reference Krymholz and Tazikhin1957; Kirina, Reference Kirina1966). The scarcity of materials, misidentification of age, and the fact that the publication appeared in Russian in the local proceedings volume, meant that the contribution of Menner remained largely unnoticed. Subsequent workers re-identified the materials described by Menner as Plesiosauria indet., but have not corrected the data on suggested age (Storrs et al. Reference Storrs, Arkhangel’sky, Efimov, Benton, Shishkin, Unwin and Kurochkin2000; Arkhangelsky & Sennikov, Reference Arkhangelsky, Sennikov, Ivakhnenko and Kurochkin2008). The current repository of the specimens is unclear; although they are probably stored in the Palaeontological Institute of RAS, Moscow (AG Sennikov, pers. comm., 2019) we were unable to find this collection during work on this contribution.
Further geological surveys, prospecting and other works on the Lower Jurassic Series of Siberia brought a number of marine reptile occurrences. Some of those findings were mentioned in the geological literature, but not formally described and/or figured. Among those, the brief mention of “remains of a sea turtle of the genus Chelonia (identification by S.M. Kurzanov PIN)” from the lower Toarcian substage of the Anabar River (palaeolatitude c. 86° N; Knyazev et al. Reference Knyazev, Devyatov and Shurygin1991, p. 20; Rogov et al. Reference Rogov, Zverkov, Zakharov and Arkhangelsky2019) is likely a misidentification of plesiosaurian or ichthyosaurian remains, as (1) the oldest record of the true sea turtles (Chelonioidea) is late Barremian – early Aptian (Cadena & Parham, Reference Cadena and Parham2015) and (2) high-latitude turtle occurrences are rare and interpreted in connection with high mean annual temperature (e.g. Brinkman & Tarduno, Reference Brinkman and Tarduno2005; Nicholson et al. Reference Nicholson, Holroyd, Benson and Barrett2015). As the current location of this specimen is unknown, the verification of its former taxonomic identification is not possible.
The records of marine reptile occurrences in Siberia were recently summarized by Rogov et al. (Reference Rogov, Zverkov, Zakharov and Arkhangelsky2019), who collected data on 25 localities of Early Jurassic marine reptiles in Siberia spanning the lower Hettangian – uppermost Toarcian stages. The calculation of the palaeolatitudes for these marine reptile occurrences demonstrate that all of them are located at incomparably higher palaeolatitudes (mostly above 80th) than any other finding of Early Jurassic marine reptiles globally (cf. Table 1). However, only one isolated plesiosaurian vertebra from the Eren Formation (Toarcian) of the Anabar Bay was described by Rogov et al. (Reference Rogov, Zverkov, Zakharov and Arkhangelsky2019). At present, this specimen remains the only Lower Jurassic plesiosaurian material from Siberia formally described since the publication of Menner (Reference Menner1948). Our knowledge of marine reptiles from these extreme palaeolatitudes is therefore still poor.
Of great importance in this respect is the contribution of expeditions in Vilyuy River basin led by TA Kirina during 1958–1964. Kirina and colleagues managed to collect a significant number of plesiosaurian and ichthyosaurian remains from the Pliensbachian and Toarcian stages as well as several specimens from the Middle Jurassic deposits. The materials were transferred to LI Khozatsky, a prominent Soviet palaeoherpetologist (see Danilov et al. Reference Danilov, Cherepanov and Vitek2013), and stored in the Department of Vertebrate Zoology, Leningrad State University (today’s Saint Petersburg State University) and in the Zoological Institute of the Academy of Sciences of the USSR (currently Zoological Institute of RAS, St Petersburg). However, due to their fragmental nature, the study of these materials remained limited to several unpublished student courseworks supervised by Khosatsky in 1961, 1962, 1969 and 1970 (Danilov & Cherepanov, Reference Danilov and Cherepanov2014). Knowledge of marine reptiles has significantly increased since then, enabling a fresh look at these specimens. This contribution is based on a study of the collection of TA Kirina and represents the first formal description of the rich marine reptile assemblages from the Lower Jurassic Series of Siberia.
Institutional abbreviations: SPbU VZ – Department of Vertebrate Zoology, Faculty of Biology, Saint Petersburg State University, Saint Petersburg, Russia; PIN – Borissiak Paleontological Institute of the Russian Academy of Sciences, Moscow, Russia; ZIN PH – Paleoherpetological Collection, Zoological Institute of the Russian Academy of Sciences, Saint Petersburg, Russia.
2. Geological and palaeoenvironmental settings
The Vilyuy River basin is mostly located within the Vilyuy Syneclise. During the Early Jurassic Epoch, this territory was covered by a shallow epicontinental sea (Fig. 1b). In the peripheral part of the Vilyuy Syneclise mostly continental and deltaic deposits of the Ukugut Formation (Hettangian – lower part of the upper Pliensbachian) are present, overlain by marine terrigenous deposits with rich invertebrate fauna of the Tyung (upper Pliensbachian) and Suntar (Toarcian – lower Aalenian) formations (Knyazev et al. Reference Knyazev, Devyatov and Shurygin1991, Reference Knyazev, Devyatov, Kutygin, Nikitenko and Shurygin2003; Goryacheva, Reference Goryacheva2017). Both the Tyung and Suntar formations yield abundant remains of marine reptiles (Kirina, Reference Kirina1966; Knyazev et al. Reference Knyazev, Devyatov and Shurygin1991, Reference Knyazev, Devyatov, Kutygin, Nikitenko and Shurygin2003).
The Tyung Formation (upper Pliensbachian) consists of the dark siltstones and clay with occasional rounded pebbles and gravels at the bottom, and one or a few levels with glendonites at the top (Kirina, Reference Kirina1966; Knyazev et al. Reference Knyazev, Devyatov and Shurygin1991). These sediments were deposited in a shallow sublittoral setting (Knyazev et al. Reference Knyazev, Devyatov and Shurygin1991). The abundance of glendonites and the occurrence of dropstones (Suan et al. Reference Suan, Nikitenko, Rogov, Baudin, Spangenberg, Knyazev, Glinskikh, Goryacheva, Adatte, Riding, Föllmi, Pittet, Mattioli and Lécuyer2011) is evidence of cold conditions and possible near-freezing seasonal bottom temperatures. The palaeotemperatures calculated based on the isotopic composition of oxygen in bivalve shells as reported are 14.4–19.8 °C (Devyatov, Reference Devyatov, Bogatov, Gol’bert, Gurari and Surkov1983), although their reliability is unknown because of a lack of a proper analytical protocol. Studies of invertebrates emphasize the end-Pliensbachian biotic crisis in Siberian seas and almost complete faunal renewal during early Toarcian time (e.g. Nikitenko & Mickey, Reference Nikitenko, Mickey, Beaudoin and Head2004; Zakharov et al. Reference Zakharov, Shurygin, Il’ina and Nikitenko2006).
The Suntar Formation (Toarcian – lowermost Aalenian) is dominated by dark-grey (occasionally black or blue) clays (Knyazev et al. Reference Knyazev, Devyatov and Shurygin1991). From the bottom upwards there are trends of coarsening of terrigenous material, a decrease in organic matter and an increase in the abundance of macrofauna; in the uppermost part of the formation, the facies vary as a result of various transitions to the overlying Middle Jurassic sediments (Knyazev et al. Reference Knyazev, Devyatov and Shurygin1991). In the early Toarcian, the westwards ingression of the Vilyuy Bay is recorded, which resulted in the maximum sedimentation in the peripheral part of the basin. This was followed by a regression in the second half of the Toarcian (Knyazev et al. Reference Knyazev, Devyatov and Shurygin1991).
Earliest Toarcian time was characterized by a warmer climate (e.g. Ilyina, Reference Ilyina1985; Zakharov et al. Reference Zakharov, Shurygin, Il’ina and Nikitenko2006; Korte et al. Reference Korte, Hesselbo, Ullmann, Dietl, Ruhl, Schweigert and Thibault2015). The palaeotemperatures for the Vilyuy Syneclise in the early Toarcian based on the isotopic composition of oxygen in bivalve shells are 16.9–24.5 °C (median, 20.5 °C; Devyatov, Reference Devyatov, Bogatov, Gol’bert, Gurari and Surkov1983). In the Eastern Siberia, this time is characterized by a marked northwards shift of the ranges of thermophilic invertebrate taxa paralleled by disappearance of Boreal taxa (Zakharov et al. Reference Zakharov, Shurygin, Il’ina and Nikitenko2006). However, the early Toarcian warming in Siberia was short-lived; there are clear signs of cooling during late Toarcian time (Ilyina, Reference Ilyina1985; Vakhrameev, Reference Vakhrameev1987) and an increase of endemism of ammonite and belemnite boreal faunas (Meledina et al. Reference Meledina, Shurygin and Dzyuba2005). Furthermore, relatively few glendonites are recorded at the top of the Toarcian Stage of Eastern Siberia, whereas their lower Toarcian occurrences are unknown (Rogov et al. Reference Rogov, Zverkov, Zakharov and Arkhangelsky2019). The temperature continued to decrease during the earliest Middle Jurassic Epoch, as inferred by the closure of the Viking Strait and thus the strong reduction of heat transport to the isolated Boreal Sea (Korte et al. Reference Korte, Hesselbo, Ullmann, Dietl, Ruhl, Schweigert and Thibault2015; Rogov et al. Reference Rogov, Zverkov, Zakharov and Arkhangelsky2019).
For more details on the stratigraphy, palaeoenvironments and palaeoclimates of Eastern Siberia we direct the reader to Kirina (Reference Kirina1966), Knyazev et al. (Reference Knyazev, Devyatov and Shurygin1991, Reference Knyazev, Devyatov, Kutygin, Nikitenko and Shurygin2003), Devyatov et al. (Reference Devyatov, Nikitenko and Shurygin2011), Shurygin et al. (Reference Shurygin, Nikitenko, Meledina, Dzyuba and Knyazev2011), Suan et al. (Reference Suan, Nikitenko, Rogov, Baudin, Spangenberg, Knyazev, Glinskikh, Goryacheva, Adatte, Riding, Föllmi, Pittet, Mattioli and Lécuyer2011), Goryacheva (Reference Goryacheva2017) and Rogov et al. (Reference Rogov, Zverkov, Zakharov and Arkhangelsky2019).
3. Materials and methods
3.a. Materials
The total of 143 specimens comprising the collection of TI Kirina and seven additional specimens collected by other workers from the Lower Jurassic Series of Eastern Siberia are stored in ZIN PH (122 specimens) and SPbU VZ (28 specimens). Three of these specimens were collected in 2019 during the expedition of SPbU VZ to Vilyuy River basin. The studied materials are summarized in online Supplementary Table S1 (available at http://journals.cambridge.org/geo).
Since the discovery, the materials have remained largely unprepared. For this study, a number of specimens were prepared from matrix and nodules using pneumatic and hand preparation tools, and then soaked with consolidant and glued.
Occurrences of marine reptile remains in the Vilyuy River basin were reported from several beds (corresponding to different biostratigraphic units) within the Pliensbachian, Toarcian and Aalenian stages (Kirina, Reference Kirina1966). However, for only a limited number of specimens, the information regarding their precise stratigraphic position and the corresponding bed was recorded in the field sample card and, for most of them, it was restricted to an identification number of the outcrop and age (see Fig. 2 and Table S1). Some specimens were collected ex situ on a beach and from screes. Furthermore, during the students’ coursework the specimen sample cards were separated from many specimens and replaced by brief and generalized annotations of geography and presumed age. In the current situation, for many specimens it is only possible to identify the series/stage and locality or wider area from which specimens originated (with a few exceptions; Table S1). In rare cases, it is possible to identify the corresponding bed and ammonite zone (Table S1). The stratigraphic columns of outcrops from which marine reptile remains are reported as well as their possible correlation, based on the work of Kirina (Reference Kirina1966), are presented in Figure 2.

Fig. 2. Stratigraphic sections reproduced from Kirina (Reference Kirina1966) with updated stratigraphic context (Knyazev et al. Reference Knyazev, Devyatov and Shurygin1991, Reference Knyazev, Devyatov, Kutygin, Nikitenko and Shurygin2003; Shurygin et al. Reference Shurygin, Nikitenko, Meledina, Dzyuba and Knyazev2011). (a) Tung River, outcrops 29–31. (b–e), Markha River, outcrops (c) 14, (d) 15 and (e) 19a. (f–h), Vilyuy River outcrops (f) 18, (g) 16 and (h) 12. Lithology and other symbols: 1, conglomerate; 2, pebbles and breccia; 3, sand; 4, loose sandstone; 5, carbonatic sandstone; 6, silt; 7, clay, sandy; 8, clay and shale; 9, marlstone; 10, limestone; 11, siderite; 12, coal; 13, limestone concretions; 14, sandstone concretions; 15, dolerite clasts; 16, cross-bedding; 17, phosphorite; 18, unconformity; 19, wood; 20, flora; 21, bivalves and other invertebrates; 22, ammonites; 23, marine reptile occurrences from Kirina (Reference Kirina1966) with no details on their taxonomic assignment; 24, ichthyosaurians; 25, plesiosaurians.
Ichthyosaurian remains are reported from the late Pliensbachian and Toarcian intervals of the Vilyuy, Markha, Ygyatta and Sinyaya rivers (Kirina, Reference Kirina1966). However, according to the available data (preserved field sample cards and the catalogue of VG Borkhvardt), the studied material from the collection of Kirina includes ichthyosaurian remains from only the lower Toarcian of the Vilyuy and Markha rivers. Additionally, one small vertebra (ZIN PH 1/212) was found by SG Parkhomenko in 1921 at Cape Tumul, mouth of the River Olenyok (Fig. 1b; Table S1), which is Hettangian–Sinemurian in age. Two more vertebra (ZIN PH 1/213 and 2/213) were collected by VV Zhukov in 1959 from the Middle Oinyohoy River (tributary of the Udzha River) (Fig. 1b; Table S1), which are late Pliensbachian – Toarcian in age. The studied materials are largely represented by isolated vertebral centra, but also include three propodial bones (ZIN PH 39/211, ZIN PH 89/211 and SPbU VZ R Ip27), a fragment of rostrum with teeth (ZIN PH 24/211) and a partial skeleton (ZIN PH 3/211).
Plesiosaurian occurrences are relatively more abundant. They are reported for the upper Pliensbachian, Toarcian and Aalenian deposits of the Vilyuy, Markha, Ygyatta and Sinyaya rivers (Kirina, Reference Kirina1966). In the available materials, the majority of specimens are from the Vilyuy and Markha rivers; one rib fragment is from the Ygyatta River (ZIN PH 40/211) and no specimens are from the Sinyaya River (Table S1). One rib of a large plesiosaurian (SPbU VZ R Sp8, and its part that is stored in ZIN, ZIN PH 26/211) is from the upper Toarcian of the Linde River, tributary of the Lena River. Additionally, one dorsal vertebral centrum (SPbU VZ R Sp9) and numerous rib fragments were collected by NN Tasikhin in 1963 from the Eksa River, tributary of the Chona River. There are only five specimens reliably assigned to the upper Pliensbachian deposits: the isolated cervical centrum (ZIN PH 6/211) from outcrop 12 of the Vilyuy River; and two rib fragments (ZIN PH 42/211 and 43/211), one phalanx (ZIN PH 44/211) and one caudal rib (ZIN PH 45/211) from outcrop 16 of the Vilyuy River. Several specimens of unambiguously Middle Jurassic age will be described elsewhere. The remaining materials are Toarcian in age (Table S1). These are largely represented by isolated vertebrae, rib fragments and propodial bones, rarely occurring epipodial elements and phalanges, as well as girdle elements. Two specimens are represented by associated skeletal elements: a partial vertebral column (SPbU VZ R Sp11) and an articulated pelvic girdle and sacral vertebrae (SPbU VZ R Sp38). Although insufficient for a low-rank taxonomic identification, these two specimens provide encouragement to search for articulated skeletons in the Vilyuy River basin.
3.b. Methods
Present-day GPS coordinates for the fossiliferous outcrops reported by Kirina (Reference Kirina1966) and other marine reptile localities (Table 1) were used to calculate palaeolatitudes by applying the Paleolatitude.org online calculator (van Hinsbergen et al. Reference van Hinsbergen, de Groot, van Schaik, Spakman, Bijl, Sluijs, Langereis and Brinkhuis2015) and its default palaeomagnetic frame (Torsvik et al. Reference Torsvik, Van der Voo, Preeden, Mac Niocaill, Steinberger, Doubrovine, van Hinsbergen, Domeier, Gaina, Tohver, Meert, McCausland and Cocks2012). The resulting palaeolatitude calculations are presented in Tables 1 and 2.
Table 2. Palaeolatitudes for Early Jurassic marine reptile localities of Eastern Siberia (highest in bold)
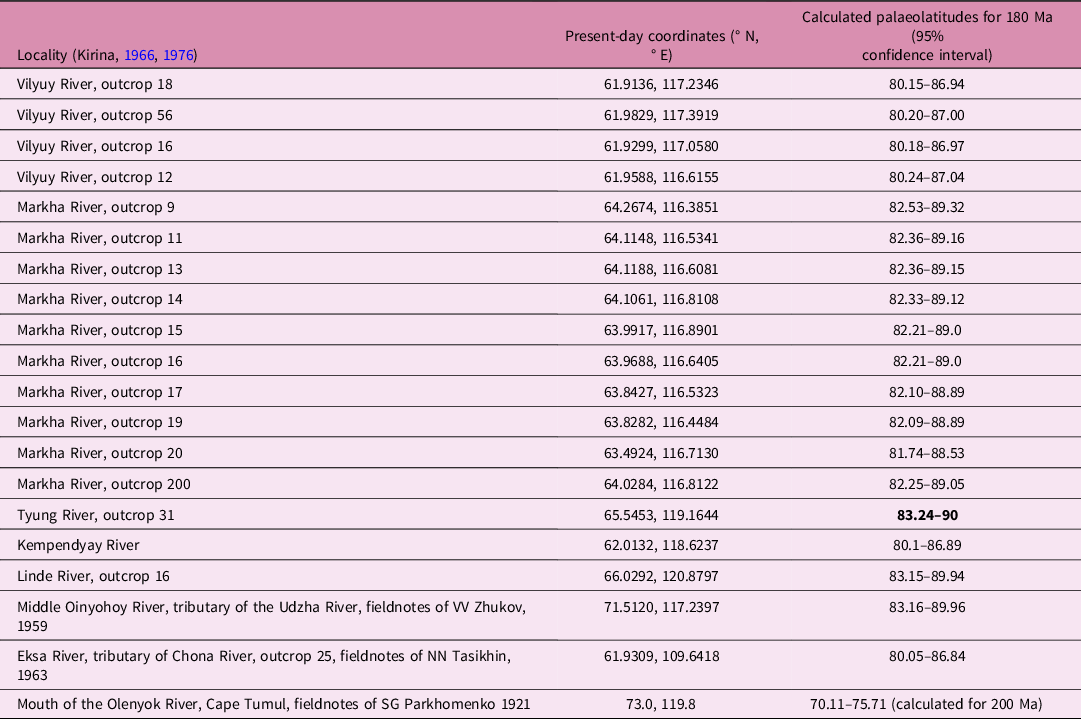
4. Survey of the marine reptile material
4.a. Ichthyosauria
The specimen ZIN PH 24/211 is a partial rostrum of a large ichthyosaurian with its structure revealed by transverse breakages (Fig. 3a). It has robust and large teeth, with the largest preserved tooth being 60 mm high apicobasally, although incomplete (Fig. 3a2). The crowns are broken in all preserved teeth. However, their impressions in matrix provide taxonomically important information: at least one carina could be observed from the impression (Fig. 3a5) and its presence is also supported by the keeled cross-sectional shape of the pulp cavity filled with calcite (Fig. 3a6). Temnodontosaurus Lydekker, Reference Lydekker, Nicholson and Lydekker1889 is the only known genus of post-Triassic ichthyosaurians that evolved carinate morphology of the tooth crown, known for three of its species, namely T. platyodon, T. trigonodon and T. eurycephalus (McGowan, Reference McGowan1974, Reference McGowan1996; McGowan & Motani, Reference McGowan and Motani2003; Martin et al. Reference Martin, Fischer, Vincent and Suan2012). We therefore refer ZIN PH 24/211 to Temnodontosaurus sp. The ammonites reported as collected with this specimen and originally identified as Dactylioceras gracile and D. athleticum (information from the label; Kirina, Reference Kirina1966) allow the referral to Dactylioceras commune Ammonite Biozone of the lower Toarcian strata (Kutygin & Knyazev, Reference Kutygin and Knyazev2000; Knyazev et al. Reference Knyazev, Devyatov, Kutygin, Nikitenko and Shurygin2003; Shurygin et al. Reference Shurygin, Nikitenko, Meledina, Dzyuba and Knyazev2011).

Fig. 3. Ichthyosaurian remains from the Pliensbachian–Toarcian stages of Eastern Siberia. (a) Fragmental rostrum of Temnodontosaurus sp. ZIN PH 24/211 in (a1, a2) left lateral and (a3, a4) posterior views, and (a5, a6) details of its teeth. (b) Neoichthyosauria indet. caudal centrum ZIN PH 4/211 in (b1) articular and (b2) lateral views. (c) Neoichthyosauria indet. fragmental humerus ZIN PH 89/211 in (c1) anterior, (c2) ventral, (c3) proximal and (c4) distal views. (d) Left humerus, SPbU VZ R Ip 27, in (d1) ventral, (d2) proximal, (d3) anterior and (d4) distal views. (e–i) Remains of a small ichthyosaurian ZIN PH 2/211 here referred to as Stenopterygius sp. from the lower Toarcian of the Markha River. (e) Partially articulated vertebral column, neural arches and ribs. (f) Partial frontal region of the skull and (f2) its interpretation. (g) Isolated tooth. (h) Left coracoid in (h1) dorsal, (h2) medial and (h3) anterior views; the dotted line shows the reconstructed outline of the element. (i) Right femur in (i1) proximal, (i2) anterior, (i3) ventral and (i4) distal views. Scale bars: (a1–4, b–f, h, i) 50 mm; (a5, a6) 20 mm; and (g) 10 mm.
Two large ichthyosaurian vertebrae are present in the collection: a caudal centrum (ZIN PH 4/211; Fig. 3b) is 123 mm in maximum diameter and originates from the Harpoceras falciferum zone of outcrop 18 at Vilyuy River; and a probably presacral (uncertain because of poor preservation) centrum (ZIN PH 2/213; Supplementary Fig. S1c) is 130 mm in maximum diameter and comes from the upper Pliensbachian–Toarcian deposits of the Udzha River basin. These vertebrae further support the presence of large ichthyosaurians during late Early Jurassic time in Eastern Siberia. However, their taxonomic identification is equivocal due to the existence of not only Temnodontosaurus but also large leptonectids during the Early Jurassic Epoch (e.g. McGowan & Motani, Reference McGowan and Motani2003). We therefore refer the specimens to Neoichthyosauria indet.
ZIN PH 89/211 (Fig. 3c) and SPbU VZ R Ip27 (Fig. 3d) are propodial bones (most likely humeri, see extended description in online Supplementary Material S1). Both of these have dorsoventrally compressed distal facets and lack an anterodistal facet for a preaxial accessory epipodial element; instead there is a characteristic anteriorly protruding leading edge tuberosity, which is common in Early Jurassic non-ophthalmosaurid neoichthyosaurians (e.g. McGowan & Motani, Reference McGowan and Motani2003). ZIN PH 89/211 is markedly larger than SPbU VZ R Ip27 (95 mm in preserved proximodistal length, c. 13–14 cm in reconstructed total length; compare with 8-cm-long SPbU VZ R Ip27). Judging from the preserved part of ZIN PH 89/211, the deltopectoral crest and the dorsal process were relatively well pronounced (Fig. 3c3); it is therefore more likely that the specimen represents a thunnosaurian ichthyosaurian, rather than a more basal neoichthyosaurian in which the trochanters are poorly developed (e.g. Johnson, Reference Johnson1979; McGowan & Motani, Reference McGowan and Motani2003). In contrast, the processes on SPbU VZ R Ip27 are poorly developed (Fig. 3d2), indicating that it more likely belongs to a non-thunnosaurian neoichthyosaurian. The precise spatiotemporal data on these specimens are currently lacking.
ZIN PH 2/211 is a partial skeleton of a small ichthyosaurian (Fig. 3e–i) originating from the lowermost Toarcian deposits of outcrop 9, Markha River. This is the only bone association in the collection that undoubtedly represents a single individual. The specimen comprises some fragments of the dermatocranium, one tooth, partially articulated vertebral column, partial coracoid and a nearly complete left femur. The preserved portion of the dermatocranium (Fig. 3f) is interpreted as a frontal region with partially exposed nasal, prefrontal, frontal, postfrontal and parietal. If this interpretation is correct, we observe no contact of the prefrontal and frontal, characteristic of basal thunnosaurians (Motani, Reference Motani2005; Maxwell et al. Reference Maxwell, Fernández and Schoch2012 b; Marek et al. Reference Marek, Moon, Williams and Benton2015). This condition makes ZIN PH 2/211 more similar to basal neoichthyosaurians and to ophthalmosaurids. The preserved tooth has a relatively stout conical crown (basal diameter to apicobasal length ratio, 0.55). The enamel ornamentation is weak and composed of rare striations (Fig. 3g). The root is only slightly wider than the basal crown and bears plications, characteristic of non-ophthalmosaurid neoichthyosaurians (Maxwell et al. Reference Maxwell, Caldwell and Lamoureux2012 a).
The available vertebral centra of ZIN PH 2/211 are from presacral and caudal regions. Their description and figures are provided in the online Supplementary Material S1.
The coracoid of ZIN PH 2/211 is markedly elongated (preserved anteroposterior length to mediolateral width ratio, 1.4). The anteromedial process of the coracoid is relatively poorly developed (Fig. 3h1). The anterior notch was likely small and narrow. Based on the preserved part, the posterolateral notch is likely absent or it was extremely reduced. Among moderate-sized Early Jurassic ichthyosaurians, this condition is characteristic of Stenopterygius (e.g. Johnson, Reference Johnson1979; Maisch & Matzke, Reference Maisch and Matzke2000). The intercoracoidal facet is lenticular in outline with its dorsoventral thickness increasing anteriorly (Fig. 3h2). Judging from the angle between the intercoracoidal surface and the dorsal surface, it could be suggested that the angle between the articulated coracoids was close to 170°.
Several available distal limb elements are all hexagonal in dorsal/ventral outline.
The left femur is nearly completely preserved. It is oriented based on criteria of Reference Maxwell, Zammit and DruckenmillerMaxwell et al. (2012c ). The femur is markedly proximodistally elongated and slender. The distal end is not strongly anteroposteriorly expanded, unlike the distal femoral blade in basal neoichthyosaurians (see e.g. Fischer et al. Reference Fischer, Bardet, Benson, Arkhangelsky and Friedman2016, character 83; Moon, Reference Moon2019, character 269). The shaft is straight, unlike the curved shaft in Ichthyosaurus (Massare & Lomax, Reference Massare and Lomax2019). The dorsal and ventral processes are weak, compared with those of ophthalmosaurids (e.g. Maxwell et al. Reference Maxwell, Zammit and Druckenmiller2012 c). There is a decrease in dorsoventral width between the tibial and fibular facets (Fig. 3i4).
In summary, in our opinion the combination of features (weak ornamentation of the crown; absence of chevron facets in the postflexural centra; elongated coracoids with relatively short anteromedial process, narrow anterior notch and reduced posterior notch; hexagonal phalanxes; slender and straight femur with minor distal expansion) allows the referral of ZIN PH 2/211 to Stenopterygius (see Johnson, Reference Johnson1979; Maisch & Matzke, Reference Maisch and Matzke2000; McGowan & Motani, Reference McGowan and Motani2003; Maisch, Reference Maisch2008; Maxwell et al. Reference Maxwell, Fernández and Schoch2012 b).
A number of specimens are isolated vertebral centra belonging to moderate and small ichthyosaurians (Table S1). These are all identified as Neoichthyosauria indet. based primarily on the temporal setting (all post-Triassic ichthyosaurians are known to belong to Neoichthyosauria), and also on bicipital rib articulations in the presacral centra and on the marked anteroposterior shortening of the centra relative to their width and height (e.g. Maisch, Reference Maisch2010; Moon, Reference Moon2019). For descriptions and figures of these specimens we direct the reader to the Supplementary Material S1 (including online Supplementary Fig. S3).
4.b. Plesiosauria
Below we provide a description of the plesiosaurian materials, ranging them by their observed morphotypes.
Specimens ZIN PH 48/211, 85/211, 86/211, 93/211 and 110/211, SPbU VZ R Sp22c and SPbU VZ R Sp36 (Fig. 4a–c; online Supplementary Fig. S4) are the cervical vertebrae with articulated neural arches and ribs lacking any visible trace of a suture (criterion of maturity sensu Brown, Reference Brown1981; although in most of these specimens the neural arches and ribs are largely broken). The centra are mediolaterally wider than they are long anteroposteriorly and high dorsoventrally (width > length > height). The articular surfaces are slightly concave, overall flat, giving the centrum a ‘platycoelous’ condition. The anterior surfaces bear a distinct U- or V-shaped notochordal pit (e.g. Fig. 4b1, c1), whereas on the posterior surface the notochordal pit is less pronounced as a horizontal scar or a circular depression (Fig. 4c4). There are concentric grooves on articular surfaces, especially marked in their peripheral region. We suggest that these structures represent cyclical growth marks, similar to those of some other vertebrates (e.g. Newbrey et al. Reference Newbrey, Siversson, Cook, Fotheringham and Sanchez2013; Skutschas et al. Reference Skutschas, Kolchanov, Bulanov, Sennikov, Boitsova, Golubev and Syromyatnikova2020), possibly indicating the maturity of these specimens. The peripheral region of articular surfaces is slightly raised over the remaining outer surface and bears rugose ornamentation laterally and ventrally. The ventral surface of each centrum lacks a ventral keel and bears small paired foramina. The lateral surface has a poorly pronounced lateral keel, located close to the presumed articulation with the cervical rib. The rib articulations are located ventrolaterally and are anteroposteriorly elongated and dorsoventrally compressed; they occupy no less than one-half of the centrum length. Proximally, the ribs bear anterior and posterior grooves terminating in pits. The pits are origins of a thin horizontal canal, separating the rib articulation with the centrum onto two parts. This canal could be observed in the broken rib of ZIN PH 85/211 (online Supplementary Fig. S4d). The neural arch is nearly completely preserved in ZIN PH 86/211 with only the dorsal portion of the neural spine broken (Fig. 4a). The prezygapophyses are not medially united to each other and their combined width is narrower that the centrum width. The prezygapophyseal facets are somewhat trough-like and face dorsomedially (Fig. 4a1). The postzygapophyseal facets face ventrolaterally (Fig. 4a4). The neural canal is mediolaterally narrow and, where preserved, is circular in cross-section (Fig. 4a, b).

Fig. 4. Cervical and pectoral vertebrae of plesiosaurians from the Toarcian (and (h) upper Pliensbachian) stages of Eastern Siberia. (a–c) Microcleidus sp.: (a) ZIN PH 86/211, (b) SPbU VZ R Sp22c and (c) SPbU VZ R Sp36. (d–g) Cervical and pectoral vertebrae of ?Microcleididae indet.: (d) pectoral vertebra ZIN PH 5/211; cervical centra of juveniles (e) ZIN PH 87/211, (f) ZIN PH 14/211 and (g) ZIN PH 16/211. (h, i, k, n–p) Cervical vertebrae of pliosaurids, similar to Hauffiosaurus: (h) ZIN PH 6/211, (i) ZIN PH 67/211, (k) ZIN PH 109/211, (n) SPbU VZ R Sp22b, (o) SPbU VZ R Sp31 and (p) SPbU VZ R Sp22a. (j, l, m) Cervical vertebrae of Rhomaleosauridae indet.: (j) ZIN PH 68/211, (l) ZIN PH 83/211 and (m) ZIN PH 15/211. Numbers indicate the following views: 1, anterior view; 2, lateral view; 3, ventral view; 4, posterior view for (a–c) and dorsal view for (e–g, i–l); 5, dorsal view for (a–c). Scale bars: 5 cm.
Considering a unique combination of their proportions, platycoelous type, complete fusion of neural arch and ribs, bipartite rib articulation, lack of ventral keel and particularly the presence of lateral keels, these vertebrae could be referred to Microcleidus Watson, Reference Watson1909 sensu Benson et al. (Reference Benson, Evans and Druckenmiller2012) (see also Vincent et al. Reference Vincent, Weis, Kronz and Delsate2019).
A number of small cervical centra and one pectoral centrum belong to osteologically immature and likely juvenile plesiosaurians (Fig. 4e–g; online Supplementary Fig. S5). This is suggested based on their small size (the width of the largest specimen, ZIN PH 14/211, is 47 mm; the length is 31 mm) and that the neural arches and ribs are not fused to the centrum (criterion of immaturity sensu Brown, Reference Brown1981, see also Vincent, Reference Vincent2010; Vincent et al. Reference Vincent, Allemand, Taylor, Suan and Maxwell2017). The description and additional figures of these centra are provided in online Supplementary Material S1. Based on their general proportions, platycoelous type and absence of ventral keel, these cervical and pectoral centra (online Supplementary Fig. S5) are somewhat conventionally referred to as Microcleididae indet.; however, their affinity to other plesiosauroids – for example, Plesiopterys wildi O’Keefe, Reference O’Keefe2004, recovered as a sister taxon to derived plesiosauroids by some researchers (Benson et al. Reference Benson, Evans and Druckenmiller2012), despite being considered a junior subjective synonym of the microcleidid Seeleyosaurus guilelmiimperatoris (Dames, Reference Dames1895) by Großmann (Reference Großmann2007) – cannot be excluded, but requires additional data on the vertebral morphology and phylogenetic relations of the Early Jurassic plesiosaurians.
Nine cervical vertebrae are characterized by markedly concave articular surfaces (commonly referred to as amphicoelous type), the presence of the ventral keel and proportional shortening (Fig. 4h–p; online Supplementary Fig. S6). Based solely on these features, the specimens could be referred to Rhomaleosauridae and/or Pliosauridae (e.g. Benson et al. Reference Benson, Evans and Druckenmiller2012) or to basal plesiosaurians with somewhat uncertain phylogenetic position, such as Anningasaura or Lindwurmia (Vincent & Benson, Reference Vincent and Benson2012; Vincent & Storrs, Reference Vincent and Storrs2019).
Among those, vertebrae ZIN PH 6/211 (Fig. 4h), ZIN PH 67/211 (Fig. 4i), ZIN PH 109/211 (Fig. 4k) and SPbU VZ R Sp31 (Fig. 4o) are similar in their proportions and morphology. They are slightly shorter anteroposteriorly than high dorsoventrally and slightly broader mediolaterally than dorsoventrally. ZIN PH 67/211 (Fig. 4i) is the smallest among these and lacks its neural arch that was not fused to the centrum. Ventrally, it has a well-pronounced, sharp ventral keel. The ventral foramina are small and located close to the median keel (Fig. 4i3). The area adjacent to anterior and posterior articular surfaces is rugose ventrally. Dorsally, the floor of the neural canal is hourglass-shaped; the neural arch facets are somewhat lenticular. In lateral view, the neural arch facets form V-shaped notches and contact the diapophysis of the rib facet (Fig. 4i2; this feature, when occurred along the entire length of the neck, is suggested to be a synapomorphy of Hauffiosaurus O’Keefe, Reference O’Keefe2001 and included in the diagnosis of the genus; Benson et al. Reference Benson, Ketchum, Noé and Gómez-Pérez2011). The rib facet of ZIN PH 67/211 is bipartite with its dorsal part triangular in outline and twice smaller than the ventral part, which is elongated oval in outline, nearly as long as the centrum length (Fig. 4i2). The two parts are deeply concave and are separated by a thin longitudinal ridge. Other three specimens (ZIN PH 6/211, ZIN PH 109/211 and SPbU VZ R Sp31) differ in their larger size and in having neural arches and ribs completely fused to the centrum. The ventral keel in these specimens is less sharp than in ZIN PH 67/211, but also narrow and plate-like and has closely located and relatively small ventral foramina (Fig. 4h3, k3, o3). The prezygapophyses preserved in SPbU VZ R Sp31 were nearly as wide as the centrum mediolateral width. Following the orientation of bone texture, and considering the presence of a vertical eminence between the rib and the neural arch, it could be suggested that these two elements are articulated.
The above-described cervical centra are very similar in their morphology to the cervical vertebrae of the pliosaurid Hauffiosaurus (White, Reference White1940; Vincent, Reference Vincent2011; Benson et al. Reference Benson, Ketchum, Noé and Gómez-Pérez2011). The neural arch pedicles contacting the diapophysis of the rib facet along the entire length of the neck, was suggested to be a synapomorphy of Hauffiosaurus and included in the diagnosis of the genus (Benson et al. Reference Benson, Ketchum, Noé and Gómez-Pérez2011). Later Benson et al. (Reference Benson, Evans and Druckenmiller2012) reported that the condition with this contact occurs in some cervical vertebrae of a basal pliosaurid Thalassiodracon hawkinsii (Owen, Reference Owen1838) and a similar condition was later reported for a Triassic plesiosaurian Rhaeticosaurus mertensi Wintrich et al. Reference Wintrich, Hayashi, Houssaye, Nakajima and Sander2017, which was also recovered within Pliosauridae (Wintrich et al. Reference Wintrich, Hayashi, Houssaye, Nakajima and Sander2017). Given that the studied vertebrae are isolated, it is impossible to unambiguously define their relative position in the neck. We also cannot be sure that they all belong to one taxon; it is therefore impossible to say if this contact of the neural arch pedicle and rib occurred along the entire length of the neck. We therefore refer these specimens to Pliosauridae indet., although their affinity to Hauffiosaurus is possible.
Vertebrae SPbU VZ R Sp22b (Fig. 4n) and SPbU VZ R Sp22a (Fig. 4p) are larger than the above-described vertebrae. The mediolateral width of SPbU VZ R Sp22a is 65 mm and it is unambiguously from the posterior part of the cervical series, closest to the pectoral region, with widely spaced ventral foramina (Fig. 4p3), reduced diapophyses and enlarged parapophyses connected to the neural arch by the vertical ridge (Fig. 4p2). The mediolateral width of SPbU VZ R Sp22b is 54 mm. It has an incompletely developed ventral keel disrupted in the middle (Fig. 4n3) and relatively closely spaced ventral foramina, but overall is similar to the above-described morphotype. Considering the proportional elongation of these two centra (anteroposterior length is only slightly less than the dorsoventral height), we are inclined to refer them to basal pliosaurids rather than to rhomaleosaurids.
Specimens ZIN PH 68/211 (Fig. 4j), ZIN PH 83/211 (Fig. 4l) and ZIN PH 15/211(Fig. 4m) are cervical centra that are anteroposteriorly shortened relative to their nearly equal mediolateral width and dorsoventral height. The neural arch facets are deeply concave and form a V-shaped notch in lateral view, but not reaching the rib facet (Fig. 4l2). The rib facets are relatively large and semicircular in outline. They are separated into two nearly equal parts by the longitudinal ridge. In ventral view, there is a pair of well-pronounced circular foramina, each situated in a squared depression, constricted medially by a ventral keel and laterally by a raised border of the rib facet. This condition is typical of rhomaleosaurids (e.g. Smith & Vincent, Reference Vincent2010; Smith & Benson, Reference Smith and Benson2014; Smith & Araujo, Reference Smith and Araujo2017). The ventral keel is less sharp than in the above-described pliosaurid vertebrae. Given the described traits, the rhomaleosaurid attribution of these three specimens is most plausible.
Five dorsal vertebrae (ZIN PH 92/211, 91/211, 90/211, 50/211 and SPbU VZ R Sp30) are characteristically shortened anteroposteriorly (length to width ratio, 0.55) and have distinctly concave articular surfaces with raised rims (Fig. 5a, b); for descriptions, please see online Supplementary Material S1. Considering that the anteroposteriorly shortened and amphicoelous pectoral and dorsal vertebrae are reported for both basal pliosaurids (White, Reference White1940 sensu Benson et al. Reference Benson, Ketchum, Noé and Gómez-Pérez2011; Sachs & Kear, Reference Sachs and Kear2018) and derived rhomaleosaurids (e.g. Smith & Benson, Reference Smith and Benson2014), these vertebrae could be referred to both these groups. Further study of the axial skeleton of plesiosaurians is required for clarification of their affinity.

Fig. 5. Dorsal, pectoral and/or sacral and caudal vertebrae as well as partial pelvis of Plesiosauria indet. from the Toarcian Stage of Eastern Siberia. (a, b) Dorsal vertebrae of pliosaurids and/or rhomaleosaurids: (a) ZIN PH 92/211 and (b) ZIN PH 90/211. (c) Caudal centrum of pliosaurid or rhomaleosaurid ZIN PH 107/211. (d–g) Dorsal vertebrae of Plesiosauria indet.: (d) ZIN PH 17/211, (e) ZIN PH 66/211, (f) SPbU VZ R Sp33 and (g) SPbU VZ R Sp34. (h) Partial dorsal series of Plesiosauria indet. SPbU VZ R Sp11 in right lateral view. (i) Concretion with associated sacral vertebrae and partial pelvis SPbU VZ R Sp38: (i1) sacral vertebra in anterior articular view, ilium in lateral view; and (i2) sacral vertebra in left lateral view, ilium, ischium and pubis in ventral view. (j) Sacral vertebra ZIN PH 65/211. (k) Anterior dorsal vertebra ZIN PH 108/211. (l) Pectoral vertebra ZIN PH 84/211. (m) Caudal vertebra ZIN PH 46/211. Numbers indicate the following views: 1, articular view for (a–g, i–m); 2, lateral view; 3, dorsal view for (a–e) and ventral view for (j–m); 4, ventral view for (a–c) and posterior view for (d). Scale bar: 5 cm.
Of certain interest are the specimens SPbU VZ R Sp11 and Sp38, representing the articulated plesiosaurian remains from the uppermost Toarcian to lowermost Aalenian strata of the Markha River (Fig. 5h, i). SPbU VZ R Sp11 is a part of the dorsal vertebral column consisting of six articulated vertebrae. The morphology of these vertebrae is highly similar to that of the isolated vertebrae depicted in Figure 5d–g. The vertebrae of this morphotype are characterized by the articular surfaces of ovoid (or somewhat heart-shaped) outline; the centra are nearly as dorsoventrally high as mediolaterally wide, being widest in the dorsal half and constricting in width ventrally. The anteroposterior length is less than the mediolateral width, but it is not as shortened as in the above-described morphotype of dorsal vertebrae (length to width ratio, 0.61–0.80; compare with the value of 0.55 in the morphotype described in the previous paragraph).
Specimen SPbU VZ R Sp38 comprises associated sacral vertebrae and a partial pelvis. The sacral vertebrae have circular articular surfaces (Fig. 5i1) and bear extensive dorsoventrally elongated rib facets divided between the centrum and the neural arch (Fig. 5i2). The acetabular surface of the pelvis is irregularly pitted and relatively dorsoventrally thick. The proximal pubis is convex and tapered laterally and posteriorly. Its ischial facet of the pubis is well demarcated from the acetabular contribution, forming an angle close to 90°. The acetabular head of the ischium is massive and it has three clearly demarcated facets. The ischial neck, which connected the acetabular head and the ischial blade (not preserved), is relatively slender and has concave anterior and posterior edges (the ischial neck to acetabular process ratio is 0.5). The ilium is a slender and curved element articulated to the ischium. The proximal end of the ilium is expanded transversely, forming the ischial facet and iliac portion of the acetabulum, squared in articular outline (Fig. 5i2). Although both these specimens lack diagnostic features and could be assigned only as Plesiosauria indet., they are significant indicators that provide encouragement to search for articulated skeletal remains in the Lower Jurassic Series of the Vilyuy River basin.
Specimens ZIN PH 32/211 and SPbU VZ R Sp8 are two parts of a large and robust dorsal rib (Fig. 6q) that is 40 mm in maximum diameter. This is the only specimen from the Linde River, tributary of the Lena River and is late Toarcian in age. Specimen ZIN PH 26/211 is interpreted as a sacral rib in having expanded and strongly compressed proximal and distal ends with their long axes twisted relative to each other at approximately 90° (Fig. 6p). One of the ends bears an elongated depression in its middle (Fig. 6p1), similar to that present on proximal ends of sacral ribs in Rhomaleosaurus (Smith & Benson, Reference Smith and Benson2014). The preserved proximodistal length of this rib is 125 mm. These large ribs indicate the presence of large-bodied plesiosaurians during late Toarcian time in Siberia. They are here referred to as indeterminate plesiosaurians; however, it is possible that they belonged to rhomaleosaurids since other plesiosaurian groups, including pliosaurids, were smaller during the Toarcian Age (e.g. Benson et al. Reference Benson, Evans and Druckenmiller2012).

Fig. 6. Elements of the appendicular skeleton and ribs of plesiosaurians from the Toarcian of Eastern Siberia. Distal parts of the propodial bones: (a) ZIN PH 11/211, (b) ZIN PH 97/211, (d) ZIN PH 51/211 and (e) ZIN PH 96/21 in (a1, b1, c1, d1) dorsal or ventral, (a2, d2) anterior, (b2) posterior and (a3, b3, d3, e2) distal views. (c) Proximal part of the humerus ZIN PH 12/211 in (c1) dorsal and (c2) proximal views. Radius or tibia (f) ZIN PH 13/211 and (h) SPbU VZ R Sp29 in (f1, h1) dorsal or ventral, (f2, h2) anterior and (f3, h3) proximal views. (g) Ulna or fibula ZIN PH 36/211 in (g1) dorsal or ventral, (g2) anterior and (g3) proximal views. (i) Right ischium, ZIN PH 37/211, in (i1) ventral, (i2) medial and (i3) acetabular views. (j) Acetabular portion of the pathologic ischium ZIN PH 95/211 in (j1) ventral and (j2) acetabular views. (k–o) Propodial bones of juvenile and/or infant plesiosaurians: (k) ZIN PH 21/211 in (k1) ventral or dorsal, (k2) anterior and (k3) distal views; (l) proximal part ZIN PH 25/211 in (l1) ventral and (l2) proximal views; (m) ZIN PH 119/211 in (m1) dorsal or ventral and (m2) proximal views; (n) ZIN PH 100/211 in (n1) dorsal or ventral and (n2) distal views; (o) ZIN PH 104/211 in dorsal or ventral view. (p) Sacral? rib ZIN PH 26/211 in different views. (q) Dorsal rib (q1) ZIN PH 32/211 and (q2, q3) SPbU VZ R Sp8. The lines in g1, i1 and i3 show the hypothetically reconstructed outlines of the elements. Scale bar: 10 cm.
Numerous plesiosaurian vertebrae from the pectoral, dorsal, sacral and caudal regions as well as elements of the appendicular skeleton are difficult to attribute to anything more precise than Plesiosauria indet. In this regard, we reduce their description in the main text and direct the reader to online Supplementary Material S1 for details. Some of these specimens are depicted in Figures 5 and 6.
Several propodial elements in the studied material are relatively small and osteologically immature, with poorly ossified distal and proximal ends, including poorly demarcated distal facets and, most importantly, poorly demarcated capitulum and dorsal trochanter proximally (Fig. 6k–m). These specimens are identified as belonging to juvenile and/or infant animals (for juvenile criteria see e.g. Brown, Reference Brown1981). From these, the smallest propodial (ZIN PH 21/211) is 104 mm in proximodistal length (Fig. 6k) and the largest (ZIN PH 119/211) is 182 mm long (Fig. 6m). During the preparation of ZIN PH 119/211 it was possible to observe the bone structure in the diaphysis cross-section. The observed cortical bone was exclusively radially vascularized, similar to the condition reported for fetal polycotylids (O’Keefe et al. Reference O’Keefe, Sander, Wintrich and Werning2019). This further supports the identification of the early ontogenetic stage of the specimen.
5. Discussion
5.a. Note on palaeogeography of Early Jurassic marine reptiles
The history of opinions on the palaeogeography of Early Jurassic marine reptiles is complex. The unambiguous occurrences of Ichthyosaurus, a genus typical of the Lower Lias of the UK, in the Sinemurian of São Pedro de Moel, Portugal (Zbyszewski & Moitinho de Almeida, Reference Zbyszewski and Moitinho de Almeida1952; Bardet et al. Reference Bardet, Pereda Suberbiola and Ruiz Omeñaca2008) and Alberta, Canada (McGowan, Reference McGowan1978) were the first direct evidence that some genera of Early Jurassic marine reptiles had a global distribution (McGowan, Reference McGowan1978). Otero & Sepúlveda (Reference Otero and Sepúlveda2020) recently reported on the first find of Temnodontosaurus, another ichthyosaurian genus previously known exclusively from Europe, in the Hettangian–Sinemurian stages of the Atacama Desert, South America, further emphasizing the global distribution of Early Jurassic marine reptile taxa. In this regard, it is somewhat rational to expect the occurrences of other well-known European marine reptile taxa in coeval strata of other regions. However, even for the basins of Western Europe, it was believed that several biogeographic provinces for marine reptiles existed during the Early Jurassic Epoch. Although the palaeogeographic reconstructions of the Early Jurassic European Archipelago imply no insurmountable barriers between the basins, and invertebrate faunas do not show geographic provincialism (e.g. Dera et al. Reference Dera, Neige, Dommergues and Brayard2011), several authors have previously suggested faunal differentiation for marine reptiles, including ichthyosaurians, plesiosaurians and thalattosuchians (Godefroit, Reference Godefroit1994; Maisch & Ansorge, Reference Maisch and Ansorge2004; O’Keefe, Reference O’Keefe2004; Großmann, Reference Großmann2007; Smith & Vincent, Reference Vincent2010). It is only recently that these suggestions were questioned by taxonomic revisions and new discoveries (Benson et al. Reference Benson, Ketchum, Noé and Gómez-Pérez2011; Fischer et al. Reference Fischer, Guiomar and Godefroit2011; Reisdorf et al. Reference Reisdorf, Maisch and Wetzel2011; Vincent et al. Reference Vincent, Martin, Fischer, Suan, Khalloufi, Suchéras-Marx, Lena, Janneau, Rousselle and Rulleau2013 b, 2019; Sachs et al. Reference Sachs, Hornung, Lierl, Kear, Kear, Lindgren, Hurum, Milàn and Vaida2016).
Our identifications of ichthyosaurian genera Temnodontosaurus and Stenopterygius, as well as plesiosaurian genus Microcleidus, which all are typical for the European Lower Jurassic Series (e.g. McGowan & Motani, Reference McGowan and Motani2003; Benson et al. Reference Benson, Evans and Druckenmiller2012), in the upper Lower Jurassic Series of Siberia provide evidence of a herpetofaunal exchange between the NW Tethys and the Boreal Sea during the Early Jurassic Epoch. This is further supported by the presence of indeterminate rhomaleosaurids and pliosaurids similar to Hauffiosaurus, indicating that the major Early Jurassic ichthyosaurian and plesiosaurian clades were widely distributed.
According to palaeogeographical reconstruction (Fig. 1a), during the Early Jurassic Epoch, the NW Tethys (that corresponded to an epicontinental sea) and the Boreal Sea were directly connected by a narrow seaway named the ‘Viking Corridor’, a part of the Greenland–Norwegian Seaway alternatively called ‘north–south Laurasian Seaway’ (Ziegler, Reference Ziegler1988; Korte et al. Reference Korte, Hesselbo, Ullmann, Dietl, Ruhl, Schweigert and Thibault2015). This seaway was operational during Sinemurian–Toarcian times (Doré, Reference Doré1991). It was closed during the earliest Middle Jurassic Epoch, resulting in the isolation of the Boreal Sea and strongly reduced heat transport to the Arctic regions (Doré, Reference Doré1991; Korte et al. Reference Korte, Hesselbo, Ullmann, Dietl, Ruhl, Schweigert and Thibault2015). It is most likely that the Greenland–Norwegian Seaway was used by marine reptiles as the main route for distribution between the NW Tethys and the Boreal Sea during the Early Jurassic Epoch, as in the case of other fossil groups (Nikitenko & Mickey, Reference Nikitenko, Mickey, Beaudoin and Head2004; Zakharov et al. Reference Zakharov, Shurygin, Il’ina and Nikitenko2006; van de Schootbrugge et al. Reference van de Schootbrugge, Houben, Ercan, Verreussel, Kerstholt, Janssen, Nikitenko and Suan2020).
5.b. Marine reptiles at high latitudes
Recent progress in the study of palaeobiology of Mesozoic marine reptiles significantly changed our understanding of these animals. Isotopic evidence has suggested homeothermy for plesiosaurians, ichthyosaurians and mosasaurians (Bernard et al. Reference Bernard, Lécuyer, Vincent, Amiot, Bardet, Buffetaut, Cuny, Fourel, Martineau, Mazin and Prieur2010; Harrell et al. Reference Harrell, Perez-Huerta and Suarez2016). Moreover, for ichthyosaurians, anatomical adaptation for cold resistance such as insulating blubber was reported (Lindgren et al. Reference Lindgren, Sjövall, Thiel, Zheng, Ito, Wakamatsu, Hauff, Kear, Engdahl, Alwmark, Eriksson, Jarenmark, Sachs, Ahlberg, Marone, Kuriyama, Gustafsson, Malmberg, Thomen, Rodríguez-Meizoso, Uvdal, Ojika and Schweitzer2018). Although live birth is well documented for ichthyosaurians (e.g. Böttcher, Reference Böttcher1990), evidence of live birth in plesiosaurians and mosasaurians was only reported more recently (O’Keefe & Chiappe, Reference O’Keefe and Chiappe2011; Field et al. Reference Field, LeBlanc, Gau and Behlke2015). Furthermore, it is now considered that, based on histological evidence, at least some plesiosaurians had very high fetal growth rates and large birth size (O’Keefe & Chiappe, Reference O’Keefe and Chiappe2011; Wintrich et al. Reference Wintrich, Hayashi, Houssaye, Nakajima and Sander2017; Fleischle et al. Reference Fleischle, Wintrich and Sander2018; O’Keefe et al. Reference O’Keefe, Sander, Wintrich and Werning2019). Rapid postnatal growth and additional histological evidence for high metabolic rates was also reported for derived ichthyosaurians (Buffrénil & Mazin, Reference Buffrénil and Mazin1990; Houssaye, Reference Houssaye2013; Houssaye et al. Reference Houssaye, Scheyer, Kolb, Fischer and Sander2014). In summary, this evidence implies that many derived marine reptiles (including plesiosaurians and neoichthyosaurians) could have been capable of living and reproducing in a relatively cold environment, but also indicates that they were fast-swimming and active animals capable of long-range movements.
Differing from abundant plesiosaurian and ichthyosaurian occurrences, at present there are no findings of thalattosuchian crocodilomorph remains in Siberia. This contrasts with the Lower Jurassic localities of Western Europe and elsewhere at low palaeolatitudes (Table 1), where teleosaurid thalattosuchian remains are common. This is consistent with the suggestion that non-metriorhynchoid thalattosuchian biodiversity was linked to temperature (Martin et al. Reference Martin, Amiot, Lécuyer and Benton2014; although see Mannion et al. Reference Mannion, Benson, Carrano, Tennant, Judd and Butler2015). The recent study of thalattosuchian termophysiologies based on the oxygen isotope composition in their tooth apatite (Séon et al. Reference Séon, Amiot, Martin, Young, Middleton, Fourel, Picot, Valentin and Lécuyer2020) suggests that teleosaurid thalattosuchians were ecto-poikilothermic and further supports the ideas of climatic constraints for their distribution. In our opinion, the absence of thalattosuchian remains from Early Jurassic Siberia is indirect evidence that this group, in contrast to neoichthyosaurians and plesiosaurians, was unable to survive in the polar environment even during the summer period, or that their biology precluded the possibility of long-term seasonal migrations.
Of great interest is the discovery of remains belonging to juvenile (and possibly infant) plesiosaurians in Siberia. The findings of juvenile plesiosaurian remains has been previously reported for high-latitude Cretaceous localities representing the shallow, nearshore and even non-marine deposits (Wiffen et al. Reference Wiffen, De Buffrénil, De Ricqlès and Mazin1995; Martin, Reference Martin2002; Kear, 2006a, b, Reference Kear2007; Martin et al. Reference Martin, Sawyer, Reguero and Case2007; Campbell et al. Reference Campbell, Schröder-Adams, Haggart, Druckenmiller, Ryan and Zazula2013; Vavrek et al. Reference Vavrek, Wilhelm, Maxwell and Larsson2014; Rogov et al. Reference Rogov, Zverkov, Zakharov and Arkhangelsky2019). The presence of juvenile and possibly infant plesiosaurians during the Early Jurassic Epoch in the shallow Vilyuy Bay of the Boreal Sea is further evidence that these animals may have used the Vilyuy Bay as a breeding area, as was similarly suggested for several shallow high-latitude basins of the Cretaceous period (Wiffen et al. Reference Wiffen, De Buffrénil, De Ricqlès and Mazin1995; Martin, Reference Martin2002; Kear, Reference Kear2006 a, Reference Kear2007; Martin et al. Reference Martin, Sawyer, Reguero and Case2007; Vavrek et al. Reference Vavrek, Wilhelm, Maxwell and Larsson2014). Indeed, the environment in the Boreal Sea during the polar summer with continuous daylight as well as dynamic and nutrient conditions may result in high productivity, which is evidenced by data on phytoplankton (e.g. van de Schootbrugge et al. Reference van de Schootbrugge, Houben, Ercan, Verreussel, Kerstholt, Janssen, Nikitenko and Suan2020), and therefore favourable conditions for nektonic animals, including cephalopods, fishes and marine reptiles. This might be a favourable breeding ground and nursery area. Similarly, the Cretaceous low-latitude marine reptile assemblages, which include individuals of different ontogenetic stages, were suggested to be attributed to upwelling-related environments of high nutrient input and food availability (Vincent et al. Reference Vincent, Bardet, Houssaye, Amaghzaz and Meslouh2013 a; Martin et al. Reference Martin, Vincent, Tacail, Khaldoune, Jourani, Bardet and Balter2017).
The climates of late Early Jurassic Siberia were varying. Significant cooling has been inferred for the late Pliensbachian age based on glendonite and dropstone occurrence, as well as palynological data and the composition of benthic invertebrate fauna (e.g. Zakharov et al. Reference Zakharov, Shurygin, Il’ina and Nikitenko2006; Rogov et al. Reference Rogov, Zverkov, Zakharov and Arkhangelsky2019). In contrast, a temperature maximum during early Toarcian time is evidenced by palynological data (e.g. Vakhrameev, Reference Vakhrameev1987). However, this short warming episode rapidly ended with the drop in temperature of the late Toarcian age that continued into the earliest Middle Jurassic Epoch, inferred from the closure of the Viking Strait and thus the strong reduction of heat transport to the isolated Boreal Sea (Korte et al. Reference Korte, Hesselbo, Ullmann, Dietl, Ruhl, Schweigert and Thibault2015; Rogov et al. Reference Rogov, Zverkov, Zakharov and Arkhangelsky2019). Although the marine reptile remains are indeed most abundant in the Toarcian Stage, their occurrence in the upper Pliensbachian deposits, as well as throughout the Toarcian strata in all of its ammonite zones (including the Toarcian–Aalenian boundary interval; Fig. 2), implies their regular presence in the Boreal Sea, regardless of the varying climate. The question remains whether marine reptiles inhabited the Boreal Sea year-round, or whether they undertook seasonal migrations.
Many extant marine animals migrate in the oceanic environment (e.g. Luschi, Reference Luschi2013); this is especially common for pelagic amniotes such as sea turtles (e.g. Broderick et al. Reference Broderick, Coyne, Fuller, Glen and Godley2007; Southwood & Avens, Reference Southwood and Avens2009) and cetaceans (e.g. Wells et al. Reference Wells, Rhinehart, Cunningham, Whaley, Baran, Koberna and Costa1999; Boyd, Reference Boyd and Werner2004; Rasmussen et al. Reference Rasmussen, Palacios, Calambokidis, Saborío, Dalla, Secchi, Steiger, Allen and Stone2007; Bailey et al. Reference Bailey, Mate, Palacios, Irvine, Bograd and Costa2010; Stevick et al. Reference Stevick, Neves, Johansen, Engel, Allen, Marcondes and Carlson2011; Durban & Pitman, Reference Durban and Pitman2012; Bröker et al. Reference Bröker, Gailey, Tyurneva, Yakovlev, Sychenko, Dupont, Vertyankin, Shevtsov and Drozdov2020), which could be considered the closest modern analogues of Mesozoic marine reptiles. In the case of Mesozoic marine reptiles, the major constraint for the permanent presence at high latitudes is not the seasonal change of the climate, but the condition of polar night (Grigoriev & Grabovsky, Reference Grigoriev and Grabovsky2020). The palaeolatidue calculations for marine reptile localities in the Vilyuy River basin (Table 2) indicate that during the Pliensbachian and Toarcian ages the Vilyuy Bay of the Boreal Sea (Fig. 1) was located very close to the palaeo north pole (over the 80th latitude). Disregarding the cyclic oscillation of Earth’s obliquity and axial precession for a long time interval such as 10 Ma (late Pliensbachian – late Toarcian time), the general pattern of light distribution at latitudes above 80th implies 4.7 (up to 6) months of continuous daylight and no less than 3 months of the polar night, with an abrupt transition between the two conditions including a month of twilight (e.g. Berge et al. Reference Berge, Renaud, Darnis, Cottier, Last, Gabrielsen, Johnsen, Seuthe, Weslawski, Leu, Moline, Nahrgang, Søreide, Varpe, Lønne, Daase and Falk-Petersen2015). The significant drop in primary production during the polar night apparently results in the consequent reduction of potential feeding habitats for large amniotes. Furthermore, the survival of marine reptiles in complete darkness for nearly half a year will require some specific adaptations for hunting in such harsh conditions, whether night vision or echolocation, or any sensory organs that enable hunting in complete darkness. An alternative for marine reptiles to overcome long-term starvation at high latitudes during the polar night could be dormancy (hibernation/brumation); however, this is difficult to assume for air-breathing pelagic animals. Considering the occurrence of various plesiosaurian and ichthyosaurian taxa at high palaeolatitudes, these assumptions will further imply multiple origins of those hypothetical adaptations, which is hard to verify based on fossil material. Moreover, the evidence of glaciations during late Pliensbachian time (glendonites and dropstones) indicates that, at least for some part of the history of marine reptile presence at high latitudes, there were possibly additional winter barriers (sea ice coverage) critical for these air-breathing animals. In summary, it seems unlikely that marine reptiles lived permanently in the contrasting polar environment. A more parsimonious and plausible scenario, in our opinion, is that marine reptiles migrated to the Boreal Sea in spring and returned in the autumn to lower latitudes.
Acknowledgements
We thank TA Kirina (who passed away in 2000) and VG Borkhvardt (SPbU VZ) who collected the specimens in Siberia and transferred them to SPbU VZ and ZIN PH during 1958–1964. We are grateful to PP Skutschas, DD Vitenko, IT Kuzmin (SPbU VZ) and R Schellhorn (Institute of Geosciences, Bonn) who collected additional specimens during the SPbU expedition in 2019 and to II Malyshev (local resident from Suntar) who handed over the specimen he found. PP Skutschas and other colleagues from SPbU VZ are thanked for hospitality during NGZ visits. Alexander Averianov (ZIN) is thanked for his help in obtaining the financial support for this project. MA Rogov (Geological Institute, Moscow) is thanked for help with the literature and consultations on the Jurassic stratigraphy. We thank M Tanimoto (independent researcher, Japan) for providing valuable information on the Early Jurassic marine reptiles of Japan. The review of Peggy Vincent was beneficial for this work. We also thank the editor and two anonymous reviewers for their influential efforts in bringing this contribution into a more laconic form. We thank the copy-editor Elaine Rowan for valuable stylistic corrections. Financial support was provided by the Russian Foundation for Basic Research (project no. 19-34-50032, ‘Early Jurassic marine reptiles of Siberia’).
Conflict of Interest
None.
Supplementary material
To view supplementary material for this article, please visit https://doi.org/10.1017/S0016756820001351